DOI:
10.1039/C9RA08983G
(Paper)
RSC Adv., 2020,
10, 3539-3543
Zinc-tetracarboxylate framework material with nano-cages and one-dimensional channels for excellent selective and effective adsorption of methyl blue dye†
Received
31st October 2019
, Accepted 14th January 2020
First published on 21st January 2020
Abstract
An example of a zinc-tetracarboxylate framework material (FJI-11) was solvothermally synthesized and structurally characterized. FJI-11 presented 3D cage-stacking frameworks with octahedral cages, cuboctahedral cages and two kinds of 1D channel along the c-axis. In addition, FJI-11 exhibited the excellent selective and effective adsorption of methyl blue (MB) dye by guest molecule exchange, and its adsorption process was in accordance with the second-order kinetic model and the Freundlich model.
Introduction
Organic dye wastewater comes from the cosmetic, textile, paper and pharmaceutical industries, and has become one of the most serious environmental pollutants due to being highly toxic and difficult to degrade; its release into the aquatic ecosystem can destroy the ecological environment.1,2 As one of the stable organic dye molecules, methyl blue (MB) is toxic and might cause the unwanted effects of nausea, abdominal pain, dizziness, headache, sweating and confusion.3 Currently, many methods have been employed for the treatment of MB wastewater, such as the degradation method, coagulation method, oxidation method, filtration method, adsorption method, radiation method and biological method, but each method has different advantages and disadvantages.1a,4 The adsorption method with the advantages of simplicity, convenience, high efficiency and obvious adsorption effect, has been considered as a very effective and proven technology for the removal of MB dye.5 Many adsorption materials including activated carbon, zeolites, molecular sieves and metal organic frameworks (MOFs) have been reported, while the search for and development of adsorption materials for the selective and effective adsorption of MB dye is of great significance and also a challenge.6
MOFs often have multifarious intriguing topologies and fascinating structures, which have the potential application as functional materials in the fields of fluorescence, magnetic, gas storage, catalysis, organic dye adsorption and so forth.7,8 Compared with the zeolite, molecular sieve and activated carbon, MOFs have the advantages of adjustable frame structure, high porosity, large specific surface area and simple synthesis process.9 To date, some MOFs have been used for the removal or degradation of MB dye, while the MOFs with the excellently selective and effective adsorption capacity have been rarely investigated.10,11 However, the design and construction of more MOFs with the excellently selective and effective adsorption of MB dye are desperately required.
Hence, we prepared an example of zinc-tetracarboxylate framework, formulated as [Zn2(bptc)(H2O)2]·5DMA (FJI-11, H4bptc = biphenyl-3,3′,5,5′-tetracarboxylic acid), which displayed the 3D cage-stacking frameworks with octahedral cages, cuboctahedral cages and two kinds of 1D channels along the c-axis. In addition, FJI-11 exhibited the excellently selective and effective adsorption of MB dye and its adsorption process was in accordance with the second-order kinetic model and Freundlich model.
Results and discussion
Synthesis and structure description
Cubic shaped crystals of FJI-11 are obtained by the solvothermal reaction of Zn(NO3)2·6H2O and H4bptc in the mixed-solvent of DMA and 1,4-dioxane (1
:
1, v/v) with the addition of 0.2 mL nitric acid (65 wt%) at 85 °C for 5 days. The phase purity of the as-synthesized product of FJI-11 was confirmed by the powder X-ray diffraction analysis (XRD). The final molecular formula of FJI-11 was calculated from the SQUEEZE results combined with the thermogravimetric analysis (TGA) data and elemental analysis (EA) data.
Single crystal X-ray diffraction analysis indicates that FJI-11 is crystallized in the trigonal space group R
m with the lattice parameters a = b = 18.7428(8) Å, c = 24.915(2) Å, α = β = 90° and γ = 120°, and its asymmetry unit consists of one half of independent Zn(II) ion, a quarter of bptc4− ligand and one half of terminally coordinated water molecule (Fig. S1†). The central Zn(II) ions are five-coordinated by four carboxylate O atoms from four different bptc4− ligands and one half O atom from the coordinated water. The bptc4− ligand displays a (κ1-κ1)-(κ1-κ1)-(κ1-κ1)-(κ1-κ1)-μ8 coordination mode and two Zn(II) ions are linked by four bridging carboxylate generate the Zn2(COO)4 paddle wheel SBUs (Fig. S2†). FJI-11 is analogue of MOF-505, MOF-505 series and NOTT-100 series, which consists of two different types of alternative packed open cages.12 Every twelve bptc4− ligands connects six Zn2(COO)4 paddle wheel SBUs, to generate a octahedral cage with the diameter of about 9 Å (Fig. 1a), then eight octahedral assembled into a cuboctahedral cage with the elliptical cavity is about 14 × 8 Å2 (Fig. 1b). Two different types of cages are connected to each other by sharing the triangular and quadrangular windows and arranging in an alternating fashion,12 forming a 3D cage-stacking framework with two kinds of 1D channels (the diameter of about 7.5 Å) along the c-axis (Fig. 1c and Fig. S3†).
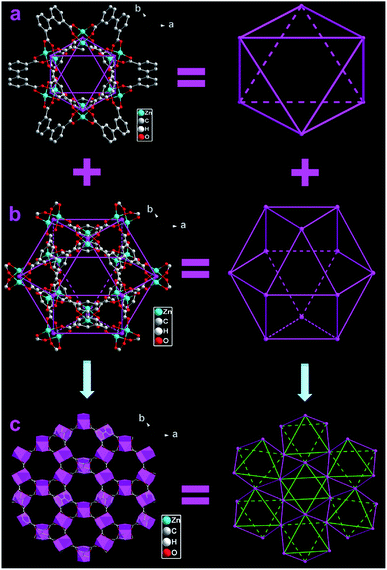 |
| Fig. 1 (a) The octahedral cage composed of Zn2(CO2)4 paddle wheel SBUs and bptc4− ligands. (b) The cuboctahedral cage constructed by eight octahedral cages. (c) The 3D cage-stacking framework in FJI-11. | |
Luminescence property
The solid state luminescence properties of FJI-11 and the free H4bptc ligand were explored at room temperature. The free H4bptc ligand exhibited an emission with the peaking at 372 nm (λex = 321 nm), while FJI-11 displayed a maximum emission at 439 nm (λex = 341 nm) (Fig. 2 and S6†). The maximum emission peak of FJI-11 exhibited the red shift phenomenon compared with that of the free H4bptc ligand. In addition, the luminescence mechanism of FJI-11 might be assigned to the π–π* fluorescent emissions of bptc4− ligand to Zn(II) charge transition (LMCT).13
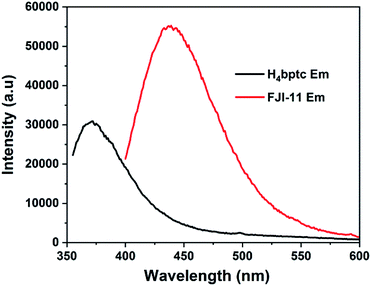 |
| Fig. 2 Solid state emission spectra of the free H4bptc ligand and FJI-11. | |
Sorption behaviour
The total volume of cavity in FJI-11 with the removal of the disordered guest solvent molecules and coordinated water molecules was calculated by the PLATON Program,14 which is approximately 61.5% (solvent area volume 4659.4 Å3 and unit cell volume 7579.90 Å3). In order to characterize the porosity of FJI-11, the crystal samples of FJI-11 were activated by the supercritical carbon dioxide (SCD) method and the N2 adsorption–desorption isotherms of activated FJI-11 sample was performed at 77 K. FJI-11 exhibited the pseudo-type I nitrogen isotherm with the saturated N2 capacity of 299.8 cm3 g−1 at 77 K (Fig. 3), and the corresponding to Brunauer–Emmett–Teller (BET) and Langmuir surface areas of 1121.01 m2 g−1 and 1283.88 m2 g−1.15 Meanwhile, the DFT pore size distribution (PSD) analysis reveals that the percentage of microspores centered at 6–8 Å and the maximum distribution centered approximately at 7.25 Å (Fig. 3), which accords well with the internal structural features of nano-cages and one-dimensional channels.
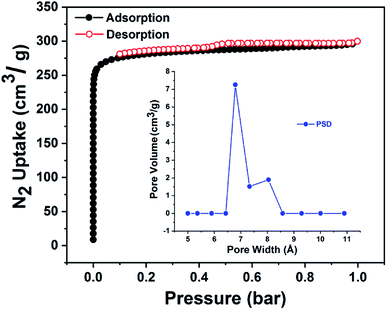 |
| Fig. 3 Experimental nitrogen adsorption–desorption isotherms at 77 K for FJI-11; inset shows the pore size distribution (PSD) calculated by the DFT method. | |
Adsorption capability of FJI-11 towards organic dye
In order to investigate the dye adsorption behaviors in FJI-11, the methylene blue (MB), methyl orange (MO), rhodamine B (RB) and rhodamine 6G (R6G) with different sizes and configurations were chosen for the adsorption experiments (Fig. S7†). The prepared crystalline sample (10 mg) of FJI-11 was immersed in the dye-containing acetonitrile solution (10 mg L−1), to evaluate its adsorption abilities by the guest molecule exchange, and the concentrations of the organic dyes before and after the adsorption experiments were menstruated by the UV/Vis spectrophotometer. As shown in Fig. 4, the color of the MB solution obviously changed from blue to the colorless, but the colors of the MO, RB and R6G solutions were no significant change, which were consistent with the UV-Vis spectroscopy results. These results indicated that FJI-11 could exhibit the excellently selective and effective adsorption of MB dye (Table S2†).16
 |
| Fig. 4 The adsorption experiments of (a) methylene blue (MB), (b) methyl orange (MO), (c) rhodamine B (RB) and (d) rhodamine 6G (R6G) in FJI-11. | |
In order to investigate the MB adsorption process in FJI-11, the kinetic adsorption and thermodynamics adsorption experiment were carried out. The kinetic adsorption experiment was investigated by the initial MB concentration of 10 mg L−1 under different concussion times, the experiment data was well fitted by the pseudo-second-order kinetic model17,18 (Fig. 5a). In addition, the thermodynamics adsorption experiment was investigated under different initial MB concentration at 25 °C, the experiment data was well fitted by the Freundlich model17,18 (Fig. 5b).
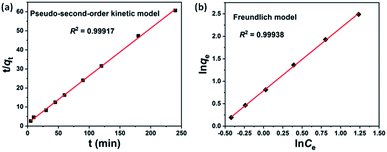 |
| Fig. 5 (a) Pseudo-second-order kinetic model of MB adsorption in FJI-11. (b) Freundlich model of MB adsorption in FJI-11. | |
These results suggest that the proposed adsorption mechanism of MB in FJI-11 was a chemical process, which occurred into the nano-cages and one-dimensional channels by the hydrogen bonding interaction and guest molecule exchange in FJI-11.18 Due to the cavity size effect (the maximum pore size distribution centered about at 7.25 Å in FJI-11, but the molecular sizes of RB and R6G were more than 7.25 Å) and the hydrogen bonding interaction (the N atom from the MB and the coordinated water molecules from FJI-11), FJI-11 only exhibited the excellently selective and effective adsorption of MB dye.17,18
Experimental
Materials and physical measurements
Reactions were carried out in 20 mL glass vials under autogenous pressure. All the reactants are of reagent-grade quality and used as purchased commercially without further purification. The power X-ray diffraction patterns (XRD) were collected by a Rigaku D using Cu Kα radiation (λ = 0.154 nm). Elemental analyses for C, H, N were carried out on a German Elementary Vario EL III instrument. Thermogravimetric analyses (TGA) were recorded on a NETZSCH STA 449C unit at a heating rate of 10 °C min−1 under flowing nitrogen atmosphere. Fluorescence spectra of the solid samples were performed on an Edinburgh Analytical instrument FLS920. Single gas adsorption measurements were performed in the Accelerated Surface Area and Porosimetry 2020 (ASAP2020). UV-Vis absorption spectra were recorded on a Purkinje General Instrument T6 new century.
Synthesis of [Zn2(bptc)(H2O)2]·5DMA (FJI-11)
A mixture of Zn(NO3)3·6H2O (0.40 mmol, 120 mg) and H4bptc (0.1 mmol, 33 mg), in N,N-dimethylacetamide (DMA) (3.0 mL) and 1,4-dioxane (3.0 mL) with an additional HNO3 (0.2 mL, 65 wt%) was sealed in a 20 mL glass vial, which was heated at 85 °C for 5 days, and cooled down to room temperature. After washing with fresh DMA, the crystals were obtained in ca. 58% yield based on the Zn(NO3)3·6H2O. Elemental analysis was calculated for FJI-11: C, 49.38%; H, 4.96%; N, 12.52%. Found: C, 49.12%; H, 4.93%; N, 12.55%.
Single-crystal X-ray crystallography
The structures data of FJI-11 was collected on a Rigaku Mercury CCD diffract meter equipped with a graphite-monochromated Mo Kα radiation (λ = 0.71073 Å) at room temperature and the structure was resolved by the direct method and refined by full-matrix least-squares fitting on F2 by Olex2 program.19 We employed the PLATON/SQUEEZE to calculate the contribution to the diffraction from the solvent region and thereby produced a set of solvent-free diffraction intensities.20 The final formula of FJI-11 was calculated from the SQUEEZE results combined with elemental analysis data and TGA data. More details on the crystallographic studies as well as atomic displacement parameters are given in ESI† as CIF files. Crystallographic data for the structures reported in this paper have been deposited. The following crystal structure has been deposited at the Cambridge Crystallographic Data Centre and allocated the deposition number CCDC: 1909611 for FJI-11. Crystallographic data and structure refinement parameters for FJI-11 are summarized in Table 1 and the selected bond lengths and angles of FJI-11 are listed in Table S1.†
Table 1 The crystal data and structure refinement for FJI-11
R = ∑(||Fo| − |Fc||)/∑|Fo|, wR = {∑w[(Fo2 − Fc2)2]/∑w[(Fo2)2]}1/2; [Fo > 4 (Fo)]. |
Compounds |
FJI-11 |
CCDC |
1909611 |
Formula |
C46H55N10O15Zn2 |
Mr |
1118.76 |
Space group |
R m |
a (Å) |
18.7428(8) |
b (Å) |
18.7428(8) |
c (Å) |
24.915(2) |
α (deg.) |
90 |
β (deg.) |
90 |
γ (deg.) |
120 |
V (Å3) |
7579.9(10) |
Z |
9 |
Dc (g cm−3) |
2.206 |
M (mm−1) |
1.481 |
F(000) |
3429.0 |
GOF |
1.062 |
R1a |
0.0352 |
wR2a |
0.0878 |
Conclusions
In summary, an example of zinc-tetracarboxylate framework material (FJI-11) has been successfully synthesized and structurally characterized. The 3D cage-stacking framework of FJI-11 was constructed by the octahedral cages, cuboctahedral cages and two kinds of 1D channel along the c-axis. FJI-11 exhibited the excellently selective and effective adsorption of MB dye by the guest molecule exchange, and its adsorption process was in accordance with second-order kinetic model and Freundlich model. Our results are helpful for the preparation of more porous MOFs with the excellently selective and effective adsorption of the organic dyes in the future.
Conflicts of interest
There are no conflicts to declare.
Acknowledgements
This work was supported by the National Natural Science Foundation of China (21861044 and 21561033), the Project funded by China Postdoctoral Science Foundation (2018M633426) and the Project funded by Yunnan Province Postdoctoral Science Foundation (2018).
Notes and references
-
(a) C. C. Wang, J. Li, X. L. Lv, Y. Q. Zhang and G. S. Guo, Energy Environ. Sci., 2014, 7, 2831–2867 RSC;
(b) Y. Shen, Q. Fang and B. Chen, Environ. Sci. Technol., 2015, 49, 67–84 CrossRef CAS PubMed;
(c) Q. Gao, J. Xu and X. H. Bu, Coord. Chem. Rev., 2019, 378, 17–31 CrossRef CAS.
-
(a) I. Ali, M. Asim and T. A. Khan, J. Environ. Manage., 2012, 113, 170–183 CrossRef CAS PubMed;
(b) G. Liu, X. Li, J. Zhao, H. Hidaka and N. Serpone, Environ. Sci. Technol., 2000, 34, 3982–3990 CrossRef CAS;
(c) C. C. Jin, D. M. Liu, J. Hu, Y. Wang, Q. Zhang, L. Lv and F. W. Zhuge, Nano Energy, 2019, 59, 372–379 CrossRef CAS.
-
(a) C. Jack and L. Jerrold B, Am. J. Therapeut., 2003, 10, 289–291 CrossRef PubMed;
(b) M. Rafatullah, O. Sulaiman, R. Hashima and A. Ahmad, J. Hazard. Mater., 2010, 177, 70–80 CrossRef CAS PubMed.
-
(a) M. B. Ahmed, J. L. Zhou, H. H. Ngo, W. S. Guo, N. S. Thomaidis and J. Xu, J. Hazard. Mater., 2017, 323, 274–298 CrossRef CAS PubMed;
(b) P. M. Pakdel and S. J. Peighambardoust, Carbohydr. Polym., 2018, 201, 264–279 CrossRef PubMed;
(c) Y. J. Yao, F. F. Xu, M. Chen, Z. X. Xu and Z. W. Zhu, Bioresour. Technol., 2010, 101, 3040–3046 CrossRef CAS PubMed;
(d) R. Wo, Q. L. Li, C. Zhu, Y. Zhang, G. F. Qiao, K. Y. Lei, P. Du and W. Jiang, J. Chem. Eng. Data, 2019, 64, 2455–2463 CrossRef.
-
(a) S. W. Lv, J. M. Liu, H. Ma, Z. H. Wang, C. Y. Li, N. Zhao and S. Wang, Microporous Mesoporous Mater., 2019, 282, 179–187 CrossRef CAS;
(b) Y. Ren, T. Li, W. M. Zhang, S. Wang, M. Q. Shi, C. Shan, W. B. Zhang, X. H. Guan, L. Lv, M. Hua and B. C. Pan, J. Hazard. Mater., 2019, 365, 312–321 CrossRef CAS PubMed.
-
(a) Y. Xu, J. Jin, X. Li, Y. Han, H. Meng, T. Wang and X. Zhang, RSC Adv., 2015, 5, 19199–19202 RSC;
(b) S. Lin, Z. Song, G. Che, A. Ren, P. Li, C. Liu and J. Zhang, Microporous Mesoporous Mater., 2014, 193, 27–34 CrossRef CAS;
(c) S. L. Xiao, Y. H. Li, P. J. Ma and G. H. Cui, Inorg. Chem. Commun., 2013, 37, 54–58 CrossRef CAS;
(d) R. Ahmad and R. Kumar, Appl. Surf. Sci., 2010, 257, 1628–1633 CrossRef CAS;
(e) M. Bhaumik, R. McCrindle and A. Maity, Chem. Eng. J., 2013, 228, 506–515 CrossRef CAS;
(f) W. Cai, Y. Hu, J. Chen, G. Zhang and T. Xia, CrystEngComm, 2012, 14, 972–977 RSC;
(g) W. Yan, L. J. Han, H. L. Jia, K. Shen, T. Wang and H. G. Zheng, Inorg. Chem., 2016, 55, 8816–8821 CrossRef CAS PubMed;
(h) X. Wang, Z. J. Ma, L. L. Chai, L. Q. Xu, Z. Y. Zhu, Y. Hu, J. J. Qian and S. M. Huang, Carbon, 2019, 141, 643–651 CrossRef CAS;
(i) X. Wang, L. L. Chai, J. Y. Ding, L. Zhong, Y. J. Du, T. T. Li, Y. Hu, J. J. Qian and S. M. Huang, Nano Energy, 2019, 62, 745–753 CrossRef CAS.
-
(a) G. Maurin, C. Serre, A. Cooper and G. Férey, Chem. Soc. Rev., 2017, 46, 3104–3107 RSC;
(b) N. S. Bobbitt, M. L. Mendonca, A. J. Howarth, T. Islamoglu, J. T. Hupp, O. K. Farha and R. Q. Snurr, Chem. Soc. Rev., 2017, 46, 3357–3385 RSC.
-
(a) C. H. Wang, X. L. Liu, N. K. Demir, J. P. Chen and K. Li, Chem. Soc. Rev., 2016, 45, 5107–5134 RSC;
(b) Y. Bai, Y. B. Dou, L. H. Xie, J. R. Li and H. C. Zhou, Chem. Soc. Rev., 2016, 45, 2327–2367 RSC.
-
(a) G. M. Espallargas and E. Coronado, Chem. Soc. Rev., 2018, 47, 533–557 RSC;
(b) M. O. Keeffe and O. M. Yaghi, Chem. Rev., 2012, 112, 675–702 CrossRef PubMed;
(c) M. Li, D. Li, M. O. Keeffe and O. M. Yaghi, Chem. Rev., 2014, 114, 1343–1370 CrossRef CAS PubMed.
-
(a) P. P. Yu, Q. P. Li, Y. Hu, N. N. Liu, L. J. Zhang, K. Z. Su, J. J. Qian, S. M. Huang and M. C. Hong, Chem. Commun., 2016, 52, 7978–7981 RSC;
(b) Y. Y. Cui, J. Zhang, L. L. Ren, A. L. Cheng and E. Q. Gao, Polyhedron, 2019, 161, 71–77 CrossRef CAS;
(c) C. Li, Z. h. Xiong, J. M. Zhang and C. S. Wu, J. Chem. Eng. Data, 2015, 60, 3414–3422 CrossRef CAS;
(d) J. H. Qiu, Y. Feng, X. F. Zhang, M. M. Jia and J. F. Yao, J. Colloid Interface Sci., 2017, 499, 151–158 CrossRef CAS PubMed;
(e) M. L. Gao, W. J. Wang, L. Liu, Z. B. Han, N. Wei, X. M. Cao and D. Q. Yuan, Inorg. Chem., 2017, 56, 511–517 CrossRef CAS PubMed.
-
(a) M. Z. Wu, J. Y. Shi, P. Y. Chen, L. Tian and J. Chen, Inorg. Chem., 2019, 58, 3130–3136 CrossRef CAS PubMed;
(b) X. J. Gao, G. H. Sun, F. Y. Ge and H. G. Zheng, Inorg. Chem., 2019, 58, 8396–8407 CrossRef CAS PubMed;
(c) Y. Li, F. T. Liu, H. X. Zhang, X. Li, X. F. Dong and C. W. Wang, Appl. Surf. Sci., 2019, 484, 144–151 CrossRef CAS.
-
(a) Y. Wang, H. F. Cao, B. S. Zheng, R. F. Zhou and J. G. Duan, Cryst. Growth Des., 2018, 18, 7674–7682 CrossRef CAS;
(b) Q. P. Li, J. G. Luo, L. Wang, C. Qi, Y. S. Yang, X. J. Zhang and J. J. Qian, CrystEngComm, 2017, 19, 214–217 RSC;
(c) B. L. Chen, N. W. Ockwig, A. R. Millward, D. S. Contreras and O. M. Yaghi, Angew. Chem., Int. Ed., 2005, 44(30), 4745–4749 CrossRef CAS PubMed;
(d) Y. X. Hu, S. C. Xiang, W. W. Zhang, Z. X. Zhang, L. Wang, J. F. Bai and B. L. Chen, Chem. Commun., 2009, 48, 7551–7553 RSC;
(e) Y. Yan, S. H. Yang, A. J. Blake and M. Schroder, Acc. Chem. Res., 2014, 47(2), 296–307 CrossRef CAS PubMed.
-
(a) W. P. Lustig, S. Mukherjee, N. D. Rudd, A. V. Desai, J. Li and S. K. Ghosh, Chem. Soc. Rev., 2017, 46, 3242–3285 RSC;
(b) Q. P. Li, Z. Y. Zhu and J. J. Qian, Polyhedron, 2018, 155, 218–222 CrossRef CAS;
(c) Q. P. Li and J. J. Qian, RSC Adv., 2014, 4, 32391–32397 RSC;
(d) L. Chen, C. Yan, M. Pan, H. P. Wang, Y. N. Fan and C. Y. Su, Eur. J. Inorg. Chem., 2016, 17, 2676–2680 CrossRef.
-
(a) A. L. Spek, PLATON, A Multipurpose Crystallographic Tool, Utrecht University, 2001 Search PubMed;
(b) J. G. Duan, Z. Yang, J. F. Bai, B. S. Zheng, Y. Z. Li and S. H. Li, Chem. Commun., 2012, 48, 3058–3060 RSC.
-
(a) Y. J. Du, L. Zhong, Y. Hu, Q. P. Li and J. J. Qian, CrystEngComm, 2019, 21, 5045–5049 RSC;
(b) J. J. Qian, Q. P. Li, L. F. Liang, T. T. Li, Y. Hu and S. M. Huang, Dalton Trans., 2017, 46, 14102–14106 RSC;
(c) S. H. Yang, J. L. Sun, A. J. Ramirez-Cuesta, S. K. Callear, W. I. F. David, D. P. Anderson, R. New by, A. J. Blake, J. E. Parker, C. C. Tang and M. Schröder, Nat. Chem., 2012, 4, 887–894 CrossRef CAS PubMed;
(d) M. Savage, I. Silva, M. Johnson, J. H. Carter, R. Newby, M. Suyetin, E. Besley, P. Manuel, S. Rudić, A. N. Fitch, C. Murray, W. I. F. David, S. H. Yang and M. Schröder, J. Am. Chem. Soc., 2016, 138, 9119–9127 CrossRef CAS PubMed.
-
(a) M. Hasanzadeh, A. Simchi and H. S. Far, J. Ind. Eng. Chem., 2020, 81, 405–414 CrossRef;
(b) M. Hasanzadeh, A. Simchi and H. S. Far, Mater. Chem. Phys., 2019, 233, 267–275 CrossRef CAS;
(c) M. Pęgier, K. Kilian and K. Pyrzynska, Monatshefte Chem., 2019, 150, 1569–1572 CrossRef;
(d) L. Zhang, J. S. Sun, F. X. Sun, P. Chen, J. Liu and G. S. Zhu, Chem.–Eur. J., 2019, 25, 3903–3908 CrossRef CAS PubMed.
-
(a) P. Huang, C. Chen, M. Wu, F. Jiang and M. Hong, Dalton Trans., 2019, 48, 5527–5533 RSC;
(b) M. Mon, R. Bruno, J. F. Soria, D. Armentano and E. Pardo, J. Mater. Chem. A, 2018, 6, 4912–4947 RSC;
(c) F. L. Hu, Z. Y. Di, P. Lin, P. Huang, M. Y. Wu, F. L. Jiang and M. C. Hong, Cryst. Growth Des., 2018, 18, 576–580 CrossRef CAS;
(d) K. Z. Su, W. J. Wang, B. B. Li and D. Q. Yuan, ACS Sustainable Chem. Eng., 2018, 6, 17402–17409 CrossRef CAS;
(e) M. Zhou, Z. F. Ju and D. Q. Yuan, Chem. Commun., 2018, 54, 2998–3001 RSC.
-
(a) L. N. Jin, X. Y. Qian, J. G. Wang, H. Aslan and M. Dong, J. Colloid Interface Sci., 2015, 453, 270–275 CrossRef CAS PubMed;
(b) J. M. Yang, R. J. Ying, C. X. Han, Q. T. Hu, H. M. Xu, J. H. Li, Q. Wang and W. Zhang, Dalton Trans., 2018, 47, 3913–3920 RSC;
(c) S. Lin, Z. Song, G. Che, A. Ren, P. Li, C. Liu and J. Zhang, Microporous Mesoporous Mater., 2014, 193, 27–34 CrossRef CAS;
(d) L. Xiao, Y. Xiong, Z. Wen and S. Tian, RSC Adv., 2015, 5, 61593–61600 RSC;
(e) P. Z. Li, X. J. Wang, S. Tan, C. Ang, H. Chen, J. Liu, R. Zou and Y. Zhao, Angew. Chem., Int. Ed., 2015, 54, 12748–12752 CrossRef CAS PubMed;
(f) J. Ai, H. R. Tian, X. Min, Z. C. Wang and Z. M. Sun, Dalton Trans., 2020 10.1039/c9dt01545k.
-
(a) G. M. Sheldrick, SHELXS, Acta Crystallogr., 2008, A64, 112–122 CrossRef PubMed;
(b) M. Sheldrick, Acta Crystallogr., 2015, C71, 3–8 Search PubMed.
-
(a) A. L. Spek, J. Appl. Crystallogr., 2003, 36, 7 CrossRef CAS;
(b) P. v. d. Sluis and A. L. Spek, Acta Crystallogr., Sect. A: Found. Crystallogr., 1990, 46, 194 CrossRef;
(c) A. L. Spek, Acta Crystallogr., 2015, C71, 9 Search PubMed;
(d) Q. P. Li, Z. Y. Zhu and J. J. Qian, Polyhedron, 2018, 155, 218–222 CrossRef CAS;
(e) Q. P. Li, Y. Peng, J. J. Qian, T. Yan, L. Du and Q. H. Zhao, Dalton Trans., 2019, 48, 12880–12887 RSC.
Footnote |
† Electronic supplementary information (ESI) available: Crystal data, TGA, PXRD patterns, UV data, luminescent spectra and other materials for FJI-11. CCDC 1909611. For ESI and crystallographic data in CIF or other electronic format see DOI: 10.1039/c9ra08983g |
|
This journal is © The Royal Society of Chemistry 2020 |