DOI:
10.1039/C9RA09887A
(Paper)
RSC Adv., 2020,
10, 7221-7231
Retracted Article: CircBANP acts as a sponge of let-7a to promote gastric cancer progression via the FZD5/Wnt/β-catenin pathway†
Received
26th November 2019
, Accepted 22nd January 2020
First published on 18th February 2020
Abstract
Gastric cancer (GC) is one of the leading causes of cancer-related deaths in our country. Circular RNAs (circRNAs) are being found to have relevance to human cancers, including GC. The purpose of this study was to investigate the functional role of circRNA BTG3 associated nuclear protein (circBANP) in GC and underlying mechanisms governing it. CircBANP was identified using RNase R assay and polymerase chain reaction (PCR) with specific primers. The levels of circBANP, let-7a and Frizzled-5 (FZD5) mRNA were assessed by quantitative real-time PCR (qRT-PCR). Cell proliferation, colony formation ability, apoptosis, and migration and invasion were determined by Cell Counting Kit-8 (CCK-8) assay, colony formation assay, flow cytometry, transwell assay, respectively. The targeted interaction between let-7a and circBANP or FZD5 was confirmed by dual-luciferase reporter assay or RNA pull-down assay. Western blot analysis was performed to detect the indicated protein expression. A xenograft model assay was established to observe the role of circBANP in vivo. We found that circBANP was up-regulated in GC tissues and cell lines, and associated with clinicopathologic features of GC patients. CircBANP knockdown repressed the proliferation, migration, invasion, and promoted the apoptosis in GC cells. CircBANP sequestered let-7a by acting as a molecular sponge of let-7a. Moreover, the regulatory effect of circBANP on GC cell progression in vitro was mediated by let-7a. CircBANP protected against FZD5 repression by sponging let-7a in GC cells. Wnt/β-catenin signaling was involved in the regulatory network of the circBANP/let-7a axis in GC cell progression. Additionally, circBANP depletion retarded tumor growth in vivo. In conclusion, our study suggested that the knockdown of circBANP suppressed GC cell progression in vitro and in vivo at least partially through sponging let-7a and regulating FZD5/Wnt/β-catenin signaling, providing a novel mechanism for understanding the pathogenesis of GC.
1 Introduction
Gastric cancer (GC) remains an important cancer worldwide, with an estimated more than 1
000
000 new cases and 783
000 deaths in 2018.1 Despite a decline in mortality and despite significant advances in the therapeutic methods, the burden remains very high.2,3 A better understanding of GC pathology and molecular mechanisms is urgently needed for improving GC treatment.
Circular RNAs (circRNAs), a novel type of non-coding stable transcripts, form covalently closed loop structures with neither 5′ to 3′ ends nor poly-adenylated (pA) tails.4,5 Emerging evidence has shown that circRNAs are related to the development and progression of human diseases, especially in cancers.6,7 Recent studies have reported that circRNAs serve as crucial regulators in GC, highlighting their roles as GC diagnostic and prognostic biomarkers and therapeutic targets.8,9 As for circRNA BTG3 associated nuclear protein (circBANP), derived from exon 5–11 of BANP gene, it had been identified to be up-regulated in colorectal cancer (CRC) and lung cancer.10,11 Moreover, Zhu et al. reported that circBANP depletion triggered a significant repression of cell proliferation in CRC cells.10 Han and colleague validated that the knockdown of circBANP suppressed lung cancer cell proliferation, invasion, and migration in vitro and weakened tumor growth in vivo.11 However, the functional role and underlying mechanism of circBANP in GC progression remain undefined.
MicroRNAs (miRNAs) are endogenous, small non-coding RNA molecules with ∼22 nucleotides long that play vital roles in a broad array of physiopathology processes.12,13 They regulate gene expression through pairing to the 3′-untranslated regions (3′-UTR) of specific mRNA targets to direct their repression. Previous reports had demonstrated that let-7a was down-regulated in GC and implicated in GC tumorigenesis and progression.14,15 Recently, competing endogenous RNA (ceRNA) hypothesis proposes that circRNAs modulate gene expression by functioning as efficient sponges of specific miRNAs, illuminating the importance of such interactions in cancers.16 The database of software algorithms predicted a potential ceRNA regulatory network of circBANP/let-7a/Frizzled-5 (FZD5) axis. In this study, we aimed to investigate the detailed role of circBANP in GC progression in vitro and in vivo and the molecular mechanism governing it. We found that the knockdown of circBANP repressed GC progression at least partially through let-7a/FZD5/Wnt/β-catenin pathway.
2 Materials and methods
2.1. Clinical specimens and cell culture
This study used 112 tissue specimens including 56 matched pairs of GC tissues and corresponding normal gastric tissues from 56 GC patients from the First Affiliated Hospital of Zhengzhou University, without any treatment before surgery. Tissue specimens were collected from these patients when gastrectomy was performed and stored at −80 °C until use. The study was approved by the Institutional Ethics Committee of the First Affiliated Hospital of Zhengzhou University in accordance with the Declaration of Helsinki Principles. Informed consents were obtained from all participants.
Four GC cell lines (MKN-45, MKN-74, AGS and HGC-27) and human normal gastric epithelial GES-1 cell line were obtained from the China Center for Type Culture Collection (CCTCC, Wuhan, China). All cell lines were maintained at 37 °C, 5% CO2 in RPMI-1640 medium (Life Technologies, Bleiswijk, The Netherlands) plus 10% fetal bovine serum (Bodinco, Alkmaar, The Netherlands), 1% penicillin/streptomycin (Solarbio, Beijing, China).
2.2. RNA extraction and RNase R digestion
Total RNA was isolated from GC tissues and cells using TRIzol reagent (Invitrogen, Karlsruhe, Germany) referring to the producer's guidance. For RNase R digestion, total RNA (100 μg) was treated with RNase R (3 U mg−1, Epicenter Technologies, Madison, WI, USA) at 37 °C for 15 min, followed by the purification using the RNeasy MinElute Cleaning Kit (Qiagen, Hiden, Germany), according to the protocols of manufacturers.
Reverse transcription and quantitative real-time polymerase chain reaction. Complementary DNA (cDNA) synthesis from total RNA (100 μg) was implemented with random hexamer primers using a High Capacity cDNA Reverse Transcription Kit (Thermo Fisher Scientific, Leiden, The Netherlands). Polymerase chain reaction (PCR) for circular transcript identification was carried out with divergent and convergent primers using DreamTaq DNA polymerase (Thermo Fisher Scientific). Quantitative real-time polymerase chain reaction (qRT-PCR) for the quantification of circBANP, linear BANP mRNA and FZD5 was performed using a Roto-Gene Probe RT-PCR Kit (Qiagen) on a Rotor-Gene Q instrument (Qiagen), with glyceraldehyde 3-phosphate dehydrogenase (GAPDH) as a loading control. Level of let-7a was determined using miScript Reverse Transcription Kit (Qiagen) and miScript SYBR Green PCR Kit (Qiagen), with U6 snRNA as the internal control. The relative expression of each gene and let-7a were calculated by the 2−ΔΔCt method. The primers used for PCR amplification were listed in ESI Table 1.†
2.3. Cell transfection
For circBANP knockdown, a total of 50 nM of siRNA against circBANP (si-circBANP-1, si-circBANP-2 or si-circBANP-3) was transfected into MKN-74 and MKN-45 cells, with nontarget siRNA (si-NC) as a negative control. For circBANP up-regulation, cells were introduced with 100 ng of circBANP overexpression plasmid, and empty vector was used as the negative control. Let-7a overexpression was implemented using 50 nM of modified let-7a mimic or a scrambled oligonucleotide sequence (miR-NC mimic). The TranslT-TKO® Transfection Reagent (Mirus Bio, Madison, WI, USA) was used for each transfection following the instructions of manufacturers, and the transfection efficiency was assessed by qRT-PCR assay. All oligonucleotides and plasmids were obtained from GenePharma (Shanghai, China). Oligonucleotide sequences (5′–3′) were as follows: si-circBANP-1: GTCAGCGTCGTCCCCCAGACT, si-circBANP-2: GACGGTCAGCGTCGTCCCCCA, si-circBANP-3: GGGCAGGACGGTCAGCGTCGT, si-NC: UUCUCCGAACGUGUCACGUTT, let-7a mimic: UGAGGUAGUAGGUUGUAUAGUU, miR-NC mimic: UUCUCCGAACGUGUCACGUTT.
2.4. Determination of cell proliferation
A Cell Counting Kit-8 (CCK-8, Dojindo, Kumamoto, Japan) was applied to evaluate the ability of cell proliferation, according to the producer's recommendations. Briefly, cells (5.0 × 104 per well) were placed in 96-well plates and introduced with the indicated oligonucleotides and/or plasmids. At 0, 24, 48 and 72 h post-transfection, CCK-8 solution (10 μL per well) was used, followed by the incubation for 3 h at 37 °C. Cell proliferation ability was proportional to the absorbance which was measured using a microplate reader (Spark 10M multimode, Tecan Trading AG, Zurich, Switzerland) at an optical density (OD) of 450 nm.
2.5. Colony formation assay
A standard colony formation assay was carried out to assess cell survival based on the ability of single cells to grow into colonies, as described previously with modification.17 In brief, transfected cells (500 per well) were seeded in the 6-well plates in growth media. 14 days later, colonies were stained with 0.1% crystal violet and colonies containing more than 50 cells were counted.
2.6. Flow cytometry for cell apoptosis
Cells were transfected as described above and harvested 48 h post-transfection. Then, cells were trypsinized in trypsin–EDTA and washed three times with PBS. Annexin V-FITC/propidium iodide (PI) staining experiments were performed using an Annexin V-FITC/PI Detection Kit (Thermo Fisher Scientific) referring to the protocols of manufacturers. Apoptotic cells were determined using a FACScanto II flow cytometer (BD Biosciences, Erembodegem, Belgium) with Cell Quest software.
2.7. Transwell migration and invasion assay
Cell migration assay was implemented using 24-Transwell chambers (8 μm pore size, Costar, Corning, Flintshire, UK) and Matrigel-coated chambers (Costar) were used for cell invasion assay, as described previously.11 To be brief, transfected cells (5.0 × 104) in serum-free media were added into the upper chamber, and the lower chamber was placed with 700 μL of growth media containing 10% FBS as a chemoattractant. Following the 24 h incubation, the images were obtained at ×200 magnification using an Olympus BX41 microscope (Olympus, Tokyo, Japan) and the number of cells that had migrated or invaded through the pores was counted by 10 random fields.
2.8. Bioinformatics
Analysis for the endogenous targeted miRNAs of circBANP was performed using starbase v3.0 online software at http://starbase.sysu.edu.cn/. The direct targets of let-7a were predicted by PITA software available at https://genie.weizmann.ac.il/pubs/mir07/mir07_prediction.html.
2.9. Dual-luciferase reporter assay
CircBANP luciferase reporter plasmid (circBANP-Wt) harboring the wild-type seed sequence for let-7a and site-directed mutant of seed sequence (circBANP-Mut), FZD5 3′-UTR wild-type luciferase reporter (FZD5-Wt) and its mutant in the let-7a-binding sequence (FZD5-Mut) were designed and synthesized by Hanbio (Shanghai, China). To confirm the targeted interaction between circBANP and let-7a, cells were introduced with 100 ng of circBANP-Wt or circBANP-Mut and 50 nM of miR-NC mimic or let-7a mimic. To validate whether FZD5 was a direct target of let-7a, 100 ng of FZD5-Wt or FZD5-Mut was cotransfected into cells together with 50 nM of miR-NC mimic or let-7a mimic. Luciferase activity was measured 48 h post-transfection using the dual-luciferase reporter assay system (Promega, Madison, WI, USA). Firefly luciferase activity was normalized against Renilla luciferase activity.
2.10. RNA pull-down assay
Biotinylated let-7a mimic (Bio-let-7a) and the mutation of seed sequence for circBANP (Bio-let-7a-Mut), biotinylated circBANP RNA (Bio-circBANP) and its mutant in the let-7a-binding sequence (Bio-circBANP-Mut), and corresponding negative control (Bio-miR-NC or Bio-NC) were synthesized by Boster Biological Technology (Wuhan, China). Cell lysates were prepared using RIPA lysis buffer (Beyotime, Shanghai, China) and incubated with the indicated biotinylated oligonucleotides for 1 h at room temperature, followed by the incubation with Streptavidin agarose beads (Sigma-Aldrich, Steinheim, Germany) for 1 h. Beads were harvested, washed three times with ice-cold PBS and total RNA was extracted for the enrichment of circBANP or let-7a by qRT-PCR.
2.11. Western blot
Total protein was extracted, separated, and transferred to polyvinylidene difluoride (PVDF, Millipore, Molsheim, France) membranes, as described previously.15 After being blocked with 5% non-fat milk, the membranes were probed with primary antibodies overnight at 4 °C, followed by the incubation with horseradish peroxidase (HRP)-conjugated IgG (ab6721, Abcam, Cambridge, UK; dilution 1
:
10
000) as the secondary antibody. Immunoreactive bands were detected using the Immobilon ECL Substrate Kit (Millipore) and analyzed by ImageJ software (National Institutes of Health, Bethesda, MD, USA). Primary antibodies were used as follows: anti-FZD5 (ab75234, Abcam; dilution 1
:
800), anti-β-catenin (ab32572, Abcam; dilution 1
:
5000), anti-phosphorylated (p)-β-catenin (ab11350, Abcam; dilution 1
:
1000), anti-c-Myc (ab32072, Abcam; dilution 1
:
1000), anti-Cyclin D1 (ab16663, Abcam; dilution 1
:
100) and anti-GAPDH (ab181602, Abcam; dilution 1
:
10
000).
2.12. Lentivirus transduction
Lentivirus vectors containing circBANP-encoding sequences (sh-circBANP) were obtained from Fulengen (Guangzhou, China), and nontarget lentivirus particles (sh-NC) were used as the negative control. MKN-45 cells were infected by sh-circBANP or sh-NC in medium containing 8 μg mL−1 of polybrene. 48 h later, the cells with positive transduction were selected using puromycin at a final concentration of 1 μg mL−1.
2.13. Xenograft model assay
Animal processes were performed according to the Institutional Guidelines approved by the Ethics Committee for Animal Experimentation of the First Affiliated Hospital of Zhengzhou University. Female 5 week-old BALB/c nude mice were purchased from Guangdong Research Center of Laboratory Animal (Foshan, China) and grown in a specific-pathogen-free (SPF) environment. About 1.0 × 107 MKN-45 cells stably transduced with sh-NC or sh-circBANP were subcutaneously implanted into the left flank of nude mice (n = 9 each group). 30 days after implantation, all mice were sacrificed and tumor tissues were weighed, followed by the further analyses of circBANP, let-7a, and FZD5 expression in tumor tissues.
2.14. Statistical analysis
SPSS 20.0 software (SPSS Inc., Chicago, IL, USA) was used for all statistical analyses in this study. All results were presented as mean ± SD from at least three independent experiments. Differences between groups were compared by a Student's t-test or one-way ANOVA, followed by Tukey's test. The Mann–Whitney U test was performed to verify the aberrant expression of circBANP in GC tissues. Correlation between circBANP expression and let-7a level in GC tissues was determined using Spearman test. The association between circBANP expression and clinicopathologic features of GC patients was analyzed by χ2 test. Statistical significant differences were inferred at a P < 0.05.
3 Results
3.1. CircBANP was up-regulated in GC tissues and cell lines
For the preliminary investigation for the involvement of circBANP in GC progression, we firstly detected the expression of circBANP in GC tissues and cells. As demonstrated by qRT-PCR, circBANP expression was significantly increased in GC tissues compared with adjacent normal gastric tissues (Fig. 1A and B). In line with GC tissues, the expression of circBANP was higher in GC cells than that of control (Fig. 1C). We also observed the correlation between circBANP level and clinicopathologic features of GC patients. These data showed that circBANP expression was strongly associated with tumor size, TMN stage, lymph node metastasis and distant metastasis (P < 0.05, Table 1).
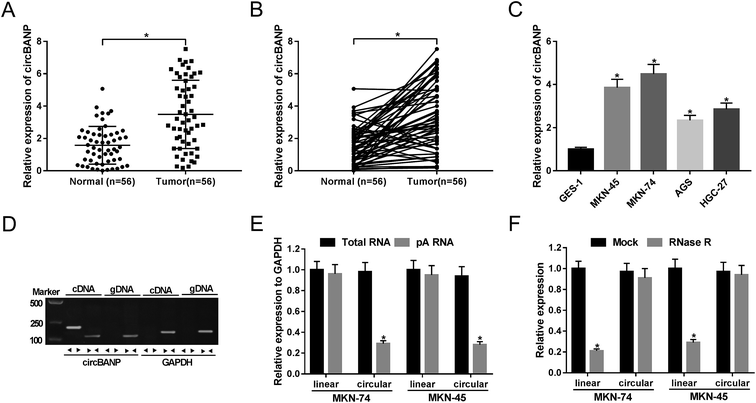 |
| Fig. 1 CircBANP was indeed circular transcripts and significantly up-regulated in GC. The expression of circBANP was assessed by qRT-PCR in 56 pairs GC tissues and adjacent normal gastric tissues (A and B), GC cell lines (MKN-45, MKN-74, AGS and HGC-27) and human normal gastric epithelial cell line GES-1 (C). (D) Divergent and convergent primers were used to amplify circBANP and linear BANP mRNA, respectively, with cDNA and gDNA from GC cells as templates. GAPDH was used as negative control. (E) Total RNA was extracted from MKN-74 and MKN-45 cells, and random and oligo-dT primers were used for reverse transcription, respectively, followed by the detection of linear transcripts and circular transcripts by qRT-PCR. (F) Total RNA was extracted from MKN-74 and MKN-45 cells, and then incubated with RNase R, followed by the measurement of linear transcripts and circular transcripts by qRT-PCR. *P < 0.05 vs. normal, total RNA or mock control. | |
Table 1 Correlation between circBANP expression and clinicopathologic features of GC patients. *P < 0.05
Characteristic |
Total |
CircBANP (%) |
P-Value |
Low n = 28 |
High n = 28 |
Gender |
Male |
37 |
19 (51.4%) |
18 (48.6%) |
0.778 |
Female |
19 |
9 (47.4%) |
10 (52.6%) |
![[thin space (1/6-em)]](https://www.rsc.org/images/entities/char_2009.gif) |
Age (years) |
≤60 |
21 |
10 (47.6) |
11 (52.4) |
0.783 |
>60 |
35 |
18 (51.4) |
17 (48.6) |
![[thin space (1/6-em)]](https://www.rsc.org/images/entities/char_2009.gif) |
Size (cm) |
≥5 cm |
14 |
3 (21.4) |
11 (78.6) |
0.014* |
<5 cm |
42 |
25 (59.5) |
17 (40.5) |
![[thin space (1/6-em)]](https://www.rsc.org/images/entities/char_2009.gif) |
Histological differentiation |
Well |
3 |
2 (66.7) |
1 (33.3) |
0.668 |
Moderate |
20 |
11 (55.0) |
9 (45.0) |
Poor |
33 |
15 (45.5) |
18 (54.5) |
![[thin space (1/6-em)]](https://www.rsc.org/images/entities/char_2009.gif) |
TNM stage |
I |
13 |
10 (76.9) |
3 (23.1) |
0.021* |
II |
12 |
8 (66.7) |
4 (33.3) |
III |
25 |
9 (36.0) |
16 (64.0) |
IV |
6 |
1 (16.7) |
5 (83.3) |
![[thin space (1/6-em)]](https://www.rsc.org/images/entities/char_2009.gif) |
Lymph node metastasis |
Yes |
33 |
12 (36.4) |
21 (63.6) |
0.015* |
No |
23 |
16 (69.6) |
7 (30.4) |
![[thin space (1/6-em)]](https://www.rsc.org/images/entities/char_2009.gif) |
Distant metastasis |
Yes |
8 |
1 (12.5) |
7 (87.5) |
0.022* |
No |
48 |
27 (56.3) |
21 (43.8) |
To determine that circBANP was indeed circular transcripts, we designed divergent and convergent primers to amplify circBANP and linear BANP mRNA, respectively. Using cDNA and genomic DNA (gDNA) from GC cells as templates, circBANP was amplified with the divergent primers on cDNA instead of gDNA (Fig. 1D). After that, total RNA was extracted from MKN-74 and MKN-45 cells, and random and oligo-dT primers were used for reverse transcription. Owing to the depleted effect of pA-enriched samples on circRNAs, circBANP level was prominently lower compared to linear templates in the two GC cells (Fig. 1E). Additionally, RNase R analysis revealed that circBANP could not digested by RNase R (Fig. 1F). These data together pointed that circBANP was indeed circular transcripts in GC cells.
3.2. Knockdown of circBANP weakened the proliferation, migration, invasion and enhanced the apoptosis in GC cells
In order to explore the functional role of circBANP in GC progression, loss-of-function experiments were carried out using siRNA against circBANP (si-circBANP). In contrast to a scrambled negative sequence, transient transfection of si-circBANP led to a significant reduction in circBANP expression in both MKN-74 and MKN-45 cells (Fig. 2A). Notably, si-circBANP-1 introduction triggered the most obvious decrease in the two cells (Fig. 2A), and thus si-circBANP-1 was selected for further experiments. CCK-8 assays showed that circBANP knockdown inhibited cell proliferation compared with negative control (Fig. 2B and C). Colony formation assays revealed that the depletion of circBANP markedly repressed the colony formation ability of the two GC cells (Fig. 2D). In comparison to negative group, circBANP knockdown led to a significant promotion in cell apoptosis using flow cytometry analysis (Fig. 2E). Moreover, transwell assays showed that circBANP depletion resulted in decreased capacity of cell migration and invasion (Fig. 2F and G). Additionally, qRT-PCR data showed that compared with vector negative control, circBANP expression was significantly elevated by transfection of circBANP overexpression plasmid (ESI Fig. 1†). Besides, circBANP overexpression led to a distinct enhancement in cell proliferation, colony formation, migration and invasion, as well as a clear suppression in cell apoptosis (ESI Fig. 2†).
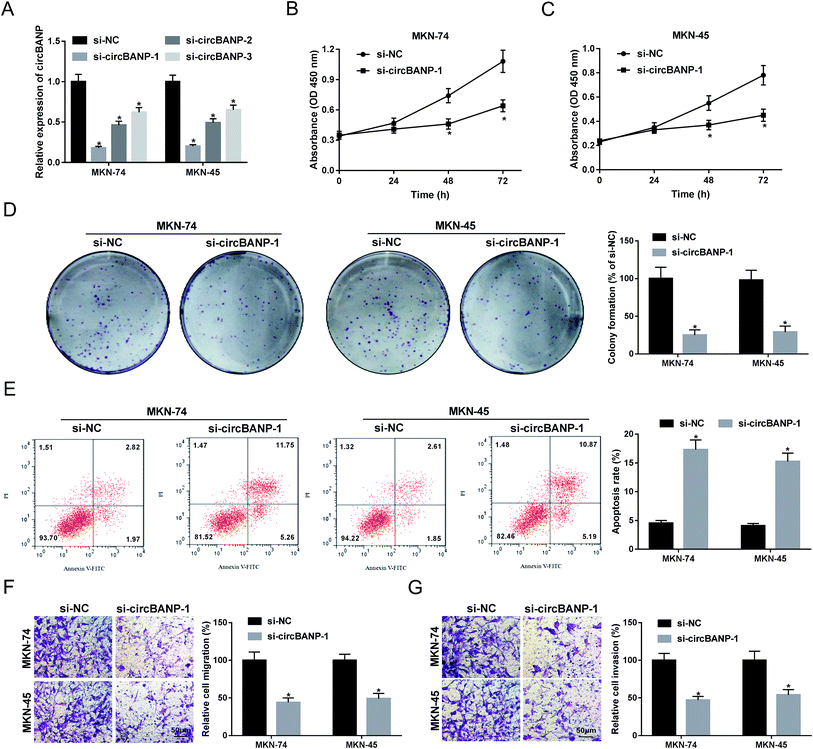 |
| Fig. 2 CircBANP knockdown suppressed the proliferation, migration, invasion, and enhanced the apoptosis in GC cells. (A) MKN-74 and MKN-45 cells were transfected with si-NC, si-circBANP-1, si-circBANP-2 or si-circBANP-3 for 48 h, and then circBANP expression was detected by qRT-PCR. MKN-74 and MKN-45 cells were transfected with si-NC or si-circBANP-1, followed by the determination of cell proliferation by CCK-8 assay after 0, 24, 48 and 72 h transfection (B and C), cell colony formation using a standard colony formation assay 14 days after transfection (D), cell apoptosis by flow cytometry after 48 h transfection (E), cell migration (F) and invasion (G) by transwell assay after 24 h transfection. *P < 0.05 vs. si-NC. | |
3.3. CircBANP acted as a molecular sponge of let-7a to sequester let-7a
Our above data demonstrated that circBANP regulated GC cell progression in vitro. Herein, we further explore how circBANP achieved it. Using software starbase v3.0, the predicted data showed a putative target sequence for let-7a in circBANP (Fig. 3A). To confirm this, circBANP luciferase reporter plasmid harboring the wild-type seed sequence for let-7a or a site-directed mutant of seed sequence was transfected into MKN-74 and MKN-45 cells together with miR-NC mimic or let-7a mimic. qRT-PCR data revealed a prominent increase of let-7a level in the presence of let-7a mimic in the two GC cells (Fig. 3B). With circBANP wild-type reporter and let-7a overexpression caused a significant reduction in luciferase activity (Fig. 3C and D). When the let-7a-binding sites were mutated, little change was observed in luciferase upon let-7a overexpression (Fig. 3C and D). Afterwards, to validate the endogenous correlation between circBANP and let-7a, RNA pull-down experiments were performed. In contrast to their counterparts, the enrichment of circBANP was strongly elevated by treatment of Bio-let-7a (Fig. 3E), and let-7a enrichment was manifestly increased when Bio-circBANP incubation (Fig. 3F). However, these effects were strikingly abrogated by the mutation of target sequence in the two GC cells (Fig. 3E and F). Then, we further observed whether circBANP could modulate let-7a expression. Given our data that si-circBANP-1 transfection resulted in decreased circBANP expression (Fig. 3G), qRT-PCR results also revealed that let-7a expression was significantly increased by down-regulated circBANP in both MKN-74 and MKN-45 cells (Fig. 3H). Additionally, our data demonstrated that let-7a level was down-regulated in GC tissues and cell lines compared with their counterparts (Fig. 3I and J). Spearman test showed an inverse correlation between circBANP level and let-7a expression in 56 GC tissues (Fig. 3K). These results together strongly established a notion that circBANP sequestered let-7a by acting as a molecular sponge of let-7a in GC cells.
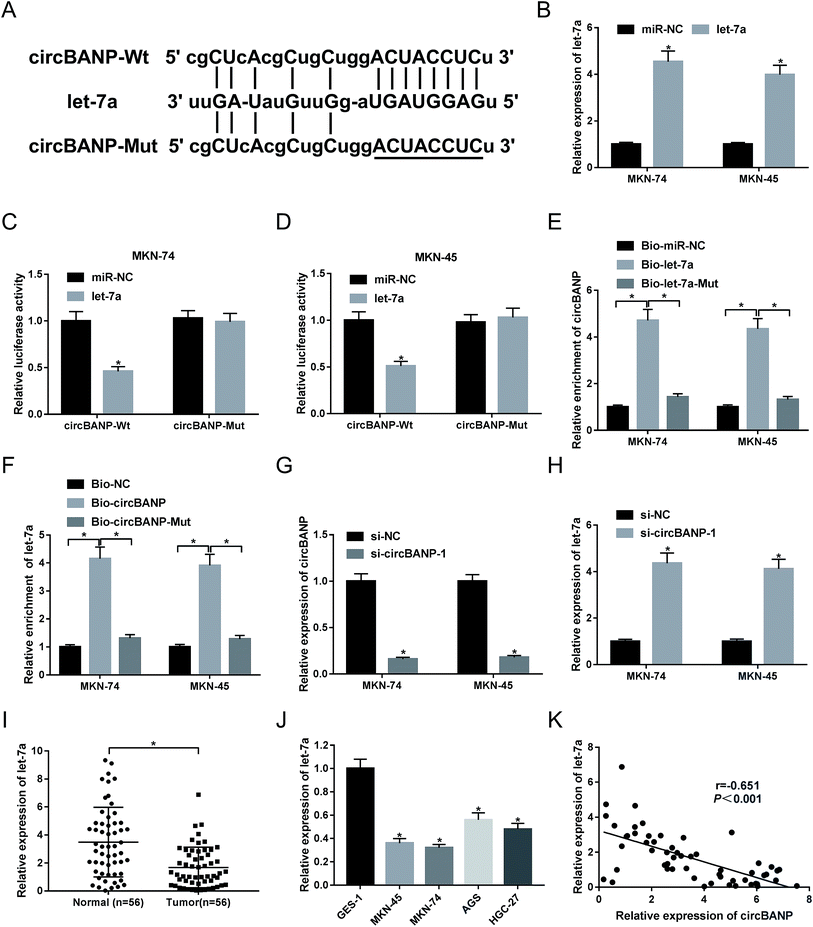 |
| Fig. 3 CircBANP sequestered let-7a in GC cells. (A) Schematic of the putative let-7a-binding sequence in circBANP and the mutated seed sequence. (B) Let-7a expression was assessed by qRT-PCR in MKN-74 and MKN-45 cells transfected with miR-NC mimic or let-7a mimic. (C and D) CircBANP luciferase reporter plasmid harboring the wild-type seed sequence for let-7a (circBANP-Wt) or a site-directed mutant of seed sequence (circBANP-Mut) was transfected into MKN-74 and MKN-45 cells together with miR-NC mimic or let-7a mimic, followed by the measurement of the luciferase activity. (E) The enrichment of circBANP was determined by qRT-PCR in cell lysates incubated with Bio-miR-NC, Bio-let-7a or Bio-let-7a-Mut. (F) The enrichment of let-7a was evaluated by qRT-PCR in cell lysates incubated with Bio-NC, Bio-circBANP or Bio-circBANP-Mut. (G and H) The levels of circBANP and let-7a were detected by qRT-PCR in MKN-74 and MKN-45 cells transfected with si-NC or si-circBANP-1. (I and J) Let-7a expression was assessed in 56 pairs GC tissues and adjacent normal gastric tissues, GC cell lines (MKN-45, MKN-74, AGS and HGC-27) and GES-1 cells. (K) Correlation between circBANP expression and let-7a level in GC tissues was determined using Spearman test. *P < 0.05 vs. miR-NC mimic, Bio-miR-NC mimic, Bio-NC, si-NC or normal control. | |
3.4. Let-7a mediated the regulatory effect of circBANP on GC cell proliferation, migration, invasion and apoptosis
Previous researches had reported that let-7a played a tumor-suppressive role in GC progression.14,18 To validate the functional role of let-7a in GC cell progression, gain-of-function experiments were performed by let-7a mimic. Transient introduction of let-7a mimic, but not miR-NC mimic control, significantly increased let-7a expression in both MKN-74 and MKN-45 cells (Fig. 4A). Subsequent experiments results revealed that let-7a overexpression resulted in a clear repression in cell proliferation (Fig. 4B and C), colony formation (Fig. 4D), and a distinct enhancement in cell apoptosis (Fig. 4E), as well as an obvious inhibition in cell migration (Fig. 4F) and invasion (Fig. 4G).
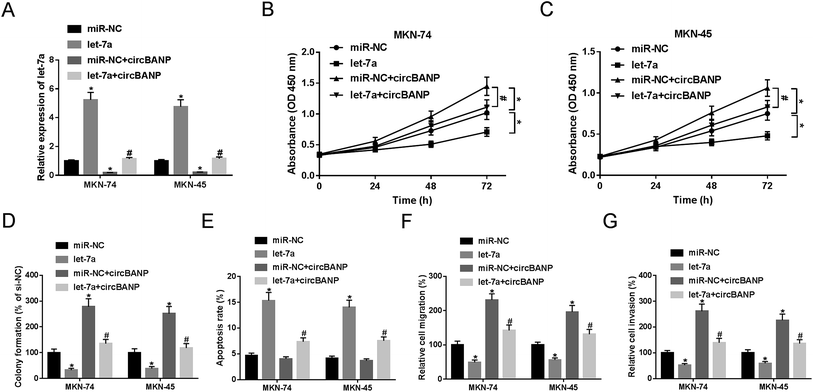 |
| Fig. 4 CircBANP-mediated regulatory effects on GC cell proliferation, migration, invasion and apoptosis were abated by cotransfection of let-7a mimic. MKN-74 and MKN-45 cells were transfected with miR-NC mimic, let-7a mimic, miR-NC mimic + circBANP or let-7a mimic + circBANP, followed by the detection of let-7a expression by qRT-PCR after 48 h transfection (A), cell proliferation by CCK-8 assay after 0, 24, 48 and 72 h transfection (B and C), cell colony formation by a standard colony formation assay 14 days after transfection (D), cell apoptosis by flow cytometry after 48 h transfection (E), cell migration (F) and invasion (G) by transwell assay after 24 h transfection. CircBANP: circBANP overexpression plasmid. *P < 0.05 vs. miR-NC mimic, #P < 0.05 vs. miR-NC + circBANP. | |
To verify whether circBANP exerted its regulatory effects on GC cell progression by let-7a, MKN-74 and MKN-45 cells were transfected with circBANP overexpression plasmid together with let-7a mimic or miR-NC mimic. In comparison to negative group, circBANP overexpression plasmid transfection triggered a significant decrease in let-7a expression in the two GC cells (Fig. 4A). However, circBANP-mediated reduced let-7a level was dramatically abated by cotransfection of let-7a mimic (Fig. 4A). Subsequent functional experiments demonstrated that the introduction of circBANP overexpression plasmid led to the significant enhancement in cell proliferation (Fig. 4B and C), colony formation (Fig. 4D), migration (Fig. 4F) and invasion (Fig. 4G). While, no change in cell apoptosis was observed in the presence of circBANP overexpression plasmid (Fig. 4E). Nevertheless, the regulatory effects of circBANP overexpression were evidently reversed by let-7a mimic cotransfection in the two GC cells (Fig. 4B–G).
3.5. CircBANP regulated FZD5 expression by sponging let-7a
Next, we investigated the molecular mechanisms by which let-7a influenced GC cell progression in vitro. In silico prediction by PITA software of let-7a target revealed that the FZD5 contained a potential complementary site for let-7a in its 3′-UTR (Fig. 5A). When we carried out a dual-luciferase reporter assay, cotransfection of FZD5 wild-type luciferase reporter and let-7a mimic into MKN-74 and MKN-45 cells produced lower luciferase activity than cells cotransfected with miR-NC mimic (Fig. 5B and C). However, site-directed mutant of the let-7a-binding sequence highly abolished the effect of let-7a on reporter gene expression (Fig. 5B and C). QRT-PCR and western blot results showed that compared with negative group, FZD5 expression was significantly reduced by up-regulated let-7a at mRNA (Fig. 5D) and protein (Fig. 5E and F) levels in MKN-74 cells. In parallel, the mRNA (Fig. 5G) and protein (Fig. 5H and I) levels of FZD5 in MKN-45 cells were remarkably decreased when let-7a overexpression. These results together indicated that FZD5 was directly targeted and repressed by let-7a in GC cells.
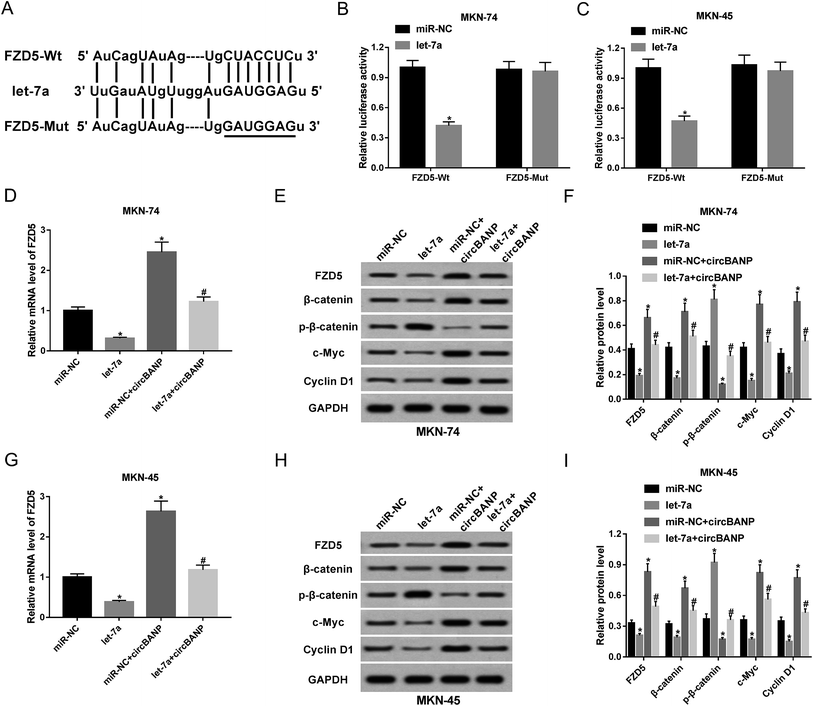 |
| Fig. 5 CircBANP regulated FZD5 expression by acting as a sponge of let-7a. (A) Schematic of the potential let-7a-binding sequence in FZD5 3′-UTR and site-directed mutant in target sequence. (B and C) FZD5 3′-UTR wild-type luciferase reporter plasmid (FZD5-Wt) or its mutant in seed region (FZD5-Mut) was transfected into MKN-74 and MKN-45 cells together with miR-NC mimic or let-7a mimic, followed by the determination of the luciferase activity. MKN-74 cells were transfected with miR-NC mimic, let-7a mimic, miR-NC mimic + circBANP or let-7a mimic + circBANP for 48 h, followed by the detection of FZD5 mRNA expression by qRT-PCR (D), FZD5, β-catenin, p-β-catenin, c-Myc and Cyclin D1 protein levels by western blot (E and F). MKN-45 cells were transfected with miR-NC mimic, let-7a mimic, miR-NC mimic + circBANP or let-7a mimic + circBANP, followed by the measurement of FZD5 mRNA level (G), FZD5, β-catenin, p-β-catenin, c-Myc and Cyclin D1 protein levels (H and I). CircBANP: circBANP overexpression plasmid. *P < 0.05 vs. miR-NC mimic, #P < 0.05 vs. miR-NC + circBANP. | |
Then, we further observed whether, if so, how circBANP modulated FZD5 expression in MKN-74 and MKN-45 cells. qRT-PCR and western blot analyses revealed that the mRNA and protein levels of FZD5 were significantly elevated by introduction of circBANP overexpression plasmid in the two GC cells (Fig. 5D–I). Moreover, circBANP-mediated increased FZD5 level was evidently abolished by cotransfection of let-7a mimic (Fig. 5D–I). These data together suggested that circBANP sequestered let-7a to protect against FZD5 repression.
Wnt/β-catenin signaling, an important pathway in development processes and homeostasis, underlies a wide range of human cancers, including GC.19,20 FZD5 has been identified as a good candidate to activate this pathway.21 Thus, we further investigated whether Wnt/β-catenin signaling was involved in the regulatory mechanism of circBANP/let-7a axis in GC cell progression. As expected, let-7a overexpression in MKN-74 cells led to the inactivation of Wnt/β-catenin pathway, as evidenced by the decrease of β-catenin, c-Myc and Cyclin D1 levels and the increase of p-β-catenin (Ser33/37) level (Fig. 5E and F). In parallel, up-regulated let-7a blocked the pathway in MKN-45 cells (Fig. 5H and I). Conversely, transfection of circBANP overexpression plasmid significantly activated Wnt/β-catenin signaling in both MKN-74 and MKN-45 cells (Fig. 5E, F, H and I). More interestingly, circBANP-mediated activation in this pathway was substantially abrogated by cotransfection of let-7a mimic in the two GC cells (Fig. 5E, F, H and I). All these data strongly hinted that Wnt/β-catenin signaling was involved in the regulatory network of circBANP/let-7a axis in GC cell progression.
3.6. Knockdown of circBANP retarded tumor growth in vivo
Given our data that circBANP regulated GC cell progression in vitro, we further investigated the effect of circBANP on tumor growth in vivo. Xenograft mice model was constructed by sh-circBANP-transduced MKN-45 cells. These results revealed that transduction of sh-circBANP repressed tumor growth, as presented by the reduction of tumor volume (Fig. 6A) and tumor weight (Fig. 6B). Moreover, qRT-PCR data showed that circBANP and FZD5 levels were significantly decreased and let-7a expression was remarkably increased in xenograft tissues derived from sh-circBANP-transduced MKN-45 cells (Fig. 6C). Additionally, circBANP depletion triggered the obvious repression in FZD5, β-catenin, c-Myc and Cyclin D1 protein levels, as well as a distinct enhancement in p-β-catenin (Ser33/37) expression (Fig. 6D).
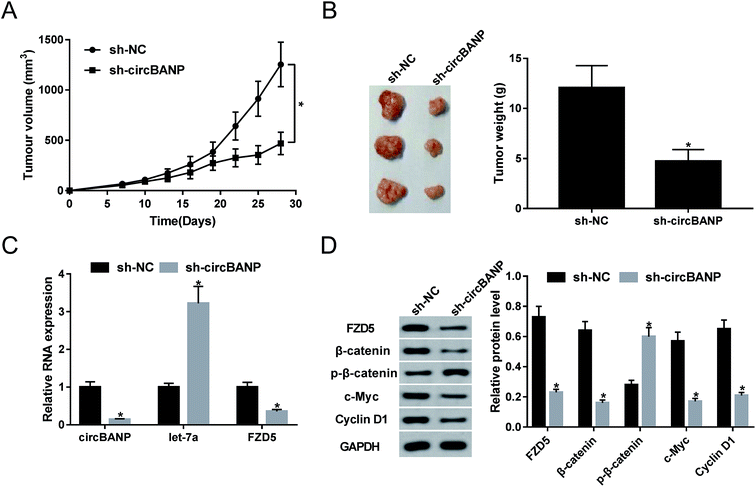 |
| Fig. 6 CircBANP depletion hampered tumor growth in vivo. Approximately 1.0 × 107 MKN-45 cells stably transduced with sh-NC or sh-circBANP were subcutaneously implanted into the left flank of nude mice (n = 9 each group). 30 days later, all mice were euthanized and tumor tissues were excised. (A) After 7 days implantation, tumor volume was measured by the callipers every 3 days. (B) Representative images and tumor average weight was calculated. (C) The expression levels of circBANP, let-7a and FZD5 were detected by qRT-PCR in xenograft tissues. (D) The protein levels of FZD5, β-catenin, p-β-catenin, c-Myc and Cyclin D1 were evaluated by western blot in excised tumor. GAPDH was used as internal control. *P < 0.05 vs. sh-NC. | |
4 Discussion
In recent years, circRNAs have been postulated to implicate in GC tumorigenesis and progression, providing a possibility of circRNAs as biomarkers for GC diagnosis, prognosis and treatment.9,22 For instance, Zhang et al. manifested that circRNA_100269 was down-regulated in GC tissues and highly expressed circRNA_100269 suppressed GC cell proliferation via sponging miR-630.8 Cheng et al. underscored that circRNA homeodomain-interacting protein kinases 3 (circHIPK3) was associated with the histological classification of GC and regulated GC cell proliferation through miR-124/miR-29b.23 Zhang and colleague reported that circRNA nuclear receptor interacting protein 1 (circNRIP1) silencing hampered GC cell proliferation, migration, and invasion through acting as a ceRNA of miR-149-5p.24 In this study, our data suggested that the knockdown of circBANP suppressed GC cell progression in vitro and in vivo by sponging let-7a and modulating FZD5/Wnt/β-catenin signaling pathway.
In the present study, our data firstly demonstrated that circBANP was up-regulated in GC tissues and cells, similar to previous works.10,11 Using divergent and oligo-dT primers and RNase R assay, we validated that circBANP was indeed circular transcripts in GC cells. Moreover, we were first to uncover that circBANP knockdown hindered the proliferation, migration, and invasion, and facilitated apoptosis in GC cells, and circBANP overexpression exhibited opposite effects. In short, circBANP performed a potential oncogenic effect in GC, consistent with the findings by Zhu and Zhang.10,11
It is widely accepted that circRNAs exert biological function through mechanisms including acting as miRNA sponges. Thus, starbase v3.0 software was applied to search for the targeted miRNAs of circBANP. Among these predicted candidates, let-7a was of particular interest in the present study, considering its tumor-suppressive role in many human cancers, such as pancreatic ductal adenocarcinoma and acute myeloid leukemia.25,26 Additionally, high level of let-7a drove cell cycle arrest, apoptosis, and mitigated cell migration and invasion in glioma cells through targeting K-ras independently of phosphatase and tensin homolog (PTEN).27 In this study, we firstly uncovered that circBANP acted as a molecular sponge of let-7a to sequester let-7a in GC cells using dual-luciferase reporter, RNA pull-down and qRT-PCR assays. Our data also validated that let-7a was down-regulated in GC tissues and cells, and let-7a overexpression inhibited GC cell proliferation, migration, invasion, and accelerated cell apoptosis, in accordance with previous researches.14,18,28 More interestingly, our results substantiated that let-7a mediated the regulatory effects of circBANP on GC cell proliferation, migration, invasion and apoptosis. In a word, circBANP enhanced GC progression in vitro possibly through sponging let-7a. Han et al. manifested that circBANP contributed to the progression of lung cancer via acting as a ceRNA of miR-503.11 Huang et al. reported that circ_0005519 functioned as a let-7a sponge to regulate asthma.29
Then, we carried out a detailed analysis for the direct targets of let-7a using PITA software. Intriguingly, the predicted data revealed that FZD5 was a potential target of let-7a. Subsequently, we confirmed that FZD5 was directly targeted and repressed by let-7a in GC cells. FZD family receptors have been implicated in carcinogenesis through transducing Wnt/β-catenin signaling.30,31 FZD5, a member of FZD family receptors, functions as WNT5A receptor and plays key roles in cancer biology.32,33 For example, Long et al. uncovered that FZD5 was directly targeted by tumor suppressor miR-124 and FZD5/protein kinase C (PKC) signaling was closely associated with P-glycoprotein-mediated chemoresistance and cancer cell survival in renal cell carcinoma.34 Zhu et al. manifested that FZD5/Wnt/β-catenin signaling was involved in the regulatory network of circ_0067934/miR-1324 axis on the growth and metastasis in hepatocellular carcinoma.35 Moreover, an earlier document demonstrated a significant up-regulation of FZD5 in GC cells.36 In the present study, our data also provided evidence for the role of circBANP as an effective sponge of let-7a to protect against FZD5 repression in GC cells. Besides, we firstly illuminated that Wnt/β-catenin signaling was involved in the regulatory mechanism of circBANP/let-7a axis in GC cell progression.
Lastly, xenograft model assay demonstrated that the knockdown of circBANP mitigated tumor growth in vivo. Moreover, circBANP depletion resulted in increased let-7a level and decreased FZD5 expression in xenograft tumor. In all, circBANP knockdown suppressed GC progression in vivo possibly via let-7a/FZD5/Wnt/β-catenin signaling. More researches in vivo about the novel mechanism will be implemented in further work.
5 Conclusion
In conclusion, our study suggested that the knockdown of circBANP repressed GC cell progression in vitro and in vivo at least partially through sponging let-7a and regulating FZD5/Wnt/β-catenin signaling. Targeting circBANP might highlight a potential alternative therapeutic strategy for improving GC treatment.
Funding
No funding was received.
Availability of data and materials
The analyzed data sets generated during the present study are available from the corresponding author on reasonable request.
Authors' contribution
Conceptualization and methodology: Chunfneg Wang and Jianning Yao; formal analysis and data curation: Bing Gao and Lianfeng Zhang; validation and investigation: Jin Xun and Bing Gao; writing – original draft preparation and writing – review and editing: Jin Xun, Chunfneg Wang and Jianning Yao; approval of final manuscript: all authors.
Ethics approval and consent to participate
The present study was approved by the ethical review committee of the First Affiliated Hospital of Zhengzhou University.
Patient consent for publication
Not applicable.
Conflicts of interest
The authors declare that they have no competing interests.
References
- F. Bray, J. Ferlay, I. Soerjomataram, R. L. Siegel, L. A. Torre and A. Jemal, Ca-Cancer J. Clin., 2018, 68, 394–424 CrossRef PubMed.
- Z. Song, Y. Wu, J. Yang, D. Yang and X. Fang, Tumor Biol., 2017, 39, 1010428317714626 Search PubMed.
- E. Smyth, M. Verheij, W. Allum, D. Cunningham, A. Cervantes and D. Arnold, Ann. Oncol., 2016, 27, v38–v49 CrossRef CAS PubMed.
- L.-L. Chen and L. Yang, RNA Biol., 2015, 12, 381–388 CrossRef PubMed.
- S. Meng, H. Zhou, Z. Feng, Z. Xu, Y. Tang, P. Li and M. Wu, Mol. Cancer, 2017, 16, 94 CrossRef PubMed.
- M. Su, Y. Xiao, J. Ma, Y. Tang, B. Tian, Y. Zhang, X. Li, Z. Wu, D. Yang, Y. Zhou, H. Wang, Q. Liao and W. Wang, Mol. Cancer, 2019, 18, 90 CrossRef PubMed.
- L. S. Kristensen, T. B. Hansen, M. T. Veno and J. Kjems, Oncogene, 2018, 37, 555–565 CrossRef CAS PubMed.
- Y. Zhang, H. Liu, W. Li, J. Yu, J. Li, Z. Shen, G. Ye, X. Qi and G. Li, Aging, 2017, 9, 1585–1594 CAS.
- W. Tang, K. Fu, H. Sun, D. Rong, H. Wang and H. Cao, Mol. Cancer, 2018, 17, 137 CrossRef PubMed.
- M. Zhu, Y. Xu, Y. Chen and F. Yan, Biomed. Pharmacother., 2017, 88, 138–144 CrossRef CAS PubMed.
- J. Han, G. Zhao, X. Ma, Q. Dong, H. Zhang, Y. Wang and J. Cui, Biochem. Biophys. Res. Commun., 2018, 503, 2429–2435 CrossRef CAS PubMed.
- D. P. Bartel, Cell, 2009, 136, 215–233 CrossRef CAS PubMed.
- H. O. Iwakawa and Y. Tomari, Trends Cell Biol., 2015, 25, 651–665 CrossRef CAS PubMed.
- Q. Yang, Z. Jie, H. Cao, A. R. Greenlee, C. Yang, F. Zou and Y. Jiang, Carcinogenesis, 2011, 32, 713–722 CrossRef CAS PubMed.
- H. Fan, M. Jiang, B. Li, Y. He, C. Huang, D. Luo, H. Xu, L. Yang and J. Zhou, Oncol. Rep., 2018, 39, 1207–1214 CAS.
- L. Salmena, L. Poliseno, Y. Tay, L. Kats and P. P. Pandolfi, Cell, 2011, 146, 353–358 CrossRef CAS PubMed.
- H. Braselmann, A. Michna, J. Hess and K. Unger, Radiat. Oncol., 2015, 10, 223 CrossRef PubMed.
- R. Tang, C. Yang, X. Ma, Y. Wang, D. Luo, C. Huang, Z. Xu, P. Liu and L. Yang, Oncotarget, 2016, 7, 5972–5984 Search PubMed.
- M. A. Chiurillo, World J. Exp. Med., 2015, 5, 84–102 CrossRef PubMed.
- K. W. McCracken, E. Aihara, B. Martin, C. M. Crawford, T. Broda, J. Treguier, X. Zhang, J. M. Shannon, M. H. Montrose and J. M. Wells, Nature, 2017, 541, 182–187 CrossRef CAS PubMed.
- Z. Lin, C. Gao, Y. Ning, X. He, W. Wu and Y.-G. Chen, J. Biol. Chem., 2008, 283, 33053–33058 CrossRef CAS PubMed.
- Y. Shao, J. Li, R. Lu, T. Li, Y. Yang, B. Xiao and J. Guo, Cancer Med., 2017, 6, 1173–1180 CrossRef CAS PubMed.
- J. Cheng, H. Zhuo, M. Xu, L. Wang, H. Xu, J. Peng, J. Hou, L. Lin and J. Cai, J. Transl. Med., 2018, 16, 216 CrossRef CAS PubMed.
- X. Zhang, S. Wang, H. Wang, J. Cao, X. Huang, Z. Chen, P. Xu, G. Sun, J. Xu, J. Lv and Z. Xu, Mol. Cancer, 2019, 18, 20 CrossRef PubMed.
- S. Ottaviani, J. Stebbing, A. E. Frampton, S. Zagorac, J. Krell, A. de Giorgio, S. M. Trabulo, V. T. M. Nguyen, L. Magnani, H. Feng, E. Giovannetti, N. Funel, T. M. Gress, L. R. Jiao and Y. Lombardo, et al., Nat. Commun., 2018, 9, 1845 CrossRef PubMed.
- Y. Chen, R. Jacamo, M. Konopleva, R. Garzon, C. Croce and M. Andreeff, J. Clin. Invest., 2013, 123, 2395–2407 CrossRef CAS PubMed.
- X. R. Wang, H. Luo, H. L. Li, L. Cao, X. F. Wang, W. Yan, Y. Y. Wang, J. X. Zhang, T. Jiang, C. S. Kang, N. Liu and Y. P. You, Neurooncology, 2013, 15, 1491–1501 CAS.
- Y. Zhu and F. Xu, Chin. Med. Sci. J., 2017, 32, 44–47 Search PubMed.
- Z. Huang, Y. Cao, M. Zhou, X. Qi, B. Fu, Y. Mou, G. Wu, J. Xie, J. Zhao and W. Xiong, Clin. Exp. Allergy, 2019, 49, 1116–1127 CrossRef CAS PubMed.
- M. Katoh and M. Katoh, Int. J. Mol. Med., 2007, 19, 273–278 CAS.
- R. K. Swain, M. Katoh, A. Medina and H. Steinbeisser, Cell Commun. Signaling, 2005, 3, 12 CrossRef PubMed.
- K. Benhaj, K. C. Akcali and M. Ozturk, Oncol. Rep., 2006, 15, 701–707 CAS.
- Z. Steinhart, Z. Pavlovic, M. Chandrashekhar, T. Hart, X. Wang, X. Zhang, M. Robitaille, K. R. Brown, S. Jaksani, R. Overmeer, S. F. Boj, J. Adams, J. Pan, H. Clevers and S. Sidhu, et al., Nat. Med., 2017, 23, 60–68 CrossRef CAS PubMed.
- Q. Z. Long, Y. F. Du, X. G. Liu, X. Li and D. L. He, Tumor Biol., 2015, 36, 7017–7026 CrossRef CAS PubMed.
- Q. Zhu, G. Lu, Z. Luo, F. Gui, J. Wu, D. Zhang and Y. Ni, Biochem. Biophys. Res. Commun., 2018, 497, 626–632 CrossRef CAS PubMed.
- H. Kirikoshi, H. Sekihara and M. Katoh, Int. J. Oncol., 2001, 19, 767–771 CAS.
Footnote |
† Electronic supplementary information (ESI) available. See DOI: 10.1039/c9ra09887a |
|
This journal is © The Royal Society of Chemistry 2020 |