DOI:
10.1039/C9QO01115C
(Research Article)
Org. Chem. Front., 2020,
7, 64-68
One-pot generation of benzynes from 2-aminophenylboronates via a Rh(II)-catalyzed N–H amination/oxidation/elimination cascade process†
Received
9th September 2019
, Accepted 4th October 2019
First published on 18th November 2019
Abstract
This article describes the first application of 2-aminophenylboronates as precursors for benzynes. Utilizing Rh2(HNCOCF3)4 as the catalyst, Rh(II)-nitrene-mediated N–H amination of the starting material triggered a cascade of oxidation/elimination processes resulting in the generation of benzynes, thus providing suitable conditions for a one-pot cycloaddition with azides or furans. The transformation proceeded under acid-, base-, and fluoride-free conditions, below ambient temperature, and was applicable to a range of substrates containing glycoside and nucleoside moieties, as well as silyl-functional groups.
Introduction
Benzynes are unique reactive intermediates that participate in the simultaneous creation of two bonds, including C–C, C–H, or C–X (X = heteroatom) bonds, on adjacent aromatic carbons via a cycloaddition reaction or the sequential addition of a nucleophile and an electrophile.1 With a view to further enhancing the synthetic utility of these reactive species, significant efforts have been devoted to developing efficient benzyne precursors.2–6 Among the known precursors, 2-trimethylsilylphenyl triflates, which generate benzynes on treatment with fluoride salts, have been widely used. The advantages of using these compounds include availability, stability, and mild reaction conditions.2–4 Aside from phenol derivatives, aniline derivatives comprise a more classical class of benzyne precursors such as 1-aminobenzotriazole, 1,2,3-benzothiadiazole 1,1-dioxide, and diazonium salts derived from anthranilic acids.5 However, with the exception of anthranilic acids, the aforementioned precursors are not currently commonly used. Following early examples and some notable advancements in the area of aryne chemistry, also referred to as its renaissance, the development of novel aniline-based precursors has been stagnated over the last few decades.6
As part of the research on the transformation of anilines using the Rh(II)-nitrene species,7,8 we recently reported the catalytic single-step synthesis of N-aryl-N′-tosyldiazenes from primary anilines.8a In the presence of dirhodium(II) complexes as the catalysts and 2 equiv. of (tosylimino)-2,4,6-trimethylphenyliodinane (TsN
IMes), anilines underwent N–H amination by Rh(II)-nitrene followed by oxidation to provide the products in one pot (Scheme 1a). Although tosyldiazenes displayed higher stability than diazonium salts, their C–N bonds were readily transformed into C–C bonds via the Suzuki–Miyaura or Sonogashira coupling.9 Thus, we successfully validated the compounds as safer surrogate of diazonium salts for C–N bond transformation.
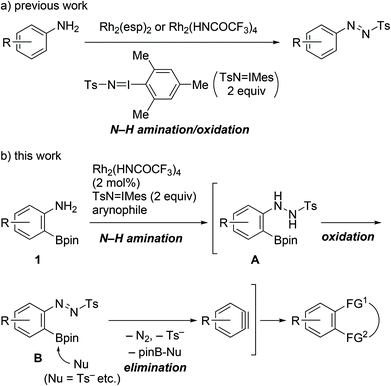 |
| Scheme 1 (a) Rh(II)-Catalyzed synthesis of N-aryl-N′-tosyldiazene. (b) Utilization of 2-aminophenylboronates as benzyne precursors through a Rh(II)-catalyzed N–H amination/oxidation/elimination cascade process. | |
In this context, we herein report the utilization of 2-aminophenylboronates as novel benzyne precursors through the combined use of Rh2(HNCOCF3)4 and TsN
IMes (Scheme 1b). Under the described conditions, the Rh(II)-nitrene-mediated N–H amination of the starting materials triggered a cascade of oxidation/elimination processes resulting in the generation of benzynes, thus providing suitable conditions for a one-pot cycloaddition with azides or furans.
Results and discussion
During the course of our previous study (Scheme 1a),8a we found that the reaction of a commercially available 2-aminophenylboronic acid pinacol ester (1a) under Rh2(HNCOCF3)4 catalysis unexpectedly provided phenyl p-tolyl sulfone (3) in 30% yield, instead of tosyldiazene 2 (Scheme 2). This result, including simultaneous transformation of the neighboring C–N and C–B bonds, strongly indicated the generation of the benzyne intermediate from 1ain situ. On the basis of this hypothesis, we subsequently attempted to trap benzyne with an arynophile, methyl 4-azidobenzoate (4a). As expected, the reaction in the presence of 4a (2 equiv.), and under otherwise identical conditions, led to the formation of cycloadduct 5aa in 57% yield (Table 1, entry 1). Therefore, 2-aminophenylboronate undeniably functioned as a benzyne precursor under the described conditions. Virtually the same result was obtained using the inverse ratio of the precursor and the arynophile (entry 2, 1a
:
4a = 1.5
:
1). Changing the solvent from CH2Cl2 to MeCN had little impact on the product yields, whereas toluene and benzotrifluoride led to a slight drop in the obtained yields (entries 3–5). The influence of iminoiodinane was also investigated (entry 6).10 In contrast to previous work, no significant difference was observed between TsN
IMes and TsN
IPh. Utilizing Rh2(HNCOCF3)4 as the catalyst was remarkably effective for the transformation, particularly in comparison to the commonly used Rh(II) catalysts such as Rh2(OAc)4 and Rh2(esp)2 (entry 1 vs. entries 7 and 8). A review of the starting boronates indicated that pinanediolate 1b provided a comparable outcome to pinacolate 1a, whereas less bulky neopentylglycolate 1c and unprotected boronic acid 1d resulted in a noticeable drop in the yields (entries 1, 9–11).
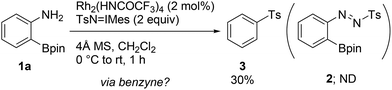 |
| Scheme 2 Unexpected transformation of 2-aminophenylboronate 1a under Rh(II)-catalyzed amination conditions. pin = pinacolato. | |
Table 1 Dirhodium(II)-catalyzed cycloaddition of 2-aminophenylboronates 1 with methyl 4-azidobenzoate (4a)a
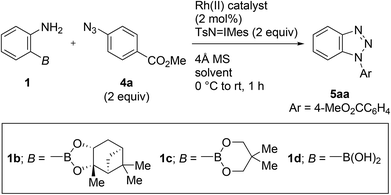
|
Entry |
Aniline |
Rh(II)-Catalyst |
Solvent |
Yieldb (%) |
Reaction conditions: 1 (0.10 mmol), 4a (0.20 mmol), Rh(II) catalyst (0.002 mmol, 2 mol%), iminoiodinane (0.20 mmol), and 4 Å MS (powder, 40 mg) in the indicated solvent (1.0 mL).
Isolated yield.
1a : 4a : TsN IMes = 1.5 : 1.0 : 3.0.
3 Å MS was used.
TsN IPh was used. Ts = tosyl. esp = α,α,α,α-tetramethyl-1,3-benzenedipropanoate.
|
1 |
1a
|
Rh2(HNCOCF3)4 |
CH2Cl2 |
57 |
2c |
1a
|
Rh2(HNCOCF3)4 |
CH2Cl2 |
58 |
3d |
1a
|
Rh2(HNCOCF3)4 |
MeCN |
57 |
4 |
1a
|
Rh2(HNCOCF3)4 |
Toluene |
41 |
5 |
1a
|
Rh2(HNCOCF3)4 |
CF3C6H5 |
51 |
6e |
1a
|
Rh2(HNCOCF3)4 |
CH2Cl2 |
53 |
7 |
1a
|
Rh2(OAc)4 |
CH2Cl2 |
17 |
8 |
1a
|
Rh2(esp)2 |
CH2Cl2 |
33 |
9 |
1b
|
Rh2(HNCOCF3)4 |
CH2Cl2 |
58 |
10 |
1c
|
Rh2(HNCOCF3)4 |
CH2Cl2 |
12 |
11 |
1d
|
Rh2(HNCOCF3)4 |
CH2Cl2 |
12 |
With the optimized conditions in hand, we then investigated the cycloaddition using a range of azides (Scheme 3a). All the aryl and alkyl azides that were examined gave cycloadducts 5ab–ah in 48–61% yield. It is notable that the trialkylsilyl and the enolizable carbonyl groups remained unaffected during the transformation (5ab, 5ae, 5af, 5ag, and 5ah). These results indicate that the novel methodology is orthogonal to the conventional fluoride- or strong base-mediated approaches in terms of functional group tolerance. The present protocol was also applicable to complex azides, associated with biomolecules including glycoside and nucleoside moieties. The reaction with 2-azidoethyl β-D-glucopyranoside proceeded without anomerization and resulted in the formation of 5ag in 58% yield. The late-stage functionalization of O-(tert-butyldimethylsilyl) (TBS) protected zidovudine, an azide-containing anti-HIV nucleoside used in the clinic, was achieved, and cycloadduct 5ah was obtained in 58% yield. Aside from the cycloaddition with azides, the reactions with furans, including 2,5-dimethylfuran and 2-acetylfuran, gave cycloadduct 6a and 6b in 52% and 46% yields, respectively (Scheme 3b). Utilizing 2-trimethylsilyloxyfuran led to the formation of 1-naphthol 6c′ in 31% yield via the ring opening of cycloadduct 6c.11 Unfortunately, reactions with other arynophiles such as 2,5-diphenylisobenzofuran, anthracene, nitrone, and β-ketoester failed to provide the expected cycloadducts.12 However, the reason is currently unclear.
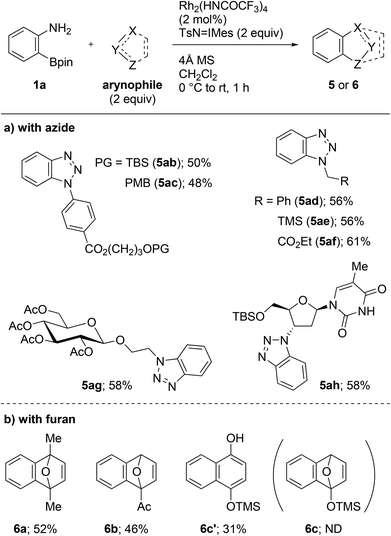 |
| Scheme 3 Rh2(HNCOCF3)4-catalyzed cycloadditions of 2-aminophenylboronate 1a with azides or furans. TBS = tert-butyldimethylsilyl. PMB = para-methoxybenzyl. TMS = trimethylsilyl. | |
Our attention next turned to the preparation of functionalized 2-aminophenylboronates (Table 2). Although the Miyaura borylation of 2-haloaniline derivatives or the corresponding nitrobenzenes provides reliable access to the described compounds,13,14 the post-functionalization of 2-aminophenylboronate would have the advantage of a rapid and divergent conversion to a series of precursors. The brominated precursor 1e was prepared from 1a by treatment with N-bromosuccinimide (NBS) (entry 1). A gold-catalyzed C–H insertion of phenyldiazoacetate into the N-Boc-protected aniline 1a′ proceeded at the para position of the amino group, albeit in low yield,15 and the desired benzyne precursor 1f was obtained after removal of the Boc group by treatment with trifluoroacetic acid (TFA) (entry 2). A methoxycarbonyl-substituted precursor 1g was readily available from the corresponding commercially available starting materials such as 2-aminophenylboronic acid or the more inexpensive 2-nitrophenylboronic acid (see ESI†). Reduction of 1g with DIBAL-H, followed by protection with the TBS group gave benzyl silyl ether 1h (entry 3).16
Table 2 Synthesis of functionalized 2-aminophenylboronates 1e–h
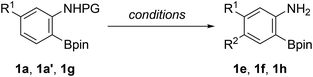
|
Entry |
Substrate |
Conditions (yield) |
Product |
1 |
1a
|
NBS, NH4OAc |
1e
|
R1 = H |
MeCN (95%) |
R1 = H |
PG = H |
|
R2 = Br |
2 |
1a′
|
(1) Ph3PAuNTf2 |
1f
|
R1 = H |
PhCH(N2)CO2Me |
R1 = H |
PG = Boc |
CH2Cl2 (28%) |
R2 = CHPhCO2Me |
|
(2) TFA |
|
|
CH2Cl2 (46%) |
|
3 |
1g
|
(1) DIBAL-H, THF |
1h
|
R1 = CO2Me |
−40 °C (66%) |
R1 = CH2OTBS |
PG = H |
(2) TBSCl, TBAB |
R2 = H |
|
CH2Cl2 (47%) |
|
Similarly to 1a, all the synthesized precursors 1e–h uneventfully generated substituted benzyne species under identical conditions, and cycloadducts 5ea–ha were obtained as mixture of the regioisomers (Scheme 4).
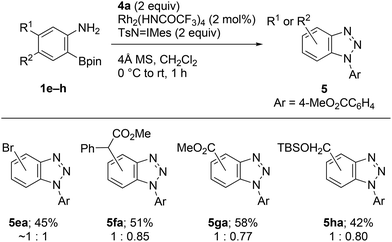 |
| Scheme 4 Rh2(HNCOCF3)4-catalyzed cycloaddition of 2-aminophenylboronates 1e–h with methyl 4-azidobenzoate (4a). | |
A plausible reaction pathway for this transformation is illustrated in Scheme 1b. The N–H amination of aniline 1 with Rh(II)-nitrene leads to the formation of N-aryl-N′-tosylhydrazine A, which on reacting with TsN
IMes is immediately oxidized into N-aryl-N′-tosyldiazene B.8a It is suspected that after activation of the boronate group with internal nucleophiles (i.e., Ts−, TsNH2, etc.),4d,f,17 elimination of the boronate and tosyldiazene moieties results in the one-pot generation of benzynes. To verify the proposed pathway, we then attempted to isolate the putative intermediate A or B utilizing the described conditions; however, all our efforts were unsuccessful. Instead, N-tosylhydrazine 8c was prepared through an alternative synthetic pathway, which included electrophilic amination of 3-methoxyphenylboronate 7 with benzyl 2,2,2-trichloroethyl azodicarboxylate, followed by a three-step manipulation of the protecting groups (Scheme 5).18,19 The cycloaddition of 8c with azide 4a was subsequently investigated. Treatment of the starting material with TsN
IMes resulted in the formation of the expected cycloadduct 9 in the presence or absence of the Rh2(HNCOCF3)4 catalyst. Consequently, the reaction pathway presented in Scheme 1b was verified.
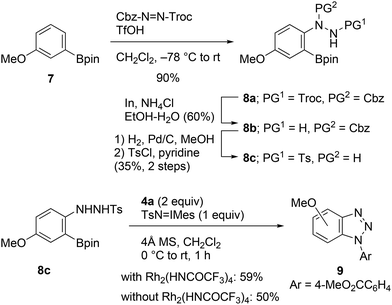 |
| Scheme 5 Synthesis of N-aryl-N′-tosylhydrazine 8c and cycloaddition with methyl 4-azidobenzoate (4a). | |
Conclusions
In conclusion, we demonstrated that 2-aminophenylboronates can be used as novel benzyne precursors. In the presence of the dirhodium(II)-complex catalyst, Rh(II)-nitrene-mediated N–H amination of the precursors triggered a cascade of oxidation/elimination processes resulting in the generation of benzynes, thus providing the desired cycloadducts in one pot. The transformation proceeded under acid-, base-, and fluoride-free conditions, below ambient temperature, and was orthogonal to the conventional methods in terms of functional group tolerance. Consequently, this methodology was applicable to a range of substrates containing glycoside and nucleoside moieties, as well as silyl-functional groups. Further extension of this methodology to a range of (hetero)aryne precursors as well as further mechanistic evaluation are currently in progress.
Conflicts of interest
There are no conflicts to declare.
Acknowledgements
We thank T. Koseki of the Analytical Center of Meiji Pharmaceutical University for mass spectral measurements. This work is financially supported by a Grant-in-Aid for Young Scientists (B) (No. 17K15428) from JSPS, Japan.
Notes and references
-
(a) P. M. Tadross and B. M. Stoltz, Chem. Rev., 2012, 112, 3550 CrossRef CAS PubMed;
(b) A. V. Dubrovskiy, N. A. Markina and R. C. Larock, Org. Biomol. Chem., 2013, 11, 191 RSC;
(c)
H. Yoshida, Nucleophilic Coupling with Arynes, in Comprehensive Organic Synthesis, ed. P. Knochel and G. A. Molander, Elsevier, Amsterdam, 2nd edn, 2014, vol. 4, p. 517 Search PubMed;
(d) A. E. Goetz, T. K. Shah and N. K. Garg, Chem. Commun., 2015, 51, 34 RSC;
(e) S. Yoshida and T. Hosoya, Chem. Lett., 2015, 44, 1450 CrossRef CAS;
(f) F. M. Idiris and C. R. Jones, Org. Biomol. Chem., 2017, 15, 9044 RSC;
(g) A. Yoshimura, A. Saito and V. V. Zhdankin, Chem. – Eur. J., 2018, 24, 15156 CrossRef CAS;
(h) H. Takikawa, A. Nishii, T. Sakai and K. Suzuki, Chem. Soc. Rev., 2018, 47, 8030 RSC.
-
(a) Y. Himeshima, T. Sonoda and H. Kobayashi, Chem. Lett., 1983, 1211 CrossRef CAS;
(b) N. Furukawa, T. Shibutani and H. Fujihara, Tetrahedron Lett., 1987, 28, 2727 CrossRef CAS;
(c) T. Matsumoto, T. Hosoya, M. Katsuki and K. Suzuki, Tetrahedron Lett., 1991, 32, 6735 CrossRef CAS;
(d) T. Kitamura and M. Yamane, J. Chem. Soc., Chem. Commun., 1995, 983 RSC.
-
(a) M. Uchiyama, T. Miyoshi, Y. Kajihara, T. Sakamoto, Y. Otani, T. Ohwada and Y. Kondo, J. Am. Chem. Soc., 2002, 124, 8514 CrossRef CAS;
(b) I. Sapountɀis, W. Lin, M. Fischer and P. Knochel, Angew. Chem., Int. Ed., 2004, 43, 4364 CrossRef;
(c) T. Hamura, T. Arisawa, T. Matsumoto and K. Suzuki, Angew. Chem., Int. Ed., 2006, 45, 6842 CrossRef CAS;
(d) H. S. Kim, S. Gowrisankar, E. S. Kim and J. N. Kim, Tetrahedron Lett., 2008, 49, 6569 CrossRef CAS;
(e) A. A. Cant, L. Roberts and M. F. Greaney, Chem. Commun., 2010, 46, 8671 RSC;
(f) T. Ikawa, T. Nishiyama, T. Nosaki, A. Takagi and S. Akai, Org. Lett., 2011, 13, 1730 CrossRef CAS;
(g) S. Kovács, Á. I. Csincsi, T. Z. Nagy, S. Boros, G. Timári and Z. Novák, Org. Lett., 2012, 14, 2022 CrossRef;
(h) T. Hamura, Y. Chuda, Y. Nakatsuji and K. Suzuki, Angew. Chem., Int. Ed., 2012, 51, 3368 CrossRef CAS;
(i) T. R. Hoye, B. Baire, D. Niu, P. H. Willoughby and B. P. Woods, Nature, 2012, 490, 208 CrossRef CAS;
(j) S. Yoshida, K. Uchida and T. Hosoya, Chem. Lett., 2014, 43, 116 CrossRef CAS;
(k) Q. Chen, H. Yu, Z. Xu, L. Lin, X. Jiang and R. Wang, J. Org. Chem., 2015, 80, 6890 CrossRef CAS;
(l) S. K. Sundalam, A. Nilova, T. L. Seidl and D. R. Stuart, Angew. Chem., Int. Ed., 2016, 55, 8431 CrossRef CAS;
(m) E. Gorobets, M. Parvez, D. J. Derksen and B. A. Keay, Chem. – Eur. J., 2016, 22, 8479 CrossRef CAS;
(n) M. Wang and Z. Huang, Org. Biomol. Chem., 2016, 14, 10185 RSC;
(o) M. Mesgar and O. Daugulis, Org. Lett., 2016, 18, 3910 CrossRef CAS;
(p) T. Ikawa, S. Masuda, H. Nakajima and S. Akai, J. Org. Chem., 2017, 82, 4242 CrossRef CAS;
(q) J. Shi, H. Xu, D. Qiu, J. He and Y. Li, J. Am. Chem. Soc., 2017, 139, 623 CrossRef CAS;
(r) Y. Nishiyama, S. Kamada, S. Yoshida and T. Hosoya, Chem. Lett., 2018, 47, 1216 CrossRef CAS;
(s) K. Devaraj, F. J. L. Ingner, C. Sollert, P. J. Gates, A. Orthaber and L. T. Pilarski, J. Org. Chem., 2019, 84, 5863 CrossRef CAS.
- In recent years, 2-borylphenyl triflates have also emerged as efficient precursors for the generation of benzynes under various conditions, including treatment with transition metal catalysts, organolithium reagents, and fluoride salts.
(a) M. Retbøll, A. J. Edwards, A. D. Rae, A. C. Willis, M. A. Bennett and E. Wenger, J. Am. Chem. Soc., 2002, 124, 8348 CrossRef;
(b) Y. Sumida, T. Kato and T. Hosoya, Org. Lett., 2013, 15, 2806 CrossRef CAS;
(c) J.-A. García-López and M. F. Greaney, Org. Lett., 2014, 16, 2338 CrossRef;
(d) T. Ikawa, R. Yamamoto, A. Takagi, T. Ito, K. Shimizu, M. Goto, Y. Hamashima and S. Akai, Adv. Synth. Catal., 2015, 357, 2287 CrossRef CAS;
(e) Y. Sumida, T. Sumida, D. Hashizume and T. Hosoya, Org. Lett., 2016, 29, 5600 CrossRef;
(f) A. Yoshimura, J. M. Fuchs, K. R. Middleton, A. V. Maskaev, G. T. Rohde, A. Saito, P. S. Postnikov, M. S. Yusubov, V. N. Nemykin and V. V. Zhdankin, Chem. – Eur. J., 2017, 23, 16738 CrossRef CAS.
-
(a) L. Friedman and F. M. Logullo, J. Am. Chem. Soc., 1963, 85, 1549 CrossRef CAS;
(b) G. Wittig and R. W. Hoffmann, Org. Synth., 1967, 47, 4 CrossRef CAS;
(c) C. D. Campbell and C. W. Rees, J. Chem. Soc. C, 1969, 742 RSC;
(d) C. Spiteri, C. Mason, F. Zhang, D. J. Ritson, P. Sharma, S. Keeling and J. E. Moses, Org. Biomol. Chem., 2010, 8, 2537 RSC;
(e) W. Huang, Q. Gao and G.-J. Boons, Chem. – Eur. J., 2015, 21, 12920 CrossRef CAS;
(f) E. M. Serum, S. Selvakumar, N. Zimmermann and M. P. Sibi, Green Chem., 2018, 20, 1448 RSC.
- A. W. Gann, J. W. Amoroso, V. J. Einck, W. P. Rice, J. J. Chambers and N. A. Schnarr, Org. Lett., 2014, 16, 2003 CrossRef CAS.
- Reviews on Rh(II)-nitrene, see;
(a) P. Müller and C. Fruit, Chem. Rev., 2003, 103, 2905 CrossRef;
(b) A. R. Dick and M. S. Sanford, Tetrahedron, 2006, 62, 2439 CrossRef CAS;
(c) J. L. Roizen, M. E. Harvey and J. Du Bois, Acc. Chem. Res., 2012, 45, 911 CrossRef CAS;
(d) J. Buendia, G. Grelier and P. Dauban, Adv. Organomet. Chem., 2015, 64, 77 CrossRef CAS;
(e) B. Darses, R. Rodrigues, L. Neuville, M. Mazurais and P. Dauban, Chem. Commun., 2017, 53, 493 RSC.
-
(a) M. Ito, A. Tanaka, K. Higuchi and S. Sugiyama, Eur. J. Org. Chem., 2017, 1272 CrossRef CAS;
(b) M. Ito, T. Nakagawa, K. Higuchi and S. Sugiyama, Org. Biomol. Chem., 2018, 16, 6876 RSC.
-
(a) M. J. Evers, L. E. Christiaens, M. R. Guillaume and M. J. Renson, J. Org. Chem., 1985, 50, 1779 CrossRef CAS;
(b) J.-B. Liu, H. Yan, H.-X. Chen, Y. Luo, J. Weng and G. Lu, Chem. Commun., 2013, 49, 5268 RSC;
(c) J.-B. Liu, F.-J. Chen, N. Liu and J. Hu, RSC Adv., 2015, 5, 45843 RSC;
(d) S. Crespi, S. Protti and M. Fagnoni, J. Org. Chem., 2016, 81, 9612 CrossRef CAS;
(e) M. Malacarne, S. Protti and M. Fagnoni, Adv. Synth. Catal., 2017, 359, 3826 CrossRef CAS;
(f) Y. Xu, X. Yang and H. Fang, J. Org. Chem., 2018, 83, 12831 CrossRef CAS PubMed;
(g) L. Blank, M. Fagnoni, S. Protti and M. Rueping, Synthesis, 2019, 51, 1243 CrossRef CAS.
- The use of other iminoiodinanes such as pNsN
IMes, 4-ClC6H4SO2N
IMes, 4-MeOC6H4SO2N
IMes did not improve product yield (36–51%).
- M. Ballantine, M. L. Menard and W. Tam, J. Org. Chem., 2009, 74, 7570 CrossRef CAS.
- The structures of sluggish arynophiles are shown in below.
.
-
(a) P.-E. Broutin, I. Čerňa, M. Campaniello, F. Leroux and F. Colobert, Org. Lett., 2004, 6, 4419 CrossRef CAS;
(b) H. Fang, G. Kaur, J. Yan and B. Wang, Tetrahedron Lett., 2005, 46, 1671 CrossRef CAS;
(c) J. Lu, Z.-Z. Guan, J.-W. Gao and Z.-H. Zhang, Appl. Organomet. Chem., 2011, 25, 537 CrossRef CAS;
(d) H. Ji, L.-Y. Wu, J.-H. Cai, G.-R. Li, N.-N. Gan and Z.-H. Wang, RSC Adv., 2018, 8, 13643 RSC.
- Recently, M. R. Smith III developed the NH2-directed ortho C–H borylation using Ir catalyst.
(a) S. M. Preshlock, D. L. Plattner, P. E. Maligres, S. W. Krska, R. E. Malecɀka Jr. and M. R. Smith III, Angew. Chem., Int. Ed., 2013, 52, 12915 CrossRef CAS;
(b) M. R. Smith III, R. Bisht, C. Haldar, G. Pandey, J. E. Dannatt, B. Ghaffari, R. E. Malecɀka Jr. and B. Chattopadhyay, ACS Catal., 2018, 8, 6216 CrossRef.
-
(a) Z. Yu, B. Ma, M. Chen, H.-H. Wu, L. Liu and J. Zhang, J. Am. Chem. Soc., 2014, 136, 6904 CrossRef CAS;
(b) Y. Xi, Y. Su, Z. Yu, B. Dong, E. J. McClain, Y. Lan and X. Shi, Angew. Chem., Int. Ed., 2014, 53, 9817 CrossRef CAS.
- B. Sreedhar and V. S. Rawat, J. Appl. Chem., 2012, 1, 174 CAS . Silylation using conventional conditions (TBSCl/imidazole, TBSOTf/2,6-lutidine) did not give the desired outcome.
- While the fate of boronate moiety is currently unclear, we considered that p-toluenesulfinate activated the boronate as O- or S-nucleophile. It is reported that boronates are even activated by water to generate benzynes from aryl(2-borylphenyl)iodonium salts (see ref. 4f). Similar to this, in our case, p-toluenesulfonamide can also be
an activator.
-
(a) Y. Leblanc and N. Boudreault, J. Org. Chem., 1995, 60, 4268 CrossRef CAS;
(b) H. Mitchell and Y. Leblanc, J. Org. Chem., 1994, 59, 682 CrossRef CAS.
- T. Mineno, S.-R. Choi and M. A. Avery, Synlett, 2002, 883 CrossRef CAS.
Footnote |
† Electronic supplementary information (ESI) available: Experimental procedures, compound characterization data, and copies of NMR spectra for all new compounds. See DOI: 10.1039/c9qo01115c |
|
This journal is © the Partner Organisations 2020 |