DOI:
10.1039/D0QO00377H
(Review Article)
Org. Chem. Front., 2020,
7, 1395-1417
Recent developments in palladium-catalyzed C–S bond formation
Received
27th March 2020
, Accepted 18th April 2020
First published on 22nd April 2020
Abstract
Transition metal-catalyzed C–S bond formation reactions have attracted considerable attention in the recent years. In this regard, palladium-catalyzed C–S bond formation is typically attractive because it can effectively assemble structurally diverse unsymmetrical aryl sulfides and functionalized sulfones under mild conditions with high regioselectivities. In this review, palladium-catalyzed C–S bond formation reactions are summarized and discussed in detail with the focus on sulfenylation and sulfonylation reactions involving aryl/heteroaryl C–H bonds, alkynes, aryl halides, electron-rich benzoic acid derivatives, arylhydrazines, and organosilicon reagents. Some representative synthetic strategies and their transformation application along with reaction mechanisms are also highlighted.
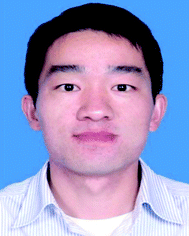 Jianxiao Li | Jianxiao Li received his B.Sc. degree (2008) from Fuyang Normal University. He earned his M.Sc. under the supervision of Prof. Zhao-Yang Wang at South China Normal University in 2011. He completed his Ph.D. study in 2014 under the direction of Prof. Huanfeng Jiang in South China University of Technology. From 2014 to 2017, Dr Li worked as a postdoctoral fellow with Prof. Huanfeng Jiang. He has now joined the School of Chemistry and Chemical Engineering at South China University of Technology as a lecturer. His current research interests include transition metal-catalyzed coupling reactions and green chemistry. |
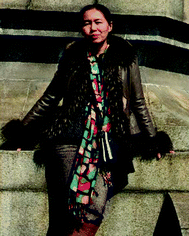 Shaorong Yang | Shaorong Yang obtained her B.Sc. and M.Sc. degree from Wuhan University in 1997 and 2000, respectively. She then moved to South China University of Technology, and received her Ph.D. in 2008 under the supervision of professor Huanfeng Jiang. Since 2016 she has been an associate professor at the School of Chemistry and Chemical Engineering at the same University. Her research interests include palladium-catalyzed cascade reactions of unactivated alkenes in green solvents, especially in water and ionic liquids. |
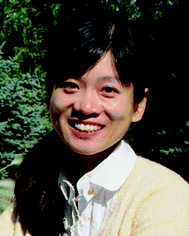 Wanqing Wu | Prof. Wanqing Wu received her Ph.D. from Peking University with Professor Zhen Yang and Chi-Sing Lee in 2010. Then she joined Professor Huanfeng Jiang's group as a postdoctoral researcher at South China University of Technology (SCUT). In 2014, she was promoted to Professor and her research interests include the development and applications of new synthetic methods. |
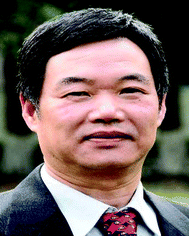 Huanfeng Jiang | Prof. Huanfeng Jiang received his PhD from Shanghai Institute of Organic Chemistry (SIOC) with Professor Xiyan Lu in 1993. Then he joined Guangzhou Institute of Chemistry as a research fellow. In 2003, he moved to the South China University of Technology (SCUT) as the Leading Professor of Chemistry. He received Chinese Chemical Society-BASF Young Investigator's Award in 2002 and National Natural Science Funds for Distinguished Young Scholar in 2006. His research interests focus on synthetic methodology, and green and sustainable chemistry. |
1 Introduction
Organosulfurs are ubiquitous structural frameworks present in a number of natural products, pharmaceutically and biologically active molecules and functional materials,1 as well as for applications in food industries.2 These motifs also play important roles in the field of photoelectric materials because of the sulfur atom's higher resonance energy than other heteroatoms.3 According to the results of a survey in 2014, more than 25% of top 200 U.S. prescription drugs are sulfur-containing pharmaceuticals.4 In the past few years, various novel synthetic strategies have been extensively established for the assembly of structurally diverse functionalized sulfur-containing architectures. Since Migita and co-workers disclosed the first palladium-catalyzed intermolecular coupling of aryl halides with thiols for the construction of structurally complex thioethers,5 transition metal-catalyzed sulfenylation reactions have been proven to be the straightforward and flexible synthetic methodologies for the preparation of valuable polyfunctionalized organosulfur motifs in contemporary sulfur chemistry. In this regard, several transition metal catalysts such as copper,6 cobalt,7 nickel,8 gold,9 rhodium,10 and other metal catalysts11 have been identified as extremely efficient catalysts for this type of chemical transformation. Correspondingly, various practical sulfenylating agents, thiols, disulfides, N-thioarylphthalimides, arylsulfonyl chlorides, and sulfonyl hydrazides have been typically investigated as the sulfur species. However, the above-mentioned sulfenylating reagents have certain disadvantages such as repulsive odor, expensive sulfur sources, air or water instability, and toxicity. From the perspective of environmentally friendly and green chemistry, transition metal-free strategies have been well established for the synthesis of organosulfur skeletons with highly atom- and step-economical manners. Moreover, alternative inorganic sulfenylating reagents such as S8, Na2S, K2S, sodium sulfinates, sodium thiosulfate, and sulfourea, have also been exploited in recent years.12
In addition, a palladium-catalyzed cross-coupling reaction has emerged as the most fascinating and powerful tool for the rapid and straightforward preparation of value-added functionalized molecules.13 However, the palladium catalyst may be poisonous because of the strong coordination of sulfenylating reagents with palladium catalytic species in the chemical transformation process. As a result, palladium-catalyzed sulfenylation transformation has been less reported compared to other metal catalysts. Despite all this, palladium-catalyzed sulfenylation reactions are especially attractive, which can assemble structurally flexible sulfur-containing skeletons. Furthermore, there are several related reviews reported based on transition metal-catalyzed sulfenylation reactions.14 For instance, in 2014, Lee and co-workers reviewed the transition metal-catalyzed C–S bond coupling reaction.15 The transition metal catalysts including palladium, copper, nickel, cobalt, indium, gold, rhodium, iron and others were mainly summarized for the coupling of thiols with aryl halides and pseudo halides. After that, Ghaderi also elucidated the transition metal-catalyzed thioetherification reactions of aromatic compounds in 2016.16 Recently, the application of different sulfenylating reagents for the synthesis of structurally diverse organosulfur frameworks via an eco-friendly approach has also been demonstrated by Jiang and co-workers.17 To the best of our knowledge, no palladium-catalyzed sulfenylation was especially and exclusively reviewed in recent years. Developing efficient and green synthetic methods of palladium-catalyzed coupling reactions for the synthesis of polyfunctionalized fine chemicals is a long-term objective of our group.18 Recently, we have summarized palladium-catalyzed cascade cyclization/alkynylation reactions for the assembly of structurally diverse alkyne derivatives with excellent chemoselectivities.19 Additionally, we also made some important achievements in transition metal-catalyzed coupling reactions for the synthesis of a wide range of organosulfur frameworks.20 In this minireview, we highlight the significant and representative achievements in palladium-catalyzed sulfenylation and sulfonylation from 2010 to the beginning of 2020, including our recent contributions. It is noteworthy that this review will cover some selected examples, which have been recently reviewed elsewhere and will not be discussed in detail.
2 Sulfenylation reactions
2.1 Sulfenylation of aryl/heteroaryl C–H bonds
In the past two decades, transition metal-catalyzed straightforward sulfenylation of unactivated aryl/heteroaryl C–H bonds would be an economic and benign synthetic strategy, allowing direct and concise construction of diversiform sulfur-containing skeletons from the ready availability of starting materials. For instance, Anbarasan and co-workers discovered a palladium-catalyzed aryl(alkyl)thiolation of arenes (1) with electrophilic sulfenylating reagents (2) for the direct construction of diverse unsymmetrical diaryl or arylalkyl sulfides (3) in good yields with highly selectivity (Scheme 1).21 Optimization conditions indicated that 2 mol% Pd(OAc)2 as the catalyst and TFA (trifluoroacetic acid) as the solvent were essential for this protocol. Moreover, under the optimized arylthiolation conditions, various aryl, alkyl, and heteroaryl substituted sulfenylating reagents 2 were confirmed as good coupling partners. Most importantly, using the obtained diaryl sulfides as the synthetic intermediates, dibenzothiophene (4) and benzo[a]phenoxathiine (5) derivatives can be effectively assembled by intramolecular cyclization of the C–H bond (Scheme 1a and b), which can be widely used in materials sciences.22
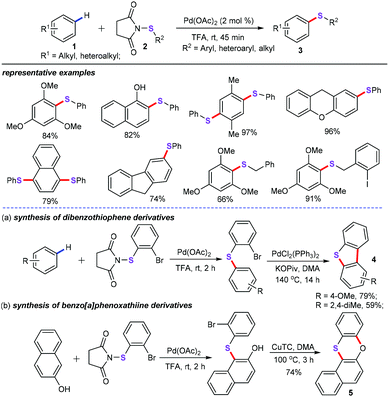 |
| Scheme 1 Palladium-catalyzed aryl(alkyl)thiolation of arenes with N-thioaryl(alkyl)phthalimides. | |
A detailed catalytic mechanism is described in Scheme 2. Initially, the palladium complex reacts with arenes 1 to generate the arylpalladium species Int-1via C–H functionalization.23 Then, oxidative addition of the N–S bond of 2 with intermediate Int-1 produces the PdIV species Int-2. Consequently, reductive elimination of PdIV intermediate leads to the target products 3 and PdII species Int-3. Finally, ligand exchange of Int-3 with TFA gives the active palladium species to complete the catalytic cycle.
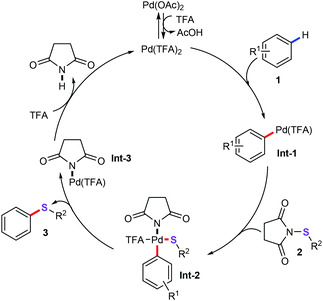 |
| Scheme 2 Plausible mechanism for palladium-catalyzed aryl(alkyl)thiolation. | |
The trifluoromethylthio group represents the privileged structures in pharmaceuticals and biological activities of molecules.24 For this reason, several transition metal-catalyzed practical and atom economical methods have been investigated in detail in the past few years.25 In this respect, Shen and co-workers demonstrated elegant palladium-catalyzed intermolecular highly selective C–H monotrifluoromethylthiolation of arenes (6) for the first time (Scheme 3).26 In the presence of 10 mol% of Pd(CH3CN)4(OTf)2 in AcOH at 110 °C for 24 h, this approach provided a facile and environmentally benign way to access trifluoromethylthiolated products (8) in moderate to good yields with exceptional functional group tolerance. Both internal and external competition KIE studies disclosed that the C–H activation process is not the limiting step for this catalytic cycle. As a result, the plausible mechanism for this chemical transformation was proposed as follows: (i) cyclopalladation of 2-pyridylbenzene (6) with PdII produces palladacycle intermediates Int-4; (ii) oxidative addition of the N-SCF3 bond of 7 affords the trifluoromethylthio-substituted PdIII or PdIV intermediate Int-5; and (iii) reductive-elimination generates the final products.
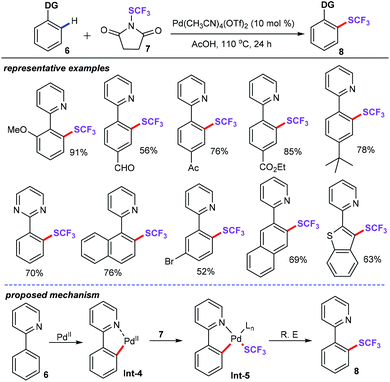 |
| Scheme 3 Palladium-catalyzed trifluoromethylthiolation of aryl C–H bonds. | |
Meanwhile, Nishihara group successfully accomplished a palladium and copper co-catalyzed direct thiolation of aryl C–H bonds with disulfides or thiols (Scheme 4).27 In the presence of 10 mol% of PdCl2(PhCN)2 and 10 mol% of CuCl2 as the co-catalyst, 20 mol% of trimesitylphosphine as the optimized ligand, DMSO as the solvent at 140 °C for 12 h, both the arenes (9) bearing directing groups such as pyridyl, 2-quinolyl, 2-pyrimidyl, and the bidentate 8-aminoquinoline groups and diaryl disulfides (10) proved to be the suitable substrates for preparing highly functionalized aryl sulfides (11) in moderate to excellent yields. However, a lower yield was obtained with aliphatic disulfides, such as dibenzyl disulfide, under the optimized catalytic system. The mechanism studies indicated that the dimeric chloro(2-pyridylphenyl)palladium(II) (12) could participate in oxidative addition of the disulfide to form a palladium(IV) intermediate. As a result, the authors also proposed that this chemical transformation may involve a Pd(II)/Pd(IV) catalytic cycle.
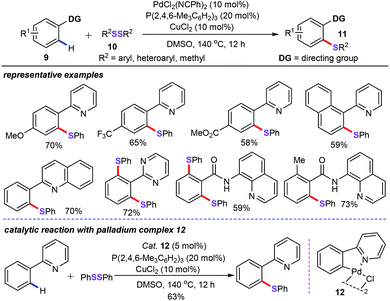 |
| Scheme 4 Palladium and copper co-catalyzed thiolation of aryl C–H bonds with disulfides. | |
Subsequently, Nishihara and co-workers have extended the above-mentioned method to the palladium-catalyzed, picolinamide-directed C–H thiolation of naphthylamine derivatives (13) with diaryl disulfides and diaryl diselenides (14) for the assembly of 8-chalcogen-1-naphthylamines derivatives (15) (Scheme 5).28 Additional investigation of the acid additives revealed that the PivOH was essential for this chemical transformation, while other acids such as AcOH, p-TsOH, CF3CO2H, and CF3SO3H did not improve the yields. From the combination of PdCl2(PhCN)2 (10 mol%) as the catalyst, CuCl2 (10 mol%) as the co-catalyst, and PivOH (1.2 equiv.) as the additives, a wide range of functional groups such as –CF3, –CO2Et, –Cl, –Br, and –NO2 were well tolerated under the reaction conditions. Of note, the directing group picolinamide was readily removable after the chalcogenation. However, unlike the previous ortho-functionalization, this chalcogenation of naphthylamine derivatives exclusively yields peri-position products. The control experiments suggested that this protocol proceeds via a thermodynamically stable five-membered palladacycle intermediate process.
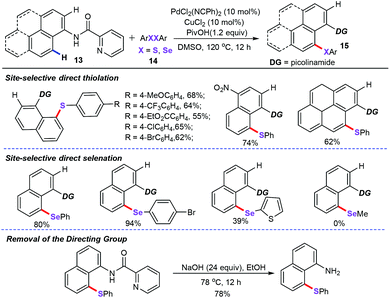 |
| Scheme 5 Palladium and copper co-catalyzed chalcogenation of naphthylamine derivatives. | |
In 2015, Kambe and co-workers described a palladium-catalyzed intermolecular C–H chalcogenation process for the formation of various synthetically useful diaryl or alkyl aryl sulfides and selenides (18) (Scheme 6).29 This chalcogenation process can be performed with 10 mol% Pd(OAc)2 in DMF in the presence of 2 equiv. of CuBr2 as the oxidant under air atmospheric conditions. With a pyrimidyl or pyridyl group as the directing group, the ortho-C–H functionalization of arenes and heteroarenes (16) including carbazole, 2-phenylpyridine, benzoquinoline, and indole derivatives was compatible with the present catalytic system, giving the corresponding products in good yields with excellent regioselectivities. Notably, CuBr2 plays an important role in the conversion of C–Pd bonds into C–S bonds in the presence of disulfides, which may transfer ArS groups to Pd (Int-7) and/or by generating Pd intermediates with a higher oxidation state (Int-8 or Int-9).30
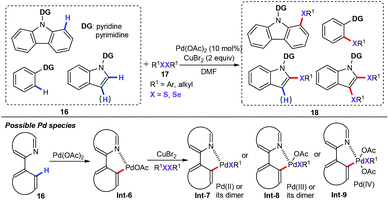 |
| Scheme 6 Palladium-catalyzed intermolecular C–H chalcogenation of arenes and heteroarenes. | |
Different from the usually used thiolation reagent such as disulfides or thiols, Zhang and co-workers used N-arylthiobenzamides (20) as both the thiolation reagent and oxidant for palladium-catalyzed sulfenylation of arenes (19) (Scheme 7).31 It was found that Pd(MeCN)2Cl2 could efficiently catalyze this sulfenylation protocol in the absence of phosphine ligands or any other additives. Under optimized conditions, arenes bearing a direction group were allowed to react with various N-arylthiobenzamides, affording the corresponding aryl thioethers (21) or dithioethers (22) in moderate to good yields. A variety of functional groups, such as cyano, trifluoromethyl, methoxyl, and ester groups were perfectly tolerated. The authors proposed that this approach may involve a Pd(II)/Pd(IV) catalytic cycle process. As shown in Scheme 8, cyclopalladation of 2-phenylpyridine (19) affords the vinyl palladacycle complex Int-10. Afterwards, oxidative addition of palladacycle complex Int-10 with N-(phenylthio)benzamide (19) gives Pd(IV) intermediate Int-11. Reductive elimination followed by another C–H cyclopalladation produces the five-membered palladacycle species Int-12. Finally, subsequent oxidative addition and reductive elimination delivers the desired products 22 and Pd(II) catalytic species.
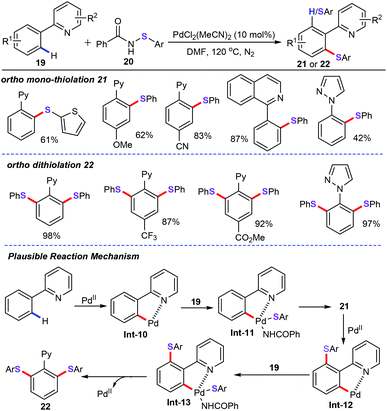 |
| Scheme 7 Palladium-catalyzed sulfenylation using N-arylthiobenzamides as a thiolation reagent. | |
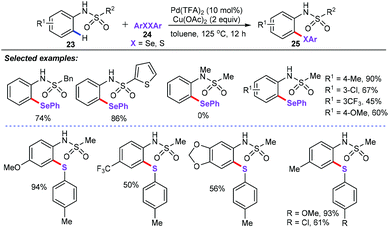 |
| Scheme 8 Palladium-catalyzed chalcogenation reactions of sulfonamides. | |
More importantly, the above-mentioned achievements are accomplished mainly through the strong coordination of N-containing directing groups such as pyrimidine, pyridine, and bidentate directing groups. On the contrary, the directing groups with the weak coordination have been rarely reported. Recently, Ma and co-workers have disclosed the first ligand-free palladium-catalyzed ortho-C–H chalcogenation reactions of sulfonamides (23) via weak coordination (Scheme 8).32 The limitations and scope of the sulfonamide substrates bearing diverse substituents at sulfonyl moiety were then comprehensively investigated. The N-alkylsulfonyl- and N-arylsulfonyl-substituted substrates were good candidates in this chalcogenation reaction. However, tertiary amide was not compatible with this catalytic system. The substrates scope showed that electron-donating substituents of diaryl disulfides (24) gave the best results. In addition, the intermolecular competitions in KIE experiments (kH/kD = 1.1) indicated that the C–H activation is unlikely involved in the rate-limiting step.
From the mechanistic point of view, the six-membered palladacycle intermediate Int-14 was obtained by subsequent coordination of the palladium catalyst to the weakly coordinated O-atom of the sulfonamide, ortho-C–H bond activation, and oxidative addition process. Then, reductive elimination affords the target products 25 and the PdII catalytic species. The authors also proposed another plausible catalytic mechanism. The cyclopalladation of PdII catalytic species with sulfonamides forms palladacycle species Int-15, which underwent reductive elimination to generate the final products 25 and Pd0 species. Finally, the resulting Pd0 is additionally oxidized by external oxidant Cu(OAc)2 to the palladium(II) active species to complete this catalytic cycle (Scheme 9).
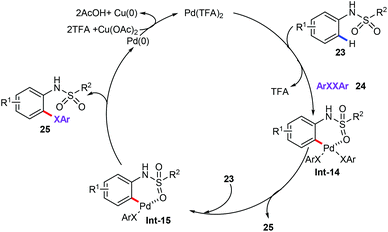 |
| Scheme 9 Proposed mechanism of the palladium-catalyzed chalcogenation reactions of sulfonamides. | |
In particular, Yuan and Xiang group developed an elegant strategy of palladium-catalyzed oxidative arylthiolation of alkanes and ethers (27) with arylsulfonyl hydrazides (26) for the preparation of alkyl aryl sulfides (28) (Scheme 10).33 Using Pd(OAc)2 (2 mol%) as the catalyst and DTBP (di-tert-butyl peroxide, 2 equiv.) as the oxidant, a library of functional groups such as alkyl, alkoxyl, halide, and trifluoromethyl, even five-, six-, or eight-membered rings, were perfectly tolerated under the reaction condition, delivering the desired products 28 in moderate to good yields with excellent regioselectivity. Mechanistically, the oxidant DTBP plays an important role for the formation of the corresponding alkyl radical intermediates by abstract hydrogen from alkanes and ethers.
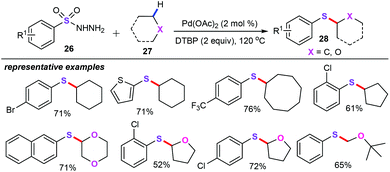 |
| Scheme 10 Palladium-catalyzed radical coupling reaction for the synthesis of alkyl aryl sulfides. | |
In 2018, Jiang and co-workers developed a nice protocol of palladium-catalyzed C–S cyclization for the straightforward synthesis of sulfur-containing benzoheterocyclic motifs (40) from thioacetates (39) (Scheme 11).34 The optimal conditions were obtained in toluene solvent at 120 °C in the presence of 10 mol% Pd(OAc)2, 2.0 equiv. PtBu3·HBF4, and 2.0 equiv. Ag2CO3 in a N2 atmosphere. This transformation allowed for a range of C–H functionalization and carbon–sulfur cyclization cascade reactions, thereby delivering a series of structurally diverse sulfur-containing conjugated heterocyclic molecules in moderate yields. Notably, these obtained benzoheterocyclic derivatives are more useful intermediates, which can allow for the assembly of semiconductor materials such as BTBT (31a), BTT (31b), and BBTT (31c). The kinetic isotope effect (KIE) value of kH/kD 5.3 was obtained under optimal conditions, thus suggesting that the rate-determining step involves the cleavage of the CAr–H bond.
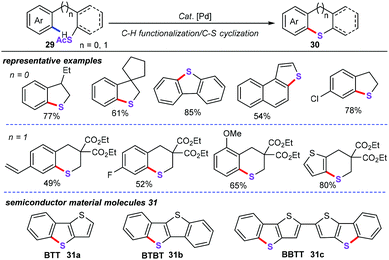 |
| Scheme 11 Palladium-catalyzed C–S cyclization reaction. | |
Based on the above-mentioned results, a plausible pathway of this reaction is described in Scheme 12. The palladium species coordinated to thioacetates to from palladacycle species Int-16. Subsequently, palladium intermediate Int-16 underwent metalation-deprotonation to generate palladium complex Int-17, which was confirmed as the rate-determining step in this chemical transformation. Then, reductive elimination of intermediate Int-17 produces target products 30 and Pd(0) species. Finally, the oxidation of Pd(0) to Pd(II) by silver completes the catalytic cycle.
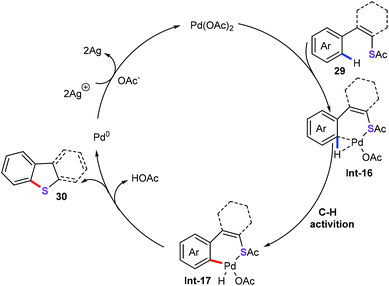 |
| Scheme 12 Proposed mechanism for the palladium-catalyzed C–S cyclization. | |
In recent contributions, our group also successfully accomplished palladium-catalyzed three-component cascade oxidative sulfenylation using elemental sulfur (S8) as a sulfenylation reagent in ionic liquids (Scheme 13).35 The scope of electron-rich heteroarenes (31) as substrates was then evaluated with a variety of electron-rich and electron-deficient substituents even with some sensitive functional groups such as amino groups, ketones, halogens, cyano groups, and esters, which were well accommodated under optimized conditions. As for aryl boronic acids (32), various functional groups such as alkyls, halogens, heteroaryls, and cyano groups were also compatible with these reaction conditions. As a result, this approach provides an effective and green pathway for the synthesis of a range of 3-sulfenylheteroarenes (33) in moderate to excellent yields. Remarkably, the bissulfenylation reaction for the assembly of 3,3′-bis(phenylthio)biindole (35) under optimized conditions was also well performed.
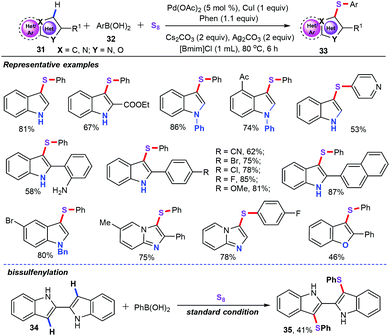 |
| Scheme 13 Palladium-catalyzed oxidative sulfenylation of electron-rich heteroarenes. | |
We then suggested a plausible mechanism for this oxidative sulfenylation (Scheme 14). The first step is the electrophilic palladation of heteroarenes, which generates vinyl-palladium intermediate Int-18.36 Meanwhile, using CuI as the catalyst, aryl boronic acid reacts with S8, affording organocopper thiolate complex Int-19.37 Subsequently, transmetalation of intermediate Int-19 with Int-18 produces vinyl-palladium intermediate Int-20. Finally, reductive elimination of intermediate Int-20 gives the target products 33. Then, Ag2CO3 was used as the oxidant for the regeneration of Pd(II) from Pd(0).
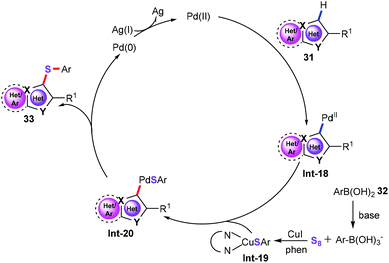 |
| Scheme 14 Plausible mechanism for palladium-catalyzed oxidative sulfenylation. | |
2.2 Sulfenylation of alkynes
Alkynes served as important chemical participants, displaying high reactivity in transition metal-catalyzed sulfenylation. For example, Ananikov and co-workers described a remarkable and highly efficient approach for the preparation of Markovnikov-type vinyl sulfides (39) starting from readily available thiols (37) with alkynes (36) (Scheme 15).38 Air and moisture stable Pd-NHC complex [(IMes)Pd(acac)Cl] (38) can be easily synthesized by the reaction of Pd(acac)2 and the IMes·HCl imidazolium salt. With 1 mol% Pd-NHC complex as the catalyst and 1 equiv. γ-terpinene as the additive and under solvent-free conditions, a variety of thiols including tertiary, secondary, and primary aliphatic thiols, as well as benzylic and aromatic thiols, could be excellently tolerated, furnishing the desired products 39 in moderate to good yields with excellent selectivity. Additionally, this approach did not require additional ligands and solvents, and avoided the use of complicated experimental techniques.
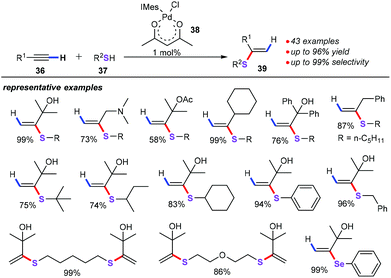 |
| Scheme 15 Palladium-catalyzed regioselective addition of thiols to alkynes. | |
The mechanism was subsequently postulated (Scheme 16). First, the activation of the precatalyst 38 by replacement of the Cl and acac ligands with SR ligands forms monomeric (Int-21) or dimeric (Int-21a) Pd complexes. Particularly noteworthy was that dimeric complexes Int-21a were established by X-ray analysis. Then, three-coordinated monomeric complex activated alkynes to give π complex Int-22, which further undergoes alkyne insertion and affords vinyl palladium species Int-23. Finally, the vinyl palladium complex Int-23 with thiols 37 generates the target products. Notably, the authors also gave some evidence from the DFT calculations for this catalytic mechanism.
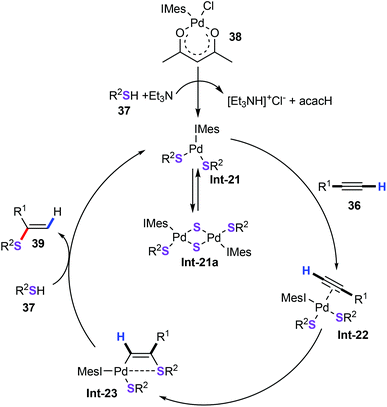 |
| Scheme 16 Plausible mechanism for palladium-catalyzed hydrothiolation of alkynes. | |
In addition, Singh and co-workers developed a palladium-catalyzed sequential Sonogashira coupling and annulation reaction of ortho-alkynyl aldehyde (40) for the construction of various 3-alkylsulfanyl-cyclopenta[b]quinolin-1-ones (42) with sodium sulphide (Na2S) as inorganic sulfur sources (Scheme 17).39 The optimized system was successfully applied to a wide array of ortho-alkynyl aldehyde, including those bearing synthetically useful functional groups such as halogens and aromatic heterocycles. Moreover, the scope of this protocol was further expanded to a variety of alkyl and benzyl halides (41). The authors also showcased the utility of 3-sulfenylated cyclopenta[b]quinolinone by performing a number of elaboration reactions.
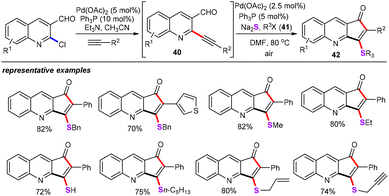 |
| Scheme 17 Palladium-catalyzed sequential Sonogashira coupling and annulation reaction of ortho-alkynyl aldehydes. | |
In 2016, our group disclosed an attractive and concise approach for the synthesis of 3-sulfenylbenzofurans (46) and 3-sulfenylindoles (47) via palladium and copper co-catalyzed cascade annulation/arylthiolation of 2-alkynylphenols (43) or 2-alkynylamines (44) with arylboronic acid (45) and S8 in ionic liquids (Scheme 18).40 This strategy tolerated a broad range of electron-neutral, electron-withdrawing, and electron-donating substrates, thereby furnishing the corresponding sulfenylated heterocycles scaffolds in moderate to excellent yields. Additionally, a variety of conventional solvents (such as DMF, DMSO, CH3CN, and toluene) and different types of ionic liquids were examined, and ionic liquids [Bmim]Cl were found to be the best solvent for this transformation. Other easily available sulfur sources such as Na2S, S8 and K2S, were also screened, and S8 was the most effective inorganic sulfur source. Considering the environmental impacts, ionic liquids as the solvent makes this transformation green and reusable.
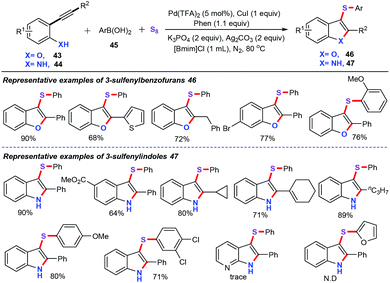 |
| Scheme 18 Palladium-catalyzed cascade annulation/arylthiolation reaction. | |
Based on previous relevant reports,41 and our experimental results, a tentative pathway is proposed in Scheme 19. In this transformation, a Pd complex (Int-24) is formed in situ in ionic liquids.42 The nucleopalladation of 2-alkynylphenols or 2-alkynylamines gives vinyl palladium species Int-25. Then, the transmetalation of organocopper thiolate complex Int-26 with intermediate Int-25 forms aryl palladium complex Int-27. Reductive elimination leads to the formation of the target products. Finally, the resulting palladium(0) is additionally oxidized by silver salts to palladium(II) to complete the catalytic cycle.
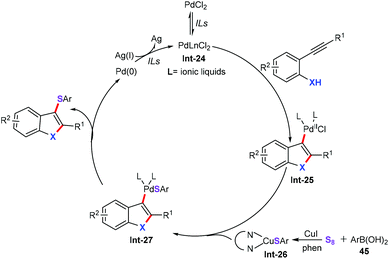 |
| Scheme 19 Plausible mechanism for palladium-catalyzed cascade annulation/arylthiolation reaction. | |
Recently, our group has exploited another green and expeditious synthetic approaches for the assembly of various valuable 4-sulfenylisoxazole derivatives (51) by NHC-palladium-catalyzed three-component cascade S-transfer reaction in ionic liquids (Scheme 20).43 Detailed investigations demonstrated that the best conditions for this chemical transformation are as follows: NHC-Pd(II) (50, 0.25 mol%), and ionic liquid (2 mL) under air at 80 °C for 12 h. Under optimized reaction conditions, both aromatic and aliphatic acetylinic oximes (48) proceeded smoothly in this reaction system, and different functional groups worked well and afforded the desired products 51 in satisfactory yield. However, when aliphatic halide (49) was explored as the substrate, there was no corresponding 4-sulfenylisoxazole product obtained. Moreover, excellent stereoselectivity was observed for one C–O and two C–S bond formation in the present protocol. Particularly, using ionic liquids as the green solvent and stabilizing agents, the palladium catalyst can be efficiently separated from the reaction mixture and recycled. Notably, Na2S2O3 as an odorless sulfenylation reagent under aerobic conditions in ionic liquids also makes this developed approach more eco-friendly.
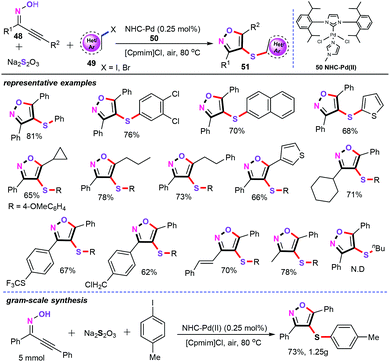 |
| Scheme 20 Palladium-catalyzed three-component cascade S-transfer reaction in ionic liquids. | |
The mechanism of palladium-catalyzed three-component cascade S-transfer reaction in ionic liquids for different 4-sulfenylisoxazoles was proposed (Scheme 21). Initially, trans-oxypalladation of oxime 48 gives σ-isooxazolylpalladium species Int-28 along with losing a HCl molecule.44 Meanwhile, aryl halides reacting with thiosulfate affords thiosulfate salt Int-29. Subsequently, successive ligand exchange/reductive elimination generates the target products 51. Finally, Pd(0) is additionally oxidized by air to Pd(II) species, which completes the catalytic cycle.
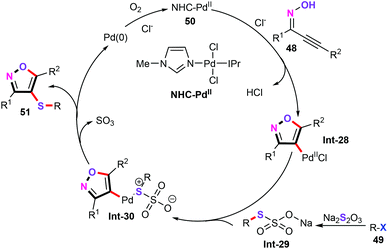 |
| Scheme 21 Plausible mechanism for palladium-catalyzed 4-sulfenylisoxazoles synthesis. | |
In recent years, transition metal-catalyzed difunctionalization of alkynes have attracted considerable attention since they allow the rapid assembly of molecular complexity in an atom- and step-economical manners.45 In this regard, a novel and atom-economical syn-chlorothiolation of terminal alkynes (52) with sulfenyl chlorides (53), furnishing (Z)-2-chloroalkenyl sulfides (54) with high regio- and stereoselective, has been recently developed by Nishihara and co-workers (Scheme 22).46 In this report, functionalized sulfenyl chlorides were treated with various terminal alkynes with catalytic amounts of Pd(TFA)2 (10 mol%) in toluene at 25 °C for 2 h. It is interesting to note that both aliphatic and aromatic alkynes were successfully introduced in this difunctionalization process. Compared with the previous anti-chlorothiolation of terminal alkynes, this discovered protocol offers a complementary synthetic route to chloroalkenyl sulfides. One-pot synthesis of (Z)-2-chloroalkenyl sulfides from benzenethiol with NCS (N-chlorosuccinimide) was also achieved.
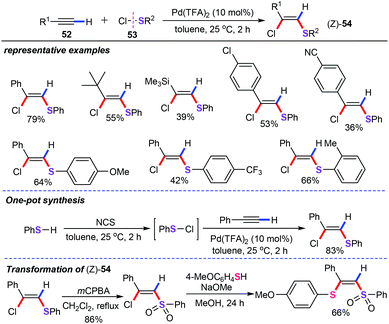 |
| Scheme 22 Palladium-catalyzed selective chlorothiolation of alkynes. | |
After that, the same group described an atom-economical protocol for palladium-catalyzed carbothiolation of azolyl sulfides (55) with terminal alkynes (56) for constructing various 2-(azolyl)alkenyl sulfides (58) with good regio- and stereoselectivities (Scheme 23).47 The optimization of reaction conditions showed that the conventional palladium/phosphine catalytic systems were found to be less active for this chemical transformation, and the palladium catalysts Pd-PEPPSI-IPr (57) and ([1,3-bis(2,6-diisopropylphenyl)imidazol-2ylidene](3-chloropyridine) palladium(II) dichloride) were superior to the others. Further screening of reaction conditions revealed that both nBuLi and MeOH were indispensable for this protocol. Remarkably, this approach could be used for the synthesis of various valuable polysubstituted olefin skeletons in a step- and atom-economical way, which are often found in natural products and materials science. Mechanistically, the selective alkynes insertion of a metal–sulfur bond was considered as the definitive step for the whole catalytic cycle.
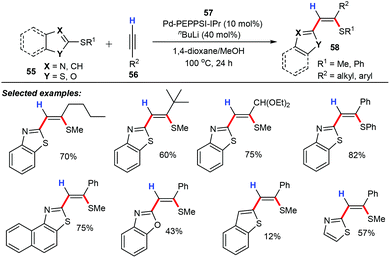 |
| Scheme 23 Palladium-catalyzed carbothiolation of terminal alkynes with azolyl sulfides. | |
Recently, Gulea and co-workers have demonstrated a unique palladium-catalyzed cascade Suzuki-Miyaura coupling/desulfenylative coupling/hydrothiolation of 2-sulfenyl benzimidazoles (59) with boronic acids (60) for the preparation of various substituted benzimidazoles bearing a stereodefined alkenyl sulfide (61) (Scheme 24).48 This cascade protocol was carried out with excess boronic acids (3 equiv.), Pd2(dba)3 (5 mol%), PCy3 (20 mol%), and K2CO3 (3 equiv.) in dioxane/H2O (v
:
v 4/1) at 130 °C for 19 h. In most cases, the yields of the target products ranged between 42% and 82%. However, for alkyne moieties bearing a benzyl group, the reaction gave a lower yield (only 3%). The authors suggested a reaction mechanism that involves classical Suzuki debrominative cross-coupling, followed by desulfenylative coupling and intermolecular regioselective and stereoselective hydrothiolation.
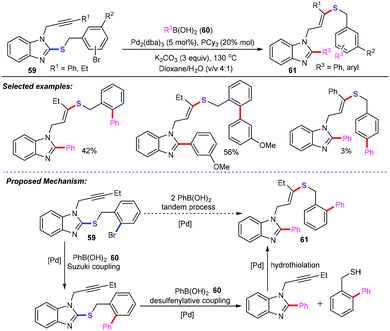 |
| Scheme 24 Palladium-catalyzed cascade approach for the synthesis of substituted benzimidazoles. | |
In parallel to this line, our group accomplished a novel and practical palladium-catalyzed regioselective three-component cascade bisthiolation of terminal alkynes (Scheme 25).49 This reaction was carried out under the following conditions: NHC-Pd (50) (3 mol%), 100 mg 4 Å MS, [C2OHmim]Cl (2 equiv.), and toluene at 80 °C for 12 h. This methodology was successfully used for the synthesis of a variety of functionalized (Z)-1,2-bis(arylthio)alkene derivatives (64) in moderate to good yields with K2S as a stable and odourless sulfur reagent. Under optimized reaction conditions, both aliphatic terminal alkynes and aryl alkynes (62) bearing electron-withdrawing and electron-donating groups were smoothly transformed into the corresponding products with high regioselective. As for various diaryliodonium salts (63), the nature of the anion (such as OTs−, BF4−, and Br−) markedly affected the yields of the investigated substrates. Additionally, when hepta-1,6-diyne (65) was employed to react with diphenyliodonium triflate (63a, 5 equiv.), the desired bisthiolation product 66 was obtained in 79% yield.
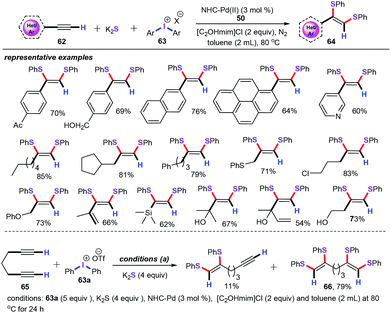 |
| Scheme 25 Palladium-catalyzed three-component cascade bisthiolation. | |
Based on the above-mentioned results and previous reports, the reaction pathway is proposed in Scheme 26. This chemical transformation begins with cis-nucleopalladation of the terminal alkyne with an arylthiolate anion (Int-31) and gives vinylpalladium intermediate Int-32. Then, further oxidative coordination with an arylthiolate anion (Int-31) affords PdIV intermediate Int-33.50 Finally, reductive elimination produces the desired products 64.
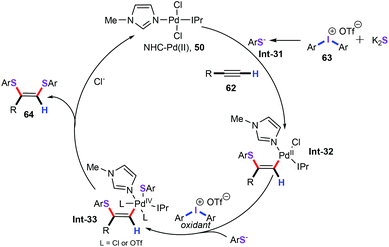 |
| Scheme 26 Plausible mechanism for palladium-catalyzed three-component cascade bisthiolation. | |
2.3. Sulfenylation of aryl halides
In the past two decades, transition metal-catalyzed straightforward sulfenylation of unactivated aryl/heteroaryl C–H bonds would be an economic and benign synthetic strategy, allowing direct and concise construction of diversiform sulfur-containing skeletons from the ready availability of starting materials. For instance, in 2013, Nolan and co-workers reported the well-defined palladium N-heterocyclic carbene (NHC) complex [Pd(IPr*OMe)(cin)Cl] (69)-catalyzed carbon–sulfur bond formation between nonactivated and deactivated aryl halides (67) with aliphatic or aromatic thiols (68) for the synthesis of aryl thioethers (70) (Scheme 27).51 This newly active catalyst showed a remarkable catalytic performance in this sulfenylation reaction. With 0.1 mol% 69 as the catalyst, sterically hindered and deactivated compounds are well-tolerated without any additives or ligands.
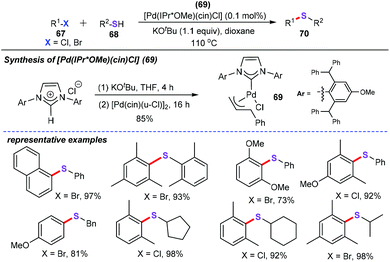 |
| Scheme 27 NHC-Pd-catalyzed sulfenylation of aryl/heteroaryl C–H bonds. | |
Hierso and Roger also presented an efficient and flexible synthetic strategy access to a variety of heteroaryl thioethers through palladium-catalyzed cross-coupling reaction (Scheme 28).52 With the [PdCl(allyl)]2 (1 mol%) as the catalyst, and dppf as the ligand (1,1′-bis[(diphenyl)phosphanyl]-ferrocene), a broad range of electron-rich thiols (71) were allowed to react with 2-chloroheteroaromatics (72), which furnished diverse functionalized heteroaromatic thioethers (73) in good yields. However, electron-deficient groups of thiols such as trifluoromethyl, nitro, and ester groups did not participate in this chemical transformation. It is worth noting that the obtained thioethers could smoothly transform into sulfone derivatives by simple chemical transformation.
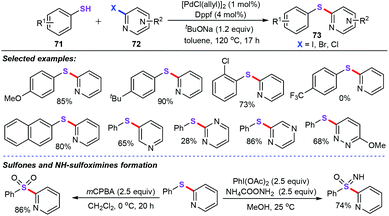 |
| Scheme 28 Palladium-catalyzed thioetherification of thiols and 2-chloroheteroaromatics. | |
Jiang group developed a palladium-catalyzed double C–S bond construction with Na2S2O3 as the sulfurating reagent. It was found that PdCl2(dppf) can efficiently catalyze this approach in the presence of dppf (10 mol%) as the ligand and Cs2CO3 (3 equiv.) as the best base, thus offering diverse 1,4-benzothiazines (75) in moderate to good yields (Scheme 29).53 Substrates (74) bearing Csp3-I, Br, Cl, OMs, and OTs on the saturated carbon could afford good to excellent yields. Additionally, neither S8 nor Na2S as the nontoxic and odourless sulfur source did favor this chemical transformation. In particular, gram-scale synthesis of this method was also achieved, showing potential capabilities to assemble specialized aromatic thioethers in synthetic and pharmaceutical chemistry. More importantly, via modification and synthetic transformation, the obtained 1,4-benzothiazines could be converted into the ion channel antagonistic activity molecule 76 and rufloxacin analogues 77.
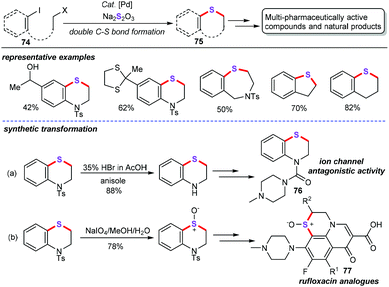 |
| Scheme 29 Palladium-catalyzed double C–S bond formation coupling reaction. | |
In a subsequent study, the double C–S bond formation strategy can be applied to more challenging reaction systems. For example, palladium-catalyzed double C–S bond formation with Na2S2O3 as the sulfurating reagent resulted in a variety of unsymmetrically aromatic thioethers (80) with high regioselectivity, and this was accomplished by the same group (Scheme 30).54 Under optimized conditions, a wide range of arylheterocycle substrates (78) bearing furan, pyridine, thiophene, and pyrazine frameworks were perfectly tolerated. It is remarkable that a variety of halides (79) such as primary halides, secondary halides, even with sterically bulky halides were all amenable with this catalytic system, delivering the corresponding products in good to excellent yields. Furthermore, this protocol provides the potential synthetic application for accurately decorating some biologically active and pharmaceutical molecules.
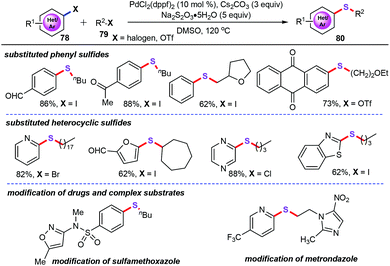 |
| Scheme 30 Palladium-catalyzed cross-coupling access to diverse aromatic sulfides. | |
Afterwards, the above synthetic methodologies were extended to the thiomethylation of aryl chlorides. Jiang group described palladium-catalyzed three-component cascade thiomethylation of aryl chlorides (80), masked inorganic sulfur (KSAc) and dimethyl carbonate (DMC, 81), which afforded a variety of synthetically versatile aryl methyl sulfides (82) in good to excellent yields (Scheme 31).55 In particular, this strategy offers a practical and green method for thiomethylation via the combination of commercially available inorganic sulfur source with an efficient methylation reagent. A gram-scale reaction for the preparation of diverse pesticides was performed under the standard conditions, showing potential capabilities in agriculture and industrial process. Importantly, this approach also provides a rapid and straightforward channel for the assembly of drug and biologically active molecules such as the marketed drug Vismodegib (83) and anti-inflammatory drug Firocoxib (84) through the developed thiomethylation reaction and further derivatizations.
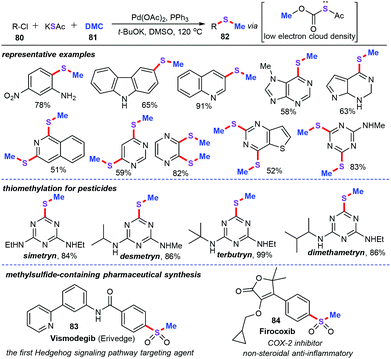 |
| Scheme 31 Palladium-catalyzed three-component thiomethylation reaction. | |
Lee and Kim group reported elegant carbonylation of thioacetates and aryl iodides under a palladium/dppb catalytic system with 8.8 atm pressure of CO (Scheme 32).56 In the presence of 2.5 mol% of Pd2(dba)3 as the catalyst, and 7 mol% of dppb as the ligand, treatment of a variety of aryl iodides with thioacetates at 110 °C for 6 h provided the corresponding S-aryl thioesters in good to excellent yields. Other ligands such as dppp and dppe also displayed the outstanding reactivity. With the Xantphos as the ligand, the sequential coupling of aryl iodides with KSAc followed by the carbonylation also offered the thioesters in good yields. Notably, several functional groups such as methoxyl, formyl, trifluoromethyl, acetyl, nitro, ester, and halogen groups were compatible with the current catalytic system. More importantly, this protocol represented the first deacetylative carbonylation for constructing versatile thioesters.
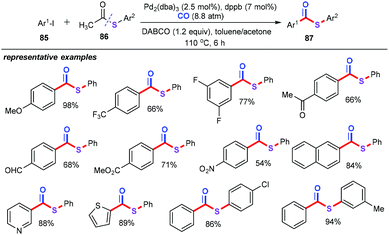 |
| Scheme 32 Palladium-catalyzed carbonylation for the synthesis of thioesters. | |
The reaction would be initiated by oxidative addition generated from Pd(0) with aryl iodides (Scheme 33). Then, migratory insertion of CO into the C–Pd bond of the vinylpalladium intermediate Int-34 forms the acylarylpalladium Int-35. Concomitantly, with the aid of base, thioacetate gives the corresponding thiol.57 Subsequently, ligand exchange between palladium species Int-35 with thiol affords palladium complex Int-36. Finally, the catalytic cycle would be closed by the reductive elimination to generate the expected products 87.
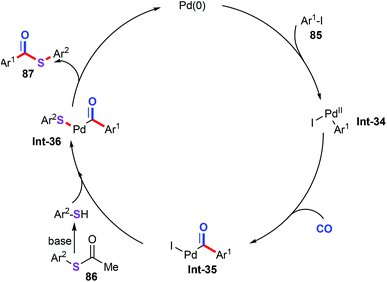 |
| Scheme 33 Plausible mechanism for carbonylation of thioacetates and aryl iodides. | |
As a seminal work, Wu and co-workers developed a new palladium-catalyzed intermolecular transthioetherification reaction of aryl halides (88) with thioethers and thioesters (89) to afford a broad range of the corresponding thiomethylation and carbonylative thiomethylation products (>70 examples) in good to excellent yields with satisfying functional group compatibility (Scheme 34).58 Moreover, this method provided the first example of carbonylative methylthioesterification of aryl halides under the similar reaction conditions. The scope of this system showed that both electron-poor and -rich substrates could be converted into the desired products with alkyl sulfides as the sulfur source. For further applications, some natural products of structural skeletons are also investigated for this transthioetherification reaction.
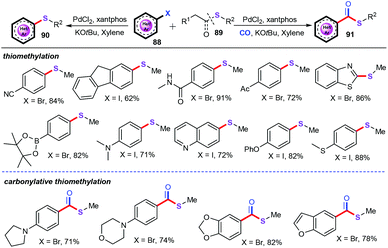 |
| Scheme 34 Palladium-catalyzed transthioetherification reaction. | |
2.4 Other types of sulfenylation reaction
2.4.1 Decarboxylative cross-coupling reactions. Becht and co-workers disclosed the synthesis of diaryl sulfides (94) from the decarboxylative coupling reaction of electron-rich 2,6-dialkoxybenzoic acid derivatives (92) with diaryl disulfides (93) (Scheme 35).59 As shown in Scheme 35, using 15 mol% Pd(CF3CO2)2 as the catalyst, 2.2 equiv. Ag2CO3 as the base, and a mixture of 1,4-dioxane and tetramethylene sulfoxide (TMSO) (v
:
v, 65
:
1) as the solvent at 100 °C for 12 h, the corresponding sulfenylation products 94 were obtained in moderate yields. Additionally, this approach was adequate for the selenation process from arenecarboxylic acid for the first time. Disadvantageously, pentafluorobenzoic acid or 2-thiophenecarboxylic acid were not compatible with the developed conditions.
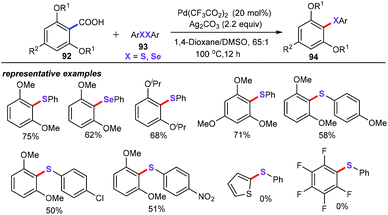 |
| Scheme 35 Palladium-catalyzed decarboxylative cross-coupling reactions. | |
2.4.2 Desulfitative cross-coupling reactions. A later investigation by Jiang and co-workers discovered a palladium-catalyzed ligand-controlled divergent cross-coupling reaction involving an organosilicon reagent (Scheme 36).60 With dppm (bis(diphenylphosphino)methane) as the ligand, the three-component cascade carbonylation of organic thiosulfate salts and organosilicon reagents (96) with carbon monoxide (CO) as a readily available C1 source successfully yielded structurally diverse thioesters (98) in moderate yields. In addition, in the absence of ligands, the Hiyama-type coupling of thiosulfate salts and organosilicon reagents could also react smoothly to deliver the corresponding thioesters (97) in moderate to good yields. Different types of silicon reagents including phenyltriethoxysilane (PhSi(OEt)3), phenyltrimethoxysilane (PhSi(OMe)3), diethoxydiphenylsilane (Ph2Si(OEt)2), and trimethyl(phenyl)silane (PhSiMe3) were also screened, and the results indicated that PhSi(OEt)3 was superior to others. In particular, thiosulfate is odorless, base-free, and compatible with the oxidizing system, showing unique advantages for this type of chemical transformation.
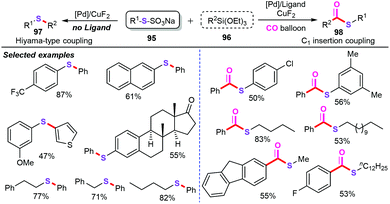 |
| Scheme 36 Palladium-catalyzed ligand-controlled cross-coupling involving an organosilicon reagent. | |
2.4.3 Oxidative dehydrazinative cross-coupling reaction. Zhao and co-workers also discovered a palladium-catalyzed oxidative arylthiolation of arylhydrazines (99) with arenethiols (100) using molecular oxygen as the sole oxidant.61 As shown in Scheme 37, in the presence of 5 mol% of Pd(OAc)2, PCy3 (10 mol%), and Na2CO3 (1 equiv.), in toluene under an O2 balloon at 100 °C for 12 h, a range of unsymmetrical diaryl sulfides (101) were obtained in good to high yields. More importantly, the substrates with different substituents on the phenyl ring such as halogen, trifluoromethoxyl, and nitro group were well tolerated under the optimized reaction conditions. However, 4-nitrobenzenethiol was not compatible with this arylthiolation, and no corresponding product was detected.
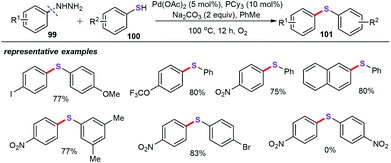 |
| Scheme 37 Palladium-catalyzed oxidative cross-coupling of arylhydrazines with arenethiols. | |
2.4.4 Dehydroxylation cross-coupling reaction. Khakyzadeh and Rostami group designed and synthetized a novel Pd-nanoparticle ([SiO2@organic-linker(OL)@Pd]) catalyst. With the new Pd-nanoparticle and CuI as the co-catalysts, the cross-coupling reaction of unreactive free-phenols (102) with arylboronic acids (103) performed well under optimized conditions (Scheme 38).62 The developed catalyst [SiO2@OL@Pd] showed ultra-high catalytic activity with TONs up to 19
000. Recycling experiments indicated that the palladium nanoparticle catalyst could be recycled up to five times without any decrease in the activity and regioselectivities. Notably, S8 as an odorless sulfur source makes this protocol green and practical.
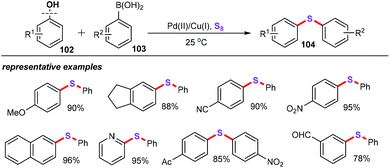 |
| Scheme 38 Pd/Cu-co-catalyzed synthesis of unsymmetrical aryl sulfides. | |
3 Sulfonylation reaction
In recent years, cascade sulfonylation reaction has been identified as the most powerful and practical synthetic methodology for generating new C–S bonds from the corresponding sulfur source.63 In this regard, a number of elegant developments have been typically discovered by transition metal-catalyzed cross-coupling approach. Moreover, many reviews on this topic have already extensively summarized.64 For instance, Willis and co-workers reviewed the development and application of sulfur dioxide surrogates in synthetic organic chemistry in 2015.65 Recently, Luo has also summarized the recent achievements in the synthesis of vinyl sulfones.66 As a result, the cited papers were extensively selected from the latest published works that were not covered in the above reviews. Thus, we confine ourselves to recent improvements and new discoveries in the literature that have not been discussed before.
3.1 Potassium/sodium metabisulfites as SO2 surrogates
In 2015, Shavnya and co-workers developed a novel palladium-catalyzed three-component cascade sulfonylation reaction for the preparation of functionalized aryl alkyl sulfones (107) from unactivated alkyl halides (105), aryl boronic acids (106), and potassium metabisulfite (K2S2O5) (Scheme 39).67 It was found that Pd(MeCN)2Cl2 (10 mol%) could efficiently promote this protocol in the presence of tBuXPhos (10 mol%) as the ligand and TBAB (1.1 equiv.) as the additive in DMF at 85 °C for 22 h, giving the corresponding sulfone derivatives in moderate to good yields. Under the optimized reaction conditions, various activated and unactivated alkyl electrophiles containing primary alkyl bromides, iodides, tosylates, even more hindered electrophiles were nicely tolerated, offering a breadth of aryl alkyl sulfones in good to excellent yields with high step-economy.
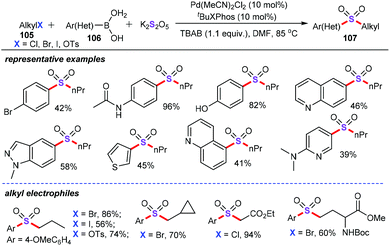 |
| Scheme 39 Palladium-catalyzed three-component cascade sulfonylation reaction. | |
The authors also proposed the mechanism for this sulfonylation reaction as follows (Scheme 40): (i) transmetalation of arylboronic acid with palladium catalyst forms a vinylpalladium intermediate Int-37; (ii) SO2 (generated from K2S2O5)68 insertion into the C–Pd bond produces the palladium sulfinate complex Int-38;69 (iii) alkylation of alkyl halides gives the target alkyl sulfone products 107.
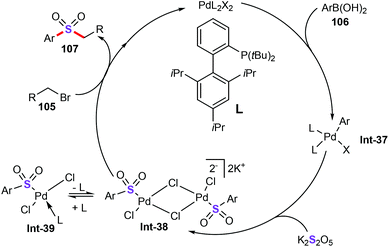 |
| Scheme 40 Plausible mechanism for the three-component cascade sulfonylation reaction. | |
Similarly, Tu and co-workers reported an NHC-Pd-catalyzed alkylsulfonylation of boronic acids (108), (hetero)alkyl halides (109), and potassium metabisulfites for the construction of diverse structurally distinct sulfones (111) (Scheme 41).70 The well-defined NHC-Pd catalyst (110) exhibited the highest activity for this kind of redox-neutral reaction. Remarkably, it was found that the NHC ligand is crucial for this alkylsulfonylation approach. Various substituents on the phenyl ring of arylboronic acids, such as alkyl, trifluoromethoxy, halo, and sulfonyl groups, were well tolerated. The scope of diverse alkyl halides electrophiles such as α-bromoacetates, benzyl bromide and benzyl chloride as substrates was then thoroughly investigated. A detailed mechanistic study revealed that the dimeric palladium complex may be the true active species in this alkylsulfonylation protocol.71
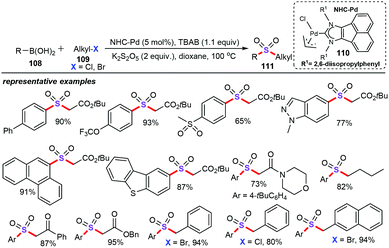 |
| Scheme 41 Palladium-catalyzed alkylsulfonylation of boronic acids with alkyl halides. | |
Recently, Jiang and co-workers have described a novel palladium-catalyzed three-component cascade sulfonylation reaction for the synthesis of synthetically versatile aryl methyl sulfones (114) from boronic acid (112), sodium metabisulfite (Na2S2O5), and dimethyl carbonate (DMC, 113) (Scheme 42).72 As one important sulfur dioxide surrogate, sodium metabisulfite was usually applied as an effective and eco-friendly sulfur dioxide source for this type of cascade sulfonylation reaction.73 In addition, the experimental results indicated that dimethyl carbonate acts as both the methylating reagent and the essential base for this protocol.74 The optimized reaction conditions showed that PdCl2 (10 mol%) can accurately catalyze the three-component cascade sulfonylation reaction in the presence of tBuXPhos (20 mol%) as the ligand, and TBAB (3 equiv.) as the additive in DMF in a N2 atmosphere, affording a broad range of the desired aryl methyl sulfone products in moderate to good yields. Specifically, various fused aromatic rings, such as diphenyl, phenanthryl, naphthyl, and pyrenyl, were successfully accommodated under optimized conditions.
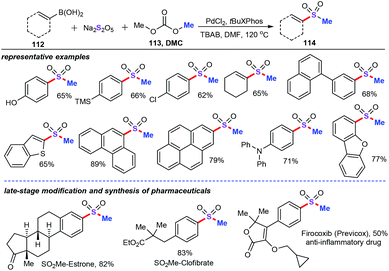 |
| Scheme 42 Palladium-catalyzed three-component cascade sulfonylation reaction. | |
A plausible reaction mechanism was subsequently postulated in Scheme 43. Initially, PdCl2 could coordinate with the ligand forms of palladium species, followed by the transmetalation of aryl boronic acid in the presence of alkoxide anion, producing aryl palladium intermediates Int-40.75 Then, ligand exchange between aryl palladium complex Int-40 with sodium metabisulfite (Na2S2O5) generates intermediate Int-41. Afterwards, with the assistance of the electron-rich ligand, SO2 insertion into the Pd–C bond produces intermediate Int-42. Finally, alkylation process stimulating DMC gives the desired sulfone products 114.
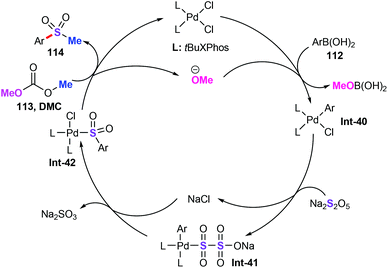 |
| Scheme 43 Plausible mechanism for the three-component sulfonylation reaction. | |
A year later, Jiang group described elegant palladium-catalyzed multicomponent reductive cross-coupling of aryl halides, alkyl halides, and sodium metabisulfite for the straightforward assembly of diversely functionalized sulfone derivatives (Scheme 44).76 In the presence of 10 mol% PdCl2(dppf), 20 mol% dppf, 3 equiv. zinc, 2 equiv. K2HPO4, and 1.5 equiv. TBAB in DMSO conditions, both the intermolecular and intramolecular reductive cross-coupling reactions were successfully explored, and generated a series of sulfones from the corresponding alkyl and aryl halides in good to excellent yields. Moreover, naturally occurring aliphatic compounds, such as steroids, saccharides, and amino acids, were well compatible with this SO2-insertion reductive coupling approach. More importantly, four clinically applied drug molecules were also successfully prepared via alate-stage SO2 insertion process. Mechanistically, control experiment demonstrated that alkyl radicals and sulfonyl radicals were both involved as the important intermediates in this transformation.
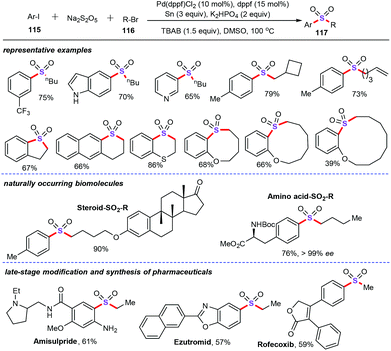 |
| Scheme 44 Palladium-catalyzed multicomponent reductive cross-coupling reaction. | |
3.2 DABSO as the SO2 surrogate
In 1957, Meerwein and co-workers reported that the first example of SO2 gas was applied as the SO2 surrogate for the preparation of aromatic sulfonyl chlorides via a new modification of the Sandmeyer reaction.77 Furthermore, DABSO (DABCO·SO2, the combination of DABCO with sulfur dioxide) as an air-stable and easy handling reagent was first reported in 1988 by Santos and co-workers.78 After that, DABSO as the ideal SO2 surrogate was pioneered by Willis79 and Wu80 in sulfonylation reaction in 2010 and 2012, respectively.
In 2016, Willis and co-workers described a palladium-catalyzed one-pot, two-step, redox-neutral and ligand-free synthetic method for the generation of sulfinate derivatives by employing DABSO as the sulfur dioxide surrogate (Scheme 45).81 Treatment of arylboronic acids (118) with various N-/C-electrophiles in the presence of Pd(OAc)2 (5 mol%) and TBAB (2 equiv.) in 1,4-dioxane/MeOH afforded the corresponding sulfinates (119) in moderate yields. Additionally, this one-pot approach can be applied for the gram scale synthesis. In particular, this sulfonylation reaction is exemplified in the derivatization and elaboration of the sulfinic acid derivatives into the anti-androgen pharmaceutical Casodex.82
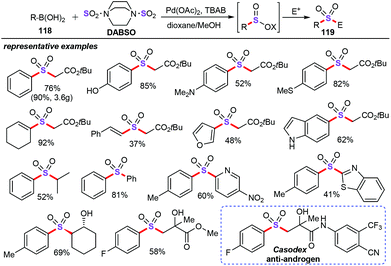 |
| Scheme 45 Palladium-catalyzed synthesis of sulfinates from boronic acids and DABSO. | |
After that, the Willis group accomplished a novel and mild palladium-catalyzed sulfonylation reaction for the construction of sulfonyl fluorides (121) from aryl and heteroaryl bromides (120) with DABSO as the green SO2 source (Scheme 46).83 This protocol provides an efficient and straightforward synthetic strategy for the assembly of structurally diverse heterocyclic or aryl sulfonyl fluorides in moderate yields with high atom- and step-economy and exceptional functional group tolerance. Notably, this transformation allowed rapid construction of sulfonyl fluoride containing pharmaceutical and biologically important molecules.
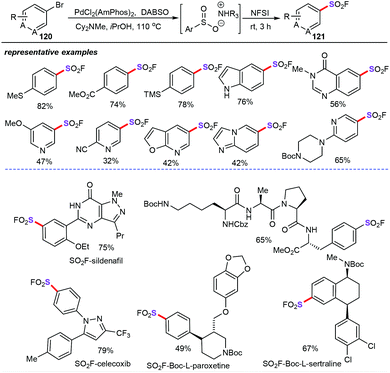 |
| Scheme 46 Palladium-catalyzed sulfonylation reaction for the construction of sulfonyl fluorides. | |
In further investigation of this type of sulfonylation process, Tu group also reported an elegant one-pot bimetallic Pd/Cu-catalyzed three-component cascade sulfonylation reaction (Scheme 47).84 With DABSO as the ideal sulfur source, various substituted arylboronic acids (122) were treated with cyclic and acyclic O-benzoylhydroxylamines (123) using Pd/Cu as the co-catalysts, and a series of structurally diverse sulfonamides (124) were obtained in good to excellent yields without additional ligands. Mechanistically, the authors also performed some control experiments to gain further insights into this synthetic strategy. Moreover, sodium sulfinate has been identified as the crucial intermediate for this transformation.
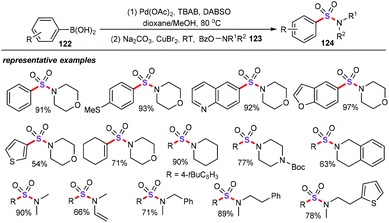 |
| Scheme 47 Pd/Cu co-catalyzed three-component cascade sulfonylation reaction. | |
Especially, Xia and Wu and co-workers also demonstrated a facile and novel palladium-catalyzed direct sulfonylation strategy for the construction of diverse structurally distinct sulfonylative products (127) by C–H bond functionalization (Scheme 48).85 In some cases, dual C–H functionalization products (128) were also detected with a lower yield. Notably, this observation may indicate that this approach presents the good combination of radical chemistry and palladium-catalyzed C–H bond activation.
 |
| Scheme 48 Palladium-catalyzed direct sulfonylation reaction of C–H bonds. | |
3.3 Arylsulfonyl chlorides as the SO2 surrogate
Zhang and Su and co-workers designed a strategy of highly efficient palladium-catalyzed C–H bond activation/functionalization to produce ortho-sulfonylated azobenzenes (131) (Scheme 49).86 Using PdCl2(CH3CN)2 (10 mol%) as the catalyst, and K2CO3 (2 equiv.) as the base, azobenzene substrates (129) were successfully coupled with a whole variety of arylsulfonyl chlorides (130) to furnish a diverse range of unsymmetrical diarylsulfones in good to excellent yields with highly regioselectivities. Because of the mild reaction conditions, some sensitive functional groups such as nitro, halogen, methoxyl, and ester groups were compatible. On the basis of their mechanistic studies, the authors argued that this approach may occur through a cyclopalladated intermediate transition states by the ortho-C–H bond insertion.87
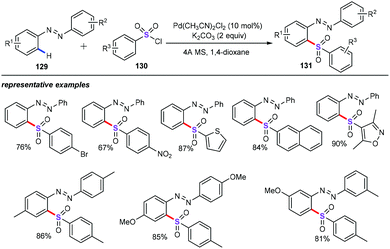 |
| Scheme 49 Palladium-catalyzed C–H bond activation/sulfonylation reaction. | |
3.4 Sodium dithionite as the SO2 surrogate
In comparison to the usually used SO2 surrogate such as potassium/sodium metabisulfite, DABSO, and arylsulfonyl chlorides, sodium dithionite as the alternative SO2 surrogate has rarely reported in recent years. In 2019, Jiang and co-workers discovered a nice and fascinating protocol for the synthesis of alkyl–alkyl and aryl–alkyl sulfones derivatives with a facile combination of halides (132), SO2 surrogates, and phosphate esters (133) (Scheme 50).88 Amazingly, under transition metal-free conditions, alkyl–alkyl sulfones (136) were obtained in moderate to good yields with the thiourea dioxide (135) as a reductive SO2 surrogate. Additionally, aryl–alkyl sulfones (137) were achieved by an extremely low Pd catalyst loading using sodium dithionite (134) as the SO2 surrogate. Specifically, the phosphate esters were employed as the stable and readily available alkyl source in this chemical transformation. Notably, this protocol has been applied to the late-stage modification of natural products and bioactive molecules.
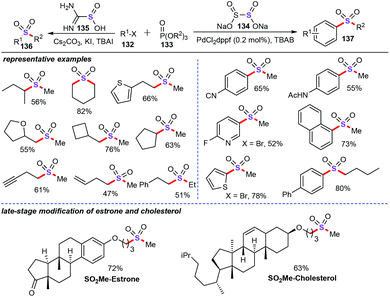 |
| Scheme 50 General sulfone construction via SO2 surrogate control. | |
In the beginning of 2020, Jiang and colleagues also successfully accomplished elegant multicomponent decarboxylative cross coupling of esters (138), sodium dithionite (134), and electrophiles for the preparation of sterically bulky sulfone derivatives (138) (Scheme 51).89 Notably, the inorganic salt sodium dithionite not only served as the SO2 source, but also acted as an efficient radical initiator for the decarboxylation process. Under the optimized conditions, various naturally abundant carboxylic acids and synthetically prepared carboxyl-containing drugs underwent efficient decarboxylative sulfonylation for the construction of diversely functionalized tertiary sulfones. Mechanistic studies suggested that decarboxylation was the rate-determining step and occurred via a single-electron transfer (SET) process with the assistance of sodium dithionite.
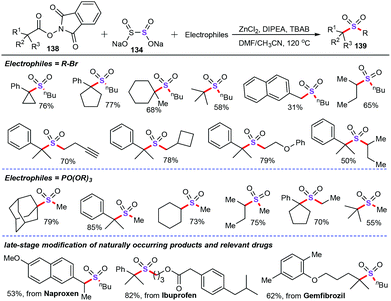 |
| Scheme 51 Sodium dithionite-mediated decarboxylative sulfonylation. | |
4 Summary
In this review, a good many of palladium-catalyzed sulfenylation and sulfonylation reactions have been summarized from 2010 to the beginning of 2020. Although many achievements have been made in C–H, C–C, as well as C–N bond functionalization, the widely existing C–S bond formation reactions were less reported and still highly desirable. In general, there are two main reasons: (i) many sulfur-containing reagents are toxic and unstable, with repulsive odor and (ii) catalyst poisoning owing to the strong coordination of sulfur atom with transition metals catalytic species. Despite all these, numerous chemists have addressed themselves to the development of novel and useful synthetic strategies for constructing new and valuable C–S bonds. Moreover, a series of unique synthetic methodologies have been well developed for the assembly of diverse structurally sulfur-containing skeletons with excellent regio- and stereo-selectivities. As a result, various organic sulfur sources including thiols, disulfides, arylsulfonyl chlorides, sulfonyl hydrazides, and N-thioarylphthalimides have been typically investigated. In addition, several green and readily available inorganic sulfur reagents such as elemental sulfur (S8), sodium sulfide (Na2S), potassium sulfide (K2S), sodium sulfinates (NaSO2R), potassium thioacetate (KSAc), sodium metabisulfite (Na2S2O5), potassium metabisulfite (K2S2O5), and sodium thiosulfate (Na2S2O3) have also been applied in the recent years. Most notably, some of the described methods in this review manifested that the above-mentioned palladium-catalyzed sulfenylation and sulfonylation reactions could provide efficient and rapid approaches for the assembly of complex pharmaceutical and biologically active molecules through further synthetic transformation and structural modification. In brief, this study on the palladium-catalyzed C–S bond formation reaction is now established as a captivating branch for transition metal-catalyzed C–S bond transformation.
Despite the tremendous achievements that have been made in this line, more challenges need to be overcome in this area. For instance, new-type, safe, inexpensive, nontoxic, and readily available sulfur reagents still need to be exploited. Additionally, the “inexpensive” and recyclable metal catalysts such as Fe, Zn, and Al were also explored. In particular, more eco-friendly conditions such as electrochemistry, photochemistry, and free radical chemistry protocols need to be explored in future research. As the old saying goes, “nothing is impossible to a willing heart”, we profoundly believe that palladium-catalyzed C–S bond formation is continuously expanding. We hope this review will give the researchers a new perspective in this area.
Conflicts of interest
The authors declare no competing financial interest.
Acknowledgements
We thank the Ministry of Science and Technology of the People's Republic of China (2016YFA0602900), the National Natural Science Foundation of China (21971072 and 21672072), the Fundamental Research Funds for the Central Universities (2018ZD16), the Pearl River S&T Nova Program of Guangzhou (201906010072), Guangdong Provincial Training Program of Innovation and Entrepreneurship for Undergraduates (S201910561219), and the Open Fund of Guangdong Provincial Key Laboratory of Luminescence from Molecular Aggregates (2019B030301003) for financial support.
References
- For selected reviews, see.
(a) Organic Sulfur Chemistry, Theoretical and Experimental Advances, ed. F. Bernardi, I. G. Csizmadia and A. Mangini, Elsevier, Amsterdam, The Netherlands, 1985, vol. 19 Search PubMed;
(b) K. C. Nicolaou, C. R. H. Hale, C. Nilewski and H. A. Ioannidou, Constructing molecular complexity and diversity: total synthesis of natural products of biological and medicinal importance, Chem. Soc. Rev., 2012, 41, 5185–5238 RSC;
(c) M. Feng, B. Tang, S. H. Liang and X. Jiang, Sulfur containing scaffolds in drugs: synthesis and application in medicinal chemistry, Curr. Top. Med. Chem., 2016, 16, 1200–1216 CrossRef CAS PubMed.
-
(a) D. Y. Lin, S. Z. Zhang, E. Block and L. C. Katz, Encoding social signals in the mouse main olfactory bulb, Nature, 2005, 434, 470–477 CrossRef CAS PubMed;
(b) C. E. Sansom, V. S. Jones, N. I. Joyce, B. M. Smallfield, N. B. Perry and J. W. van Klink, Flavor, glucosinolates, and isothiocyanates of nau (cook's scurvy grass, lepidium oleraceum) and other rare New Zealand lepidium species, J. Agric. Food Chem., 2015, 63, 1833–1838 CrossRef CAS PubMed.
- M. E. Cinar and T. Ozturk, Thienothiophenes, dithienothiophenes, and thienoacenes: syntheses, oligomers, polymers, and properties, Chem. Rev., 2015, 115, 3036–3140 CrossRef CAS PubMed.
- E. A. Ilardi, E. Vitaku and J. T. Njardarson, Data-mining for sulfur and fluorine: an evaluation of pharmaceuticals to reveal opportunities for drug design and discovery, J. Med. Chem., 2014, 57, 2832–2842 CrossRef CAS PubMed.
- T. Migita, T. Shimizu, Y. Asami, J. Shiobara, Y. Kato and M. Kosugi, The palladium catalyzed nucleophilic substitution of aryl halides by thiolate anions, Bull. Chem. Soc. Jpn., 1980, 53, 1385–1389 CrossRef CAS.
- For selected examples, see.
(a) C. Ravi, N. N. K. Reddy, V. Pappula, S. Samanta and S. Adimurthy, Copper-catalyzed three-component system for arylsulfenylation of imidazopyridines with elemental sulfur, J. Org. Chem., 2016, 81, 9964–9972 CrossRef CAS PubMed;
(b) X. Xiao, M. Feng and X. Jiang, New design of a disulfurating reagent: facile and straightforward pathway to unsymmetrical disulfanes by copper-catalyzed oxidative cross-coupling, Angew. Chem., Int. Ed., 2016, 55, 14121–14125 CrossRef CAS PubMed;
(c) M. Wang, J. Wei, Q. Fan and X. Jiang, Cu(II)-catalyzed sulfide construction: both aryl groups utilization of intermolecular and intramolecular diaryliodonium salt, Chem. Commun., 2017, 53, 2918–2921 RSC;
(d) F. Liu and W. Yi, A thiol-free synthesis of alkynyl chalcogenides by the copper-catalyzed C–X (X=S, Se) cross-coupling of alkynyl carboxylic acids with Bunte salts, Org. Chem. Front., 2018, 5, 428–433 RSC;
(e) Q. Yu, Y. Yang, J. Wan and Y. Liu, Copper-catalyzed C5-H sulfenylation of unprotected 8-aminoquinolines using sulfonyl hydrazides, J. Org. Chem., 2018, 83, 11385–11391 CrossRef CAS PubMed.
- T. Gensch, F. J. R. Klauck and F. Glorius, Cobalt-catalyzed C-H thiolation through dehydrogenative cross-coupling, Angew. Chem., Int. Ed., 2016, 55, 11287–11291 CrossRef CAS PubMed.
- For selected examples, see.
(a) F. Gao, W. Zhu, D.-Y. Zhang, S.-J. Li, J. Wang and H. Liu, Nickel-catalyzed ortho-C-H thiolation of N-benzoyl α-amino acid derivatives, J. Org. Chem., 2016, 81, 9122–9130 CrossRef CAS PubMed;
(b) J.-M. Li, Y. Yu, J. Weng and G. Lu, Nickel-catalyzed direct C-H bond sulfenylation of acylhydrazines, Org. Biomol. Chem., 2018, 16, 6047–6056 RSC;
(c) K. Yang, Y. Wang, X.-Y. Chen, A. A. Kadi, H.-K. Fun, H. Sun, Y. Zhang and H.-J. Lu, Nickel-catalyzed and benzoic acid-promoted direct sulfenylation of unactivated arenes, Chem. Commun., 2015, 51, 3582–3585 RSC;
(d) X. Ye, J. L. Petersen and X. Shi, Nickel-catalyzed
directed sulfenylation of sp2 and sp3 C-H bonds, Chem. Commun., 2015, 51, 7863–7866 RSC.
- For selected examples, see:
(a) N. Morita and N. Krause, The first gold-catalyzed C-S bond formation: cycloisomerization of α-thioallenes to 2,5-dihydrothiophenes, Angew. Chem., Int. Ed., 2006, 45, 1897–1899 CrossRef CAS PubMed;
(b) S. Biswas and J. S. M. Samec, A gold-catalyzed route to α-sulfenylated carbonyl compounds from propargylic alcohols and aryl thiols, Chem. Commun., 2012, 48, 6586–6588 RSC;
(c) S. Biswas, R. A. Watile and J. S. M. Samec, Tandem Pd/Au-catalyzed route to α-sulfenylated carbonyl compounds from terminal propargylic alcohols and thiols, Chem. – Eur. J., 2014, 20, 2159–2163 CrossRef CAS PubMed;
(d) Y. Xi, B. Dong, E. J. McClain, Q. Wang, T. L. Gregg, N. G. Akhmedov, J. L. Petersen and X. Shi, Gold-catalyzed intermolecular C-S bond formation: efficient synthesis of α-substituted vinyl sulfones, Angew. Chem., Int. Ed., 2014, 53, 4657–4661 CrossRef CAS PubMed.
-
(a) Y. Yang, W. Hou, L.-H. Qin, J.-J. Du, H.-J. Feng, B. Zhou and Y.-C. Li, Rhodium-catalyzed directed sulfenylation of arene C-H bonds, Chem. – Eur. J., 2014, 20, 416–420 CrossRef CAS PubMed;
(b) J. Wen, A. Wu, M. Wang and J. Zhu, Rh(III)-catalyzed C7-thiolation and selenation of indolines, J. Org. Chem., 2015, 81, 396–403 Search PubMed;
(c) S. Maity, U. Karmakar and R. Samanta, Regiocontrolled direct C4 and C2-methyl thiolation of indoles under rhodium-catalyzed mild conditions, Chem. Commun., 2017, 53, 12197–12200 RSC.
- For iron catalysts, see.
(a) C. Ji, M. Ahmida, M. Chahma and A. Houmam, Radical/ion pair formation in the electrochemical reduction of arene sulfenyl chlorides, J. Am. Chem. Soc., 2006, 128, 15423–15431 CrossRef CAS PubMed;
(b) A. Correa, M. Carril and C. Bolm, Iron-catalyzed S-arylation of thiols with aryl iodides, Angew. Chem., Int. Ed., 2008, 47, 2880–2883 CrossRef CAS PubMed;
(c) Y.-Y. Lin, Y.-J. Wang, C.-H. Lin, J.-H. Cheng and C.-F. Lee, Synthesis of alkenyl sulfides through the iron-catalyzed cross-coupling reaction of vinyl halides with thiols, J. Org. Chem., 2012, 77, 6100–6106 CrossRef CAS PubMed;
(d) M. Iwasaki, T. Fujii, K. Nakajima and Y. Nishihara, Iron-induced regio- and stereoselective addition of sulfenyl chlorides to alkynes by a radical pathway, Angew. Chem., Int. Ed., 2014, 53, 13880–13884 CrossRef CAS PubMed. For ruthenium catalyst;
(e) A. Mandal, S. Dana, H. Sahoo, G. S. Grandhi and M. Baidya, Ruthenium(II)-catalyzed ortho-C-H chalcogenation of benzoic acids via weak O-coordination: synthesis of chalcogenoxanthones, Org. Lett., 2017, 19, 2430–2433 CrossRef CAS PubMed;
(f) W. Ma, Z.-Y. Weng, T. Rogge, L.-H. Gu, J.-F. Lin, A. Peng, X. Luo, X.-J. Gou and L. Ackermannc, Ruthenium(II)-catalyzed C-H chalcogenation of anilides, Adv. Synth. Catal., 2018, 360, 704–710 CrossRef CAS. For vanadium catalyst;
(g) Y. Maeda, M. Koyabu, T. Nishimura and S. Uemura, Vanadium-catalyzed sulfenylation of indoles and 2-naphthols with thiols under molecularoxygen, J. Org. Chem., 2004, 69, 7688–7693 CrossRef CAS PubMed. For magnesium catalyst;
(h) M. Tudge, M. Tamiya, C. Savarin and G. R. Humphrey, Development of a novel, highly efficient halide-catalyzed sulfenylation of indoles, Org. Lett., 2006, 8, 565–568 CrossRef CAS PubMed; For boron catalyst;
(i) L. Zhong, P. R. Savoie, A. S. Filatov and J. T. Welch, Preparation and characterization of alkenyl aryl tetrafluoro-λ6-sulfanes, Angew. Chem., Int. Ed., 2014, 53, 526–529 CrossRef CAS PubMed.
-
(a) H. Liu and X. Jiang, Transfer of sulfur: from simple to diverse, Chem. – Asian J., 2013, 8, 2546–2563 CrossRef CAS PubMed;
(b) W. Guo, K. Tao, W. Tan, M. Zhao, L. Zheng and X. Fan, Recent advances in photocatalytic C-S/P-S bond formation via the generation of sulfur centered radicals and functionalization, Org. Chem. Front., 2019, 6, 2048–2066 RSC.
-
(a) J. Tsuji, Palladium Reagents and Catalysts, John Wiley & Sons, Chichester, UK, 2nd edn, 2004, pp. 201–265 CrossRef;
(b) L.-N. Guo, X.-H. Duan and Y.-M. Liang, Palladium-catalyzed cyclization of propargylic compounds, Acc. Chem. Res., 2011, 44, 111–122 CrossRef CAS PubMed;
(c) X.-F. Wu, H. Neumann and M. Beller, Update 1 of: macrolactonizations in the total synthesis of natural products, Chem. Rev., 2013, 113, 1–35 CrossRef CAS PubMed;
(d) J. Ye and S. Ma, Palladium-catalyzed cyclization reactions of allenes in the presence of unsaturated carbon-carbon bonds, Acc. Chem. Res., 2014, 47, 989–1000 CrossRef CAS PubMed;
(e) G. Yin, X. Mu and G. Liu, Palladium(II)-catalyzed oxidative difunctionalization of alkenes: bond forming at a high-valent palladium center, Acc. Chem. Res., 2016, 49, 2413–2423 CrossRef CAS PubMed;
(f) A. Defert and D. B. Werz, Carbopalladation cascades using carbon-carbon triple bonds: recent advances to access complex scaffolds, Chem. – Eur. J., 2016, 22, 16718–16732 CrossRef PubMed;
(g) D. Wang, A. B. Weinstein, P. B. White and S. S. Stahl, Ligand-promoted palladium-catalyzed aerobic oxidation reactions, Chem. Rev., 2018, 118, 2636–2679 CrossRef CAS PubMed.
-
(a) I. P. Beletskaya and V. P. Ananikov, Transition-metal-catalyzed C-S, C-Se, and C-Te bond formation via cross-coupling and atom-economic addition reactions, Chem. Rev., 2011, 111, 1596–1636 CrossRef CAS PubMed;
(b) T. Kittikool and S. Yotphan, Metal-free direct C-H thiolation and thiocyanation of pyrazolones, Eur. J. Org. Chem., 2020, 961–970 CrossRef CAS.
- C.-F. Lee, Y.-C. Liu and S. S. Badsara, Transition-metal-catalyzed C-S bond coupling reaction, Chem. – Asian J., 2014, 9, 706–722 CrossRef CAS PubMed.
- A. Ghaderi, Advances in transition-metal catalyzed thioetherification reactions of aromatic compounds, Tetrahedron, 2016, 72, 4758–4782 CrossRef CAS.
-
(a) Z. Qiao and X. Jiang, Recent developments in sulfur-carbon bond formation reaction involving thiosulfates, Org. Biomol. Chem., 2017, 15, 1942–1946 RSC;
(b) M. Wang and X. Jiang, Sulfur-sulfur bond construction, Top. Curr. Chem., 2018, 376, 14 CrossRef PubMed;
(c) N. Wang, P. Saidhareddy and X. Jiang, Construction of sulfur-containing moieties in the total synthesis of natural products, Nat. Prod. Rep., 2020, 37, 246–275 RSC;
(d) M. Wang, Y. Li and X. Jiang, Recent advances in sulfuration chemistry enabled by bunte salts, Aldrichimica Acta, 2020, 53, 19–25 Search PubMed.
- For selected reviews, see:
(a) W. Wu and H. Jiang, Haloalkynes: a powerful and versatile building block in organic synthesis, Acc. Chem. Res., 2014, 47, 2483–2504 CrossRef CAS PubMed;
(b) J. Li, S. Yang, W. Wu and H. Jiang, Recent Advances in Pd-catalyzed cross-coupling reaction in ionic liquids, Eur. J. Org. Chem., 2018, 1284–1306 CrossRef CAS;
(c) J. Li and H. Jiang, in Noble Metal Noble Value: Ru-, Rh-, Pd-catalyzed Heterocycle Synthesis, ed. X. Wu, World Scientific Publishing Co Pte Ltd., Singapore, 2016, p. 351. For selected examples, see: Search PubMed;
(d) L. Huang, Q. Wang, X. Liu and H. Jiang, Switch of selectivity in the synthesis of α-methylene-γ-lactones: palladium-catalyzed intermolecular carboesterification of alkenes with alkynes, Angew. Chem., Int. Ed., 2012, 51, 5696–5700 CrossRef CAS PubMed;
(e) J. Li, W. Yang, S. Yang, L. Huang, W. Wu, Y. Sun and H. Jiang, Palladium-catalyzed cascade annulation to construct functionalized β- and γ-lactones in ionic liquids, Angew. Chem., Int.
Ed., 2014, 53, 7219–7222 CrossRef CAS PubMed;
(f) L. Ouyang, J. Li, J. Zheng, J. Huang, C. Qi, W. Wu and H. Jiang, Access to α-amino acid esters through palladium-catalyzed oxidative amination of vinyl ethers with hydrogen peroxide as the oxidant and oxygen source, Angew. Chem., Int. Ed., 2017, 56, 15926–15930 CrossRef CAS PubMed.
- J. Li, S. Yang, W. Wu and H. Jiang, Palladium-catalyzed cascade cyclization/alkynylation reactions, Chem. – Asian J., 2019, 14, 4114–4128 CrossRef CAS PubMed.
- For selected examples, see:
(a) X. Tang, L. Huang, Y. Xu, J. Yang, W. Wu and H. Jiang, Copper-catalyzed coupling of oxime acetates with sodium sulfinates: an efficient synthesis of sulfone derivatives, Angew. Chem., Int. Ed., 2014, 53, 4205–4208 CrossRef CAS PubMed;
(b) C. Li, J. Li, C. Tan, W. Wu and H. Jiang, DDQ-mediated regioselective C–S bond formation: efficient access to allylic sulphides, Org. Chem. Front., 2018, 5, 3158–3162 RSC;
(c) Y. Huang, X. Li, X. Wang, Y. Yu, W. Wu and H. Jiang, Copper-catalyzed cyanothiolation to incorporate a sulfur-substituted quaternary carbon center, Chem. Sci., 2017, 8, 7047–7051 RSC;
(d) W. Wu, Y. An, J. Li, S. Yang, Z. Zhu and H. Jiang, Iodine-catalyzed cascade annulation of alkynes with sodium arylsulfinates: assembly of 3-sulfenylcoumarin and 3-sulfenylquinolinone derivatives, Org. Chem. Front., 2017, 4, 1751–1756 RSC.
- P. Saravanan and P. Anbarasan, Palladium catalyzed aryl(alkyl)thiolation of unactivated arenes, Org. Lett., 2014, 16, 848–851 CrossRef CAS PubMed.
- L. Wang, W. He and Z. Yu, Transition-metal mediated carbon–sulfur bond activation and transformations, Chem. Soc. Rev., 2013, 42, 599–621 RSC.
- V. Ritleng, C. Sirlin and M. Pfeffer, Ru-, Rh-, and Pd-catalyzed C-C bond formation involving C-H activation and addition on unsaturated substrates: reactions and mechanistic aspects, Chem. Rev., 2002, 102, 1731–1770 CrossRef CAS PubMed.
- C. Hansch, A. Leo and R. W. Taft, A survey of Hammett substituent constants and resonance and field parameters, Chem. Rev., 1991, 91, 165–195 CrossRef CAS.
-
(a) N. D. Litvinas, P. S. Fier and J. F. Hartwig, A general strategy for the perfluoroalkylation of arenes and arylbromides by using arylboronate esters and [(phen)CuRF]†, Angew. Chem., Int. Ed., 2012, 51, 536–539 CrossRef CAS PubMed;
(b) T. Liu, X. Shao, Y. Wu and Q. Shen, Highly selective trifluoromethylation of 1,3-disubstituted arenes through iridium-catalyzed arene borylation, Angew. Chem., Int. Ed., 2012, 51, 540–543 CrossRef CAS PubMed.
- C. Xu and Q. Shen, Palladium-catalyzed trifluoromethylthiolation of aryl C-H bonds, Org. Lett., 2014, 16, 2046–2049 CrossRef CAS PubMed.
- M. Iwasaki, M. Iyanaga, Y. Tsuchiya, Y. Nishimura, W. Li, Z. Li and Y. Nishihara, Palladium-catalyzed direct thiolation of aryl C-H bonds with disulfides, Chem. – Eur. J., 2014, 20, 2459–2462 CrossRef CAS PubMed.
- M. Iwasaki, W. Kaneshika, Y. Tsuchiya, K. Nakajima and Y. Nishihara, Palladium-catalyzed peri-selective chalcogenation of naphthylamines with diaryl disulfides and diselenides via C-H bond cleavage, J. Org. Chem., 2014, 79, 11330–11338 CrossRef CAS PubMed.
- R. Qiu, V. P. Reddy, T. Iwasaki and N. Kambe, The palladium-catalyzed intermolecular C-H chalcogenation of arenes, J. Org. Chem., 2015, 80, 367–374 CrossRef CAS PubMed.
- D. C. Powers, E. Lee, A. Ariafard, M. S. Sanford, B. F. Yates, A. J. Canty and T. Ritter, Connecting binuclear Pd(III) and mononuclear Pd(IV) chemistry by Pd-Pd bond cleavage, J. Am. Chem. Soc., 2012, 134, 12002–12009 CrossRef CAS PubMed.
- X.-S. Zhang, G. Li, X.-G. Zhang and X.-H. Zhang, Pd(II)-catalyzed selective sulfenylation of arene C–H bonds using N-arylthiobenzamides as thiolation reagent and oxidant, Tetrahedron, 2015, 71, 5458–5464 CrossRef CAS.
- L. Gu, X. Fang, Z. Weng, Y. Song and W. Ma, Ligand-free palladium(II)-catalyzed ortho-C-H chalcogenations of N-arylsulfonamide via weak coordination, Eur. J. Org. Chem., 2019, 1825–1829 CrossRef CAS.
- S.-R. Guo, W.-M. He, J.-N. Xiang and Y.-Q. Yuan, Palladium-catalyzed thiolation of alkanes and ethers with arylsulfonyl hydrazides, Chem. Commun., 2014, 50, 8578–8581 RSC.
- S. Chen, M. Wang and X. Jiang, Pd-catalyzed C-S cyclization via C-H functionalization strategy: access to sulfur-containing benzoheterocyclics, Chin. J. Chem., 2018, 36, 921–924 CrossRef CAS.
- J. Li, C. Li, S. Yang, Y. An, W. Wu and H. Jiang, Palladium-catalyzed oxidative sulfenylation of indoles and related electron-rich heteroarenes with aryl boronic acids and elemental sulfur, J. Org. Chem., 2016, 81, 7771–7783 CrossRef CAS PubMed.
-
(a) F. Bellina, F. Benelli and R. Rossi, Direct palladium-catalyzed C-3 arylation of free (NH)-indoles with aryl bromides under ligandless conditions, J. Org. Chem., 2008, 73, 5529–5535 CrossRef CAS PubMed;
(b) Z. Shi, B. Zhang, Y. Cui and N. Jiao, Palladium-catalyzed ring-expansion reaction of indoles with alkynes: from indoles to tetrahydroquinoline derivatives under mild reaction conditions, Angew. Chem., Int. Ed., 2010, 49, 4036–4041 CrossRef CAS PubMed;
(c) J. Koubachi, S. E. Kazzouli, M. Bousmina and G. Guillaumet, Functionalization of imidazo[1,2-a]pyridines by means of metal-catalyzed cross-coupling reactions, Eur. J. Org. Chem., 2014, 5119–5138 CrossRef CAS.
-
(a) T. D. Senecal, A. T. Parsons and S. L. Buchwald, Room temperature aryl trifluoromethylation via copper-mediated oxidative cross-coupling, J. Org. Chem., 2011, 76, 1174–1176 CrossRef CAS PubMed;
(b) J.-T. Yu, H. Guo, Y. Yi, H. Fei and Y. Jiang, The Chan-Lam reaction of chalcogen elements leading to aryl chalcogenides, Adv. Synth. Catal., 2014, 356, 749–752 CrossRef CAS.
- E. S. Degtyareva, J. V. Burykina, A. N. Fakhrutdinov, E. G. Gordeev, V. N. Khrustalev and V. P. Ananikov, Pd-NHC catalytic system for the efficient atom-economic synthesis of vinyl sulfides from tertiary, secondary, or primary thiols, ACS Catal., 2015, 5, 7208–7213 CrossRef CAS.
- R. M. Singh, R. Kumar, K. C. Bharadwaj and T. Gupta, Pd catalyzed facile synthesis of cyclopenta[b]quinolin-1-one via sequential Sonogashira coupling and annulation. an unusual mode of ring closure, using sulphur as a soft nucleophile, Org. Chem. Front., 2016, 3, 1100–1104 RSC.
- J. Li, C. Li, S. Yang, Y. An, W. Wu and H. Jiang, Assembly of 3-sulfenylbenzofurans and 3-sulfenylindoles by palladium-catalyzed cascade annulation/arylthiolation reaction, J. Org. Chem., 2016, 81, 2875–2887 CrossRef CAS PubMed.
- P. Peng, B.-X. Tang, S.-F. Pi, Y. Liang and J.-H. Li, Synthesis of (E,)-3-(isobenzofuran-3(1H)-ylidene)indolin-2-ones by the palladium-catalyzed intramolecular C-H functionalization process, J. Org. Chem., 2009, 74, 3569–3572 CrossRef CAS PubMed.
-
(a) J. Li, S. Yang, H. Jiang, W. Wu and J. Zhao, Palladium-catalyzed coupling of alkynes with unactivated alkenes in ionic liquids: a regio- and stereoselective synthesis of functionalized 1,6-dienes and their analogues, J. Org. Chem., 2013, 78, 12477–12486 CrossRef CAS PubMed;
(b) J. Li, Z. Zhu, S. Yang, Z. Zhang, W. Wu and H. Jiang, Palladium-catalyzed tandem annulation: a strategy to construct 2,3-difunctionalized benzofuran derivatives in ionic liquids, J. Org. Chem., 2015, 80, 3870–3879 CrossRef CAS PubMed.
- J. Li, Y. Wu, M. Hu, C. Li, M. Li, D. He and H. Jiang, A palladium-catalyzed three-component cascade S-transfer reaction in ionic liquids, Green Chem., 2019, 21, 4084–4089 RSC.
-
(a) A. Sperança, B. Godoi and G. Zeni, Iron(III) chloride/diorganyl diselenides: a tool for intramolecular cyclization of alkynone O-methyloximes, J. Org. Chem., 2013, 78, 1630–1637 CrossRef PubMed;
(b) J. Li, R. Huang, C. Li, S. Lin, W. Wu and H. Jiang, Assembly of functionalized 4-alkynylisoxazoles by palladium-catalyzed three-component cascade cyclization/alkynylation, Chem. – Asian J., 2019, 14, 2309–2315 CrossRef CAS PubMed.
-
(a) B. M. Trost and C.-J. Li, Modern Alkyne Chemistry: Catalytic and Atom-Economic Transformations, Wiley VCH, Weinheim, 2014 Search PubMed;
(b) V. P. Boyarskiy, D. S. Ryabukhin, N. A. Bokach and A. V. Vasilyev, Alkenylation of arenes and heteroarenes with alkynes, Chem. Rev., 2016, 116, 5894–5986 CrossRef CAS PubMed;
(c) A. Defert and D. B. Werz, Carbopalladation cascades using carbon-carbon triple bonds: recent advances to access complex scaffolds, Chem. – Eur. J., 2016, 22, 16718–16732 CrossRef PubMed;
(d) M. Iwasaki and Y. Nishihara, Synthesis of multisubstituted olefins through regio- and stereo selective addition of interelement compounds having B-Si, B-B, and Cl-S bonds to alkynes, and subsequent cross-couplings, Chem. Rec., 2016, 16, 2031–2045 CrossRef CAS PubMed.
- M. Iwasaki, T. Fujii, A. Yamamoto, K. Nakajima and Y. Nishihara, Palladium-catalyzed regio- and stereoselective chlorothiolation of terminal alkynes with sulfenyl chlorides, Chem. – Asian J., 2014, 9, 58–62 CrossRef CAS PubMed.
- M. Iwasaki, N. Topolovčan, H. Hu, Y. Nishimura, G. Gagnot, R. Na nakorn, R. Yuvacharaskul, K. Nakajima and Y. Nishihara, Palladium-catalyzed regio- and stereoselective carbothiolation of terminal alkynes with azolyl sulfides, Org. Lett., 2016, 18, 1642–1645 CrossRef CAS PubMed.
- A. B. Lopes, M. Choury, P. Wagner and M. Gulea, Tandem double-cross-coupling/hydrothiolation reaction of 2-sulfenyl benzimidazoles with boronic acids, Org. Lett., 2019, 21, 5943–5947 CrossRef CAS PubMed.
- J. Li, C. Li, L. Ouyang, C. Li, S. Yang, W. Wu and H. Jiang, Palladium-catalyzed regioselective three-component cascade bisthiolation of terminal alkynes, Adv. Synth. Catal., 2018, 360, 1138–1150 CrossRef CAS.
-
(a) S. Tang, P. Peng, S.-F. Pi, Y. Liang, N.-X. Wang and J.-H. Li, Sequential intermolecular aminopalladation/ortho-arene C-H activation reactions of N-phenylpropiolamides with phthalimide, Org. Lett., 2008, 10, 1179–1182 CrossRef CAS PubMed;
(b) A. J. Hickman and M. S. Sanford, Catalyst control of site selectivity in the PdII/IV-catalyzed direct arylation of naphthalene, ACS Catal., 2011, 1, 170–174 CrossRef CAS.
- G. Bastug and S. P. Nolan, Carbon-sulfur bond formation catalyzed by [Pd(IPr*OMe)(cin)Cl] (cin=cinnamyl), J. Org. Chem., 2013, 78, 9303–9308 CrossRef CAS PubMed.
- J. Guilbaud, M. Labonde, A. Selmi, M. Kammoun, H. Cattey, N. Pirio, J. Roger and J.-C. Hierso, Palladium-catalyzed heteroaryl thioethers synthesis overcoming palladium dithiolate resting states inertness: practical road to sulfones and NH-sulfoximines, Catal. Commun., 2018, 111, 52–58 CrossRef CAS.
- Z. Qiao, H. Liu, X. Xiao, Y. Fu, J. Wei, Y. Li and X. Jiang, Efficient access to 1,4-benzothiazine: palladium-catalyzed double C-S bond formation using Na2S2O3 as sulfurating reagent, Org. Lett., 2013, 15, 2594–2597 CrossRef CAS PubMed.
- Z. Qiao, J. Wei and X. Jiang, Direct cross-coupling access to diverse aromatic sulfide: palladium-catalyzed double C-S bond
construction using Na2S2O3 as a sulfurating reagent, Org. Lett., 2014, 16, 1212–1215 CrossRef CAS PubMed.
- M. Wang, Z. Qiao, J. Zhao and X. Jiang, Palladium-catalyzed thiomethylation via a three-component cross-coupling strategy, Org. Lett., 2018, 20, 6193–6197 CrossRef CAS PubMed.
- M. Kim, S. Yu, J. G. Kim and S. Lee, Palladium-catalyzed carbonylation of thioacetates and aryl iodides for the synthesis of S-aryl thioesters, Org. Chem. Front., 2018, 5, 2447–2452 RSC.
-
(a) N. Eewari, H. Nizar, A. Mane, V. George and M. Prasad, Deacetylation of thioacetate using acetyl chloride in methanol, Synth. Commun., 2006, 36, 1911–1914 CrossRef;
(b) C.-C. Han and R. Balakumar, Mild and efficient methods for the conversion of benzylic bromides to benzylic thiols, Tetrahedron Lett., 2006, 47, 8255–8258 CrossRef CAS.
- Y. Li, G. Bao and X.-F. Wu, Palladium-catalyzed intermolecular transthioetherification of aryl halides with thioethers and thioesters, Chem. Sci., 2020, 11, 2187–2192 RSC.
- J.-M. Becht and C. Le Drian, Formation of carbon-sulfur and carbon-selenium bonds by palladium-catalyzed decarboxylative cross-couplings of hindered 2,6-dialkoxybenzoic acids, J. Org. Chem., 2011, 76, 6327–6330 CrossRef CAS PubMed.
- Z. Qiao and X. Jiang, Ligand-controlled divergent cross-coupling involving organosilicon compounds for thioether and thioester synthesis, Org. Lett., 2016, 18, 1550–1553 CrossRef CAS PubMed.
- C. Wang, Z. Zhang, Y. Tu, Y. Li, J. Wu and J. Zhao, Palladium-catalyzed oxidative cross-coupling of arylhydrazines and arenethiols with molecular oxygen as the sole oxidant, J. Org. Chem., 2018, 83, 2389–2394 CrossRef CAS PubMed.
- V. Khakyzadeh, A. Rostami, H. Veisi, B. S. Shaghasemi, E. Reimhult, R. Luque, Y. Xia and S. Darvishi, Direct C-S bond formation via C-O bond activation of phenols in a crossover Pd/Cu dual-metal catalysis system, Org. Biomol. Chem., 2019, 17, 4491–4497 RSC.
- For selected review, see:
(a) S. Shaaban, S. Liang, N.-W. Liu and G. Manolikakes, Synthesis of sulfones via selective C-H-functionalization, Org. Biomol. Chem., 2017, 15, 1947–1955 RSC;
(b) G. Qiu, K. Zhou, L. Gao and J. Wu, Insertion of sulfur dioxide via a radical process: an efficient route to sulfonyl compounds, Org. Chem. Front., 2018, 5, 691–705 RSC . For selected examples, see: ;
(c) S. Cossu, O. De Lucchi, P. Peluso and R. Volpicelli, Improved selective synthesis of (Z)- and (E,)-1,2-bis(phenylsulfonyl)-chloroethylene, Synth. Commun., 2001, 31, 27–32 CrossRef CAS;
(d) B. Xing, C. Ni and J. Hu, Coppe-mediated di-and monofluoromethane sulfonylation of arenediazonium tetrafluoroborates: probing the fluorine effect, Chin. J. Chem., 2018, 36, 206–212 CrossRef CAS;
(e) S. Ye, K. Zhou, P. Rojsitthisak and J. Wu, Metal-free insertion of sulfur dioxide with aryl iodides under ultraviolet irradiation: direct access to sulfonated cyclic compounds, Org. Chem. Front., 2020, 7, 14–18 RSC.
-
(a) A. El-Awa, M. N. Noshi, X. M. du Jourdin and P. L. Fuchs, Evolving organic synthesis fostered by the pluripotent phenylsulfone moiety, Chem. Rev., 2009, 109, 2315–2349 CrossRef CAS PubMed;
(b) D. Kaiser, I. Klose, R. Oost, J. Neuhaus and N. Maulide, Bond-forming and -breaking reactions at sulfur(IV): sulfoxides, sulfonium salts, sulfur ylides, and sulfinate salts, Chem. Rev., 2019, 119, 8701–8780 CrossRef CAS PubMed.
- E. J. Emmett and M. C. Willis, The development and application of sulfur dioxide surrogates in synthetic organic chemistry, Asian J. Org. Chem., 2015, 4, 602–611 CrossRef CAS.
- Y. Fang, Z. Luo and X. Xu, Recent advances in the synthesis of vinyl sulfones, RSC Adv., 2016, 6, 59661–59676 RSC.
- A. Shavnya, K. D. Hesp, V. Mascitti and A. C. Smith, Palladium-catalyzed synthesis of (hetero)aryl alkyl sulfones from (hetero)aryl boronic acids, unactivated alkyl halides, and potassium metabisulfite, Angew. Chem., Int. Ed., 2015, 54, 13571–13575 CrossRef CAS PubMed.
- S. Ye and J. Wu, A palladium-catalyzed reaction of aryl halides, potassium metabisulfite, and hydrazines, Chem. Commun., 2012, 48, 10037–10039 RSC.
- K. Li, I. A. Guzei and J. Darkwa, Insertion of sulfur dioxide into metal-carbon bonds of chloro(methyl)palladium complexes, Polyhedron, 2003, 22, 805–810 CrossRef CAS.
- H. Zhu, Y. Shen, Q. Deng, J. Chen and T. Tu, Pd(NHC)-catalyzed alkylsulfonylation of boronic acids: a general and efficient approach for sulfone synthesis, Chem. Commun., 2017, 53, 12473–12476 RSC.
- N. Miyaura and A. Suzuki, Palladium-catalyzed cross-coupling reactions of organoboron compounds, Chem. Rev., 1995, 95, 2457–2483 CrossRef CAS.
- M. Wang, J. Zhao and X. Jiang, Aryl methyl sulfone construction from eco-friendly inorganic sulfur dioxide and methyl reagents, ChemSusChem, 2019, 12, 3064–3068 CrossRef CAS PubMed.
-
(a) M. Wang, S. Chen and X. Jiang, Construction of functionalized annulated sulfone via SO2/I exchange of cyclic diaryliodonium salts, Org. Lett., 2017, 19, 4916–4919 CrossRef CAS PubMed;
(b) M. Wang, Q. Fan and X. Jiang, Metal-free construction of primary sulfonamides through three diverse salts, Green Chem., 2018, 20, 5469–5473 RSC.
-
(a) P. Tundo and M. Selva, The chemistry of dimethyl carbonate, Acc. Chem. Res., 2002, 35, 706–716 CrossRef CAS PubMed;
(b) P. Tundo, M. Musolino and F. Arick, The reactions of dimethyl carbonate and its derivatives, Green Chem., 2018, 20, 28–85 RSC.
- N. K. Mishra, S. Sharma, J. Park, S. Han and I. S. Kim, Recent advances in catalytic C(sp2)-H allylation reactions, ACS Catal., 2017, 7, 2821–2847 CrossRef CAS.
- Y. Meng, M. Wang and X. Jiang, Multicomponent reductive cross-coupling of an inorganic sulfur dioxide surrogate: straightforward construction of diversely functionalized sulfones, Angew. Chem., 2020, 59, 1346–1353 CrossRef CAS PubMed.
- H. Meerwein, G. Dittmar, R. Gollner, K. Hafner, F. Mensch and O. Steinfort, Aromatic diazo compounds. II. Preparation of aromatic sulfonyl chlorides, a new modification of the Sandmeyer reaction, Chem. Ber., 1957, 90, 841–852 CrossRef CAS.
- P. S. Santos and M. T. S. Mello, The Raman spectra of some molecular complexes of 1-azabicyclo[2.2.2]octane and 1,4-diazabicyclo[2.2.2]octane, J. Mol. Struct., 1988, 178, 121–133 CrossRef CAS.
- B. Nguyen, E. J. Emmet and M. C. Willis, Palladium-catalyzed aminosulfonylation of aryl halides, J. Am. Chem. Soc., 2010, 132, 16372–16373 CrossRef CAS PubMed.
- S. Ye and J. Wu, A palladium-catalyzed three-component coupling of arylboronic acids, sulfur dioxide and hydrazines, Chem. Commun., 2012, 48, 7753–7755 RSC.
- A. S. Deeming, C. J. Russell and M. C. Willis, Palladium(II)-catalyzed synthesis of sulfinates from boronic acids and DABSO: a redox-neutral, phosphine-free transformation, Angew. Chem., Int. Ed., 2016, 55, 747–750 CrossRef CAS PubMed.
- P. F. Schellhammer, An evaluation of bicalutamide in the treatment of prostate cancer, Expert Opin. Pharmacother., 2002, 3, 1313–1328 CrossRef CAS PubMed.
- A. T. Davies, J. M. Curto, S. W. Bagley and M. C. Willis, One-pot palladium-catalyzed synthesis of sulfonyl fluorides from aryl bromides, Chem. Sci., 2017, 8, 1233–1237 RSC.
- H. Zhu, Y. Shen, Q. Deng, C. Huang and T. Tu, One-pot bimetallic Pd/Cu-catalyzed synthesis of sulfonamides from boronic acids, DABSO and O-benzoyl hydroxylamines, Chem. – Asian J., 2017, 12, 706–712 CrossRef CAS PubMed.
- H. Xia, Y. An, X. Zeng and J. Wu, Palladium-catalyzed direct sulfonylation of C-H bonds with the insertion of sulfur dioxide, Chem. Commun., 2017, 53, 12548–12551 RSC.
- C. Xia, Z. Wei, C. Shen, J. Xu, Y. Yang, W. Su and P. Zhang, Palladium-catalyzed direct ortho-sulfonylation of azobenzenes with arylsulfonyl chlorides via C-H activation, RSC Adv., 2015, 5, 52588–52594 RSC.
- X. D. Zhao, E. Dimitrijević and V. M. Dong, Palladium-catalyzed C-H bond functionalization with arylsulfonyl chlorides, J. Am. Chem. Soc., 2009, 131, 3466–3467 CrossRef CAS PubMed.
- S. Chen, Y. Li, M. Wang and X. Jiang, General sulfone construction via sulfur dioxide surrogate control, Green Chem., 2020, 22, 322–326 RSC.
- Y. Li, S. Chen, M. Wang and X. Jiang, Sodium dithionite-mediated decarboxylative sulfonylation: facile access to tertiary sulfones, Angew. Chem., 2020, 59 DOI:10.1002/anie.202001589.
|
This journal is © the Partner Organisations 2020 |
Click here to see how this site uses Cookies. View our privacy policy here.