DOI:
10.1039/C9RA09316H
(Paper)
RSC Adv., 2020,
10, 11173-11181
N-Carbamylglutamate and L-arginine supplementation improve hepatic antioxidant status in intrauterine growth-retarded suckling lambs
Received
9th November 2019
, Accepted 1st March 2020
First published on 18th March 2020
Abstract
The influence of dietary supplementation of L-arginine (Arg) or N-carbamylglutamate (NCG) on the hepatic antioxidant status in intrauterine-growth-retarded (IUGR) suckling lambs remains unclear. The current work aimed to investigate the regulatory mechanisms whereby dietary Arg or NCG alter hepatic antioxidant status in suckling lambs suffering from IUGR. Forty-eight newborn Hu lambs of normal birth weight (CON) and IUGR were allocated randomly into four groups of 12 animals each: CON (4.25 ± 0.14 kg), IUGR (3.01 ± 0.12 kg), IUGR + 1% Arg (2.99 ± 0.13 kg), or IUGR + 0.1% NCG (3.03 ± 0.11 kg). All lambs were raised for a period of 21 days from 7 to 28 days after birth. Compared with the IUGR suckling animals, glutathione peroxidase (GSH-Px), superoxide dismutase (SOD), and reduced glutathione (GSH) content were greater (P < 0.05), and protein carbonyl and malondialdehyde (MDA) levels were reduced (P < 0.05) in the livers of both IUGR + 1% Arg and 0.1% NCG suckling animals. Relative to IUGR suckling lambs, supplementing with Arg or NCG markedly reduced (P < 0.05) reactive oxygen species (ROS) levels, apoptosis, and necrosis in liver. Relative to IUGR suckling lambs, protein and mRNA expression of GSH-Px1, SOD2, catalase (CAT), heme oxygenase-1 (HO-1), inducible nitric oxide (NO) synthase (iNOS), and epithelial NO synthase (eNOS) increased in IUGR animals receiving Arg or NCG (P < 0.05). Both Arg and NCG can protect neonates from IUGR-induced hepatic oxidative damage through promoting the expression of antioxidative enzymes (including SOD, CAT, and GSH-Px), phase II metabolizing enzymes, and activation of the NO pathway.
1. Introduction
Intrauterine growth restriction (IUGR) can markedly reduce embryo and fetal development during pregnancy.1 Hu lambs are precocious and prolific, but an inadequate supply of nutrients during pregnancy leads to IUGR.2 Animals experiencing IUGR have greater rates of neonatal morbidity and lower metabolic efficiency, postnatal growth rate, and reproductive performance.2 Although infant lambs survive, IUGR leads to greater susceptibility to disorders in the nerve, respiration, intestine and circulatory systems.3
Oxidative injury due to oxygen toxicity is a leading parameter during the pathogenesis of newborn disorders.4 High concentrations of reactive oxygen species (ROS) as well as oxidative injury reported in a growing number of studies suggest that IUGR leads to oxidative stress (OS) in the neonates.5 Such phenomenon can be ascribed to the dysfunction of mitochondria and the impairment of the antioxidant defense system6 both of which are related to restricted growth and development during gestation.7 Neonates experiencing IUGR demonstrate oxidative injury within the intestine,8 lung9 and liver.10 Thus, previous prior research underscores that OS is a crucial component of the phenotypes that arise from IUGR.11 Along with OS, the lower antioxidant enzyme levels and reduced scavenging capacity12,13 underscore the potential for supplementation of antioxidants to IUGR newborns as a way to relieve oxidative injury during the neonatal period.
The supply of L-arginine (Arg), a substrate for synthesizing various molecules (such as nitric oxide NO, polyamines, and proteins), is restricted under OS status.14 Thus, Arg may help relieve the health- and developmental-related issues that IUGR causes.15,16 For instance, Arg can regulate synthesis of proteins and control the transduction of signals;17 enhance production performance in livestock breeding;18 suppress inflammatory cytokines such as IL-6 and TNF-α;19 and attenuate hepatic and plasma OS responses.20
N-Carbamylglutamate (NCG), the analogue of N-acetylglutamate synthase (NAG), is metabolically stable and can help increase both plasma Arg concentrations and endogenous synthesis via activating the carbamoylphosphate synthase-1 and pyrroline-5 carboxylate synthase.2,3,21 Whether supplementing Arg or NCG in the diet alters hepatic antioxidant status in IUGR suckling lambs remains unclear. In particular, the molecular mechanism and related signaling pathways influencing endogenous antioxidant ability of these compounds remains largely unknown.
This study aimed to test the hypothesis that Arg or NCG activate the endogenous antioxidant defense systems in liver of IUGR suckling lambs. We sought to address the following key issues: (1) whether antioxidant activity is related to Arg or NCG consumption; and (2) the way by which Arg or NCG induce the antioxidant response during IUGR-induced OS.
2. Materials and methods
All animal experiments, which had gained approval from the Animal Care and Use Committee of the Yangzhou University, were carried out in accordance with the practical animal protection law and the Guide for the Care and Use of Laboratory Animals formulated by the National Research Council (China).
2.1 Diets with milk replacer (MR)
Nutritional composition of MR diets is presented in Table 1. All ingredients were provided by Tianke and Hejia Co. (Nanjing, Jiangsu, China). All suckling lambs were raised for 21 days in one of the following iso-nitrogenous dietary groups: basal diet, basal diet + 1% Arg, or basal diet + 0.1% NCG. The chosen doses of Arg (1%) and NCG (0.1%) were based on prior research with ewes and lambs.3,8,18 Diets were prepared to meet dietary requirements for suckling lambs according to the NRC (2007),22 and had similar amounts of nitrogen (equilibrated by alanine) and energy. The NCG (at 97% purity) was purchased from Sigma-Aldrich Corporate (St. Louis). Arg and L-alanine were purchased from Ajinomoto (Beijing, China) Co.
Table 1 Ingredient and nutrient composition of milk replacer (dry matter basis, %)a
Component |
Milk replacer |
DM, dry matter; GE, gross energy; CP, crude protein; EE, ether extract; Ca, calcium; TP, total phosphorus. Main contents of the pre-mixed mixture (per kg of the pre-mixed mixture): Cu (as CuSO4·5H2O) 600 mg; Mn (MnSO4·H2O) 315 mg; Fe (FeSO4·7H2O) 8400 mg; Zn (ZnSO4·7H2O) 12 500 mg; Se (as Na2SeO3) 17 mg; vitamin A 55 000 IU; vitamin E 400 IU; vitamin D 5500 IU; vitamin K 12.5 mg; biotin 2 mg; folacin 7.5 mg; choline 15 mg; riboflavin 100 mg; vitamin B6 175 mg; thiamin 317.5 mg; and vitamin B12 500 mg. Nutrient levels are all measured values. |
Ingredients (%) |
Whey protein concentrate (34% CP) |
30.00 |
Milk fat powder (11% CP) |
35.00 |
Whole milk powder |
20.00 |
a-Casein |
5.00 |
Glucose |
6.00 |
Pre-mixed mixtureb |
4.00 |
![[thin space (1/6-em)]](https://www.rsc.org/images/entities/char_2009.gif) |
Nutrient content (analyzed)c |
GE (MJ kg−1) |
19.36 |
CP (%) |
23.77 |
EE (%) |
15.65 |
Ash (%) |
7.64 |
Lysine (%) |
1.53 |
Methionine (%) |
0.41 |
Threonine (%) |
0.87 |
L-Arginine (%) |
0.63 |
Ca (%) |
0.99 |
TP (%) |
0.73 |
2.2 Animals and treatments
As proposed by Che et al.,23 suckling lambs with birth weight (BW) close to mean litter BW (standard deviation, SD 0.5) were deemed to be normal (NBW), while animals whose BW was ≥1.5 SD lower were deemed to be IUGR. On day 7 after birth, 48 newborn Hu lambs weighing 4.25 ± 0.14 kg (NBW) or 3.01 ± 0.13 kg (IUGR) were selected from a cohort of 432 twin lambs at the Jiangyan Experimental Station. (Taizhou, Jiangsu, China). Animals were weaned at 7 days of age, and based on initial BW were then randomly assigned to one of 4 groups: CON, IUGR, IUGR + 1% Arg, or IUGR + 0.1% NCG with 12 (6 males and 6 females) in each group. Each treatment was replicated three times, and 4 lambs were in each replication. All animals were on experiment from 7 to 28 days of age, and were housed within a 4 × 1 m2 indoor pen during each replication.
For each lamb on each day, the feeding level of the MR on a dry matter basis was adjusted to 2% of the live weight of the lamb every 10 days and fed 3 times daily via nipple bottle (0700, 1300, and 1900). Prior to feeding, MR was dissolved in hot water to prepare the 40 °C solution (containing 16.67% DM), which was then given to all animals. All animals had free access to clean water during the experiment. Oral administration of MR was done by skilled workers to exclude stressful events interfering with the data. MR intake for each lamb was recorded daily and calculated as the difference between the amount offered and refused. Average daily dry matter intake (ADMI) was calculated via multiplying the average daily MR intake by its DM content (%).
2.3 Collection of samples
Body weights were measured at 7 and 28 days of age. Sodium pentobarbital (15 mg kg−1) was given intravenously at the 28th day prior to sacrifice. Blood samples were collected into tubes containing EDTA as anticoagulant. Plasma was harvested through centrifugation at 3000 × g for 15 min at 4 °C, and then preserved at −80 °C to analyze NO content and NOS activity. A portion of liver tissue harvested after slaughter was extracted and weighed prior to preserving at −80 °C.
2.4 Determination of OS status
Protein carbonyls, oxidized glutathione (GSSG), malondialdehyde (MDA), and reduced glutathione (GSH) concentrations together with glutathione peroxidase (GSH-Px), glutathione reductase (GR), and superoxide dismutase (SOD) activities were determined using commercial kits (Jiancheng Bioengineering Institute, Nanjing, Jiangsu, China). Results were standardized based on total protein content. Protein content was quantified by the bicinchoninic acid protein assay kit (Nanjing Jiancheng Bioengineering Institute, Nanjing, Jiangsu, China).14
2.5 Content of NO and activity of NOS
Concentration of NO and nitric oxide synthase (NOS) activity were analyzed in plasma and liver tissue using assay kits following manufacturer's instructions.24
2.6 Hepatocyte isolation
A fraction of fresh liver samples was rapidly processed to isolate hepatocytes to determine concentrations of ROS and rate of apoptosis and necrosis. During hepatocyte isolation, the haematopoietic cell population was eliminated. More than 90% of the cells are typical hepatocytes, and the remaining 10% are fibroblast-like cells.25 Upon harvesting, livers were placed in ice-cold Hanks' balanced salt solution (HBSS). Approximately 3 g of liver was minced and then shaken for 5 min at 37 °C in 25 mL of HBSS containing 5 mM-EDTA. Minced samples were shaken for another 10 min in 25 mL of HBSS containing 0.25% (w/v) collagenase I (Sigma-Aldrich), 0.01% (w/v) DNase (Sigma-Aldrich) and 5 mM-CaCl2 at 37 °C. The supernatant was removed, and the digestion process was repeated. The cell suspension was filtered through a 70 μm nylon mesh and centrifuged at 20g for 1 min. The pellet was resuspended in PBS and centrifuged at 20 × g for 1 min. Cell viability was determined by trypan blue exclusion.26
2.7 Determination of ROS concentrations
The 2′,7′-dichlorodihydrofluorescein diacetate method (DCFH-DA; Sigma-Aldrich) was used as described previously.27 Intracellular DCFH-DA was cleaved through non-specific esterases, which led to DCFH formation; while DCFH, in the non-fluorescent form can be oxidized by ROS into the fluorescent compound 2′,7′-dichlorofluorescein. Approximately 1 × 106 hepatocytes were rinsed with PBS followed by 20 min of incubation with DCFH-DA (10 μm) at 37 °C in the dark. Following rinsing with ice-cold PBS for twice, the liver cells were collected, followed by immediate detection by the FACScan flow cytometer (Beckman Coulter).
2.8 Measurement of apoptosis and necrosis
Degree of apoptosis as well as necrosis was measured through differential staining using Annexin V (which stained the apoptotic as well as necrotic cells) combined with propidium iodide (PI, which stained the necrotic cells alone), and the Alexa Fluor® 488-Annexin V/Dead Cell Apoptosis Kit (V13241; Invitrogen Life Technologies) was used according to previous description.28 Such method was performed on the basis of the Annexin V property to bind phosphatidylserine and the propidium iodide ability to intercalate DNA. In brief, hepatocyte density was measured, and approximately 1 × 106 cells were resuspended in 1× binding buffer. Afterwards, the solution supplemented with PI as well as Alexa Fluor® 488-Annexin V was added into the cell suspension, followed by gentle vortexing and 15 min of incubation in dark under ambient temperature. Subsequently, 1× binding buffer (at 4-fold volume) was added into every tube, followed by analysis by the FACScan flow cytometer (Beckman Coulter).
2.9 mRNA abundance by real-time PCR
Total RNA was extracted from frozen liver samples using TRIzol reagent (Invitrogen Life Technologies, Gaithersburg, MD, U.S.A.), and the purity and quality of the extracted RNA were assessed by electrophoresis (A260/A280, Beckman DU-800, Beckman Coulter, Inc., Fullerton, CA, U.S.A.). Then, 100 ng of RNA was used to synthesize cDNA within the 20 μL reaction system. The RT-qPCR was performed using the ABI-7900HT instrument (Applied Biosystems, Foster City, CA, USA). The reaction was carried out in 10 μL reaction mixture containing 0.2 μL ROX Reference Dye II, 5 μL SYBR Premix Ex Taq II (Tli RNaseH Plus; TaKaRa Biotechnology Co., Ltd., Dalian, China), 1 μL cDNA, 0.4 μL of each primer (forward and reverse), and 3 μL diethylpyrocarbonate-treated water. The RT-qPCR was performed as follows: 30 s at 95 °C, 40 cycles of 5 s at 95 °C and 31 s at 60 °C, 15 s at 95 °C, 1 min at 60 °C, and 15 s at 95 °C. All samples were measured in triplicate, and the real-time PCR assay had similar efficiency (90–110%). The gene β-actin (ACTB) was used as the internal control to normalize target gene expression data. Relative gene expression was analyzed according to the 2−ΔΔCt method. The primer sequences are listed in Table 2 (Sangon Biotech, Shanghai, China).29 The mRNA level of each target gene was expressed relative to the CON group. Although ACTB has been found to have a high degree of stability in similar samples and has been previously used as an internal control gene,8 we acknowledge that using more than one internal control gene is the most appropriate approach to normalize gene expression data.30,31 Therefore, we consider that using ACTB as the only internal control gene is a limitation to this study.
Table 2 The primer sequences used in the real-time PCR
Genesa |
Sequencesb (5′–3′) |
Gene bank no. |
CAT, catalase; GSH-Px1, glutathione peroxidase 1; SOD2, superoxide dismutase 2; Nrf2, nuclear factor erythroid 2-related factor 2; HO-1, heme oxygenase-1; NQO1, quinone oxidoreductase 1; eNOS, epithelial NO synthase; iNOS, inducible NO synthase. Bax, Bcl-2-associated X protein; Bcl-2, B-cell lymphoma/leukaemia 2. F, forward; R, reverse. |
CAT |
F: CACTCAGGTGCGGGATTTCT |
GQ204786.1 |
R: ATGCGGGAGCCATATTCAGG |
GSH-Px1 |
F: GCAACCAGTTTGGGCATCAG |
JF728302.1 |
R: GCCATTCACCTCGCACTTTT |
SOD2 |
F: GTGAACAACCTCAACGTCGC |
XM_013966636.1 |
R: GCGTCCCTGCTCCTTATTGA |
Nrf2 |
F: ATCCAGATGCTCACCATGCG |
XM_005674733.2 |
R: CCCAATGCAGGACTTGGTCT |
HO-1 |
F: GAACGCAACAAGGAGAAC |
001014912.1![[thin space (1/6-em)]](https://www.rsc.org/images/entities/char_2009.gif) |
R: CTGGAGTCGCTGAACATAG |
NQO1 |
F: CAACAGACCAGCCAATCA |
001034535.1 |
R: ACCTCCCATCCTTTCCTC |
iNOS |
F: TGAGAATGGCAGCTACTGGGTCAA |
AF223942.1 |
R: GGTGATGTCCAGGAAATGGGTGA |
eNOS |
F: AGCATCACCTACGACACTCTG |
NM_001129901.1 |
R: ACTGGTTGATGAAGTCCCTGG |
Bcl-2 |
F: CGAGTGGCGGCTGAAAT |
HM630309.1 |
R: GGTCTGCCATGTGGGTGTC |
Bax |
F: ATGGGCTGGACATTGGACTT |
AF163774.1 |
R: ACTGTCTGCCATGTGGGTGT |
β-Actin |
F: GCTCTTCCAGCCGTCCTT |
NM_001009784.1 |
R: TGAAGGTGGTCTCGTGAATGC |
2.10 Western blotting
Total protein from hepatic tissue was extracted with a commercial kit (Beyotime Biotechnology, Jiangsu, China) and homogenized according to the manufacturer's protocol. Protein content was measured using the bicinchoninic acid protein assay kit (Pierce, Rockford, IL, U.S.A.). The antibodies used in this study were as follows: anti-GSH-Px1 (1
:
2000, Abcam), anti-CAT (1
:
2000, Protein Tech), anti-Nrf2 (1
:
2000, Protein Tech), anti-SOD2 (1
:
5000, Novus), anti-NQO1 (1
:
1000, Abcam), anti-HO-1 (1
:
1000, Abcam), anti-eNOS (1
:
1000, CELL SIGNALING), anti-iNOS (1
:
1000, Cell Signaling), and anti-β-actin (1
:
1500, Santa Cruz). Following 1 h of incubation by the HRP-conjugated goat anti-rabbit IgG secondary antibody, signals were detected using the enhanced chemiluminescence kits (ECL-Plus, Thermo, Waltham, MA, USA); afterwards, they were screened to detect the fluorescence using the BioRad gel detection system. The densitometric values were standardized based on β-actin, and expressed relative to control.32
2.11 Statistical analysis
Results are expressed as means with pooled SEMs. The fixed effect of sex was included in the original statistical model and was not significant (P > 0.05); thus, it was eliminated from the final model, in which only treatment was the fixed effect. Lamb within treatment was the random effect. Statistical analysis was performed using a one-way ANOVA according to the PROC GLM procedure of SAS (version 9.2). Duncan's multiple range test was used to determine differences among treatments. A probability value of ≤0.05 was used to represent significance.
3. Results
3.1 Growth performance
Compared with CON, IUGR animals had lower average daily gain (ADG) and ADMI (P < 0.05), while higher feed conversion ratio (FCR) (P < 0.05) (Table 3). Compared with IUGR animals, IUGR animals under Arg or NCG had higher ADG (P < 0.05) and reduced FCR (P < 0.05). In comparison to the IUGR lambs, the weight of the liver was greater (P < 0.05) in the Arg- or NCG-treated IUGR lambs. The liver weight/BW was not significantly different (P > 0.05) in the Arg- or NCG-treated IUGR compared to the IUGR lambs.
Table 3 Effects of dietary Arg and NCG supplementation on growth performance and liver organ indices in IUGR suckling lambs for days 7–28a
Itemb |
CON |
IUGR |
IUGR + 1% Arg |
IUGR + 0.1% NCG |
SEM |
P value |
Mean values with their SEM (n = 12/group). Within a row, means without a common letter differ (p < 0.05). ADG, averaged daily gain; ADMI, averaged dry matter intake; FCR, feed conversion ratio; CON, normal birth weight group given a control diet; IUGR, intrauterine growth retardation group given a control diet; IUGR + Arg, intrauterine growth retardation group given an arginine-supplemented diet; IUGR + NCG, intrauterine growth retardation group given a N-carbamylglutamate-supplemented diet. |
Initial weight (kg) |
4.25a |
3.01b |
2.99b |
3.03b |
0.13 |
0.014 |
Final weight (kg) |
7.66a |
5.41c |
6.01b |
6.04b |
0.28 |
0.008 |
Net weight gain (kg) |
3.41a |
2.40c |
3.02b |
3.01b |
0.09 |
0.011 |
ADG (g per day) for days 7–28 |
162.38a |
114.29c |
143.81b |
143.33b |
8.99 |
0.007 |
ADMI (g per day) for days 7–28 |
263.06a |
238.87b |
250.23ab |
246.53ab |
12.04 |
0.021 |
FCR for days 7–28 |
1.62c |
2.09a |
1.74b |
1.72b |
0.05 |
0.006 |
Liver weight (g) |
172.65a |
115.65c |
135.78b |
137.26b |
12.35 |
0.008 |
Liver weight/BW (%) |
2.25 |
2.15 |
2.26 |
2.27 |
0.07 |
0.109 |
3.2 Hepatic OS status
Compared with CON, protein carbonyl as well as MDA level in livers of IUGR animals was higher (P < 0.05), whereas GSH-Px, SOD, GSH/GSSG ratio as well as GSH level were lower (P < 0.05) (Table 4). Compared with IUGR lambs, GSH-Px, SOD, GSH/GSSG ratio as well as GSH level in the livers of IUGR lambs under 1% Arg or 0.1% NCG treatment were higher (P < 0.05), whereas protein carbonyl as well as MDA level were lower (P < 0.05).
Table 4 Effects of dietary Arg and NCG supplementation on OS status in the liver of IUGR suckling lambsa
Itemb |
CON |
IUGR |
IUGR + 1% Arg |
IUGR + 0.1% NCG |
SEM |
P value |
Mean values with their SEM (n = 12/group). Within a row, means without a common letter differ (p < 0.05). OS, oxidative stress; MDA, malondialdehyde; SOD, superoxide dismutase; GSH-Px, glutathione peroxidase; GR, glutathione reductase; GSH, reduced glutathione; GSSG, oxidized glutathione. CON, normal birth weight group given a control diet; IUGR, intrauterine-growth-retarded group given a control diet; IUGR + Arg, intrauterine growth-retarded group given a L-arginine-supplemented diet; and IUGR + NCG, intrauterine-growth-retarded group given a N-carbamylglutamate supplemented diet. |
MDA (nmol per mg protein) |
0.65b |
0.98a |
0.61b |
0.67b |
0.07 |
0.008 |
Protein carbonyl (nmol per mg protein) |
1.63b |
2.38a |
1.72b |
1.69b |
0.15 |
0.021 |
SOD (U per mg protein) |
101.23a |
62.46c |
80.24b |
86.13b |
4.26 |
0.009 |
GSH-px (U per mg protein) |
23.78a |
14.01c |
19.12b |
18.93b |
1.56 |
0.019 |
GR (U per g protein) |
12.89 |
12.06 |
13.21 |
13.05 |
0.88 |
0.209 |
GSH (nmol per mg protein) |
6.72a |
3.68b |
6.08a |
6.13a |
0.34 |
0.006 |
GSSG (nmol per mg protein) |
0.67 |
0.71 |
0.69 |
0.72 |
0.07 |
0.103 |
GSH/GSSG |
10.05a |
5.18c |
8.81b |
8.51b |
1.13 |
0.024 |
3.3 NO content and NOS activity
Relative to CON, IUGR reduced markedly NO content and activity of NOS in the serum and liver of suckling lambs (P < 0.05) (Fig. 1). Relative to the IUGR group, IUGR animals under Arg or NCG treatment had (P < 0.05) a higher content of NO and activity of NOS in the serum and liver (P < 0.05).
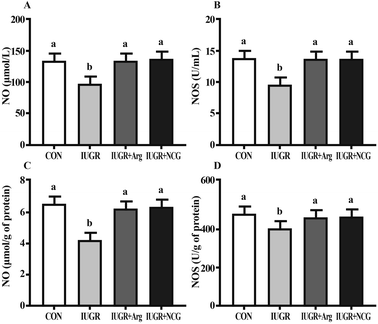 |
| Fig. 1 Effects of dietary Arg and NCG supplementation on the NO content and activity of NOS in the serum (A and B) and liver (C and D) of IUGR suckling lambs. NO, nitric oxide; NOS, nitric oxide synthase; CON, normal birth weight group given a control diet; IUGR, intrauterine growth retardation group given a control diet; IUGR + Arg, intrauterine growth retardation group given an arginine-supplemented diet; IUGR + NCG, intrauterine growth retardation group given a N-carbamylglutamate-supplemented diet. n = 12/group. Mean values in columns with unlike superscript letters were significantly different (P < 0.05). | |
3.4 Hepatic ROS and apoptosis
Compared with CON, the IUGR lambs had higher (P < 0.05) ROS levels, apoptotic as well as necrotic cell proportion in hepatocytes (Table 5). Relative to IUGR animals, supplementation with Arg or NCG reduced (P < 0.05) ROS, apoptosis, and necrosis level in the liver.
Table 5 Effects of dietary Arg and NCG supplementation on ROS and apoptosis levels in the liver of IUGR suckling lambsa
Itemb |
CON |
IUGR |
IUGR + 1% Arg |
IUGR + 0.1% NCG |
SEM |
P value |
Mean values with their SEM (n = 12/group). Within a row, means without a common letter differ (p < 0.05). ROS, reactive oxygen species; ROS was expressed in arbitrary units. The ROS levels of each lamb in the CON group were assigned a value of 1. CON, normal birth weight group given a control diet; IUGR, intrauterine-growth-retarded group given a control diet; IUGR + Arg, intrauterine growth-retarded group given a L-arginine-supplemented diet; and IUGR + NCG, intrauterine-growth-retarded group given a N-carbamylglutamate supplemented diet. |
ROS |
1.00c |
2.78a |
1.58b |
1.61b |
0.13 |
0.006 |
Apoptotic cells (%) |
5.02c |
11.35a |
7.99b |
8.13b |
0.38 |
0.014 |
Necrotic cells (%) |
0.19c |
0.63a |
0.42b |
0.38b |
0.04 |
0.005 |
3.5 mRNA abundance in liver
Compared with CON, mRNA expression of GSH-Px1, CAT, SOD2, Nrf2, HO-1, NQO1, Bcl-2, iNOS and eNOS was down-regulated (P < 0.05) in livers of IUGR animals. In contrast, those genes were up-regulated (P < 0.05) in livers of IUGR animals under Arg or NCG treatment compared with IUGR animals (Table 6). Relative to the IUGR group, Bax mRNA level was reduced (P < 0.05) in IUGR animals fed Arg or NCG.
Table 6 Effects of dietary Arg and NCG supplementation on the mRNA abundance of genes in the liver of IUGR suckling lambsa
Itemb |
CON |
IUGR |
IUGR + 1% Arg |
IUGR + 0.1% NCG |
SEM |
P value |
Mean values with their SEM (n = 12/group). Within a row, means without a common letter differ (p < 0.05). CAT, catalase; GSH-Px1, glutathione peroxidase 1; SOD2, superoxide dismutase 2; Nrf2, nuclear factor erythroid 2-related factor 2; HO-1, heme oxygenase-1; NQO1, quinone oxidoreductase 1; NO, nitric oxide; eNOS, epithelial NO synthase; iNOS, inducible NO synthase. Bax, Bcl-2-associated X protein; Bcl-2, B-cell lymphoma/leukaemia 2; CON, normal birth weight group given a control diet; IUGR, intrauterine-growth-retarded group given a control diet; IUGR + Arg, intrauterine growth-retarded group given a L-arginine-supplemented diet; and IUGR + NCG, intrauterine-growth-retarded group given a N-carbamylglutamate supplemented diet. |
CAT |
1.00a |
0.41c |
0.71b |
0.69b |
0.04 |
0.005 |
GSH-Px1 |
1.00a |
0.52c |
0.78b |
0.77b |
0.05 |
0.009 |
SOD2 |
1.00a |
0.51c |
0.74b |
0.75b |
0.08 |
0.012 |
Nrf2 |
1.00a |
0.49b |
0.93a |
0.97a |
0.08 |
0.025 |
HO-1 |
1.00a |
0.46c |
0.73b |
0.79b |
0.15 |
0.006 |
NQO1 |
1.00a |
0.41c |
0.69b |
0.71b |
0.11 |
0.011 |
iNOS |
1.00a |
0.51b |
0.89a |
0.90a |
0.15 |
0.007 |
eNOS |
1.00a |
0.53b |
0.96a |
0.98a |
0.15 |
0.006 |
Bcl-2 |
1.00a |
0.48c |
0.73b |
0.71b |
0.09 |
0.008 |
Bax |
1.00c |
2.56a |
1.79b |
1.71b |
0.13 |
0.006 |
3.6 Protein abundance in liver
Relative to CON, IUGR decreased GSH-Px1, CAT, Nrf2, SOD2, NQO1, HO-1, iNOS as well as eNOS protein levels in the liver of suckling lambs (P < 0.05) (Fig. 2). Relative to IUGR, IUGR animals under Arg or NCG treatment had higher (P < 0.05) protein levels of GSH-Px1, Nrf2, CAT, HO-1, SOD2, NQO1, iNOS and eNOS.
 |
| Fig. 2 Effects of dietary Arg and NCG supplementation on the antioxidant defense related protein expression (A–C), Nrf2-signaling-pathway-related protein expression (D–F), and L-arginine/NO pathway related protein expression (G and H) in the liver of IUGR suckling lambs. CAT, catalase; GSH-Px1, glutathione peroxidase 1; SOD2, superoxide dismutase 2; Nrf2, nuclear factor erythroid 2-related factor 2; HO-1, heme oxygenase-1; NQO1, quinone oxidoreductase 1; NO, nitric oxide; eNOS, epithelial NO synthase; iNOS, inducible NO synthase; CON, normal birth weight group given a control diet; IUGR, intrauterine growth retardation group given a control diet; IUGR + Arg, intrauterine growth retardation group given an arginine-supplemented diet; IUGR + NCG, intrauterine growth retardation group given a N-carbamylglutamate-supplemented diet. n = 12/group. Mean values in columns with unlike superscript letters were significantly different (P < 0.05). | |
4. Discussion
In addition to the previously described effect on relieving intestinal injury,8,18 the capacity of Arg or NCG for mitigating hepatic injury can also help enhance IUGR lamb growth as well as overall health status. According to our results, Arg or NCG restored the GSH pool, promoted activity of SOD as well as gene and protein expression involved in the antioxidant system. These changes likely contributed to the potential protective mechanisms of Arg or NCG in preventing hepatic disease at an early age.
Oxidative stress (OS) is caused by the oxidant-antioxidant imbalance, which is one key characteristic of the IUGR phenotype.33 Overproduction of ROS can result in lipid peroxidation, which is a vital cause of cell damage.34 The MDA molecule is a major lipid peroxidation product, while protein carbonyl content is recognized as a liver-protein oxidation marker.35 Suckling animals that underwent IUGR in the present study had higher protein carbonyl and MDA levels in the liver, which underscores the high degree of protein oxidation and lipid peroxidation in the tissue. Similar results were obtained in piglets13 and rats suffering from IUGR.36 The GSH is one of the most important intracellular antioxidants that could eliminate lipid peroxides and repair oxidative proteins through a reaction catalysed by GSH-Px.37 During these reactions, GSH is transformed into GSSG, the disulphide form, which is then reduced into GSH by GR under normal conditions. GR, a critical antioxidant enzyme, is able to protect cells from OS.38 The fact that IUGR decreased markedly GSH content, GSH-Px activity, as well as GSH/GSSG ratio in the liver suggested a greater susceptibility of lipid, protein as well as DNA to OS. In cases of severe OS, GSH content is reduced, while GSSG levels increase resulting in higher GSSG/GSH ratio.39 The present results were in agreement with those described by Zhang et al.,26 which revealed a lower metabolic efficiency in the liver GSH redox cycle of piglets suffering IUGR. According to previous research, IUGR newborns have weak protection against OS.40 Oxidative stress shifts the ratio of GSH/GSSG toward a higher GSSG content and a lower GSH content.41 Arg, which can stimulate NO production in liver, exerts a vital function in the regulation of antioxidant defense systems.15,20 Therefore, supplementing Arg or NCG in the diet would elicit protective effects on hepatic GSH as well as GSH/GSSG. As such, the latter compounds might help alleviate the IUGR-induced elevated ROS concentrations in the liver.
Increasing evidence suggests that ROS exerts a strong apoptotic effect. In our study, ROS accumulation in liver cells was markedly higher in lambs suffering from IUGR, which might have surpassed the scavenging capacity of the antioxidant defense systems. As such, numerous oxidative reactions would have begun and eventually led to oxidative injury. In the present study, the IUGR-induced increased hepatocyte apoptosis as well as necrosis level detected by Annexin V-FITC staining agreed with prior research in brain,42 kidney,43 small intestine8 and placenta.44 Such finding suggested that IUGR influenced liver development as well as metabolism through increasing apoptotic activity. Notably, this process appeared to be largely mediated by the direct or indirect action of ROS. According to prior research, mitochondrial pore oxidation induced by ROS destroyed the membrane potential of mitochondria thereby contributing to the release of cytochrome c.45 Cytochrome c oxidation plays a vital role in apoptosis,46 and availability of GSH can inactivate cytochrome c through maintaining it in a reduced state.47 Therefore, we speculate that the high proportion of apoptotic cells among IUGR lambs was mainly due to increased liver ROS content because of the lower GSH content; furthermore, the lower hepatic cell apoptosis level among animals fed with Arg or NCG supplementation diet was due partly to the higher GSH redox status of the liver.
In the present study, IUGR resulted in down-regulation of Bcl-2 mRNA expression in liver, which likely contributed to the pro-apoptotic effect of Bax.13 Bax is one of the pro-apoptotic Bcl-2 family members and can promote the release of cytochrome c by mitochondria through the formation of ion channels as well as opening of pores within the outer membrane of mitochondria.48 Thus, these results support the greater state of apoptosis and necrosis that IUGR suckling lambs experienced.
Arg has been recognized as the substrate for endothelial nitric oxide synthase (eNOS) to produce the vasoprotective molecule nitric oxide (NO).49 The NO molecule exerts vital parts in regulating Arg during intestinal epithelial cell growth as well as proliferation. Arg can promote the migration of cells through a mechanism that requires NO together with p70 S6 kinase phosphorylation.50 In the present study, supplementing Arg or NCG led to a positive effect by enhancing liver antioxidant ability in IUGR lambs potentially through its role as a precursor of NO.51 This idea is supported by the fact that supplementing Arg or NCG increased the mRNA as well as protein levels of eNOS and iNOS in liver along with NOS activity and NO content in serum and liver in animals suffering from IUG. Overall, the data suggest that Arg or NCG supplementation elicited beneficial effects on hepatic health of IUGR lambs through improving liver antioxidant ability via its effect on the NO pathway.
The antioxidant transcription factor Nrf2 can serve as an OS sensor and tightly regulate endogenous antioxidant defense systems in part by enhancing synthesis of GSH52 as confirmed by our findings. Antioxidant ability is synergistically regulated by a variety of antioxidant enzymes.20,53 Upon OS, Nrf2 disassociates from Keap1 and translocates into the nucleus, where Nrf2 can bind to the antioxidant response element (AREs) in target genes, including antioxidant enzymes such as GSH-Px, CAT and SOD together with the phase II metabolizing enzymes quinone oxidoreductase (NQO1) and heme oxygenase (HO-1).54 HO-1 is a rate-limiting enzyme and allows for the catalysis of heme into free iron, carbon monoxide (CO) and biliverdin.55 HO-1, together with metabolic products (such as bilirubin as well as CO), possesses anti-inflammatory and antioxidant properties.56 HO-1, a well-known Nrf2 target gene, can be induced in an Nrf2-dependent way.57 NQO1 is an Nrf2-mediated phase II metabolizing enzyme, which can alleviate oxidative damage through decreasing the activity of NADPH oxidase as well as the production of ROS.58 In addition, antioxidant enzymes, such as SOD, GSH-Px, CAT, and GSH exert crucial effects in maintaining overall redox homeostasis in cells.59 In the present study, Arg or NCG activated Nrf2 as well as antioxidant enzymes (such as SOD, GSH-Px1, and CAT) together with the phase II metabolizing enzymes (such as NQO1 and HO-1) in livers of IUGR animals. Arg or NCG enhanced Nrf2 protein level and up-regulate levels of antioxidant enzymes (such as SOD, GSH-Px, and CAT) together with phase II metabolizing enzymes (like NQO1 as well as HO-1) all of which contributed to reduced oxidative damage in liver of IUGR lambs.
5. Conclusions
In summary, our findings indicate that Arg or NCG can potentially protect the liver from IUGR-induced oxidative injury through a process that is achieved by enhancing levels of anti-oxidative enzymes (such as SOD, GSH-Px, and CAT), Nrf2-mediated phase II metabolizing enzymes (such as NQO1 as well as HO-1), and activating the NO pathway to alleviate oxidative damage. As such, these effects prevent excessive apoptosis and necrosis of liver tissue. Thus, Arg or NCG potentially serve as dietary supplements to help alleviate the negative effects of IUGR in newborn livestock.
Conflicts of interest
There are no conflicts to declare.
Acknowledgements
The research was supported by the fund for the National Natural Science Foundation of China (31902180), the Research Project of Natural Science Foundation of Jiangsu Province (BK20170488), China Postdoctoral Science Foundation (2017M610358), Su Bei Special Science and Technology Foundation (SZ-HA 2017008) and Jiangsu Agricultural Industry Technology System (JATS [2019]-451).
References
- G. Wu, F. Bazer, J. Wallace and T. Spencer, J. Anim. Sci., 2006, 84, 2316–2337 CrossRef CAS PubMed.
- H. Zhang, L. Sun, Z. Wang, M. Deng, G. Zhang, R. Guo, T. Ma and F. Wang, J. Anim. Sci., 2016, 94, 2072–2085 CrossRef CAS PubMed.
- H. Zhang, L. Sun, Z. Wang, M. Deng, H. Nie, G. Zhang, T. Ma and F. Wang, Reproduction, 2016, 151, 623–635 Search PubMed.
- M. Perez, M. E. Robbins, C. Revhaug and O. D. Saugstad, Free Radical Biol. Med., 2019, 49, 668–678 Search PubMed.
- S. Firmin, G. Elmhiri, D. Crepin, M. Souidi, M. Taouis and L. Abdennebi-Najar, J. Dev. Origins Health Dis., 2018, 9, 566–572 CrossRef CAS PubMed.
- J. Michiels, M. De Vos, J. Missotten, A. Ovyn, S. De Smet and C. Van Ginneken, Br. J. Nutr., 2013, 109, 65–75 CrossRef CAS PubMed.
- E. Navarro, A. N. Funtikova, M. Fíto and H. Schröder, J. Nutr. Biochem., 2017, 39, 1–14 CrossRef CAS PubMed.
- H. Zhang, F. Zhao, A. Peng, L. Dong, M. Wang, L. Yu, J. J. Loor and H. Wang, J. Agric. Food Chem., 2018, 66, 4145–4154 CrossRef CAS PubMed.
- T. Ahola, V. Fellman, I. Kjellmer, K. O. Raivio and R. Lapatto, Pediatr. Res., 2004, 56, 88–93 CrossRef CAS PubMed.
- B. Weinberger, K. Watorek, R. Strauss and G. Witz, et al., Crit. Care, 2002, 6, 521–525 CrossRef PubMed.
- A. Küster, I. Tea, V. Ferchaud-Roucher, S. L. Borgne, C. Plouzennec, N. Winer, J. C. Rozé, R. J. Robins and D. Darmaun, PLoS One, 2011, 6, e27626 CrossRef PubMed.
- Á. Ferencz, H. Orvos and E. Hermesz, Reprod. Toxicol., 2015, 53, 10–14 CrossRef PubMed.
- H. Zhang, W. Su, Z. Ying, Y. Chen, L. Zhou, Y. Li, J. Zhang, L. Zhang and T. Wang, Eur. J. Nutr., 2018, 57, 327–338 CrossRef CAS PubMed.
- H. Zhang, A. Peng, Y. Yu, S. Guo, M. Wang and H. Wang, J. Agric. Food Chem., 2019, 67, 1683–1690 CrossRef CAS PubMed.
- W. Cao, L. Xiao, G. Liu, T. Fang, X. Wu, G. Jia, H. Zhao, X. Chen, C. Wu and J. Cai, Food Funct., 2016, 7, 2303–2311 RSC.
- A. E. El-Sadek, E. G. Behery, A. A. Azab, N. M. Kamal, M. A. Salama, W. E. Abdulghany and E. A. A. Abdallah, Annals of Medicine and Surgery, 2016, 9, 22–27 CrossRef PubMed.
- X. Kong, B. Tan, Y. Yin, H. Gao, X. Li, L. A. Jaeger, F. W. Bazer and G. Wu, Biol. Reprod., 2012, 23, 1178–1183 CAS.
- H. Zhang, A. Peng, S. Guo, M. Wang, J. J. Loor and H. Wang, Food Funct., 2019, 10, 1903–1914 RSC.
- Y. Qiu, X. Yang, L. Wang, K. Gao and Z. Jiang, Int. J. Mol. Sci., 2019, 20, 1800 CrossRef CAS PubMed.
- M. Liang, Z. Wang, H. Li, L. Cai, J. Pan, H. He, Q. Wu, Y. Tang, J. Ma and L. Yang, Food Chem. Toxicol., 2018, 115, 315–328 CrossRef CAS PubMed.
- J. Liu, E. Lkhagva, H.-J. Chung, H.-J. Kim and S.-T. Hong, Nutrients, 2018, 10, 140 CrossRef PubMed.
- NRC, Nutrient requirements of small ruminants: Sheep,goats, cervids and new world camelids, National Academy Press, Washington, D.C., 2007 Search PubMed.
- L. Che and T. S. Thymann, Pediatr. Res., 2010, 67, 54–59 CrossRef PubMed.
- Y. Liu, J. Han, J. Huang, X. Wang and J. Wang, Asian-Australas. J. Anim. Sci., 2009, 22, 1667–1675 CrossRef CAS.
- R. H. Lane, S. E. Crawford, A. S. Flozak and R. A. Simmons, Am. J. Physiol.: Endocrinol. Metab., 1999, 276, E135–E142 CrossRef CAS PubMed.
- H. Zhang, Y. Chen, Y. Li, L. Yang, J. Wang and T. Wang, Br. J. Nutr., 2014, 112, 876–885 CrossRef CAS PubMed.
- H. Wang, X. Yang, Z. Zhang and H. Xu, J. Inorg. Biochem., 2003, 97, 221–230 CrossRef CAS PubMed.
- J. Xu, Liver Int., 2012, 32, 752–760 CrossRef CAS PubMed.
- H. Zhang, A. Peng, Y. Yu, S. Guo, M. Wang, D. N. Coleman, J. J. Loor and H. Wang, J. Nutr., 2019, 149, 923–932 CrossRef PubMed.
- A. Kadegowda, M. Bionaz, B. Thering, L. Piperova, R. Erdman and J. J. Loor, J. Dairy Sci., 2009, 92, 2007–2019 CrossRef CAS PubMed.
- B. Saremi, H. Sauerwein, S. Dänicke and M. Mielenz, J. Dairy Sci., 2012, 95, 3131–3138 CrossRef CAS PubMed.
- X. Tang, B. Liu, X. Wang, Q. Yu and R. Fang, Int. J. Mol. Sci., 2018, 19, 848 CrossRef PubMed.
- J. S. Chimini, J. S. Possomato-Vieira, M. L. S. da Silva and C. A. Dias-Junior, Basic Clin. Pharmacol. Toxicol., 2019, 124, 385–393 CrossRef CAS PubMed.
- R. Singh, S. Devi and R. Gollen, Diabetes/Metab. Res. Rev., 2015, 31, 113–126 CrossRef CAS PubMed.
- C. Luceri, E. Bigagli, A. P. Femia, G. Caderni, L. Giovannelli and M. Lodovici, Toxicol. Rep., 2018, 5, 141–145 CrossRef CAS PubMed.
- J. He, Y. Niu, F. Wang, C. Wang, T. Cui, K. Bai, J. Zhang, X. Zhong, L. Zhang and T. Wang, Br. J. Nutr., 2018, 120, 537–548 CrossRef CAS PubMed.
- B. R. Stockwell, J. P. F. Angeli and H. Bayir, Cell, 2017, 171, 273–285 CrossRef CAS PubMed.
- G. B. Nogueira, G. R. Punaro, C. S. Oliveira, F. R. Maciel, T. O. Fernandes, D. Y. Lima, A. M. Rodrigues, M. G. Mouro, S. R. R. Araujo and E. M. S. Higa, Nitric Oxide, 2018, S1089860317302562 Search PubMed.
- P. Korge, G. Calmettes and J. N. Weiss, Biochim. Biophys. Acta, Bioenerg., 2015, 1847, 514–525 CrossRef CAS PubMed.
- Z. Hracsko, H. Orvos, Z. Novak, A. Pal and I. S. Varga, Redox Rep., 2008, 13, 11–16 CrossRef CAS PubMed.
- F. Echeverría, R. Valenzuela, A. Bustamante, D. Álvarez, M. Ortiz, S. A. Soto-Alarcon, P. Muñoz, A. Corbari and L. A. Videla, Oxid. Med. Cell. Longevity, 2018, 2018, 5109503 Search PubMed.
- A. M. Maliszewski-Hall, M. Alexander, I. Tkáč, G. Öz and R. Rao, Neurochem. Res., 2017, 42, 133–140 CrossRef CAS PubMed.
- G. Elmhiri, D. F. D. Mahmood, C. Niquet-Leridon, P. Jacolot, S. Firmin, L. Guigand, F. J. Tessier, T. Larcher and L. Abdennebi-Najar, Mol. Nutr. Food Res., 2015, 59, 939–947 CrossRef CAS PubMed.
- S. S. Chassen, V. Ferchaud-Roucher, M. B. Gupta, T. Jansson and T. L. Powell, Clin. Sci., 2018, 132, CS20171340 Search PubMed.
- H. A. Kalpage, V. Bazylianska, M. A. Recanati, A. Fite, J. Liu, J. Wan, N. Mantena, M. H. Malek, I. Podgorski, E. I. Heath, A. Vaishnav, B. F. Edwards, L. I. Grossman, T. H. Sanderson, I. Lee and M. Hüttemann, FASEB J., 2019, 33, 1540–1553 CrossRef CAS PubMed.
- M. Pinto, U. D. Vempati, F. Diaz, S. Peralta and C. T. Moraes, Mol. Neurobiol., 2019, 56, 3722–3735 CrossRef CAS PubMed.
- A. E. Vaughn and M. Deshmukh, Nat. Cell Biol., 2008, 10, 1477–1483 CrossRef CAS PubMed.
- D. R. Green and J. C. Reed, Science, 1998, 281, 1309–1312 CrossRef CAS PubMed.
- R. E. Oberkersch and M. M. Santoro, Vasc. Pharmacol., 2019, 112, 17–23 CrossRef CAS PubMed.
- J. M. Rhoads, Y. Liu, X. Niu, S. Surendran and G. Wu, J. Nutr., 2008, 138, 1652–1657 CrossRef CAS PubMed.
- M. T. Mansouri, B. Naghizadeh, B. Ghorbanzadeh, S. Alboghobeish, G. Houshmand and N. Amirgholami, Endocr., Metab. Immune Disord.: Drug Targets, 2018, 18, 362–370 CrossRef CAS PubMed.
- H. Li, H. He, Z. Wang, J. Cai, B. Sun, Q. Wu, Y. Zhang, G. Zhou and L. Yang, Gene, 2016, 585, 256–264 CrossRef CAS PubMed.
- T. Suzuki and M. Yamamoto, Free Radical Biol. Med., 2015, 88, 93–100 CrossRef CAS PubMed.
- J.-S. Park, Y.-Y. Lee, J. Kim, H. Seo and H.-S. Kim, Free Radical Biol. Med., 2016, 97, 168–178 CrossRef CAS PubMed.
- S. You, E. Nakanishi, H. Kuwata, J. Chen, Y. Nakasone, X. He, J. He, X. Liu, S. Zhang and B. Zhang, Mol. Nutr. Food Res., 2013, 57, 2049–2060 CrossRef CAS PubMed.
- L. Rochette, M. Zeller, Y. Cottin and C. Vergely, Trends Endocrinol. Metab., 2018, 29, S1043276017301662 CrossRef PubMed.
- H. Li, Z. Tang, P. Chu, Y. Song, Y. Yang, B. Sun, M. Niu, E. Qaed, A. Shopit and G. Han, Free Radical Biol. Med., 2018, S0891584918301175 Search PubMed.
- Y. Mizunoe, S. Watanabe, Y. Sudo, M. Kobayashi and Y. Higami, Redox Biol., 2018, 15, 115–124 CrossRef CAS PubMed.
- T. Corsello, N. Komaravelli and A. Casola, Antioxidants, 2018, 7, 129 CrossRef PubMed.
|
This journal is © The Royal Society of Chemistry 2020 |