DOI:
10.1039/C9RA08121F
(Paper)
RSC Adv., 2020,
10, 5525-5532
Pre-clinical pharmacokinetic and pharmacodynamic modelling study of 4-hydroxyisoleucine using validated ultra-performance liquid chromatography-tandem mass spectrometry†
Received
7th October 2019
, Accepted 15th January 2020
First published on 4th February 2020
Abstract
A reliable and sensitive ultra-performance liquid chromatography-tandem mass spectrometry-based method has been developed for the estimation of 4-hydroxyisoleucine (4-HI), a potent insulinotropic and hypolipidemic agent. The extraction of 4-HI from plasma was accomplished by the protein precipitation technique using L-isoleucine as an internal standard. The separation of analytes was achieved with a mobile phase consisting of acetonitrile and 0.1% formic acid in an isocratic flow system on a BEH Shield RP-18 column (150 mm × 2.1 mm, 1.7 μm). 4-HI and L-isoleucine were detected using an electrospray ionization (ESI) ion source, using multiple reaction monitoring (MRM) in positive ion mode. The precursor to product ion transitions of 4-HI and L-isoleucine were found at m/z values of 148.19 > 74.02 and 132.17 > 69.04, respectively. As per the guidelines for bioanalytical methods, all validation parameter results were within the acceptable range. The method exhibited a robust and reproducible linearity range of 1–5000 ng mL−1 with a coefficient of regression of 0.9999. The method was successfully applied for the estimation of pharmacokinetic parameters after oral administration of 4-HI (10 mg kg−1) in Wistar rats, by using Thoth Pro (version: 4.3) software. Herein, the two-compartment model was statistically fitted based on AIC and SBC values for evaluation of the pharmacokinetic parameters of 4-HI. Pharmacodynamic studies were also performed by measuring the levels of triglyceride and total cholesterol, and showed that the pharmacokinetic and pharmacodynamic data of 4-HI correlated with each other.
1. Introduction
Trigonella foenum-graecum (TFG) (Leguminosae) is a known traditional plant that is frequently used in the management of various diseases in Asian, Mediterranean and Middle Eastern countries.1–3 The seeds of the TFG plant mainly contain dietary fiber (soluble fiber and insoluble fiber), essential oils, proteins, saponins, fatty acids, alkaloids (trigonelline, choline, etc.), and free amino acids (4-hydroxyisoleucine, lysine), which have proven therapeutic benefits, including anti-diabetic, anti-lipidemic, anti-hypocholesterolemic, hepatoprotective, antioxidant, anti-inflammatory, and anti-carcinogenic properties, in rodents as well as in clinical trials.4–6
4-Hydroxyisoleucine (4-HI) is a natural compound, obtained from TFG seeds and synthesized from isoleucine. It is one of the main constituents responsible for the anti-hyperglycemic effect of the TFG plant.7,8 4-HI plays a vital role in lowering glucose level by regulating the secretion of pancreatic insulin, thereby inhibiting the activation of α-amylase and sucrase, and also regulating the level of lipids.9 Hence, it shows significant potential in the treatment of insulin resistance and can possibly be used to treat cardiovascular diseases.10 Hari et al. evaluated the insulinotropic and anti-diabetic effects of 4-HI in animal models. They examined the effect of 4-HI on liver function and blood glucose in two rat models of insulin resistance, the fructose-fed and streptozotocin-induced rat models. From the study, they found that 4-HI can be used for the treatment of insulin resistance.11 T. Narender et al. estimated the anti-dyslipidemic and anti-hyperglycemic effects of 4-HI in hamsters. In this study, a high-fat diet-fed dyslipidemia hamster model was developed, and the authors found a significant decrease in the plasma triglyceride level, total cholesterol, and free fatty acids, which was accompanied by an increase in the HDL/TC ratio after the administration of 4-HI.7 Moreover, Mohammed R. Haeri and co-workers observed that 4-HI shows anti-diabetic activity and helps in restoring the levels of lipids and uric acid in diabetic rats. They concluded that 4-HI acts as an adjunct in the treatment of type 1 and type 2 diabetes.12 Therefore, these promising pharmacological effects provoked us to investigate and evaluate the pharmacokinetic and pharmacodynamic profile of 4-HI. Herein, the present study aimed to develop a rapid, precise and sensitive ultra-performance liquid chromatography-tandem mass spectrometry (UPLC-MS/MS) method for the estimation of 4-HI in rat plasma, and to the best of our knowledge, the pharmacokinetic data for 4-HI in Wistar rat plasma has been generated for the first time. The current investigation also explored possible compartmental pharmacokinetic and pharmacodynamic studies in Wistar rats after oral administration of 4-HI (10 mg kg−1). Evaluation of the pharmacokinetic parameters was performed by non-compartmental and compartmental analyses based on the data obtained from the concentration–time profile using Thoth Pro™ 4.3 software. Whereas, the pharmacodynamic studies, especially of the anti-dyslipidemic effect of 4-HI, were carried out by estimating the levels of total cholesterol (TC) and triglycerides (TG).
2. Materials and methods
2.1 Chemicals
4-HI and L-isoleucine standards were procured from Sigma Aldrich (St. Louis, MO, USA). The membrane filter (0.22 μm) was purchased from Merck Limited (Mumbai, India). LC-MS grade solvents like methanol, acetonitrile, and formic acid were obtained from Sigma Aldrich (St. Louis, MO, USA). Syringe filters (13 mm × 0.22 μm) were procured from Chromatopak Pvt. Ltd. (Mumbai, India). Deionized ultra-pure water was obtained from a Millipore Milli-Q Plus system (Millipore Bedford Corp., Bedford, MA, USA). Commercial kits for the determination of TG and TC were purchased from Coral Clinical Systems.
2.2 Experimental animals
Wistar rats (male, 200–220 g) were procured from the Central Animal Facility of Birla Institute of Technology & Science, Pilani, India. Rats were kept in polyacrylic cages and housed under a maintained 12 h/12 h light/dark cycle at ambient temperature (22 ± 3 °C) with 65% relative humidity. All experimental procedures were performed as per institutional guidelines, which are in compliance with the Institutional Animal Ethics Committee of BITS-Pilani and an approved protocol (IAEC/RES/23/10). Both food and water were provided ad libitum throughout the study period.
2.3 Instrumental and analytical conditions
Liquid chromatographic analysis was performed using an Acquity UPLC H Class system equipped with a quaternary solvent manager, an auto-sampler, a column oven, and a degasser. Chromatographic separation was achieved using an Acquity UPLC® BEH Shield RP 18 column (150 mm × 2.1 mm, 1.7 μm; Waters, USA) in isocratic mode using acetonitrile and 0.1% formic acid in a ratio of 20
:
80 with a flow rate of 0.4 mL min−1. The column oven and autosampler temperature were maintained at 30 °C and 10 °C, respectively, and the total run time was set for 1.2 min. Data acquisition and control of hardware were executed by using Mass Lynx software version 4.1.
Mass spectrometric detection was accomplished using a Waters Xevo® Triple Quadrupole mass spectrometer (MS/MS) equipped with an electrospray ionization (ESI) source. The following setup was used for analysis: ion spray voltage, 4500 V; capillary voltage, 3 kV; cone voltage, 60 V; source temperature, 140 °C; dwell time, 0.025 s; the desolvation gas flow rate and temperature were 650 L h−1 and 350 °C respectively. The detection and quantification of analytes were performed using the multiple reaction monitoring (MRM) mode, and the precursor to product ion transitions of 4-HI and L-isoleucine were found at m/z values of 148.19 > 74.02 and 132.17 > 69.04, respectively, as shown in Table 1.
Table 1 Ion transitions and other optimized parameters for 4-hydroxyisoleucine and L-isoleucine
Compound name |
Ion transition |
Cone voltage (eV) |
Collision energy (eV) |
Acquisition time (min) |
4-Hydroxyisoleucine |
148.19 > 74.02 |
24 |
12 |
0.92 |
L-Isoleucine |
132.17 > 69.04 |
24 |
12 |
0.96 |
2.4 Preparation of standard stock solutions and quality control samples
A standard solution of 4-HI (1 mg mL−1) was prepared in acetonitrile. Further, a series of dilutions of 4-HI were prepared in acetonitrile and water (50
:
50) with concentrations ranging from 0.025–125 μg mL−1. The quality control samples for 4-HI were prepared with concentrations of 0.025, 0.05, 37.5 and 100 μg mL−1 and stored at 4 °C. The calibration standards were developed by spiking 2 μL of the respective working solutions into 48 μL of blank plasma. The final concentrations of 4-HI in the plasma samples were in the range of 1–5000 ng mL−1, and quality control (QC) samples with concentrations of 1, 2, 1500 and 4000 ng mL−1 were prepared individually in replicates, independent of the calibration standards.
2.5 Sample preparation
A simple protein precipitation method was used for the extraction of 4-HI from plasma. 300 μL of acetonitrile was added to the plasma sample, vortexed for 30 s and centrifuged for 10 min at 15
000 rpm. The obtained supernatant was filtered through a syringe filter (0.22 μm) into amber colored HPLC vials and analyzed using the UPLC-MS/MS method (Table S1†).
2.6 Method validation
The validation of the bioanalytical method was achieved in terms of various parameters such as sensitivity, selectivity, linearity, accuracy, precision, recovery, matrix effect and stability studies in accordance with the US Food and Drug Administration (US FDA) and European Medical Agency (EMA) guidelines.13–16 System suitability was assessed by injecting the same concentration of the sample six times to check the efficiency of the column, reproducibility and resolution. The system suitability test was performed to confirm the function of the chromatography system on a day-to-day basis, and the selectivity was measured to test its ability to differentiate between the analyte and other components such as impurities and endogenous substances. For the selectivity test, blank plasma samples were collected randomly and analyzed by spiking with an internal standard (IS) (zero blank) or without an IS, and the method was considered selective when the extraction ion chromatogram peak intensity was less than 5 times larger than the peak intensity of the lower limit of quantification (LLOQ) and less than 20 times larger than the peak intensity of the IS. Linearity and calibration curves were obtained by using a least squares method, typically the ordinary least squares method (OLS). OLS is a simple process and usually involves the assumption of an initial point for the selection of an adequate calibration curve, but with OLS assessing the homoscedasticity.17 However, in the bioanalytical method the observed value of variance remains unequal, which suggests heteroscedasticity and leads to an inadequate fit of the OLS linear regression. Thus, an alternative approach, weighted least squares (WLS) linear regression, is used for the calculation of the standard curve (Fig. S2 and Table S4†).18,19 Calibration standards of 4-HI (1–5000 ng mL−1) were used to prepare the calibration curve for the plasma samples by plotting nominal plasma concentrations on the x-axis versus peak area ratios (drug/IS) on the y-axis. To obtain the error of accuracy and coefficient of variation, the validation criteria (CV) should be ±15% for all calibrations, except for the LLOQ (±20%).20 The limit of detection (LOD) and limit of quantification (LOQ) were measured as signal to noise ratios. Accuracy, and intra-day and inter-day assay precision were estimated by analyzing six replicate samples at three different quality control (QC) levels, i.e., lower quality control (LQC), medium quality control (MQC), and high quality control (HQC). The accuracy, and intra-day and inter-day assay precision were determined over a day or over three consecutive days. The acceptance criteria for intra and inter-day precision were limited to ≤15% (RSD, %) and accuracy was within ±15% except for the LLOQ, where it should not exceed ±20% for precision and accuracy. Recovery was estimated using the ratio of the compound concentration (relative) or peak area of extraction ion chromatogram (absolute) in plasma.21 The recoveries of 4-HI and the IS were determined through the protein precipitation extraction procedure and estimated by comparing the standard solution with equivalent concentrations. For the stability studies of 4-HI in plasma matrix, six replicates at the different QC levels (LQC, MQC, HQC) and LLOQ were estimated under the following conditions: (1) in the autosampler at 4 °C for 24 h; (2) bench top (at ambient temperature (25 ± 3 °C)) for 9 h; (3) over three freeze–thaw cycles at −80 °C. Stability studies were carried out by comparing the stability samples with freshly spiked samples. Stability samples were considered to be stable if the assay values were within the adequate limits of accuracy (i.e., ±15% bias) and precision (i.e., 15% RSD), except for the LLOQ (i.e., 20% of CV).22
2.7 Pharmacokinetic study in Wistar rats
The established method was efficiently executed for the pharmacokinetic study of 4-HI after its administration p.o. at a dose of 10 mg kg−1 in healthy Wistar rats (n = 4). Blood samples were collected in polypropylene centrifuge tubes at intervals of 0.25, 0.5, 1, 2, 4, 6, 8, 12 and 24 h. For the extraction of the plasma, blood samples were centrifuged at 7000 rpm for 10 min and stores at −80 ± 10 °C until analysis. The plasma concentration–time profile of 4-HI was plotted to generate various pharmacokinetic parameters using Thoth Pro 4.3 software with non-compartmental modeling. Further, the estimation of the pharmacokinetic models (one, two or multi-compartment) was done based on Akaike’s information criterion (AIC), Schwarz Bayesian Criteria (SBC) and the linear regression coefficient (R2) value. Lower AIC and SBC values were preferred for the estimation of a perfect pharmacokinetic model.23–25
2.8 Pharmacodynamic study
TC and TG are the commonly used biological markers for the estimation of lipid levels. The pharmacodynamic effect of 4-HI on TC and TG was explored in Wistar rats (n = 4). The TC and TG levels in plasma were estimated by using commercial kits.26,27
3. Results and discussion
3.1 Optimization of liquid chromatographic conditions and mass spectrometry conditions
A methodological approach was followed for optimizing the method for identification of the analyte in the shortest time using the simplest possible chromatographic conditions with symmetrical peaks, and high resolution and recovery. For this, the liquid chromatographic conditions such as choice of the mobile phase, pH of buffer, composition of the mobile phase, column selection, flow rate, column oven temperature, and injection volume were optimized. For the MS/MS conditions, the electrospray ionization (ESI) source was operated in different modes, and the m/z values of the analyte and the IS were determined. The compound and instrument dependent parameters were also established to improve the sensitivity towards the analyte. After continuous optimization of 4-HI, the mobile phase consisted of acetonitrile and 0.1% formic acid in a ratio of 20
:
80 with a flow rate of 0.4 mL min−1. No carryover was detected in a blank sample after injecting the calibration standard with the highest concentration.28 The positive ESI mode was selected for estimation of m/z values (Table S2†). The MRM scan was used to improve the specificity and selectivity of the analysis, and the product ions with the highest abundance were chosen as the transitions for each analyte (Fig. 1 and S1 and Table S3†).29,30 The proposed fragmentation pathways, MS/MS transitions and the compound dependent parameters for 4-HI and the IS are summarized in Table 1.
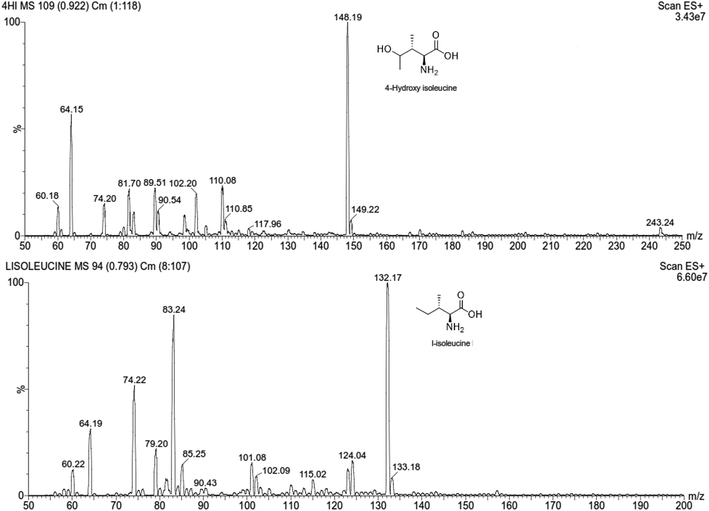 |
| Fig. 1 Product ion scan mass spectra of 4-hydroxyisoleucine and L-isoleucine. | |
3.2 Method validation
Selectivity studies of the developed LC-MS/MS method were carried out by comparing and analyzing the chromatograms of 4-HI with blank and zero blank chromatograms. Representative chromatograms of blank, zero blank (IS only), LLOQ and standard samples of 4-HI are shown in Fig. 2. The current conditions showed no significant plasma matrix and no interfering peaks at the retention times of the analytes. The calibration curve was obtained using the least squares regression technique, which is used for modeling and analyzing the relation between dependent variables and independent variables. Primary regression with the least squares method is used for fitting calibration curves in LC-MS/MS assays,31 although the evaluation of the fit of the model was completed by an alternative approach of assessing the homoscedasticity or heteroscedasticity using the F-test and a residual plot (Fig. S2†). The observed results show heteroscedasticity of the errors, so a modified least squares method, WLS, was used, which assigns an appropriate weight at each concentration level and reflects the different measurement uncertainties at different levels (Table S4†).18 Herein, a calibration curve was constructed for a calibration standard of 4-HI in plasma samples using the WLS method, and it shows linearity and reproducibility in the range of 1–5000 ng mL−1. The accuracy, and intra-day and inter-day assay precision data obtained from the QC (LQC, MQC, HQC) and LLOQ samples of 4-HI are listed in Table 2, and all the obtained values were within the range of recommended guidelines. The intra-day and inter-day assay precision for the QC samples did not exceed 7.116%, and for the LLOQ they did not exceed 11.660%. Thus, the analysis offers excellent extraction procedure, repeatability, and reproducibility for the study of 4-HI in plasma. The extraction recovery of 4-HI (at QC levels and LLOQ) was measured by comparing neat standards versus plasma-extracted standards. The obtained recovery values for the plasma samples are listed in Table 3, and the mean absolute recovery of 4-HI was found to be 41.56 ± 1.85, which indicates that the method was reliable, rapid and reproducible. Stability studies of 4-HI in plasma matrix were carried out to cover all possible conditions that would be encountered during sample processing. For the stability studies, different conditions were tested, such as freeze–thaw stability, autosampler stability and bench top stability, and the results indicated that 4-HI was stable and exhibited no significant degradation under these storage conditions as described in Table 4.
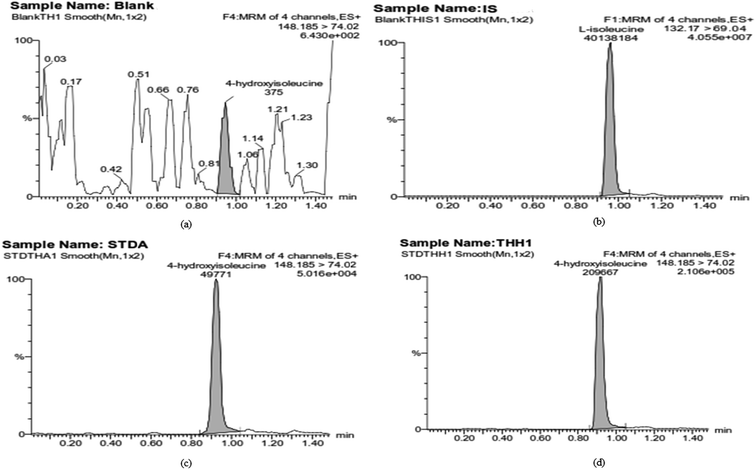 |
| Fig. 2 Representative MRM chromatograms of 4-HI: (a) blank; (b) IS; (c) LLOQ; (d) sample. | |
Table 2 Precision and accuracy of the analysis of 4-hydroxyisoleucine in rat plasma
Level |
Nominal concentration (ng mL−1) |
Inter-day |
Intra-day |
Measured concentration (mean ± SD, ng mL−1) |
Precision (% CV) |
Accuracy (% bias) |
Measured concentration (mean ± SD, ng mL−1) |
Precision (% CV) |
Accuracy (% bias) |
LLOQ |
1 |
1.00 ± 0.12 |
11.660 |
0.263 |
0.98 ± 0.10 |
9.876 |
−1.733 |
LQC |
2 |
2.02 ± 0.14 |
7.116 |
0.808 |
1.94 ± 0.13 |
6.768 |
−2.798 |
MQC |
1500 |
1370.29 ± 46.64 |
3.406 |
−8.647 |
1325.51 ± 37.23 |
2.809 |
−11.633 |
HQC |
4000 |
3716.68 ± 171.74 |
4.621 |
−7.083 |
3755.09 ± 138.91 |
3.699 |
−6.123 |
Table 3 Recovery (%) of 4-hydroxyisoleucine in rat plasma at different QC levels
Level |
Nominal concentration (ng mL−1) |
% Recovery |
Mean ± SD |
% CV |
LLOQ |
1 |
41.35 ± 2.69 |
6.515 |
LQC |
2 |
40.32 ± 0.89 |
2.213 |
MQC |
1500 |
41.99 ± 1.35 |
3.204 |
HQC |
4000 |
42.55 ± 2.46 |
5.791 |
Table 4 Stability of 4-hydroxyisoleucine in rat plasma under different storage conditions and at different QC levels
Stability |
Nominal concentration (ng mL−1) |
Measured concentration (ng mL−1 ± SD) |
Precision (% CV) |
Accuracy (% bias) |
Autosampler (24 h) |
1 |
1.02 ± 0.06 |
5.588 |
1.892 |
2 |
1.96 ± 0.17 |
8.627 |
−1.951 |
1500 |
1499.41 ± 14.62 |
0.975 |
−0.040 |
4000 |
3902.95 ± 187.10 |
4.794 |
−2.426 |
Bench top (9 h) |
1 |
1.09 ± 0.11 |
9.908 |
8.838 |
2 |
1.96 ± 0.13 |
6.814 |
−2.003 |
1500 |
1502.65 ± 31.68 |
2.108 |
0.176 |
4000 |
3894.00 ± 227.47 |
5.842 |
−2.650 |
Freeze–thaw (−80 °C) |
1 |
1.05 ± 0.13 |
12.674 |
5.271 |
2 |
2.01 ± 0.18 |
9.133 |
0.497 |
1500 |
1417.04 ± 40.97 |
2.891 |
−5.531 |
4000 |
3751.70 ± 138.34 |
3.873 |
−10.707 |
3.3 Pharmacokinetic study in Wistar rats
The validated method was successfully applied in estimating the pharmacokinetic behavior of 4-HI at a dose of 10 mg kg−1 p.o. in Wistar rats (n = 4). The plasma concentration–time profile curve of 4-HI in rat plasma is depicted in Fig. 3. The plasma concentration increased rapidly and reached the maximum level at 0.5 h after administration of 4-HI. The estimated model independent and non-compartmental pharmacokinetic parameters are listed in Table 5. A maximum concentration (Cmax) of 8140.37 ± 623.48 ng mL−1 was achieved, and the areas under the curve (AUC0−t and AUC0−α) were found to be 39
034.76 ± 1345.06 and 45
892.54 ± 702.92 ng h mL−1, respectively. The plasma elimination half-life (t1/2) obtained for 4-HI was 10.83 ± 1.96 h, and the analyte could be detected in plasma for up to 24 h.
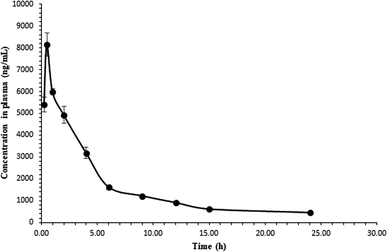 |
| Fig. 3 Plasma concentration–time profile of 4-HI after oral administration (10 mg kg−1, p.o.). | |
Table 5 Non-compartmental and compartmental pharmacokinetic parameters of 4-hydroxyisoleucine after administration at a dose of 10 mg kg−1 p.o. in rats
Parameters |
4-Hydroxyisoleucine (10 mg kg−1; p.o.) |
Non-compartmental |
One-compartmental |
Two-compartmental |
Cmax |
ng mL−1 |
8140.37 ± 623.48 |
4101.04 ± 540.10 |
8042.97 ± 279.16 |
AUC0−t |
ng h mL−1 |
39 034.76 ± 1345.06 |
39 495.34 ± 1290.42 |
40 016.39 ± 1547.58 |
AUC0−α |
ng h mL−1 |
45 892.54 ± 702.92 |
46 353.11 ± 788.01 |
47 050.02 ± 1223.01 |
Ke |
h−1 |
0.06 ± 0.01 |
0.06 ± 0.01 |
0.06 ± 0.01 |
tmax |
h |
0.5 ± 0.00 |
— |
— |
t1/2α |
h |
— |
— |
17.470 ± 12.73 |
t1/2β |
h |
— |
— |
0.021 ± 0.010 |
t1/2 |
h |
10.83 ± 1.96 |
— |
— |
CL |
mL h−1 kg−1 |
204.95 ± 23.97 |
32.372 ± 6.88 |
17.412 ± 7.71 |
Vd |
mL kg−1 |
3123.59 ± 355.86 |
— |
— |
V1 |
mL kg−1 |
— |
679.546 ± 20.22 |
309.492 ± 162.89 |
V2 |
mL kg−1 |
— |
— |
569.144 ± 82.93 |
K10 |
h−1 |
— |
0.0483 ± 0.01 |
0.149 ± 0.01 |
K12 |
h−1 |
— |
— |
0.08 ± 0.05 |
K21 |
h−1 |
— |
— |
0.12 ± 0.00 |
R2 |
— |
— |
0.947 ± 0.04 |
0.997 ± 0.00 |
AIC |
— |
— |
162.99 ± 2.37 |
156.52 ± 2.50 |
SBC |
— |
— |
164.14 ± 2.37 |
158.34 ± 2.50 |
The selection of a suitable compartmental model, either one- or two-compartmental, was mainly based upon the AIC and SBC values. Smaller AIC and SBC values indicate an appropriate profile for the concentration–time curve.25 The mean AIC and SBC values for the one-compartmental model were 162.93 ± 2.37 and 164.14 ± 2.37, respectively, and for the two-compartmental model they were 156.62 ± 2.5 and 158.34 ± 2.5, respectively. According to the data, the two-compartmental model was the best model to describe the plasma concentration–time profile of 4-HI after oral administration (Fig. 4). The generated data also suggests that there are fast and slow disposition phases, which are presented in the generated graph (Fig. 5). The equation for the extravascular two-compartmental model is Cp(t) = Ne−Ket + Le−αt + Me−βt. The pharmacokinetic parameters Ke, α and β are the absorption, distribution and elimination exponents, whereas the N, L and M values are the intercepts for absorption, distribution and elimination phases, respectively.32 Moreover, all the pharmacokinetic parameters of 4-HI are presented in Table 5.
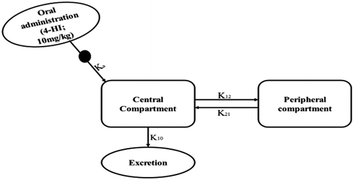 |
| Fig. 4 Schematic representation of the two-compartmental model for 4-HI. Where Ke: 4-HI absorption in the gastrointestinal tract; K12: 4-HI disposition from the central compartment to the peripheral compartment; K21: reabsorption from the peripheral compartment to the central compartment; K10: 4-HI elimination from the central compartment. | |
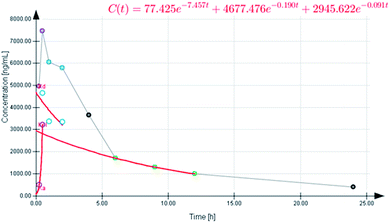 |
| Fig. 5 Plasma concentration–time profile of 4-HI obtained using the two-compartment model after oral administration (10 mg kg−1). | |
3.4 Pharmacodynamic study
Hyperlipidemia plays a crucial part in the pathogenesis of type 2 diabetes, which is triggered due to the storage of fats in tissues, and it also leads to increased incidence of cardiovascular diseases.33 According to Hannan et al., the TFG plant effectively reduces the postprandial rise of glucose in rats and also hinders fat and carbohydrate absorption in the gut due to the presence of bioactive constituents.34 Whereas, Nerander et al. observed that the amino acid (4-HI) present in the TFG plant causes a significant decrease in the levels of TC, TG and fatty acids in the dyslipidemic hamster model.7 Similarly, a report by Singh et al. also confirmed the anti-dyslipidemic effect of 4-HI in db/db mice by measuring the levels of TG, TC, low-density lipoprotein and high-density lipoprotein.35 In the present study, we have observed the decrement of TG and TC levels after the administration of 4-HI in normal rats, as presented in Fig. 6 and 7, respectively. The decrement in the levels of TG and TC was in agreement with the pharmacokinetic profile. Moreover, the maximum inhibitor effect of 4-HI was observed at 2 h; after that, an increment in the levels of TG and TC was observed in the concentration–time profiles.
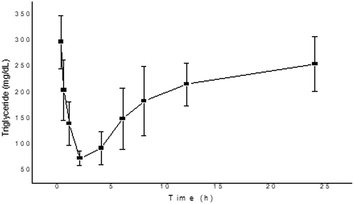 |
| Fig. 6 Change in triglyceride level after the administration of 4-HI in normal Wistar rats. Values are expressed as mean ± SEM. | |
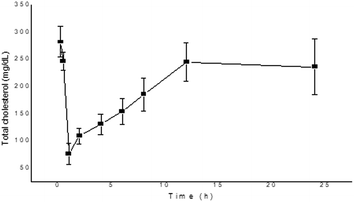 |
| Fig. 7 Change in total cholesterol level after the administration of 4-HI in normal Wistar rats. Values are expressed as mean ± SEM. | |
4. Conclusion
The present study aimed to develop a bioanalytical assay method for the quantification of 4-HI in plasma matrix and also to explore the non-compartmental as well as compartmental pharmacokinetic parameters of 4-HI. The developed UPLC-MS/MS method is simple, sensitive, and high throughput, with an eco-friendly mobile phase composition. The validated method was applied in the estimation of pharmacokinetic parameters. The plasma concentration data showed fast and slow disposition phases as fitted by the two-compartmental model. Moreover, the pharmacodynamic profile also indicated the anti-hyperlipidemic effect of 4-HI, and a good correlation was observed with the pharmacokinetic profile. To the best of our knowledge, this method is the first of its kind to be applied in the evaluation of pharmacokinetic as well as pharmacodynamic parameters of 4-HI in rat plasma. Our constructive findings offer complete information regarding the compartmental pharmacokinetics of 4-HI. These pre-clinical pharmacokinetic and pharmacodynamic studies and the relationship between them could play a significant role in the development of new dosage forms of 4-HI which may be helpful in the management of various diseases.
Abbreviations
4-HI | 4-Hydroxyisoleucine |
TFG | Trigonella foenum-graecum |
UPLC-MS/MS | Ultra-performance liquid chromatography-tandem mass spectrometry based |
TC | Total cholesterol |
TG | Triglycerides |
ESI | Electrospray ionization |
MRM | Multiple reaction monitoring |
Conflicts of interest
The authors have no conflict of interest.
Acknowledgements
The authors are immensely grateful to the Indian Council of Medical Research (ICMR), New Delhi, India for providing financial support.
References
- K. Srinivasan, Food Rev. Int., 2006, 22, 203–224 CrossRef CAS.
- S. Zameer, A. K. Najmi, D. Vohora and M. Akhtar, Nutr. Neurosci., 2018, 21, 539–545 CrossRef CAS PubMed.
- R. C. Garg, Nutraceuticals, 2016, 599–617 Search PubMed.
- M. Ouzir, K. El Bairi and S. Amzazi, Food Chem. Toxicol., 2016, 96, 145–154 CrossRef CAS PubMed.
- N. Neelakantan, M. Narayanan, R. J. de Souza and R. M. van Dam, Nutr. J., 2014, 13(1), 7 CrossRef PubMed.
- U. C. S. Yadav and N. Z. Baquer, Pharm. Biol., 2014, 52, 243–254 CrossRef PubMed.
- T. Narender, A. Puri, Shweta, T. Khaliq, R. Saxena, G. Bhatia and R. Chandra, Bioorg. Med. Chem. Lett., 2006, 16(2), 293–296 CrossRef CAS PubMed.
- Y. Sauvaire, P. Petit, C. Broca, M. Manteghetti, Y. Baissac, J. Fernandez-Alvarez, R. Gross, M. Roye, A. Leconte, R. Gomis and G. Ribes, Diabetes, 1998, 47, 206–210 CrossRef CAS PubMed.
- A. Avalos-Soriano, R. De la Cruz-Cordero, J. Rosado, T. Garcia-Gasca, A. Avalos-Soriano, R. De la Cruz-Cordero, J. L. Rosado and T. Garcia-Gasca, Molecules, 2016, 21, 1596 CrossRef PubMed.
- C. Broca, V. Breil, C. Cruciani-Guglielmacci, M. Manteghetti, C. Rouault, M. Derouet, S. Rizkalla, B. Pau, P. Petit, G. Ribes, A. Ktorza, R. Gross, G. Reach and M. Taouis, Am. J. Physiol.: Endocrinol. Metab., 2004, 287, E463–E471 CrossRef CAS PubMed.
- Y. Zhang, G. Hu, H. Lin, S. Hong, Y. Deng, J. Tang, S. W. Seto, Y. Kwan, M. M. Waye, Y. Wang and S. M. Lee, Angiogenesis, 2009, 1213, 1205–1213 Search PubMed.
- M. R. Haeri, H. K. Limaki, C. J. B. White and K. N. White, Phytomedicine, 2012, 19, 571–574 CrossRef CAS PubMed.
- FDA and CDER, Bioanalytical Method Validation Guidance for Industry Biopharmaceutics Bioanalytical Method Validation Guidance for Industry Biopharmaceutics Contains Nonbinding Recommendations, 2018.
- ICH guideline M10 on bioanalytical method validation 4 Step 2b 5, 2019.
- P. Singh, V. Bajpai, A. Gupta, A. N. Gaikwad, R. Maurya and B. Kumar, Ind. Crops Prod., 2019, 127, 26–35 CrossRef CAS.
- S. K. Dubey, R. N. Saha, H. Jangala and S. Pasha, J. Pharm. Anal., 2013, 3, 466–471 CrossRef PubMed.
- E. Rozet, R. D. Marini, E. Ziemons, B. Boulanger and P. Hubert, J. Pharm. Biomed. Anal., 2011, 55, 848–858 CrossRef CAS PubMed.
- C. P. da Silva, E. S. Emídio and M. R. R. de Marchi, Talanta, 2015, 131, 221–227 CrossRef CAS PubMed.
- S. K. Dubey, S. Duddelly, H. Jangala and R. Saha, Indian J. Pharm. Sci., 2013, 75, 89–93 CrossRef CAS PubMed.
- J. H. Jo, J. H. Kim, H. S. Lee, G. S. Jeong, J. M. Lee and S. Lee, Fitoterapia, 2017, 120, 194–198 CrossRef CAS PubMed.
- Jyotshna, P. Gaur, D. K. Singh, S. Luqman and K. Shanker, Ind. Crops Prod., 2017, 95, 33–42 CrossRef CAS.
- A. Khosa, K. V. Krishna, R. N. Saha, S. K. Dubey and S. Reddi, J. Liq. Chromatogr. Relat. Technol., 2018, 41, 692–697 CrossRef CAS.
- K. V. Krishna, R. N. Saha, G. Singhvi and S. K. Dubey, RSC Adv., 2018, 8, 24740–24749 RSC.
- W.-l. Wei, Y.-l. An, Z.-w. Li, Y.-y. Wang, H.-j. Ji, J.-j. Hou, W.-y. Wu and D.-a. Guo, Phytomedicine, 2019, 152971 CrossRef PubMed.
- K. V. Krishna, R. N. Saha, A. Puri, M. Viard, B. A. Shapiro and S. K. Dubey, Photochem. Photobiol. Sci., 2019, 18(5), 1056–1063 RSC.
- S. P. Subramanian and G. S. Prasath, Biomedicine & Preventive Nutrition, 2014, 4, 475–480 Search PubMed.
- M. Nisha, B. N. Vinod and C. Sunil, Future Journal of Pharmaceutical Sciences, 2018, 4, 150–155 CrossRef.
- A. A. Bajrai, E. Ezzeldin, K. A. Al-Rashood, M. Raish and M. Iqbal, J. Anal. Toxicol., 2016, 40, 133–139 CrossRef CAS PubMed.
- L. P. Köse, I. Gülçin, A. C. Gören, J. Namiesnik, A. L. Martinez-Ayala and S. Gorinstein, Ind. Crops Prod., 2015, 74, 712–721 CrossRef.
- N. Tlili, B. Kirkan and C. Sarikurkcu, Ind. Crops Prod., 2019, 128, 147–152 CrossRef CAS.
- H. Gu, G. Liu, J. Wang, A.-F. Aubry and M. E. Arnold, Anal. Chem., 2014, 86, 8959–8966 CrossRef CAS PubMed.
- K. M. Alkharfy, A. Ahmad, R. M. A. Khan and W. M. Al-Shagha, Eur. J. Drug Metab. Pharmacokinet., 2015, 40, 319–323 CrossRef CAS PubMed.
- V. P. Veerapur, K. R. Prabhakar, B. S. Thippeswamy, P. Bansal, K. K. Srinivasan and M. K. Unnikrishnan, Food Chem., 2012, 132, 186–193 CrossRef CAS PubMed.
- J. M. A. Hannan, B. Rokeya, O. Faruque, N. Nahar, M. Mosihuzzaman, A. K. Azad Khan and L. Ali, J. Ethnopharmacol., 2003, 88, 73–77 CrossRef CAS PubMed.
- A. B. Singh, A. K. Tamarkar, Shweta, T. Narender and A. K. Srivastava, Nat. Prod. Res., 2010, 24(3), 258–265 CrossRef CAS PubMed.
Footnotes |
† Electronic supplementary information (ESI) available. See DOI: 10.1039/c9ra08121f |
‡ Equal contributions. |
|
This journal is © The Royal Society of Chemistry 2020 |