DOI:
10.1039/C9RA09040A
(Paper)
RSC Adv., 2020,
10, 11573-11581
Liposomes co-delivery system of doxorubicin and astragaloside IV co-modified by folate ligand and octa-arginine polypeptide for anti-breast cancer
Received
1st November 2019
, Accepted 1st March 2020
First published on 20th March 2020
Abstract
Doxorubicin (DOX) is one of the core drugs in triple-negative breast cancer (TNBC) chemotherapy, but its resistance has severely limited its clinical application. Our previous study found that astragaloside IV (AS-IV) has a good reversal effect on doxorubicin resistance. In order to encapsulate DOX and AS-IV simultaneously, a new liposome-targeted co-delivery system co-modified by the folate ligand (FA) and octa-arginine polypeptide (R8) (FA-R8-LPs, for short) was prepared. In this co-delivery system, R8 not only served as a bond connecting the FA to the liposome, but also played the role of cell penetrating peptides (CPPs). This design effectively increased the tumor targeting and cellular uptake capacity of liposomes. The results of the cytotoxicity test indicated that FA-R8-LPs significantly inhibited the proliferation of the DOX resistant cell line MDA-MB-231/DOX in vitro. In nude mice tumor models inoculated with MDA-MB-231/DOX cells, FA-R8-LPs significantly inhibited tumor growth, and overcame doxorubicin resistance, exhibiting excellent antitumor effects. This study demonstrates that liposome-targeted co-delivery systems based on FA and R8 double modifying may provide a new and effective strategy for the treatment of TNBC, which is of great significance for drug combination.
1 Introduction
Breast cancer is the most common malignant tumor type in women worldwide, and the incidence of triple-negative breast cancer (TNBC) accounts for about 15% to 20% of the total number of breast cancers.1 Anthracyclines represented by doxorubicin (DOX) are indispensable core drugs for TNBC chemotherapy and can be used for the treatment of early TNBC.2 However, DOX resistance cannot be ignored and has severely limited its clinical application. Therefore, actively looking for a high-efficiency, low-toxic, broad-targeted tumor resistance reversal agent has become a key target in this research field.
Astragalus membranaceus is a Chinese herbal medicine with a long history and high medicinal value. It is often used as an immunomodulator to improve the side effects of anti-tumor drugs. Astragaloside IV (AS-IV) is a biologically active saponin in Astragalus membranaceus and has strong antioxidant activity.3 At present, most research is on the toxicity-attenuating effect of AS-IV on chemotherapeutic drugs. It has also been reported that AS-IV can sensitize liver cancer cells to drug resistance.4 Our previous study found that the half maximal inhibitory concentration (IC50) of DOX on sensitive MDA-MB-231 cells was 0.3990 ± 0.0322 μmol L−1, and it was 5.3110 ± 0.0714 μmol L−1 on MDA-MB-231/DOX cells.5 When AS-IV and DOX acted together on drug-resistant cells, the IC50 recovered to 1.5753 ± 0.0376 μmol L−1, and the reversal multiple was 3.37 times. The reversal effect of AS-IV to DOX resistance was obvious. The DOX used above is in the form of hydrochloride. Doxorubicin hydrochloride has a good solubility, but the water solubility of AS-IV is very poor. The solubility difference between them affects their combination.
Liposome is a commonly used nanocarrier with strong biocompatibility.6 It can contain not only hydrophobic components but also hydrophilic components, and is able to achieve the co-delivery of DOX and AS-IV. But the tumor targeting of common liposomes is a passive targeting based on the enhanced permeability and retention (EPR) effect, and it has disadvantages such as poor selectivity, easy diffusion, easy leakage and so on.7 Folate receptor (FR) is highly expressed on the surface of many malignant tumor cells, such as TNBC subtype tumor cells.8 Therefore, folate, as a specific recognition ligand, is usually attached to the surface of liposomes, specifically binds to the FR overexpressed on the surface of the malignant tumor cells, localizes and aggregates the liposomes into the tumor tissue to achieve active targeting. However, the number of FR on the surface of TNBC subtype tumor cells is limited, and there is a ligand–receptor-specific binding saturation phenomenon that affects the efficient uptake of drugs. Octa-arginine polypeptide (R8) is a transmembrane peptide composed of 8 arginine, which can penetrate any cell membrane in contact with it, and can high-efficiently mediate nanometer drug system, protein, and nucleic acid entering into cells.9 The transmembrane peptides characteristics has no saturation phenomenon, but often lacks tissue selectivity. Therefore, the double-modified liposome delivery system was constructed to achieve complementary advantages by combining with the targeting specificity of FA and the high-efficiency internalization ability of R8.
In order to create sufficient space for FA binding to FR, long-chain ligands have often been used, and they are known as “connecting bridge”. R8, a long polypeptide chain polymerized by 8 arginine, has the potential to be a “connecting bridge”, which can serve as a bond connecting the FA to the liposome (Fig. 1). Herein, this study successfully synthesized a novel FA-R8-cholesterol ligand (Fig. 2), and constructed a novel liposome with specific targeting and high-efficiency transmembrane action. Subsequently, mass spectrometry and high performance liquid chromatography (HPLC) were used to characterize the above liposomes, and flow cytometry and laser confocal microscopy were used to evaluate targeting ability in vitro, and small live animals imaging technology was adopt to evaluate targeting effect in vivo. Then, both DOX and AS-IV were incorporated into the co-modified liposomes to construct a drug co-delivery system (FA-R8-LPs-DOX/AS, for short). Finally, cytotoxicity test and nude mice tumor model were used for pharmacodynamic evaluation. The study of this novel liposome-targeted co-delivery system may achieve efficient targeted delivery of anti-cancer drugs to improve TNBC chemotherapy. The details are as follows.
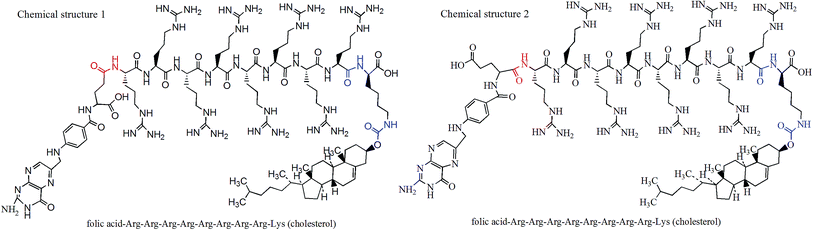 |
| Fig. 1 Chemical structure of FA-R8-cholesterol. It is a mixture of chemical structure 1 and chemical structure 2 shown in the figure. | |
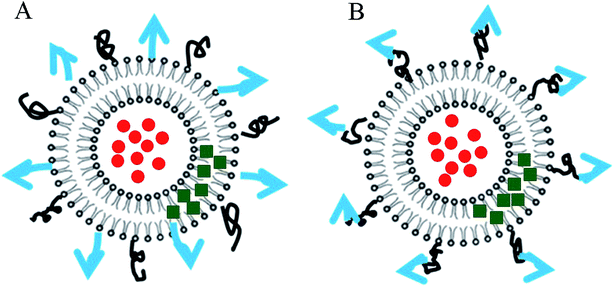 |
| Fig. 2 Schematic diagram of FA-R8-LPs. Blue arrow represents FA and black curve represents R8. (A) The conventional type (B) the new liposome designed in this study. | |
2 Materials and methods
2.1 Materials
Doxorubicin hydrochloride (DOX·HCl) and astragaloside IV were provided by Nantong Feiyu Biological Technology (Nantong, China). R8-Lys-cholesterol was received from Beijing Scilight Biotechnology LLC (Beijing, China). Folic acid was obtained from DSM Nutritional Products Ltd (Heerlen, Netherlands). 1-Ethyl-(3-dimethylaminopropyl)carbonyldiamine hydrochloride (EDC·HCL), 1-hydroxybenzotriazole (HOBT) and 4-dimethylaminopyridine (DMAP) were purchased by Beijing BioWay Biotechnology LLC (Beijing, China). Dimethylformamide (DMF), trifluoroacetic acid (TFA), triisopropylsilane (Tis) and 1,2-ethanedithiol (EDT) were provided by Beijing InnoChem Science & Technology (Beijing, China). Dichloromethane and NaHCO3 were provided by Beijing Chemical Works. 10% fetal bovine serum was provided by Zhejiang Tianhang Biotechnology (Zhejiang, China). Phosphate buffer solution (PBS), 1% penicillin streptomycin and RPMI 1640 medium were obtained from Mediatech, Inc. (USA). Dimethyl sulfoxide (DMSO) were provided by Amresco (USA). Near-infrared radiation fluorescent dye DIR was purchased by Caliper (USA). MTT reagent kit was provided by Beijing BioDee Biotechnology (Beijing, China).
2.2 Cell cultures and animals
Human breast adenocarcinoma MDA-MB-231 cell lines were initially obtained from Nanjing KeyGen Biotechnology (Nanjing, China). MDA-MB-231/DOX cell line was obtained from Shanghai YuBo Biotechnology (Shanghai, China). MDA-MB-231 cells and MDA-MB-231/DOX cells were cultured in RPMI 1640 medium containing 10% fetal bovine serum and 1% penicillin streptomycin in a 37 °C, 5% CO2 incubator. The cells were placed in an adherent state, and the cells were grown to a logarithmic growth state for use.
Female nude mice were purchased from Beijing Vital River Laboratory Animal Technology (Beijing, China). All animals received care following the guidelines outlined in the “Guide for the Care and Use of Laboratory Animals”. Cell suspension of MDA-MB-231/DOX cell line with high tumor formation rate was inoculated into the breast of nude mice to establish a breast cancer nude mouse model. All animal experiments were approved and executed under the directions of the ethical committee of Beijing University of Chinese Medicine.
2.3 Synthesis and characterization of FA-R8-cholesterol derivative
R8 was synthesized by solid phase synthesis, next its –COOH terminus connected a lysine (Lys, has two –NH2 terminus) and the other –NH2 terminus reacted with the –COCl of cholesterol methyl esters. Here the characteristics is the design of lysine as a bridge. With the help of peptide synthesis company, R8-Lys-cholesterol with –NH2 terminus was synthesized. Another synthetic ingredient is FA with a –NH2 terminus and a –COOH terminus. The target site for the specific binding of FA to its receptor is –NH2 terminus, which is highly prone to chemical reactions and lost activity. Therefore, the EDC·HCL/HOBT/DMAP system was chosen as the coupling agent, which activated the FA under mild conditions and greatly improved product purity.10,11 25 mg FA dissolved in 10 mL dimethylformamide (DMF), added 15 mg EDC·HCL, 10 mg HOBT and 1.5 mg DMAP, reacted for 2 h with continuous stirring at room temperature. Next, 70 mg R8-Lys-cholesterol dissolved in 10 mL dichloromethane (DCM) and slowly added to the above reaction solution, continue magnetic stirring at room temperature, added water to quench the reaction after 48 h, extracted with DCM, and used 5% NaHCO3 solution to remove residual FA in the reaction, repeated three times. The DCM layer wash with water, 0.1 mol L−1 hydrochloric acid and water saturated NaCl solution in turn for two times, concentrated and dried to obtain solids, redissolved with DMF. Then, added 5 mL trifluoroacetic acid–triisopropylsilane–EDT–water (95
:
2
:
2
:
1, v/v) mixed solvent to the above DMF solution, concentrated after reacting for 1.5 h, added 30 mL ether, centrifugated and acquired sediment. To remove other impurities, the sediment was subjected to dialysis for 48 h in DMF using dialysis bags with a 1000 molecular weight cut-off. Finally, the final samples were freeze-dried to obtain a novel FA-R8-cholesterol derivative, and the yield was 63.16%.
The Matrix Assisted Laser Desorption Ionization-Time of Flight-Mass Spectrometer (MALDI-TOF-MS) is often used to predict the molecular weight of large molecular compounds.12 The molecular weight was determined by MALDI-TOF-MS (Voyager-DE-PRO, Applied Biosystem, USA) to verify the successful synthesis of the FA-R8-cholesterol derivative. The conditions were set as follows: the substrate was a tetrahydrofuran saturated solution of α-cyano-4-hydroxycinnamic acid, the solid nitrogen laser emission wavelength was 355 nm, the reflector mode was positive ion mode, the acceleration voltage was 20 kV, and the product was dissolved in chloroform (10 mg mL−1). In addition, purity of the FA-R8-cholesterol derivative was measured by HPLC (LC-10AT, Shimadzu, Japan). The mobile phase consisted of 0.05% TFA–2% acetonitrile (phase A) and 0.05% TFA–90% acetonitrile (phase B), and 40–58% B-phase gradient elution at 0–18 min. The flow rate was 1 mL min−1, and the column temperature was 30 °C. The samples were injected into an Agilent SB-C18 column (4.6 mm × 150 mm, 5 μm), and purity of the product was measured at a wavelength of 220 nm.
2.4 Preparation of FA-R8-LPs-DOX/AS
FA-R8-LPs-DOX/AS was prepared by ethanol injection-ammonium sulfate gradient method.13 Firstly, the encapsulation of AS-IV was carried out by ethanol injection method. AS-IV, S100 phospholipid, cholesterol and FA-R8-cholesterol were co-dissolved in an appropriate amount of absolute ethanol to form an oil phase. The ammonium sulfate solution (200 mmol L−1) was used as an aqueous phase. Under ultrasonic conditions, the oil phase was injected into the water phase at a constant rate to form an emulsion in which the water and oil are uniformly dispersed. After recovering the ethanol, it was filtered through a 0.1 μm microporous membrane and a dialysis bag with a molecular weight cut off of 3500 was used to obtain FA-R8-LPs-AS. Secondly, the encapsulation of DOX was carried out by ammonium sulfate gradient method. DOX aqueous solution (1.0 mg mL−1) was added to FA-R8-LPs-AS, and after incubating for 30 min in a 50 °C water bath. It was magnetically stirred and filtered through an ultrafiltration membrane with a molecular weight cut off of 10
000 to obtain FA-R8-LPs-DOX/AS.
2.5 Characterization of FA-R8-LPs-DOX/AS
The particle size, PDI value and zeta potential of liposomes were determined by using a Nano-ZS90 Laser Particle Size Analyzer (Malay Instruments, UK) before and after lyophilization. After staining with 2% phosphotungstic acid, the morphology of the liposome was observed under a transmission electron microscopy (JEM-1230 (HC), JEOL Ltd.). Infrared radiation (IR) spectrum of FA-R8-LPs-DOX/AS lyophilized powder, FA-R8-LPs-empty lyophilized powder and blank liposome with DOX/AS-IV were obtained by using an ANTARIS infrared radiation spectrometer (Thermo Nicolet, USA).
To evaluate the encapsulation efficiency (EE) and drug loading (DL) of DOX and AS-IV in the co-delivery system, after demulsification with methanol, AS-IV in liposome was determined with HPLC (AGILENT 1100, Agilent, USA). The mobile phase consisted of a mixture of acetonitrile and water (35
:
65), and the flow rate was 1 mL min−1. The column temperature was 30 °C. Twenty microliters of sample were injected into a SB-C18 column (4.6 mm × 150 mm, 5 μm), and AS-IV was detected by evaporative light scattering detector. DOX in liposome was determined with HPLC (LC-10AT, Shimadzu, Japan). The mobile phase consisted of a mixture of acetonitrile and 1% phosphoric acid solution (27
:
73), and the flow rate was 1 mL min−1. Twenty microliters of sample was injected into a C18 column (4.6 mm × 250 mm, 5 μm), and DOX was measured at a wavelength of 232 nm. According to the following formula:
|
 | (1) |
|
 | (2) |
2.6 Cellular uptake assays
To investigate the targeted evaluation of FA-R8-LPs in vitro, MDA-MB-231/DOX cells were cultured in 6-well plates at a density of 1 × 106 cells per well. After 24 h of incubation, the cells were treated with free DOX, LPs-DOX, R8-LPs-DOX and FA-R8-LPs-DOX at 4.6 μmol L−1 DOX concentration for 0.5 h, 1.0 h and 2.0 h, respectively. Subsequently, the cells were washed three times with PBS, and 200 μL PBS was added to prepare a cell suspension. FACSCanto II Flow cytometry (Becton & Dickinson, USA) was used to analyze the cellular uptake of DOX in each group.
To more visually observe the tumor cells uptake results after being treated with different forms of DOX, observations were performed under a FV1000 confocal laser scanning microscope (CLSM, Olympus, Japan). Briefly, MDA-MB-231/DOX cells were cultured in the confocal small dishes at a density of 1 × 105 mL−1. After 24 h of incubation, the cells were treated with free DOX, LPs-DOX, R8-LPs-DOX and FA-R8-LPs-DOX at 4.6 μmol L−1 DOX concentration for 1 h, followed by three washes with PBS. Finally, the subcellular distribution of DOX and liposome drugs was visualized by CLSM.
2.7 Biodistribution analyses
Live imaging technology of small animals was adopted to evaluate targeting effect in vivo and the model drug is DIR (a near infrared fluorescent dyes).14 MDA-MB-231/DOX tumor-bearing nude mice were tail-intravenous injection of DIR, R8-LPs-DIR and FA-R8-LPs-DIR of 0.1 mL (that DIR dose is 1 μg), respectively. A small animal three-dimensional optical in vivo imaging system (IVIS Spectrum CT, Caliper-PerkinElmer, USA) was used to assess the biodistribution of DIR at 0.5, 1, 2, 4, 6, 8, 12, 24 h after injection, respectively. When the in vivo imaging scan was completed, the main organs including the heart, spleen, liver, kidney and tumor were excised and observed by the fluorescence imaging system.
2.8 Cell proliferation assays
To determine the antitumor efficacy of co-loaded liposomes in vitro, inhibitory effect of FA-R8-LPs-DOX/AS on the proliferation of MDA-MB-231 cells and MDA-MB-231/DOX cells were examined by MTT assay. MDA-MB-231 cells and MDA-MB-231/DOX cells were respectively cultured in 96-well plates at a density of 1.5 × 104 and 3 × 104 cells per well. After 24 h of incubation, the cells were treated with free DOX/AS-IV, LPs-DOX/AS, R8-LPs-DOX/AS, FA-R8-LPs-DOX/AS for 72 h. Subsequently, the cells were washed twice with PBS and then further added MTT solution (5 mg mL−1) and continue to incubate in the incubator for 4 h. Next, the MTT solution was discarded and 150 μL dimethyl sulfoxide (DMSO) was added. The absorbance (OD value) of each well at 550 nm was measured with an ELX-800 microplate reader (Biotek, USA). Finally, the half-inhibitory concentration (IC50, expressed as DOX) concentration of each group of tumor cells was calculated using GraphPad Prism 5.0 software.
2.9 Anti-tumor efficacy in vivo
To further investigate the antitumor efficacy of FA-R8-LPs-DOX/AS in vivo, MDA-MB-231/DOX tumor-bearing mice model was treated by different DOX formulation. When the tumor sizes of breast cancer-resistant nude mice reach about 100 mm3, the mice were randomly divided into 6 groups of 7 mice each according to tumor volume and body weight. At pre-determined time, MDA-MB-231/DOX tumor-bearing mice were intravenously given saline, free DOX, LPs-DOX, LPs-DOX/AS, R8-LPs-DOX/AS and FA-R8-LPs-DOX/AS at a DOX (0.08 mg mL−1) dose of 8 mg kg−1. Mice were weighed twice a week while measuring the tumor long diameter (a) and short diameter (b) by using a Vernier calipers. Respective tumor volume (TV) and tumor growth inhibition rate (TGI) were calculated based on the formulas: |
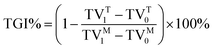 | (4) |
TVT1: mean tumor volume in treatment group, TVT0: mean tumor volume in before treatment group, TVM1: mean tumor volume in model group, TVM0: mean tumor volume in before model group.
2.10 Statistical analysis
Analysis of variance and LSD test in SPSS 21.0 statistical software was used to compare differences between groups. Only *P < 0.05 was considered significant. Experimental datum was presented as mean ± SEM.
3 Results and discussion
3.1 Synthesis and characterization of FA-R8-cholesterol derivative (FA-R8)
To combine the targeting specificity of FA and the high internalization ability of R8 to achieve complementary advantages, FA and R8 were co-modified on the surface of the liposome. Based on previous studies, a liposome membrane was selected to constitute cholesterol, which was inserted into the bilayer of the liposome to introduce a FA on the surface of the liposome. Folic acid and cholesterol were linked by transmembrane peptide R8, which acted as a “bridge” (Fig. 2). Next, a peak molecular weight of about 22
233.29 Da was shown by MALDI-TOF-MS as shown in Fig. 3, which was close to the theoretical molecular weight of 2231.70. In addition, purity of FA-R8-cholesterol was determined by HPLC to be 80.46%. These results indicated that FA-R8-cholesterol was successfully synthesized.
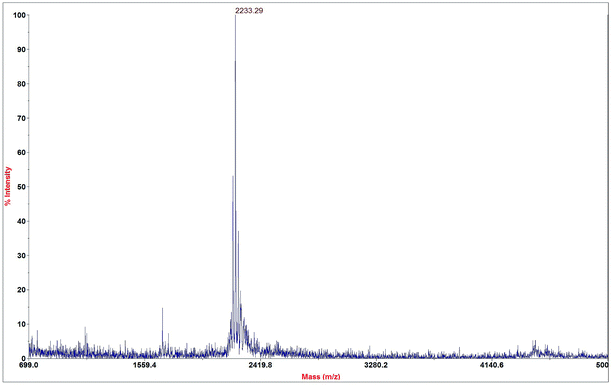 |
| Fig. 3 The MALDI-TOF-MS spectrogram of FA-R8-Cys derivative. Its peak molecular weight was 2 233.29 Da, which was approximately equal to the theoretical molecular weight. | |
3.2 Characterization of FA-R8-LPs-DOX/AS
To achieve co-administration of DOX and AS-IV, FA-R8-LPs-DOX/AS was prepared by ethanol injection-ammonium sulfate gradient method. Particle size measurement results (Table 1) showed that the liposome particle size was 109.0 ± 6.1 nm before lyophilization, which was similar to that of 130.7 ± 3.7 nm after lyophilization. It showed that FA-R8-LPs-DOX/AS had excellent physical stability. Electron microscope images (Fig. 4A) revealed that the liposome was spherical, the particle size distribution was relatively uniform, and the particle size was about 100 nm. These results indicated that FA-R8-LPs-DOX/AS was got uniform size and stable quality. The IR spectrum (Fig. 4B) shows that the blank liposome with drug has characteristic peaks at 1577.18 cm−1 and 1524.63 cm−1 which were peaks of drugs, but they were not found in both the blank liposome and the drug-loaded liposome. It indicated that the characteristic peak of the drug disappeared after liposome encapsulation. In other words, drugs were stable encapsulated in the liposome.
Table 1 Particle size, EE and DL of FA-R8-LPs-DOX/AS before and after freeze-drying
Freeze-dried state |
Particle size (nm) |
PDI value |
Zeta potential (mV) |
DOX |
AS-IV |
EE (%) |
DL (%) |
EE (%) |
DL (%) |
Before |
109.0 ± 6.1 |
0.253 ± 0.060 |
−15.9 ± 1.7 |
98.57 |
4.68 |
98.49 |
14.26 |
After |
130.7 ± 3.7 |
0.265 ± 0.008 |
−16.2 ± 1.5 |
98.06 |
4.66 |
95.79 |
13.87 |
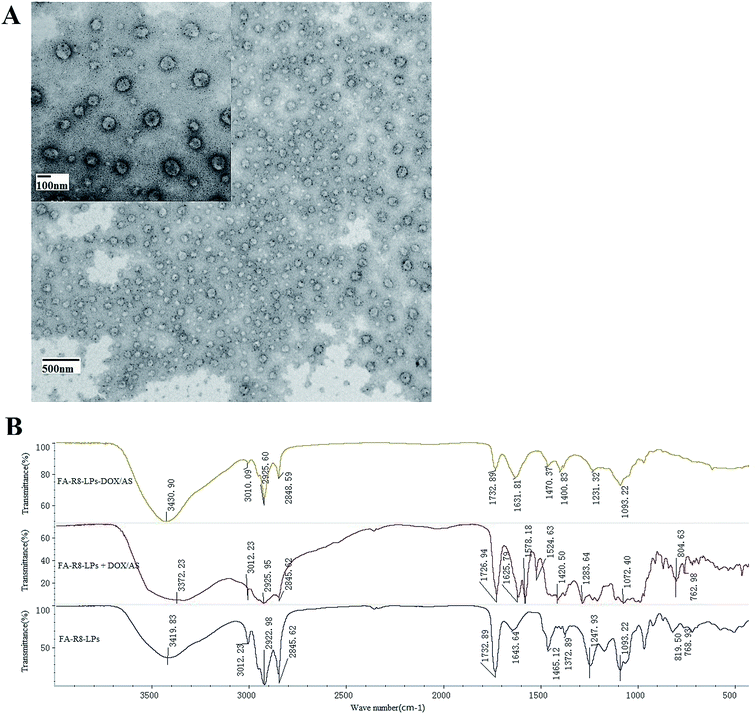 |
| Fig. 4 Characterization of FA-R8-LPs-DOX/AS. (A) Morphological characteristics under transmission electron microscope. (B) IR spectrogram of drug-carrying liposomes, blank liposomes and mixture. | |
To investigate the synergistic effects of dual-drug liposomes, the high encapsulation efficiency (EE) and drug loading (DL) of both drugs are an essential prerequisite for precise control of dual-drag ratios. The results of the EE and DL of DOX and AS-IV in FA-R8-LPs-DOX/AS liposomes before and after lyophilization are shown in Table 1. It showed that FA-R8-LPs-DOX/AS had excellent double drugs encapsulation effect, which will provide a solid foundation for its efficacy.
3.3 Results of targeted evaluation in vitro
To determine the targeting of the novel liposomes, the cellular uptake of FA-R8 double-modified liposomes was determined by flow cytometry. Free DOX, LPs-DOX and R8-LPs-DOX displayed enhanced cellular uptake by MDA-MB-231/DOX cells in a time-dependent manner (Fig. 5A). Compared with free DOX, DOX was significantly increased after being encapsulated by liposomes. In contrast, DOX uptake of FA-R8-LPs-treated tumor cells was much higher than that of other groups, and it was suggested that liposomes modified by FA-R8 can enhance drug cellular uptake.
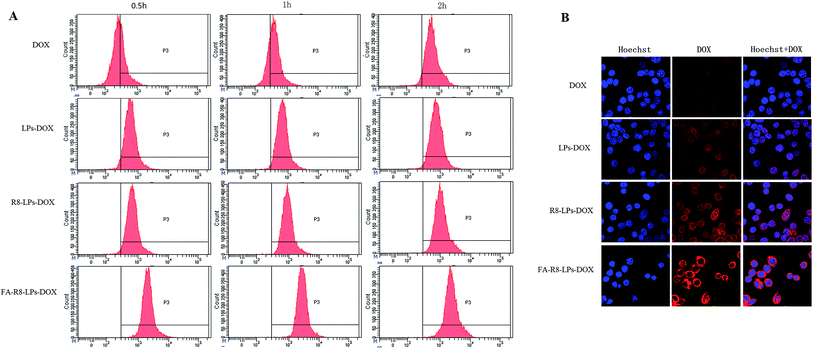 |
| Fig. 5 Cellular uptake and intracellular localization. (A) Cellular uptake diagram was obtained by flow cytometry. (B) Determination results by confocal microscopy. | |
The increased internalization of FA-R8 double-modified liposomes into MDA-MB-231/DOX cells was further confirmed by confocal laser scanning microscopy (Fig. 5B). DOX fluorescence intensity in FA-R8-LPs-treated tumor cells was much stronger than that of other groups. In addition, DOX had been found to focus around the nucleus after entering cells. The results demonstrated that FA-R8 co-modified liposomes could significantly increase the accumulation of DOX around the nucleus. The increasing of drug concentration around the nucleus will effectively increase the anti-tumor effect and gain more chemotherapy benefits.
3.4 Results of targeted evaluation in vivo
To further evaluate the targeting effect of FA-R8 co-modified liposomes in vivo, the biodistribution profiles of different liposomes were determined in tumor-bearing nude mice. After intravenous injection of DIR into the tail of tumor-bearing nude mice, fluorescence intensity of the tumor site in FA-R8-LPs-DIX group was higher than that of other three groups from the eighth hour (Fig. 6A). And fluorescence imaging of isolated organs also confirmed this (Fig. 6B). Compared with R8-LPs, FA-R8-LPs showed better targeting effect after adding FA, and R8-LPs was better than LPs. The results showed that double modification of FA and R8 increased the distribution of liposomes in tumors.
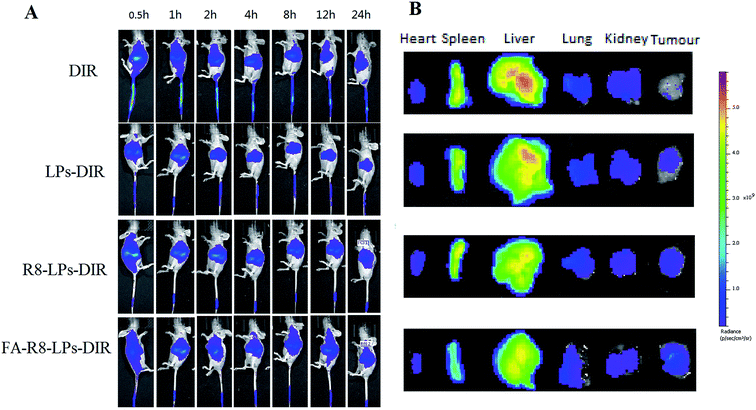 |
| Fig. 6 Results of targeted evaluation in vivo by small live animal imaging technology. (A) Fluorescence imaging diagram of small animals in vivo after tail intravenous injection of DIR. (B) Fluorescence imaging of isolated organs diagram. | |
3.5 Results of anti-tumor cell proliferation
Tumor cell proliferation effect of liposomes was determined by MTT assay. The IC50 value of DOX in MDA-MB-231 cell line was 0.3990 ± 0.0322 μM, consisting with the previous reports.15,16 MDA-MB-231/DOX cell line developed severe resistance to DOX and the IC50 value was up to about 5 μM. After intervention with AS-IV and DOX simultaneously, the IC50 value decreased to about 2 μM, which indicated that AS-IV increased the sensitivity. As listed in Table 2, the IC50 value of FA-R8-LPs-DOX/AS in MDA-MB-231/DOX cell line was less than other groups, and it was close to that of DOX in sensitive cell line, suggesting that FA-R8 double-modified co-loading liposomes had the strongest effect on MDA-MB-231/DOX cells proliferation.
Table 2 Proliferation inhibition to MDA-MB-231 cells and MDA-MB-231/DOX cells (n = 3)a
Compounds |
IC50 (μM, mean ± SEM) |
To MDA-MB-231 cells |
To MDA-MB-231/DOX cells |
*P < 0.05, **P < 0.01, compared with DOX/AS group. |
DOX |
0.3990 ± 0.0322 |
5.3110 ± 0.0714 |
DOX/AS |
0.2776 ± 0.0039 |
1.9840 ± 0.0497 |
LPs-DOX/AS |
0.2388 ± 0.0077* |
1.0310 ± 0.0012* |
R8-LPs-DOX/AS |
0.2163 ± 0.0034** |
0.9019 ± 0.0077** |
FA-R8-LPs-DOX/AS |
0.1846 ± 0.0083** |
0.3279 ± 0.0045** |
3.6 Results of anti-tumor efficacy in vivo
The anti-tumor effect of different DOX formulation was further investigated in MDA-MB-231/DOX tumor-bearing nude mice model. Changes in body weight are considered as a crucial indicator of systemic safety. There was no significant difference (P > 0.05) between each group (Fig. 7A), suggesting that FA-R8-LPs-DOX/AS did not show obvious systemic toxicity. The tumor volume change from the first day to the thirtieth day of nude mice in each group after the administration was shown in Fig. 7B. Tumor volume in the DOX group was slightly smaller than that in the model group after administration 30 days, but there was no statistical difference (p > 0.05). It indicated that the resistant animal model of breast cancer was resistant to DOX. Compared with the model group, the tumor volume of the LPs-DOX/AS, R8-LPs-DOX/AS, and FA-R8-LPs-DOX/AS group decreased significantly from 14 days after administration, and it was the smallest in the FA-R8-LPs-DOX/AS group.
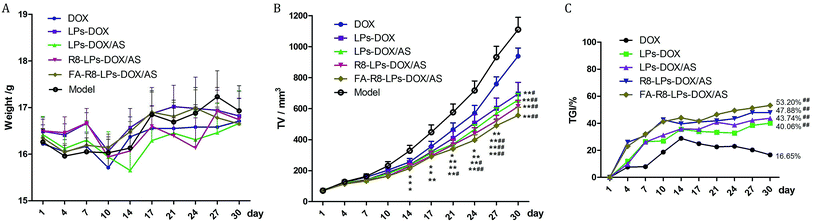 |
| Fig. 7 Results of anti-tumor efficacy in vivo. (A) Changes of animal body weight (B) changes of tumor volume. (C) Changes of TGI. Compared with the control group, *P < 0.05, **P < 0.01; compared with the DOX group, #P < 0.05, ##P < 0.01. | |
From TGI results (Fig. 7C), TGI of the DOX group gradually increased in the first 2 weeks after injection of DOX, but that gradually decreased in the second 2 weeks after administration. The above results indicated that drug resistance gradually emerged with the extension of administration time. TGI of each liposome administration group was higher than that of the DOX group at each time point of administration, and the TGI of each group was gradually increased. Among all groups, the FA-R8-LPs-DOX/AS group exhibited the best anti-tumor efficacy, achieving 53.20% inhibition efficacy 30 days after administration.
4 Conclusion
This study developed a novel liposomes co-delivery system of DOX and AS-IV co-modified by FA and R8 for anti-triple negative breast cancer. The novel liposome system not only realized the co-delivery of two drugs with greatly different solubility, but also successfully combined the targeting specificity of FA ligand and the high-efficiency internalization ability of R8 to achieve excellent tumor targeting and anti-tumor effects. Compared with drug-loaded liposomes without modification and R8 single modification, FA-R8 double-modified drug-loaded liposomes showed higher proliferation inhibition rate to MDA-MB-231/DOX cells, and the effect of reversing DOX resistance was obvious. Therefore, the FA-R8 double-modified liposome co-delivery system might provide a new method for the treatment of TNBC.
In addition, breast cancer, as a superficial cancer type, can be tried by transdermal administration to reduce the heart and kidney toxicity caused by anthracyclines such as DOX. Because of the introduction of transmembrane peptide (R8), the above drug-loading liposomes may have certain advantages in the development of new anti-breast cancer formulation. For further research, it is needed to evaluate the targeting and effectiveness of the FA-R8-LPs-DOX/AS by transdermal administration.
Funding
No funding.
Ethical approval
All animal procedures were performed in accordance with the Guidelines for Care and Use of Laboratory Animals of Beijing University of Chinese Medicine and approved by the Animal Ethics Committee of Beijing University of Chinese Medicine.
Conflicts of interest
All authors declare that they have no conflict of interest.
Acknowledgements
We gratefully acknowledge the help of Beijing Microspin Gene Technology Corporation and Beijing Scilight Biotechnology LLC in cell culture and solid-phase synthesis of R8-Lys-cholesterol, respectively.
References
- Z. M. Shao, Into a new era: 30 years of breast cancer diagnosis and treatment, J. Pract. Oncol., 2016, 31(3), 195–199 Search PubMed.
- H. Masuda, K. A. Baggerly and Y. Wang, et al., Differential response to neoadjuvant chemotherapy among 7 triple-negative breast cancer molecular subtypes, Clin. Cancer Res., 2013, 19(19), 5533–5540 CrossRef CAS PubMed.
- L. Lin, Experimental study on effect of AS IV on ADR-enduced H9C2 cardiomyocytes apoptosis in rats, Nanjing University of Chinese Medicine, 2016 Search PubMed.
- Y. Tian, Q. Miao and H. Zhao, et al., Reversal effect of astragaloside IV on multidrug resistance of drug-resistant hepatocellular carcinoma cell line HepG2/GCS, Chin. Rem. Clin., 2011, 11(7), 778–779 Search PubMed.
- G. Yue, Breast cancer targeting co-delivery system of DOX and AS-IV by FA and R8 co-modified liposome, Beijing University of Chinese Medicine, 2018 Search PubMed.
- J. Li, Z. Yang and T. Meng, et al., The use of cationic liposomes to codeliver docetaxel and siRNA for targeted therapy of hepatocellular carcinoma, J. Chin. Pharm. Sci., 2014, 23(10), 667–673 CAS.
- E. Gullotti and Y. Yeo, Extracellularly activated nanocarriers: a new paradigm of tumor targeted drug delivery, Mol. Pharm., 2009, 6(4), 1041–1051 CrossRef CAS PubMed.
- P. S. Low and S. A. Kularatne, Folate-targeted therapeutic and imaging agents for cancer, Curr. Opin. Chem. Biol., 2009, 13(3), 256–262 CrossRef CAS PubMed.
- Y. Yin, X. Wu and Z. Yang, et al., The potential efficacy of R8-modified paclitaxel-loaded liposomes on pulmonary arterial hypertension, Pharm. Res., 2013, 30, 2050–2062 CrossRef CAS PubMed.
- Li Peng and J.-C. Xu, Studies of Novel and Highly Efficient Peptide Coupling Reagents, J. Grad. Sch. Chin. Acad. Sci., 2004, 21(1), 127–134 Search PubMed.
- P. Li and J. C. Xu, The X-ray structures of HOBt-based immonium type coupling reagents and the rearrangement of benzotriazolyl esters of Na-protected amino acids or peptides: N-vs O-substituted forms, J. Chem. Soc., Perkin Trans. 2, 2001,(1), 113–120 RSC.
- M. Karas, D. Bachmann, U. Bahr and F. Hillenkamp, Matrix-assisted ultraviolet laser desorption of non-volatile compounds, Int. J. Mass Spectrom. Ion Processes, 1987, 78, 53–68 CrossRef CAS.
- X. Yin, S. Feng and Y. Chi, et al., Estrogen-functionalized liposomes grafted with glutathione-responsive sheddable chotooligosaccharides for the therapy of osteosarcoma, Drug Delivery, 2018, 25(1), 900–908 CrossRef CAS PubMed.
- Z. Guo, B. H. Lan and Y. Wen, et al., Dual targeting for metastatic breast cancer and tumor neovasculature by EphA2-mediated nanocarriers, Int. J. Pharm., 2015, 493(1–2), 380–389 CrossRef CAS PubMed.
- M. Sabzichi, J. Mohammadian and M. Ghorbani, et al., Fabrication of all-trans-retinoic acid-loaded biocompatible precirol: a strategy for escaping dose-dependent side effects of doxorubicin, Colloids Surf., B, 2017, 159, 620–628 CrossRef CAS PubMed.
- W. Wang, Study on the sensitivity of silencing egfr gene to mda mb 231 cell chemotherapeutics, Huazhong University of Science and Technology, 2011 Search PubMed.
Footnote |
† Guijuan Yue and Chengxiang Wang are joint first authors. |
|
This journal is © The Royal Society of Chemistry 2020 |