DOI:
10.1039/C9RA08551C
(Paper)
RSC Adv., 2020,
10, 2887-2896
Synthesis and study of C-substituted methylthio derivatives of cobalt bis(dicarbollide)†
Received
18th October 2019
, Accepted 23rd December 2019
First published on 16th January 2020
Abstract
The C-methylthio derivatives of cobalt bis(dicarbollide) were synthesized by reaction of anhydrous CoCl2 with nido-carborane [7-MeS-7,8-C2B9H11]− and isolated as a mixture of rac-[1,1′-(MeS)2-3,3′-Co(1,2-C2B9H10)2]− and meso-[1,2′-(MeS)2-3,3′-Co(1,2-C2B9H10)2]− isomers. The structures of both isomers were studied using DFT quantum chemical calculations. The most preferable geometry of rotamers and the stabilization energy of C-methylthio derivatives of cobalt bis(dicarbolide) were calculated. The (BEDT-TTF)[1,1′-(MeS)2-3,3′-Co(1,2-C2B9H10)2] salt was prepared and its structure was determined by single crystal X-ray diffraction. The cisoid conformation of the rac-[1,1′-(MeS)2-3,3′-Co(1,2-C2B9H10)2]− anion is stabilized by short intramolecular CH⋯S hydrogen and BH⋯S chalcogen bonds between the dicarbollide ligands, that is in good agreement with the data of quantum chemical calculations.
Introduction
The synthesis of the first carboranes in the early 1960s, followed by the rapid development of their chemistry, resulted in the creation of a number of new carborane-based materials with unique properties.1–5 Among the various derivatives of carboranes, special attention is paid to π-complexes of transition metals with carborane ligands, called metallacarboranes. The most known of them, cobalt bis(dicarbollide) [3,3′-Co(1,2-C2B9H11)2]− attracts increasing interest from researchers working in various fields from medical chemistry to6–11 materials science12–18 to due to its extraordinary high stability, low toxicity19 and almost unlimited possibilities for chemical modification.20,21
Recently, we suggested that cobalt bis(dicarbollide) can be used as a structural element in the design of molecular switches.22 Molecular switches are molecules or supramolecular complexes that can exist in two or more stable forms that differ in the mutual orientation of their components and can be converted readily from one state to another by various external stimuli such as heat, light, chemicals, etc.23 Indeed, when studying a series of isomeric B-methylthio derivatives of cobalt bis(dicarbollide), we found that in the 8,8′-isomer [8,8′-(MeS)2-3,3′-Co(1,2-C2B9H10)2]− stabilization of the transoid conformation is reached due to two pairs of intramolecular CHcarb⋯S hydrogen bonds between the ligands, whereas in the 4,4′-isomer [4,4′-(MeS)2-3,3′-Co(1,2-C2B9H10)2]− two pairs of the CHcarb⋯S hydrogen bonds stabilize the gauche conformation. In the case of the 4,7′-isomer [4,7′-(MeS)2-3,3′-Co(1,2-C2B9H10)2]−, due to steric reasons the gauche conformation is stabilized by only one pair of the CHcarb⋯S hydrogen bonds.24 The addition of an external metal of labile metallacomplex to the 8,8′-isomer leads to the breaking weak hydrogen bonds and the appearance of stronger dative S→M bonds that accompanies by a change in conformation from transoid to cisoid one.25 In this contribution we report the synthesis and study of C-methylthio derivatives of cobalt bis(dicarbollide).
Results and discussion
Synthesis of C-substituted methylthio derivatives of cobalt bis(dicarbollide)
To synthesize the C-methylthio derivatives of cobalt bis(dicarbollide) we used reaction of anhydrous CoCl2 with 7-methylthio-nido-carborane [7-MeS-7,8-C2B9H11]− (ref. 26) in 1,2-dimethoxyethane in the presence of potassium tert-butoxide (Scheme 1). The reaction results in a mixture of rac-[1,1′-(MeS)2-3,3′-Co(1,2-C2B9H10)2]− and meso-[1,2′-(MeS)2-3,3′-Co(1,2-C2B9H10)2]− isomers in 1
:
1 molar ratio; all attempts to separate them by chromatographic methods have been unsuccessful. The C-methylthio derivatives of paramagnetic iron bis(dicarbollide) were prepared in a similar way (See ESI†). The formation of an equimolar mixture of the rac- and meso-isomers in the reactions with asymmetrically substituted nido-carboranes is quite expected and was observed earlier for [9-MeS-7,8-C2B9H11]− (isomers were separated),24 [7-EtS-7,8-C2B9H10]− and [7-EtS-8-R-7,8-C2B9H10]− (R = Me, Ph) (isomers were not separated).27,28 However, in some cases, e.g. in a similar reaction with [9-MeO-7,8-C2B9H11]− only one isomer is formed,29 that could be explained by lower stability of second isomer under synthesis and/or isolation conditions. It should be noted that all our attempts to synthesize cobalt and iron bis(dicarbollide) complexes based on 7,8-di(methylthio)-nido-carborane [7,8-(MeS)2-7,8-C2B9H10]− failed.
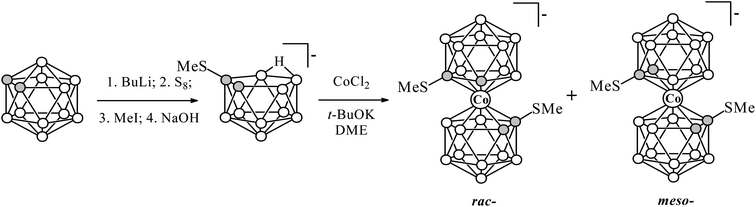 |
| Scheme 1 Synthesis of C-substituted methylthio derivatives of cobalt bis(dicarbollide). | |
The 1H NMR spectrum of the reaction product contains characteristic signals of the carborane CH groups at 4.33, 4.29 and 4.00 ppm with an integral intensity ratio of 1
:
1
:
2, as well as two signals of the MeS groups at 2.40 (narrow) and 2.28 (wide) ppm with an integral intensity ratio of 3
:
3 (Fig. 1). The appearance of three signals of the carborane CH groups indicates a confined mutual rotation of dicarbollide ligands. Moreover, it is reasonable to assume that one of the isomers has two equivalent carborane CH groups, whereas in another one these groups are nonequivalent. This nonequivalence can be caused by the participation of the CH groups in the formation of intramolecular CHcarb⋯S hydrogen bonds between the ligands similar to found in the B-substituted methylthio derivatives.24 In this case, in the rac-isomer [1,1′-(MeS)2-3,3′-Co(1,2-C2B9H10)2]− both CH groups can form intramolecular CHcarb⋯S hydrogen bonds, while in the meso-isomer [1,2′-(MeS)2-3,3′-Co(1,2-C2B9H10)2]− only one CH group can participate in such bonding for steric reasons. To verify this assumption, quantum chemical calculations were performed.
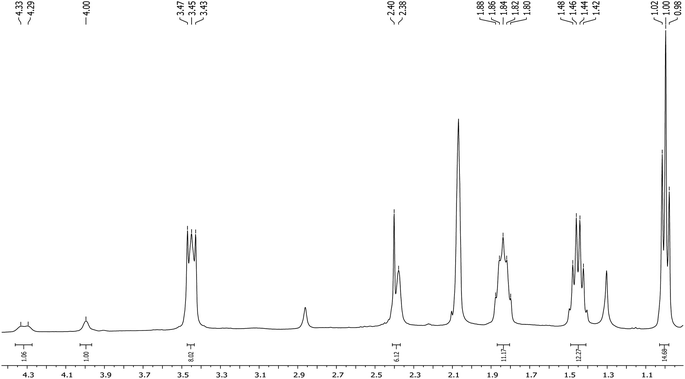 |
| Fig. 1 1H NMR spectrum of (Bu4N)[1,1′(2′)-(MeS)2-3,3′-Co(1,2-C2B9H10)2] in acetone-d6. | |
Quantum-chemical calculations of [X,Y′-(MeS)2-3,3′-Co(1,2-C2B9H10)2]− rotamers
Both for rac-[1,1′-(MeS)2-3,3′-Co(1,2-C2B9H10)2]− and meso-[1,2′-(MeS)2-3,3′-Co(1,2-C2B9H10)2]− isomers five rotamers were identified as minima on the PES, totally 10 isomers (Fig. 2, Table S1†). Due to symmetry of 1,2′-substituted cobalt bis(dicarbollide) (meso-isomer), the two possible cisoid- and gauche- rotamers are pairwise identical enantiomers. Therefore three conformers (transoid-, gauche- and cisoid-) will be discussed for meso-[1,2′-(MeS)2-3,3′-Co(1,2-C2B9H10)2]− and five (single trans-, two gauche- and two cisoid-) for rac-[1,1′-(MeS)2-3,3′-Co(1,2-C2B9H10)2]− (Fig. 3) hereinafter.
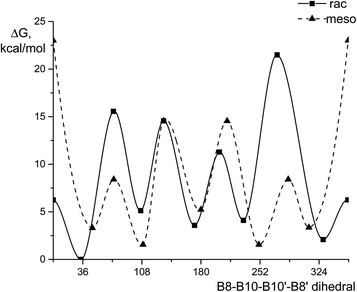 |
| Fig. 2 The rotational profile of rac-[1,1′-(MeS)2-3,3′-Co(1,2-C2B9H10)2]− and meso-[1,2′-(MeS)2-3,3′-Co(1,2-C2B9H10)2]− isomers. ΔG298 values are related to the global minimum of cisoid conformation of the rac-isomer. | |
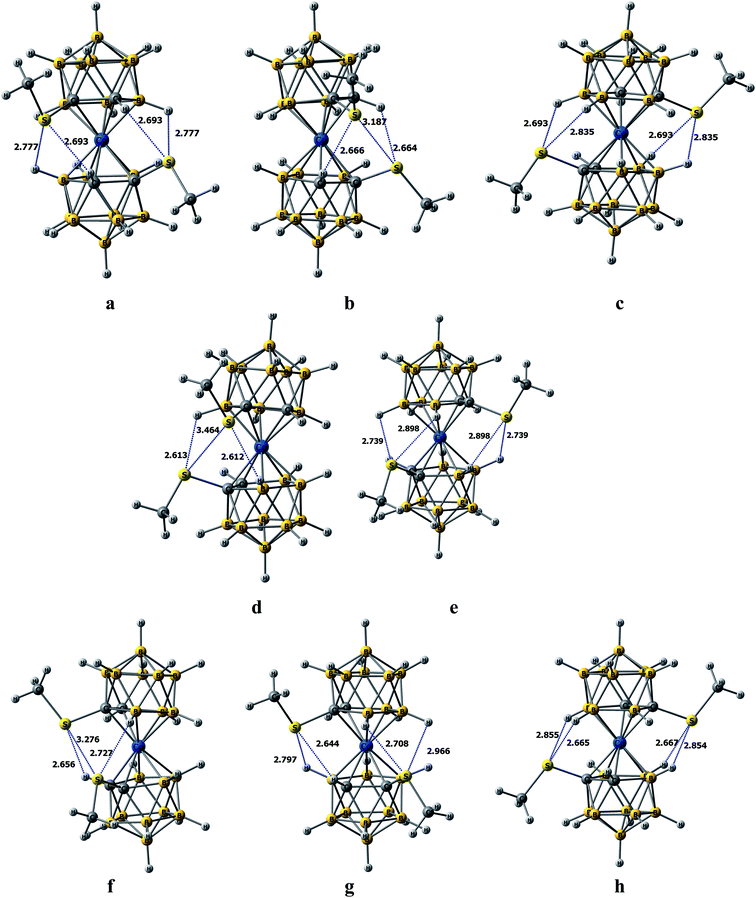 |
| Fig. 3 Structures of rotamers for rac-[1,1′-(MeS)2-3,3′-Co(1,2-C2B9H10)2]− (a – cisoid1, b – cisoid2, c – gauche1, d – gauche2, e – transoid) and meso-[1,2′-(MeS)2-3,3′-Co(1,2-C2B9H10)2]− (f – cisoid, g – gauche, h – transoid) isomers. | |
Despite geometry optimizations were performed without symmetry restrictions the all five rotamers of rac-isomer are close to the C2 symmetry, while only transoid-rotamer of the meso- has a symmetry point group (Ci). For the rac-isomer, the global minimum among all possible rotamers corresponds to the cisoid1 conformation stabilized by two CH⋯S and two BH⋯S interactions between the dicarbollide ligands (Fig. 3). It is noteworthy that this global minimum corresponds exactly to the rotamer that was found in the X-ray structure of the BEDT-TTF salt (see below, Table S2†). The same conformation was found earlier in the X-ray structure of {(Me2CO)2Na[rac-1,1′-μ-{S(CH2CH2O)3CH2CH2S}-3,3′-Co-(1,2-C2B9H10)2]}.30 The second most preferred rotamer is a cisoid2-, which is 2.1 kcal mol−1 higher, while gauche1-, gauche2- and transoid-rotamers are 5.1, 3.6 and 4.1 kcal mol−1 higher, than the cisoid1-rotamer on the ΔG298 scale. The cisoid2- and gauche2- rotamers features S⋯S interaction and as the consequence the rotational barrier between them requiring the movement of sulfur atoms close to each other is the highest on the rotational PES. Additionally, the cisoid2-rotamer is stabilized by two CH⋯S interactions, while the gauche2-rotamer has two BH⋯S interactions. In a turn, the transoid- and gauche2-rotamers possess four BH⋯S bond critical points (BCP's) each. Energy of these interactions with S atom EBCP are in -(1.6–2.3) kcal mol−1 range with their sum being 6.2–7.9 kcal mol−1.
The most favorable for the meso-[1,2′-(MeS)2-3,3′-Co(1,2-C2B9H10)2]− isomer gauche-rotamer is stabilized by three BH⋯S and one CH⋯S interactions (EBCP are in −(1.4–2.3) kcal mol−1 range, with their sum being 7.5 kcal mol−1). The corresponding cisoid conformer is stabilized by one BH⋯S and one CH⋯S interactions as well as by S⋯S interaction and is only 1.7 kcal mol−1 less preferable, than the gauche-rotamer. The barrier between two equivalent cisoid-rotamers featuring S⋯S interactions is highest on the rotational PES. It is worth noting that namely the cisoid-rotamer was found earlier in the X-ray structure of {(Me2CO)Na[meso-1,2′-μ-{S(CH2CH2O)3CH2CH2S}-3,3′-Co-(1,2-C2B9H10)2]}·2CHCl3, where mutual rotation of the dicarbollide ligands is additionally restricted by the complexation of the sodium cation with the oligoethyleneglycol bridge connecting both dicarbollide ligands.30 The transoid-rotamer slightly is stabilized by four BH⋯S interactions but is the least favorable (ΔG298 = 3.6 kcal mol−1).
It should be noted that the CH groups in the cisoid-conformation of the rac-isomer are equivalent, bonded to the S(Me) group of the second dicarbollide ligand (r(CH⋯S) = 2.693 Å, EBCP = 2.1 kcal mol−1), whereas the guache-conformation of the meso-isomer has non-equivalent CH groups, one being bonded with S atom (2.797 Å, EBCP = 1.7 kcal mol−1), while second in not connected by the bond path to any neighbor. This rationalizes the 1H NMR spectrum where two resonances of CH groups were observed.
The DFT analysis of PES of mutual rotation of the dicarbollide ligands predicts free rotation at room temperature (the second highest barrier is ΔG# = 15.6 and 13.0 kcal mol−1 for rac- and meso-isomers, respectively). The highest rotational transition states on the PES are by 21.5 kcal mol−1 higher than the lowest energy rotamer, but their passing is not required for formation of every possible isomer. The second favorable rotamer located 2.1 kcal mol−1 above the most favored one for the rac-isomer and 1.7 kcal mol−1 for the meso-isomer, suggesting their possible presence in solution at amounts <5%. Therefore observation of only one – the most favorable rotamer for each isomer is well rationalized by the DFT calculations.
Synthesis, structure and properties of (BEDT-TTF)[1,1′-(MeS)2-3,3′-Co(1,2-C2B9H10)2]
In a systematic study of the tetrathiafulvalene salts of transition metal bis(dicarbollides), we found that various substituents in the dicarbollide ligands have a great influence on their crystal structure and electroconductive properties.13,31 In addition to the stabilization of certain rotamers of bis(dicarbollide) complexes due to intramolecular hydrogen bonding, exo-polyhedral substituents also can participate in intermolecular bonding with other anions and the tetrathiafulvalene radical-cations.32–36 This prompted us to synthesize similar compounds with methylthio derivatives of cobalt bis(dicarbollide). It should be noted that our initial attempt to obtain the BEDT-TTF salt with the with [8,8′-(MeS)2-3,3′-Co(1,2-C2B9H10)2]− anion was unsuccessful due to the ease of oxidation of sulfur atoms,24 which, probably, can be explained by the strong electron-donating effect of the cobaltacarborane cage bound via boron atom. On the other hand, in the case of the MeS group attached to the carbon atom, the electron-withdrawing nature of the cobaltacarborane cage should result in an increase of the oxidative stability of the substituent.37
Indeed, we were able to obtain single crystals of the salt of the 1
:
1 radical-cation salt (BEDT-TTF)[1,1′-(MeS)2-3,3′-Co(1,2-C2B9H10)2] electrochemically by anodic oxidation of BEDT-TTF in the presence of (Me4N)[1,1′(2′)-(MeS)2-3,3′-Co(1,2-C2B9H10)2] in a mixture of 1,1,2-trichloroethane–acetonitrile (12
:
1) as a solvent. It is noteworthy that the electrocrystallization leads to selective isolation of only one isomer, namely the rac-isomer. The crystal structure is formed by the BEDT-TTF radical-cation in a general position and enantiomeric anions [1,1′-(MeS)2-3,3′-Co(1,2-C2B9H10)2]− (A) and [2,2′-(MeS)2-3,3′-Co(1,2-C2B9H10)2]− (B) in special positions on a two-fold axis of the unit cell (Fig. 4). The dicarbollide ligands in the anions are mutually rotated by 33.2 and 35.1°, for A and B respectively, adopting cisoid conformation. The cisoid conformation is stabilized by short intramolecular C(2)H⋯S(1) hydrogen (2.561 and 2.603 Å for A and B, respectively) and B(7)H⋯S(1) chalcogen (2.843 and 2.837 Å for A and B, respectively) bonds, that is in good agreement with the data of quantum chemical calculations. Similar CH⋯S (2.513 and 2.579 Å) and BH⋯S (2.901 and 3.009 Å) bonds were found earlier in X-ray structure of {(Me2CO)2Na[rac-1,1′-μ-{S(CH2CH2O)3CH2CH2S}-3,3′-Co-(1,2-C2B9H10)2]}, however in that case mutual ligand rotation is additionally restricted by the complexation of the sodium cation with the oligoethyleneglycol bridge connecting both dicarbollide ligands.30 It should be noted that the cobalt bis(dicarbollide) anions are characterized by a rather distorted geometry: the C2B3 planes of the dicarbollide ligands form a dihedral angle of 170.8° for anion A and 170.5° for anion B.
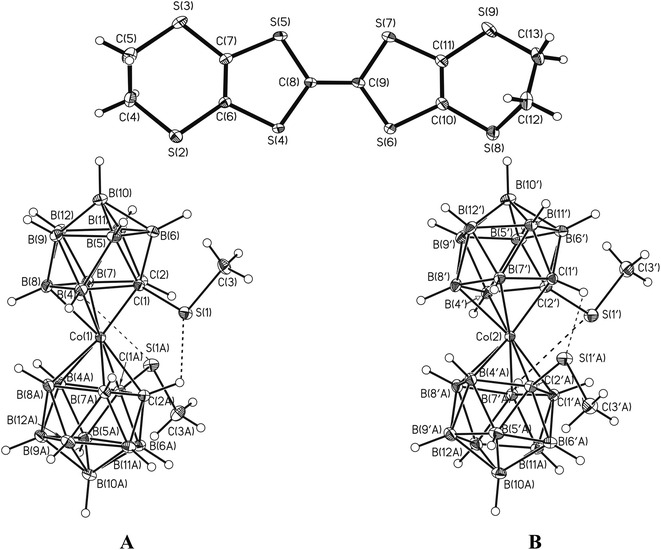 |
| Fig. 4 BEDT-TTF radical-cations and [1,1′-(MeS)2-3,3′-Co(1,2-C2B9H10)2]− (A) and [2,2′-(MeS)2-3,3′-Co(1,2-C2B9H10)2]− (B) anions. Dashed lines show intramolecular CH⋯S and BH⋯S contacts. | |
The formation of chalcogen bonds is generally explained in terms of the positive electrostatic potential present on the outermost portion of the chalcogen's surface, so-called “σ-hole”. This “σ-hole” can interact attractively with negative sites of the same molecule or other molecules.38,39 The acceptors of the chalcogen bond usually are atoms having an unshared electron pair, π-systems or halide anions. To the best of our knowledge, there are only a few examples of the formation of chalcogen bonds with boron hydrides as acceptors and all them were found in the MeS derivatives of transition metal bis(dicarbollide) complexes.24,40,41
In the crystal structure, the pairs of A and B enantiomers alternate with the BEDT-TTF radical-cation dimers. In the dimers, the radical-cations are bound by shortened S⋯S intermolecular contacts in the range 3.378–3.380 Å (the sum of the van der Waals radii S⋯S is 3.60 Å).42 Slightly shortened S⋯S intermolecular contacts of the “side-by-side type” with a length of 3.628 Å were found between the dimers, thus forming a pseudo-layered architecture (Fig. 5). The intermolecular S(1)⋯S(5) contacts with a length of 3.633 (1) Å (which insignificantly exceed the sum of the van der Waals radii) were also found between the cationic and anionic layers. In the dimer (Fig. 6) formed by the BEDT-TTF donors, the distances between the averaged donor planes drawn through all sulfur atoms are 3.55 Å; the dihedral angle formed by the donor planes in the dimer is 0° by symmetry conditions. The donor molecules are non-planar and have a semi-chair conformation with the maximum deviation of sulfur atoms from the molecule plane of 0.14 Å (S(4) and S(5) atoms) and the maximum deviation of carbon atoms from this plane of 1.54 Å (C(12) atom).
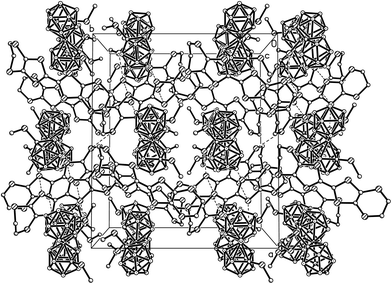 |
| Fig. 5 Crystal packing in the structure of (BEDT-TTF)[1,1′-(MeS)2-3,3′-Co(1,2-C2B9H10)2] (hydrogen atoms are omitted for clarity). | |
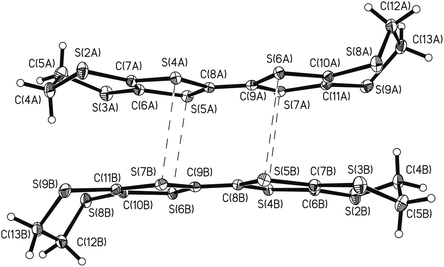 |
| Fig. 6 Dimer formed by the BEDT-TTF radical-cations in the structure of (BEDT-TTF)[1,1′-(MeS)2-3,3′-Co(1,2-C2B9H10)2]. Dashed lines show intermolecular S⋯S contacts. | |
The measurement of electric resistivity of polycrystalline sample of (BEDT-TTF)[1,1′-(MeS)2-3,3′-Co(1,2-C2B9H10)2] (Fig. 7) demonstrated that this salt is a semiconductor with the electrical conductivity at room temperature σ293 ∼ 6 × 10−7 ohm−1 cm−1 and an activation energy of about 0.43 eV. The low electrical conductivity is due to both the stoichiometry of the simple salt and the irregularity of the BEDT-TTF layers and the strong dimerization of radical-cations in the stack.
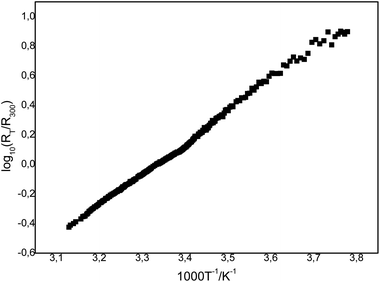 |
| Fig. 7 Temperature dependence of resistivity in polycrystalline sample of (BEDT-TTF)[1,1′-(MeS)2-3,3′-Co(1,2-C2B9H10)2]. | |
Conclusions
The C-methylthio derivatives of cobalt bis(dicarbollide) as mixture of rac-[1,1′-(MeS)2-3,3′-Co(1,2-C2B9H10)2]− and meso-[1,2′-(MeS)2-3,3′-Co(1,2-C2B9H10)2]− isomers were synthesized by reaction of anhydrous CoCl2 with nido-carborane [7-MeS-7,8-C2B9H11]− in the presence of potassium tert-butoxide. The structures of both isomers were studied using DFT quantum chemical calculations. For the rac-isomer, the optimized geometry corresponds to cisoid-rotamer stabilized by two intramolecular CHcarb⋯S(Me) hydrogen and two BH⋯S(Me) chalcogen bonds between the dicarbollide ligands, whereas for the meso-isomer the most preferable was found to be gauche-rotamer that is stabilized by stabilized by one CHcarb⋯S(Me) and three BH⋯S(Me) intramolecular bonds. It is noteworthy that the stabilization energy of in the C-methylthio derivatives of cobalt bis(dicarbolide) is significantly less than in the B-methylthio derivatives stabilized by exclusively CHcarb⋯S(Me) intramolecular bonds. Nevertheless, it was found that the geometry of the cisoid-rotamer is effectively realized in the X-ray structure of the (BEDT-TTF)[1,1′-(MeS)2-3,3′-Co(1,2-C2B9H10)2] salt which demonstrates semiconductor properties.
Experimental
Materials, instruments and general procedures
The cesium salt of 7-methylthio-nido-carborane was prepared according to the literature procedure.26 Anhydrous CoCl2 was prepared by dehydration of CoCl2·6H2O using the standard procedure.43 Tetrahydrofuran and 1,2-dimethoxyethane were dried by distillation over metallic sodium in the presence of benzophenone.44 Other reagents were purchased from commercial suppliers and used without further purification. Thin-layer chromatograms (DC Kieselgel F254 silicagel on aluminium plates, Merck) were visualized using 0.1% PdCl2 in 3 M HCl(aq). Acros Organics silica gel (0.060–0.200 mm) was used for column chromatography. The 1H, 11B, and 13C NMR spectra were collected using Bruker Avance-400 spectrometer. The residual signal of the NMR solvent relative to tetramethylsilane was taken as the internal reference for 1H NMR and 13C NMR spectra. 11B NMR spectrum was referenced using a BF3·Et2O external standard. High-resolution mass spectrum was obtained on a Bruker Daltonics microOTOF II mass spectrometer.
Synthesis of (Bu4N)[1,1′(2′)-(MeS)2-3,3′-Co(1,2-C2B9H10)2] and (Me4N)[1,1′(2′)-(MeS)2-3,3′-Co(1,2-C2B9H10)2]
The reaction was carried out under argon atmosphere. To a solution of cesium salt of 7-methylthio-nido-carborane (0.60 g, 1.92 mmol) in dry 1,2-dimethoxyethane (50 ml), anhydrous CoCl2 (2.49 g, 19.20 mmol) and potassium tert-butoxide (2.15 g, 19.20 mmol) were added. The mixture immediately warmed up and acquired dark green color. The reaction was stirred for 15 min at room temperature and heated under reflux for 30 h. The reaction mixture was allowed to cool to ambient temperature and filtered, the filtrate was evaporated to dryness in vacuo. The residue was treated with diethyl ether (50 ml) and water (50 ml). The organic layer was separated, washed with water (2 × 30 ml), dried over Na2SO4 and evaporated in vacuo. The desired product was obtained by the precipitation from water by water solution of tetramethyl- or tetrabutylammonium bromide as dark orange solid (65–80% yield). The spectral data for the tetrabutylammonium salt: 1H NMR (acetone-d6, ppm): 4.33 (1H, s, CHcarb), 4.29 (1H, s, CHcarb), 4.00 (2H, s, CHcarb), 3.45 (8H, Bu4N+), 2.40 (3H, s, SCH3), 2.28 (3H, s, SCH3), 1.84 (8H, Bu4N+), 1.45 (8H, Bu4N+), 1.00 (8H, Bu4N+), 2.7–0.8 (20H, m, BH). 13C NMR (acetone-d6, ppm): 67.4 (CHcarb), 63.6 (CSCH3), 58.4 (Bu4N+), 23.4 (Bu4N+), 20.4 (CSCH3), 19.4 (Bu4N+), 12.9 (Bu4N+). ESI HRMS for [C6H26B18CoS2]−: calcd m/z 416.2613, obsd m/z 416.2603.
Synthesis of K[1,1′-(2′)-(MeS)2-3,3′-Fe(1,2-C2B9H10)2]
To a solution of cesium salt of 7-methylthio-nido-carborane (0.45 g, 1.44 mmol) in dry THF (30 ml), anhydrous FeCl2 (0.55 g, 4.30 mmol) and potassium tert-butoxide (0.81 g, 7.20 mmol) were added and heated under reflux for 2 days under argon atmosphere. The reaction mixture was allowed to cool to room temperature, evaporated to dryness in vacuo and treated with diethyl ether (50 ml) and water (50 ml). The organic layer was separated, evaporated to dryness in vacuo and treated with toluene (4 × 50 ml) and saturated aq. solution of K2CO3 (50 ml). The organic fraction was combined and evaporated in vacuo to obtain 0.15 g (47%) of red solid. 11B NMR (acetone-d6, ppm): 104.2, 29.4, 9.9, −4.2, −11.5, −31.8, −36.1, −384.2, −556.5.
Synthesis of (BEDT-TTF)[1,1′-(MeS)2-3,3′-Co(1,2-C2B9H10)2]
Crystals of the radical-cation salt (BEDT-TTF)[1,1′-(MeS)2-3,3′-Co(1,2-C2B9H10)2] were obtained by anodic oxidation of BEDT-TTF (2 × 10−3 M) in the presence of (Me4N)[1,1′(2′)-(MeS)2-3,3′-Co(1,2-C2B9H10)2] (8 × 10−3 M) in 1,1,2-trilchloroethane–acetonitrile (12
:
1) mixture as a solvent under argon atmosphere in standard two-electrode H-cell with platinum electrodes separated by glass frit under galvanostatic conditions. The current applied was changed discretely on 0.05 μA per day from 0.20 to 1.50 μA.
Single crystal X-ray diffraction study
X-ray diffraction study of (BEDT-TTF)[1,1′-(MeS)2-3,3′-Co(1,2-C2B9H10)2] was carried out at 150 K with an Xcalibur, Eos diffractometer. The structure was solved by direct method followed by Fourier difference synthesis and refined by the full-matrix least-squares method in anisotropic approximation for all non-hydrogen atoms using SHELXL software.45,46 An empirical absorption correction of the experimental intensities was applied.47 The hydrogen atoms were fixed in positions of ideal geometry.
Crystal and experimental data: C16H34B18CoS10, M = 800.54, monoclinic, C2/c, a = 20.4048(6) Å, b = 17.4839(6) Å, c = 18.8696(6) Å, β = 93.682(2)°, V = 830(3) Å3, Z = 8, Dcalcd = 1.58 g cm−3, μ = 1.150 mm−1, reflections observed/independent 20
623/9776, 409 parameters refined, R = 0.037 for 7827 reflections with [F0 > 4σ(F0)].
Atomic coordinates, bond lengths, bond angles, and thermal parameters of the compound have been deposited at the Cambridge Crystallographic Data Centre (CCDC) with a CCDC reference number 1967240. Bond lengths and selected angles are listed in Table S3.†
Electric resistivity measurements
The temperature dependence of electric resistivity of polycrystals of (BEDT-TTF)[1,1′-(MeS)2-3,3′-Co(1,2-C2B9H10)2] was measured using two-contact-probe technique. The crystals were glued on the module with 2 platinum thin wire of diameter 15 μkm using conducting graphite paint. The module was mounted inside of stainless-steel thin-wall tube (diameter ∼11 mm), and the tube was slowly inserted to the transport helium jar with gradual cooling of the sample from 320 K to 265 K with cooling rate of 50–60 K h−1.
Quantum-chemical calculations
The geometry optimizations were performed with the BP86 (ref. 48 and 49) functional with cc-pvdz basis set50–53 without any symmetry restrictions using Gaussain09.54 The tight SCF convergence criteria and ultrafine grid were applied. All minima on the PES were confirmed to have no negative values in their diagonalized force constants matrix. The transition state structures showed only one negative eigenvalue in their diagonalized force constant matrices, and their associated eigenvectors were confirmed to correspond to the motion along the reaction coordinate. The QTAIM analysis was performed using the AIMALL program package55 based on the wave function obtained by the BP86 calculations. The energies of non-covalent intramolecular interactions were calculated using the correlation between the binding energy (EBCP) and the value of the potential energy density function VC(r) in the corresponding critical point (3, −1): EBCP = 0.5VC(r).56,57 Such approach is widely used for energetic analysis of intermolecular interactions of different types.24,40,41,58–60
Conflicts of interest
There are no conflicts to declare.
Acknowledgements
This work was supported by the Russian Science Foundation (16-03-10331). The NMR spectral data were obtained using equipment of Center for Molecular Structure Studies at A. N. Nesmeyanov Institute of Organoelement Compounds operating under support of Ministry of Science and Higher Education of the Russian Federation.
References
- I. B. Sivaev and V. I. Bregadze, Polyhedral Boron Hydrides in Use: Current Status and Perspectives, Nova Science Publishers, Hauppauge, 2009 Search PubMed.
- Boron Science: New Technologies and Applications, ed. N. S. Hosmane, CRC Press, Boca Raton, 2013 Search PubMed.
- R. N. Grimes, Carboranes, Academic Press, London, 3rd edn, 2016 Search PubMed.
- Handbook of Boron Science with Applications in Organometallics, Catalysis, Materials and Medicine (in 4 volumes), ed. N. S. Hosmane and R. Eagling, World Scientific, London, 2019 Search PubMed.
- R. Núñez, M. Tarrés, A. Ferrer-Ugalde, F. Fabrizi de Biani and F. Teixidor, Electrochemistry and Photoluminescence of Icosahedral Carboranes, Boranes, Metallacarboranes, and Their Derivatives, Chem. Rev., 2016, 116, 14307–14378 CrossRef PubMed.
- P. Řezáčová, P. Cígler, P. Matejíček, M. Lepšík, J. Pokorná, B. Grüner and J. Konvalinka, Medicinal Application of Carboranes: Inhibition of HIV Protease, in Boron Science: New Technologies and Applications, ed. N. S. Hosmane, CRC Press, Boca Raton, 2013, pp. 41–70 Search PubMed.
- A. V. Efremenko, A. A. Ignatova, M. A. Grin, I. B. Sivaev, A. F. Mironov, V. I. Bregadze and A. V. Feofanov, Chlorin e6 Fused with a Cobalt-Bis(Dicarbollide) Nanoparticle Provides Efficient Boron Delivery and Photoinduced Cytotoxicity in Cancer Cells, Photochem. Photobiol. Sci., 2014, 13, 92–102 RSC.
- Y. Zheng, W. Liu, Y. Chen, H. Jiang, H. Yan, I. Kosenko, L. Chekulaeva, I. Sivaev, V. Bregadze and X. Wang, A Highly Potent Antibacterial Agent Targeting Methicillin-Resistant Staphylococcus aureus Based on Cobalt Bis(1,2-Dicarbollide) Alkoxy Derivative, Organometallics, 2017, 36, 3484–3490 CrossRef CAS.
- A. B. Olejniczak, B. Nawrot and Z. J. Lesnikowski, DNA Modified with Boron-Metal Cluster Complexes [M(C2B9H11)2] - Synthesis, Properties, and Applications, Int. J. Mol. Sci., 2018, 19, 3501 CrossRef PubMed.
- E. Vaňková, K. Lokočová, O. Maťátková, I. Křížová, J. Masák, B. Grüner, P. Kaule, J. Čermák and V. Šícha, Cobalt Bis-Dicarbollide and Its Ammonium Derivatives are Effective Antimicrobial and Antibiofilm Agents, J. Organomet. Chem., 2019, 899, 120891 CrossRef.
- B. Grüner, J. Brynda, V. Das, V. Šicha, J. Stepankova, J. Nekvinda, J. Holub, K. Pospisilova, M. Fabry, P. Pachtl, V. Král, M. Kugler, V. Masek, M. Medvedikova, S. Matějková, A. Nova, B. Lišková, S. Gurská, P. Džubák, M. Hajdúch and P. Řezáčová, Metallacarborane Sulfamides: Unconventional, Specific, and Highly Selective Inhibitors of Carbonic Anhydrase IX, J. Med. Chem., 2019, 62, 9560–9575 CrossRef PubMed.
- R. D. Kennedy, D. J. Clingerman, W. Morris, C. E. Wilmer, A. A. Sarjeant, C. L. Stern, M. O'Keeffe, R. Q. Snurr, J. T. Hupp, O. K. Farha and C. A. Mirkin, Metallacarborane-Based Metal-Organic Framework with a Complex Topology, Cryst. Growth Des., 2014, 14, 1324–1330 CrossRef CAS.
- V. I. Bregadze, O. A. Dyachenko, O. N. Kazheva, A. V. Kravchenko, I. B. Sivaev and V. A. Starodub, Tetrathiafulvalene-Based Radical Cation Salts with Transition Metal Bis(Dicarbollide) Anions, CrystEngComm, 2015, 17, 4754–4767 RSC.
- M. Tarrés, V. S. Arderiu, A. Zaulet, C. Viñas, F. Fabrizi de Biani and F. Teixidor, How to Get the Desired Reduction Voltage in a Single Framework! Metallacarborane as an Optimal Probe for Sequential Voltage Tuning, Dalton Trans., 2015, 44, 11690–11695 RSC.
- K. R. Pichaandi, L. Nilakantan, A. V. Safronov, Y. V. Sevryugina, S. S. Jalisatgi and M. F. Hawthorne, Electronic Interactions between Ferrocenyl Units Facilitated by the Cobalt Bis(Dicarbollide) Anion Linker: An Experimental and DFT Study, Eur. J. Inorg. Chem., 2017, 666–670 Search PubMed.
- I. Fuentes, A. Andrio, A. García-Bernabé, J. Escorihuela, C. Viñas, F. Teixidor and V. Compañ, Structural and Dielectric Properties of Cobaltacarborane Composite Polybenzimidazole Membranes as Solid Polymer Electrolytes at High Temperature, Phys. Chem. Chem. Phys., 2018, 20, 10173–10184 RSC.
- A. B. Buades, V. S. Arderiu, D. Olid-Britos, C. Viñas, R. Sillanpää, M. Haukka, X. Fontrodona, M. Paradinas, C. Ocal and F. Teixidor, Electron Accumulative Molecules, J. Am. Chem. Soc., 2018, 140, 2957–2970 CrossRef CAS PubMed.
- E. I. Lozinskaya, M. Cotessat, A. V. Shmalko, D. O. Ponkratov, L. V. Gumileva, I. B. Sivaev and A. S. Shaplov, Expanding the Chemistry of Single-Ion Conducting Poly(Ionic Liquid)s with Polyhedral Boron Anions, Polym. Int., 2019, 68, 1570–1579 CrossRef CAS.
- I. Fuentes, T. García-Mendiola, S. Sato, M. Pita, H. Nakamura, E. Lorenzo, F. Teixidor, F. Marques and C. Viñas, Metallacarboranes on the Road to Anticancer Therapies: Cellular Uptake, DNA Interaction, and Biological Evaluation of Cobaltabisdicarbollide [COSAN]−, Chem.–Eur. J., 2018, 24, 17239–17254 CrossRef CAS PubMed.
- I. B. Sivaev and V. I. Bregadze, Chemistry of Cobalt Bis(Dicarbollides). A Review, Collect. Czech. Chem. Commun., 1999, 64, 783–805 CrossRef CAS.
- B. P. Dash, R. Satapathy, B. R. Swain, C. S. Mahanta, B. B. Jena and N. S. Hosmane, Cobalt Bis(Dicarbollide) Anion and Its Derivatives, J. Organomet. Chem., 2017, 849–850, 170–194 CrossRef CAS.
- I. B. Sivaev, Ferrocene and Transition Metal Bis(Dicarbollides) as Platform for Design of Rotatory Molecular Switches, Molecules, 2017, 22, 2201 CrossRef PubMed.
- B. L. Feringa and W. R. Browne, Molecular Switches, Wiley-VCH, Weinheim, 2nd edn, 2011 Search PubMed.
- S. A. Anufriev, S. A. Erokhina, K. Y. Suponitsky, I. A. Godovikov, O. A. Filippov, F. Fabrizi de Biani, M. Corsini, A. O. Chizhov and I. B. Sivaev, Methylsulfanyl-Stabilized Rotamers of Cobalt Bis(Dicarbollide), Eur. J. Inorg. Chem., 2017, 4444–4451 CrossRef CAS.
- S. V. Timofeev, S. A. Anufriev, I. B. Sivaev and V. I. Bregadze, Synthesis of Cobalt Bis(8-Methylthio-1,2-Dicarbollide)-Pentacarbonyltungsten Complexes, Russ. Chem. Bull., 2018, 67, 570–572 CrossRef CAS; S. A. Anufriev, S. V. Timofeev, K. Y. Suponitskyand I. B. Sivaev, Complexation of 8,8’-Bis(Methylsulfanyl) Bis(Dicarbollide) Cobalt with External Metallacomplexes: NMR and X-Ray Study, manuscript in preparation.
- S. V. Timofeev, M. V. Zakharova, E. M. Mosolova, I. A. Godovikov, I. V. Ananyev, I. B. Sivaev and V. I. Bregadze, Tungsten Carbonyl σ-Complexes of nido-Carborane Thioethers, J. Organomet. Chem., 2012, 721–722, 92–96 CrossRef CAS.
- C. Viñas, J. Pedrajas, J. Bertran, F. Teixidor, R. Kivekäs and R. Sillanpää, Synthesis of Cobaltabis(dicarbollyl) Complexes Incorporating Exocluster SR Substituents and the Improved Synthesis of [3,3′-Co(1-R-2-R′-1,2-C2B9H9)2]− Derivatives, Inorg. Chem., 1997, 36, 2482–2486 CrossRef PubMed.
- C. Viñas, J. Pedrajas, F. Teixidor, R. Kivekäs, R. Sillanpää and A. J. Welch, First Example of Bis(dicarbollide) Metallacarborane Containing a B,C′-Heteronuclear Bridge, Inorg. Chem., 1997, 36, 2988–2991 CrossRef PubMed.
- M. Y. Stogniy, S. A. Erokhina, I. D. Kosenko, A. A. Semioshkin and I. B. Sivaev, Dimethyloxonium and Methoxy Derivatives of nido-Carborane and Metal Complexes Thereof, Inorganics, 2019, 7, 46 CrossRef CAS.
- F. Teixidor, J. Pedrajas, I. Rojo, C. Viñas, R. Kivekäs, R. Sillanpää, I. Sivaev, V. Bregadze and S. Sjöberg, Chameleonic Capacity of [3,3′-Co(1,2-C2B9H11)2]− in Coordination. Generation of the Highly Uncommon S(thioether)-Na Bond, Organometallics, 2003, 22, 3414–3423 CrossRef CAS.
- V. I. Bregadze, O. A. Dyachenko, O. N. Kazheva, I. D. Kosenko, A. V. Kravchenko, I. B. Sivaev and V. A. Starodub, Electroconducting Radical-Cation Salts Based on Tetrathiafulvalene Derivatives and Transition Metals Bis(Dicarbollides), Russ. J. Gen. Chem., 2019, 89, 971–987 CrossRef CAS.
- O. N. Kazheva, A. V. Kravchenko, G. G. Aleksandrov, I. B. Sivaev, V. I. Bregadze, I. D. Kosenko, I. A. Lobanova, L. I. Buravov, V. A. Starodub and O. A. Dyachenko, Syntheses, Structures, and Electroconductivity of Bis(ethylenedithio)tetrathiafulvalene (BEDT-TTF) and Bis(methylenedithio)tetrathiafulvalene (BMDT-TTF) Salts with Cobalt 8,8′-Dichloro-3,3′-bis(1,2-dicarbollide), Russ. Chem. Bull., 2014, 63, 1322–1329 CrossRef CAS.
- O. Kazheva, G. Alexandrov, A. Kravchenko, V. Starodub, I. Lobanova, I. Sivaev, V. Bregadze, L. Buravov and O. Dyachenko, First Molecular Conductors with 8,8′-Dibromo Cobalt Bis(dicarbollide) Anion, Solid State Sci., 2008, 10, 1734–1739 CrossRef CAS.
- O. N. Kazheva, G. G. Alexandrov, A. V. Kravchenko, V. A. Starodub, I. A. Lobanova, I. B. Sivaev, V. I. Bregadze, L. V. Titov, L. I. Buravov and O. A. Dyachenko, Molecular Conductors with 8,8′-Diiodo Cobalt Bis(dicarbollide) Anion, J. Organomet. Chem., 2009, 694, 2336–2342 CrossRef CAS.
- O. N. Kazheva, G. G. Aleksandrov, A. V. Kravchenko, V. A. Starodub, G. G. Zhigareva, I. B. Sivaev, V. I. Bregadze, L. I. Buravov, L. V. Titov and O. A. Dyachenko, Synthesis, Structures, and Conductivities of Salts (BEDT-TTF)[9,9′(12′)-I2-3,3′-Co(1,2-C2B9H10)2] and (TTF)[9,9′,12,12′-I4-3,3′-Co(1,2-C2B9H9)2], Russ. Chem. Bull., 2010, 59, 1137–1144 CrossRef CAS.
- O. N. Kazheva, G. G. Alexandrov, A. V. Kravchenko, I. D. Kosenko, I. A. Lobanova, I. B. Sivaev, O. A. Filippov, E. S. Shubina, V. I. Bregadze, V. A. Starodub, L. V. Titov, L. I. Buravov and O. A. Dyachenko, Molecular Conductors with a 8-Hydroxy Cobalt Bis(dicarbollide) Anion, Inorg. Chem., 2011, 50, 444–450 CrossRef CAS PubMed.
- M. Y. Stogniy, S. V. Timofeev, I. B. Sivaev and V. I. Bregadze, Carborane-Based Thioethers and Complexes Thereof, in Handbook of Boron Science with Applications in Organometallics, Catalysis, Materials and Medicine, Vol. 1, Boron in Organometallic Chemistry, ed. N. S. Hosmane and R. Eagling, World Scientific, London, 2019, pp. 21–96 Search PubMed.
- K. T. Mahmudov, M. N. Kopylovich, M. F. C. G. da Silva and A. J. L. Pombeiro, Chalcogen Bonding in Synthesis, Catalysis and Design of Materials, Dalton Trans., 2017, 46, 10121–10138 RSC.
- P. Scilabra, G. Terraneo and G. Resnati, The Chalcogen Bond in Crystalline Solids: A World Parallel to Halogen Bond, Acc. Chem. Res., 2019, 52, 1313–1324 CAS.
- S. A. Anufriev, S. A. Erokhina, K. Y. Suponitsky, A. A. Anisimov, J. N. Laskova, I. A. Godovikov, F. Fabrizi de Biani, M. Corsini, I. B. Sivaev and V. I. Bregadze, Synthesis and Structure of Bis(Methylsulfanyl) Derivatives of Iron Bis(Dicarbollide), J. Organomet. Chem., 2018, 865, 239–246 CrossRef CAS.
- S. A. Anufriev, K. Y. Suponitsky, O. A. Filippov and I. B. Sivaev, Synthesis and Structure of Methylsulfanyl Derivatives of Nickel Bis(Dicarbollide), Molecules, 2019, 24, 4449 CrossRef PubMed.
- S. S. Batsanov, Van der Waals Radii of Elements, Inorg. Mater., 2001, 37, 871–885 CrossRef CAS.
- Handbook of Preparative Inorganic Chemistry, ed. G. Brauer, Academic Press, London, 1963 Search PubMed.
- W. L. F. Armarego and C. L. L. Chai, Purification of Laboratory Chemicals, Butterworth-Heinemann, Burlington, 2009 Search PubMed.
- G. M. Sheldrick, A short history of SHELX, Acta Crystallogr., Sect. A: Found. Crystallogr., 2008, 64, 112–122 CrossRef CAS PubMed.
- G. M. Sheldrick, Crystal structure refinement with SHELXL, Acta Crystallogr., 2015, C71, 3–8 CrossRef PubMed.
- CrysAlis PRO, version 171.35.19, Agilent Technologies Ltd, Yarnton, Oxfordshire, England, 2011 Search PubMed.
- J. P. Perdew, Density-Functional Approximation for the Correlation-Energy of the Inhomogeneous Electron-Gas, Phys. Rev. B: Condens. Matter Mater. Phys., 1986, 33, 8822–8824 CrossRef PubMed.
- A. D. Becke, Density-Functional Exchange-Energy Approximation with Correct Asymptotic-Behavior, Phys. Rev. A, 1988, 38, 3098–3100 CrossRef CAS PubMed.
- T. H. Dunning, Gaussian-Basis Sets for Use in Correlated Molecular Calculations. 1. The Atoms Boron through Neon and Hydrogen, J. Chem. Phys., 1989, 90, 1007–1023 CrossRef CAS.
- D. E. Woon and T. H. Dunning, Gaussian-Basis Sets for Use in Correlated Molecular Calculations. 3. The Atoms Aluminum through Argon, J. Chem. Phys., 1993, 98, 1358–1371 CrossRef CAS.
- N. B. Balabanov and K. A. Peterson, Systematically Convergent Basis Sets for Transition Metals. I. All-Electron Correlation Consistent Basis Sets for the 3d Elements Sc-Zn, J. Chem. Phys., 2005, 123, 064107 CrossRef PubMed.
- N. B. Balabanov and K. A. Peterson, Basis Set Limit Electronic Excitation Energies, Ionization Potentials, and Electron Affinities for the 3d Transition Metal Atoms: Coupled Cluster and Multireference Methods, J. Chem. Phys., 2006, 125, 074110 CrossRef PubMed.
- M. J. Frisch, G. W. Trucks, H. B. Schlegel, G. E. Scuseria, M. A. Robb, J. R. Cheeseman, G. Scalmani, V. Barone, B. Mennucci, G. A. Petersson, H. Nakatsuji, M. Caricato, X. Li, H. P. Hratchian, A. F. Izmaylov, J. Bloino, G. Zheng, J. L. Sonnenberg, M. Hada, M. Ehara, K. Toyota, R. Fukuda, J. Hasegawa, M. Ishida, T. Nakajima, Y. Honda, O. Kitao, H. Nakai, T. Vreven, J. A. Montgomery, Jr, J. E. Peralta, F. Ogliaro, M. Bearpark, J. J. Heyd, E. Brothers, K. N. Kudin, V. N. Staroverov, R. Kobayashi, J. Normand, K. Raghavachari, A. Rendell, J. C. Burant, S. S. Iyengar, J. Tomasi, M. Cossi, N. Rega, J. M. Millam, M. Klene, J. E. Knox, J. B. Cross, V. Bakken, C. Adamo, J. Jaramillo, R. Gomperts, R. E. Stratmann, O. Yazyev, A. J. Austin, R. Cammi, C. Pomelli, J. W. Ochterski, R. L. Martin, K. Morokuma, V. G. Zakrzewski, G. A. Voth, P. Salvador, J. J. Dannenberg, S. Dapprich, A. D. Daniels, Ö. Farkas, J. B. Foresman, J. V. Ortiz, J. Cioslowski and D. J. Fox, Gaussian, Inc., Wallingford, CT, USA, 2009 Search PubMed.
- T. A. Keith, AIMAll (Version 15.05.18), TKGristmill Software, Overland Park KS, USA, 2015 Search PubMed.
- E. Espinosa, I. Alkorta, I. Rozas, J. Elguero and E. Molins, About the Evaluation of the Local Kinetic, Potential and Total Energy Densities in Closed-Shell Interactions, Chem. Phys. Lett., 2001, 336, 457–461 CrossRef CAS.
- E. Espinosa, E. Molins and C. Lecomte, Hydrogen Bond Strengths Revealed by Topological Analyses of Experimentally Observed Electron Densities, Chem. Phys. Lett., 1998, 285, 170–173 CrossRef CAS.
- S. A. Anufriev, I. B. Sivaev, K. Y. Suponitsky, I. A. Godovikov and V. I. Bregadze, Synthesis of 10-Methylsulfide and 10-Alkylmethylsulfonium nido-Carborane Derivatives: B–H···π Interactions between the B–H–B Hydrogen Atom and Alkyne Group in 10-RC≡CCH2S(Me)-7,8-C2B9H11, Eur. J. Inorg. Chem., 2017, 4436–4443 CrossRef CAS.
- K. Y. Suponitsky, N. I. Burakov, A. L. Kanibolotsky and V. A. Mikhailov, Multiple Noncovalent Bonding in Halogen Complexes with Oxygen Organics. I. Tertiary Amides, J. Phys. Chem. A, 2016, 120, 4179–4190 CrossRef CAS PubMed.
- S. A. Anufriev, I. B. Sivaev, K. Y. Suponitsky and V. I. Bregadze, Practical Synthesis of 9-Methylthio-7,8-nido-carborane [9-MeS-7,8-C2B9H11]−. Some Evidences of BH···X Hydride-Halogen Bonds in 9-XCH2(Me)S-7,8-C2B9H11 (X = Cl, Br, I), J. Organomet. Chem., 2017, 849–850, 315–323 CrossRef CAS.
Footnote |
† Electronic supplementary information (ESI) available. CCDC 1967240. For ESI and crystallographic data in CIF or other electronic format see DOI: 10.1039/c9ra08551c |
|
This journal is © The Royal Society of Chemistry 2020 |
Click here to see how this site uses Cookies. View our privacy policy here.