DOI:
10.1039/D0QM00137F
(Research Article)
Mater. Chem. Front., 2020,
4, 3578-3584
Fluoro-alkyl substituted isothianaphthene bisimides as stable n-type semiconductors†
Received
9th March 2020
, Accepted 22nd April 2020
First published on 24th April 2020
Abstract
The development of air-stable organic semiconductors (OSCs) with high charge mobilities is of pivotal importance for practical applications of organic thin film transistors (OTFTs) in various fields. Although several OSCs with high hole mobilities have been realized, their n-type counterparts lag far behind in terms of charge mobilities and especially air-stability potentially due to the highly sensitive nature of electrons to ambient oxidants. Isothianaphthene, a kind of non-classical thiophene, possesses a unique electronic structure and isothianaphthene bismide (BTDI) has been demonstrated as an efficient core for stable n-type OSCs. In this work, we introduced two fluoric groups with different alkyl-chains at the N-position of isothianaphthene-2,3,6,7-tetracarboxylic acid diimide and obtained new air-stable n-type OSCs, BTDI-OCF3 and BTDI-CF. The distinct length of the alkyl-chains caused different molecular packing styles of the resulting materials, as revealed by atomic force microscopy. The crystallinity of BTDI-OCF3 is found to be much better than BTDI-CF and single crystals of BTDI-OCF3 could easily be obtained by solution process. Besides, OTFTs based on these new semiconductors showed almost no hysteresis when tested in a glovebox and very small hysteresis is observed in air with a small decay of current, indicating good stability of both of these materials for their applications in n-type OTFTs.
Introduction
As an important building block for flexible and printed organic electronics, organic thin film transistors (OTFTs) have shown extensive prospects for a variety of applications, including electronic papers (e-papers), electronic skins (e-skins), sensors, radio frequency identification (RFID) tags, active-matrix displays, and synapses, owing to their large area, low cost, mechanical flexibility, and ease of fabrication.1–5 Since the first report on OTFTs based on polythiophene in 1986,6 intensive research efforts from both academia and industry have been dedicated to realize high performance OTFTs, leading to remarkable progress in the past few decades. For instance, a number of organic semiconductors (OSCs) have been developed with charge carrier mobilities exceeding 10 cm2 V−1 s−1 for holes and 1 cm2 V−1 s−1 for electrons.7–11 However, the development of n-type OSCs is still far behind the p-type counterparts in terms of their carrier mobilities and especially the air-stability,12,13 due to the high sensitivity of electrons to ambient oxidants (such as O2 and H2O), which seriously degrade the charge mobility and device stability of n-channel OTFTs.14,15 On the other hand, it is noteworthy that a high-energy barrier between the active layer and the source/drain electrodes further hinders charge injection.16 The imbalance between the characteristics of p-type and n-type semiconductors significantly restricts the practical applications of OTFTs in complementary circuits and p–n junctions.
In general, the ambient stability of n-type OSCs for applications in OTFTs could be improved through two different molecular design strategies. One is decreasing the lowest unoccupied molecular orbital (LUMO) level below than that of the most atmospheric trapping oxidants via introducing strong electron-withdrawing groups, such as cyano (–CN), fluoroalkyl, imide/amide and so on. It is well established that a LUMO level of ca. −4.0 eV or lower is beneficial to realize air-stable carrier transport for OSCs since it could minimize most of the atmospheric trapping.17,18 On the other hand, a low LUMO level also reduces the electron injection barrier, which allows efficient and stable electron injection and transport. Until now, a series of ambient-air-stable n-type OSCs, including perylene diimdes (PDI), naphthalene diimides (NDI), thieno[3,4-c]pyrrole-4,6-dione (TPD) and isoindigo have been developed.12,19–23 Consequently, a considerable enhancement in mobility from 10−4 to 12.6 cm2 V−1 s−1 and excellent air-stability have been realized in n-channel OTFTs.24–26 The other approach to improve the ambient stability of n-type OSCs is promoting a more densely-packed steric barrier to the atmosphere, which could prevent moisture and oxygen penetration into the organic layer in steric.27 The introduction of a fluoro-alkyl chain to the N-atom of the NDI or PDI core could lead to dense self-segregation of the side fluoro-substituted chain, which impedes the movement of moisture and oxygen into the active channel.28–31 More importantly, these fluoric materials not only show excellent air-stability but also exhibit high electron mobilities with considerable on/off ratios in OTFTs.26,32–35 For instance, the highest reported electron mobility of an NDI small molecule, Cl2-NDI, is as high as 8.6 cm2 V−1 s−1 for single crystals with a slight mobility degradation of 13% (after 3 months) in ambient air.34,36
The electronic structure of isothianaphthene was first studied by Joseph37 and later, Fred Wudl et al. reported poly(isothianaphthene) by a polymerization method.38 The conductivity of poly(isothianaphthene) could be effectively adjusted by doping various ions. Isothianaphthene was also explored as an effective unit for low band gap conjugated polymers. In our previous work, we have reported an alkyl substituted isothianaphthene derivative, N,N′-bis(n-hexyl)isothianaphthene-2,3,6,7-tetracarboxylic acid diimide (BTDI-C6), which also possesses a deep LUMO level (−4.21 eV), approximately 0.32 eV lower than that of NDI-C6 and much higher air-stability of the resulting OTFT devices.39 It is well known that fluorinated substituents play a significant role in enhancing the air-stability of n-type OSCs because of their dense molecular packing, which prevents the diffusion of moisture and oxygen into the device. To date, 3-(perfluorooctyl)propyl (CF) and p-(trifluoromethoxy)benzyl (BOCF3) substituted at the N,N′-positions of NDI have shown excellent air-stability and high mobility in OTFTs.32,33 In this study, we introduced these two fluorinated substituents to the BTDI core to investigate their effect on the molecular packing and air-stability of the resulting BTDI derivatives, i.e.BTDI-CF and BTDI-OCF3. Similar LUMO energy levels with a slight distinction of 0.02 eV were observed for these materials. Remarkably, BTDI-CF showed reasonable solubility in chloroform (>2 mg mL−1) and BTDI-OCF3 also exhibited excellent solubility in chloroform (>10 mg mL−1), while NDI-OCF3 is not soluble.33 The OTFT devices based on BTDI-CF showed higher mobility (0.0368 cm2 V−1 s−1) but lower air-stability than the BTDI-OCF3 based counterparts and both types of the devices demonstrated good air-stability with small hysteresis. The relationship between the material structure and the corresponding device performance is further studied systematically.
Experimental
Synthesis and thermal analysis
The synthetic routes of BTDI-OCF3 and BTDI-CF are shown in Scheme 1. The synthetic method was similar to that reported in the literature39,40 and the obtained products were finally purified by sublimation with satisfactory yield. The corresponding synthetic procedures, chemical structure characterization (1H NMR, 13C NMR and mass spectroscopy analysis) and further experimental details are provided in the ESI.† The thermal properties of BTDI-OCF3 and BTDI-CF are characterized by thermal gravimetric analysis (TGA) and differential scanning calorimetry (DSC). BTDI-OCF3 showed better thermal stability and a higher melting point than BTDI-CF due to the more rigid substituent. Both BTDI-OCF3 and BTDI-CF exhibited good thermal stability with decomposition temperatures of up to 385 °C and 338 °C, respectively (see Fig. S1a, ESI†). In addition, the DSC results revealed melting temperatures of 264 °C and 214 °C for BTDI-OCF3 and BTDI-CF, respectively, as shown in Fig. S1b (ESI†).
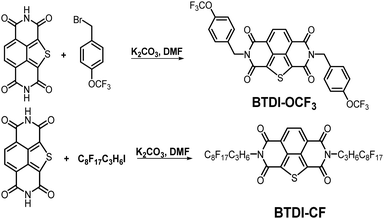 |
| Scheme 1 The synthetic routes of BTDI-OCF3 and BTDI-CF. | |
Electrochemical and optical properties
The electrochemical properties of BTDI-OCF3 and BTDI-CF are studied by cyclic voltammetry (CV) in CH2Cl2 solution; the corresponding cyclic voltammograms are presented in Fig. 1a. As expected, both BTDI derivatives exhibited two reversible reduction peaks, indicating their typical n-type charge transfer properties. The reduction potentials of BTDI-OCF3 and BTDI-CF estimated from the onsets of the first reduction peak are −0.49 eV and −0.51 eV, from which nearly-similar LUMO energy levels of −4.13 and −4.11 eV versus ferrocene were deduced. The photophysical properties of BTDI-OCF3 and BTDI-CF were investigated in solution and thin-film form (Fig. 1b). Similar ultraviolet (UV) absorption bands were observed for both of these compounds in dichloromethane (DCM) solution. However, the absorption spectrum of the BTDI-OCF3 thin-film showed a slight bathochromic shift with respect to BTDI-CF, which could be attributed to the difference in the molecular packing geometry of these materials. These results suggest that BTDI-OCF3 may exhibit stronger intermolecular interactions in the solid-state thin-film. The optical bandgap obtained from the absorption onset of thin-film spectra is estimated to be 2.43 eV for BTDI-OCF3 and 2.53 eV for BTDI-CF. On the other hand, density functional theory (DFT) calculations were performed at the B3LYP/6-13G(d) level by using the Gaussian 09 program package, which predicted nearly-similar LUMO levels of −4.25 eV and −4.28 eV for BTDI-OCF3 and BTDI-CF, respectively. Both the experimental and theoretical results revealed comparable LUMO levels of these compounds, indicating that the introduction of different fluorinated substituents at the N,N′-positions may not greatly affect the LUMO energy level of the resulting material. The corresponding electrochemical and optical properties of BTDI-OCF3 and BTDI-CF are summarized in Table 1.
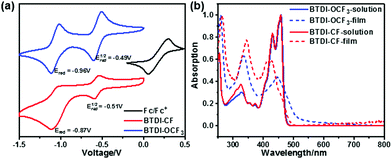 |
| Fig. 1 (a) Cyclic voltammograms of BTDI-CF, BTDI-OCF3 and ferrocene in DCM solution. (b) UV-vis absorption spectra of the BTDI derivatives in thin films and DCM solution. | |
Table 1 The electrochemical and optical properties of BTDI-OCF3 and BTDI-CF
Compound |
E
red
1/2/V |
λ
on/nm |
Experimental |
Calculated |
E
HOMO/eV |
E
LUMO/eV |
Gap/eV |
E
HOMO/eV |
E
LUMO/eV |
Gap/eV |
λ
e/eV |
VEA/eV |
BTDI-OCF3
|
−0.49 |
510.3 |
−6.56 |
−4.13 |
2.43 |
−7.03 |
−4.25 |
2.78 |
0.397 |
2.806 |
BTDI-CF
|
−0.51 |
489.5 |
−6.64 |
−4.11 |
2.53 |
−7.30 |
−4.28 |
3.02 |
0.330 |
2.809 |
Device fabrication and characterization
OTFTs were fabricated by thermal deposition at various substrate temperatures (Tsub) to optimize the device performance since Tsub could influence the molecular packing, grain size, grain boundaries and defects in the thin film. The device performance of OTFTs based on BTDI-OCF3 and BTDI-CF was tested in a glovebox; the corresponding parameters are summarized in Table 2, and the data for devices tested in air are provided in the ESI.† Both of these materials showed unique electron transfer characteristics and the BTDI-CF based device exhibited higher mobility than BTDI-OCF3.
Table 2 Device performance summary of the OTFTs tested in a glovebox
|
T
sub/°C |
μ
e/cm2 V−1 s−1 |
I
on/Ioff |
V
th/V |
Average mobility.
Room temperature.
Highest mobility.
|
BTDI-OCF3
|
RTb |
6.47 × 10−3a (7.05 × 10−3)c |
103 |
−35 |
BTDI-OCF3
|
60 |
8.94 × 10−3 (9.77 × 10−3) |
104 |
−43 |
BTDI-OCF3
|
90 |
9.21 × 10−3 (1.11 × 10−2) |
104 |
−50 |
BTDI-CF
|
RT |
1.50 × 10−2 (1.58 × 10−2) |
105 |
−50 |
BTDI-CF
|
50 |
3.58 × 10−2 (3.72 × 10−2) |
106 |
−33 |
BTDI-CF
|
80 |
3.68 × 10−2 (3.84 × 10−2) |
107 |
−15 |
We observed that when Tsub is increased, the mobility of both materials is enhanced; the mobility of BTDI-OCF3 showed a slight dependence on the Tsub, while that of BTDI-CF increased 2-fold at Tsub = 80 °C. This will be further explained by the thin film morphological study. Interestingly, the threshold voltage (Vth) of the devices based on these materials showed a contrast shift trend with an increase in the Tsub, as can be observed from the transfer curves (Fig. 2). The Id1/2–Vg curves of the fabricated devices are close to linear, indicating that the mobility is independent of the gate voltage when the influence of water and oxygen is eliminated, which is ideal for logic circuits. The Ion/Ioff ratios of both devices are improved since Ioff decreased with an increase in the Tsub. Overall, the device performance is observed to be enhanced by elevating the Tsub.
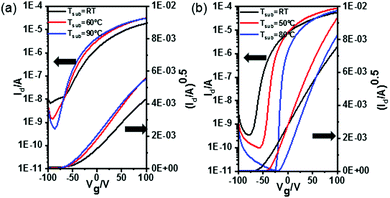 |
| Fig. 2 Transfer characteristics of (a) BTDI-BOCF3 and (b) BTDI-CF based OTFTs at different Tsub. | |
The stability of the fabricated devices is investigated under different conditions. The electrical stability of the OTFTs is evaluated by a successive scan process in a glovebox. As can be seen in Fig. 3a and c, the drain–source current of BTDI-OCF3 slightly increased during the scan process, while Vth gradually shifted in the negative direction. This improvement in Id could be attributed to the reduced electron trap density during constant scanning. For the BTDI-CF based device, after 100 cycles of scanning, the Id–Vg curve almost remained unchanged, which showed superior stability of the device performance under N2. This certified the remarkable operational stability of the BTDI-CF based device in N2 and also indicated that the device would potentially work stably for a long time upon encapsulating it effectively. Moreover, the air-stability is also investigated by measuring the device in an ambient environment. The performance of the OTFTs tested in air initially decreased a little compared with those tested in a glovebox, illustrating that the fluoro-alkyl could protect the device against oxygen and moisture to a certain extent. However, the device performance degraded gradually with time when stored in air since oxygen and moisture could slowly diffuse into the active layer. Vth of both of the devices gradually shifted in the positive direction when stored in air, which was induced by the reaction of oxygen with residual –OH in the dielectric layer.41 Moreover, oxygen and water caused extra traps for electron transfer, which led to a drop in the mobility. The devices could still maintain n-type characteristics comparable to its initial behavior for a long time exposure to air. The scope of degradation of BTDI-CF was a little larger since the three methane groups (–CH2–) negatively influenced the dense packing of the perfluorooctyl, but the device performance finally stabilized after ten days, as displayed in Table S1 (ESI†). Furthermore, OTFTs based on both BTDI-CF and BTDI-OCF3 exhibited almost no hysteresis when tested in N2 and small hysteresis in air; however, the BTDI-C6 based device showed hysteresis in N2 and much larger hysteresis in air with a higher decay of Ion (Fig. 3). In general, the hysteresis is mainly caused by water and oxygen. To our knowledge, the fluro-alkyl substituted BTDIs could exhibit densely packed molecular structures, which prevent water and oxygen from penetrating into the active layer, thereby reducing the hysteresis in the corresponding devices. This significant improvement in the device performance compared with our previous work39 demonstrates that –C3H6C8F17 and p-(trifluoromethoxy)benzyl can kinetically protect the device performance (Fig. 4).
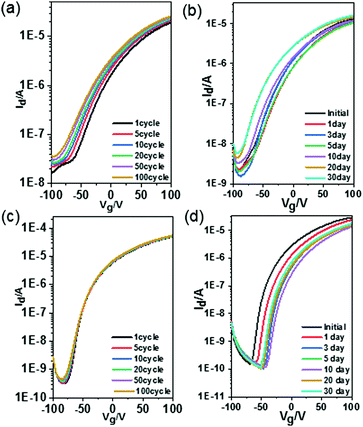 |
| Fig. 3 Operational stability of (a) BTDI-OCF3 and (c) BTDI-CF based OTFTs under N2. Transfer curves of (b) BTDI-OCF3 and (d) BTDI-CF based OTFTs stored and tested in air. | |
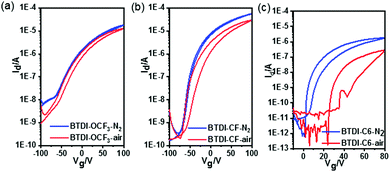 |
| Fig. 4 Transfer characteristics of (a) BTDI-OCF3, (b) BTDI-CF and (c) BTDI-C6 based OTFTs under different testing conditions. | |
Structural and morphological analysis of thin films
It is widely accepted that the morphology and the microstructure of thin-films are the key factors affecting the charge mobility. To understand the mobility difference between BTDI-CF and BTDI-OCF3 at various Tsub, we analyzed the morphologies of the corresponding thin films by atomic force microscopy (AFM) and X-ray diffraction (XRD). The out-of-plane XRD patterns of BTDI thin films deposited at different Tsub are shown in Fig. 5. For BTDI-OCF3, diffraction peaks up to the 4th order were observed at 2θ = 4.6°, which correspond to (001) reflections according to the single-crystal data. The interlayer distance (d-spacing) estimated from the primary peak at 2θ = 4.6° is 1.93 nm, which is close to the unit cell vector c-axis of 1.97 nm obtained from single crystals. The molecular length of BTDI-OCF3 (Fig. S4, ESI†) is 2.2 nm, which is similar to the step-height of the AFM image (Fig. S5, ESI†). These results indicate that BTDI-OCF3 molecules are oriented vertically with respect to the OTS-treated SiO2/Si substrate. Generally, this kind of molecular orientation in thin films on the substrate is beneficial for carrier transport. Besides, the intensity of the diffraction peaks exhibited a slight enhancement as the Tsub was increased from RT to 90 °C, indicating improved thin-film quality. This is consistent with the experimentally observed mobility of the BTDI-OCF3 based OTFTs (see Table 2). In contrast, diffraction peaks of the BTDI-CF thin films up to the 3rd order are clearly visible and the d-spacing obtained from the primary peak at 2θ = 2.86° is 3.07 nm, which corresponds to its longer molecular length, as evident in Fig. S4 (ESI†). Obviously, the d-spacing of BTDI-CF is larger than that of BTDI-OCF3, which is attributed to its long fluoroalkyl chain. As expected, a slight increase in the peak intensity of the BTDI-CF thin films is observed after increasing the Tsub, which is in good agreement with the mobility observations of the BTDI-CF based OTFTs.
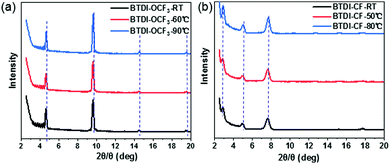 |
| Fig. 5 XRD patterns of (a) BTDI-OCF3 and (b) BTDI-CF thin films deposited on OTS-treated SiO2/Si at different Tsub. | |
In addition, the temperature-dependent thin film morphologies of BTDI-OCF3 and BTDI-CF thin films were studied by AFM, as shown in Fig. 6. Among them, BTDI-OCF3 showed a well-defined terrace structure in thin-films with good crystallinity, demonstrating a remarkable improvement in the morphology upon increasing the deposition temperature. As displayed in Fig. 6a–c, the step-heights of the terraces were measured to be approximately 2.2 nm, which is in accord with the corresponding molecular length (Fig. S4, ESI†). These observations strongly support the aforementioned XRD analysis of the molecular arrangement on the substrate. Moreover, the grain size was significantly increased with increasing the Tsub. In general, well-defined thin-films with large grain size and good crystallinity are beneficial for efficient charge transport, suggesting that the mobility of BTDI-OCF3 based OTFTs should increase with an increase in the Tsub. The thin-film morphology of BTDI-CF was observed to be considerably different from that of BTDI-OCF3. The BTDI-CF thin-film exhibited a wormlike structure with a grain size of 100–200 nm in length, as shown in Fig. 6d. In this case, Tsub only exhibited a slight effect on the thin-film morphology. As depicted in Fig. 6e, the grain size was slightly increased after increasing the Tsub from RT to 50 °C. However, the film morphology hardly changed after continuously raising Tsub (Fig. 6f), indicating that the device performance would remain unchanged at higher deposition temperatures. As expected, the above investigations are consistent with the observed mobility tendency of BTDI-OCF3 and BTDI-CF based OTFTs, as summarized in Table 2.
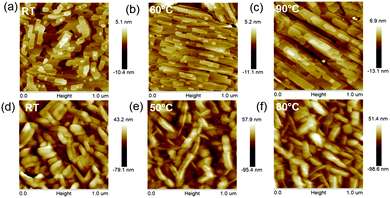 |
| Fig. 6 AFM images of (a–c) BTDI-OCF3 and (d–f) BTDI-CF thin-films deposited at different Tsub. | |
Conclusions
In summary, we have successfully synthesized two kinds of fluoro-alkyl substituted isothianaphthene materials, BTDI-OCF3 and BTDI-CF. Both of these BTDI derivatives have similar LUMO energy levels and optical properties since the substituent on the nitrogen atom does not greatly affect the LUMO energy. However, the molecular packing and thin film morphologies are quite different due to the significant difference of the alkyl-chain lengths. This further influenced the device performance; the BTDI-CF based OTFT showed higher mobility (0.0368 cm2 V−1 s−1) with excellent operational stability in a glovebox, while the BTDI-OCF3 based device demonstrated highly ordered molecular packing, which resulted in enhanced air-stability. Notably, the hysteresis phenomenon and current decay tested in air compared with those under N2 of the OTFTs based on both of these materials exhibited great improvement as compared to the BTDI-C6 reported in our previous work, indicating better stability of these newly developed fluoric BTDI derivatives. Moreover, the solubility of these compounds is much higher than the NDI analogues with the same substituent. Further investigation of solution processing of OTFTs based on these materials is underway in our lab.
Conflicts of interest
There are no conflicts to declare.
Acknowledgements
This work was financially supported by Shenzhen Science and Technology Research Grant (JCYJ20170412151139619, JCYJ20190808175216899 and JCYJ20190808182803805), Key-Area Research and Development Program of Guangdong Province (2019B010924003), NSFC-RS International Exchanges Programme (5181102182), Natural Science Foundation of Guangdong Province (2020A1515010449), Special Foundation for Science and Technology of Guangdong Province (2020S0007), and Guangdong Academician Expert Enterprise Workstation of Guangdong CNS New Material Technology Co., Ltd.
Notes and references
- A. F. Paterson, S. Singh, K. J. Fallon, T. Hodsden, Y. Han, B. C. Schroeder, H. Bronstein, M. Heeney, I. McCulloch and T. D. Anthopoulos, Recent Progress in High-Mobility Organic Transistors: A Reality Check, Adv. Mater., 2018, 30, 1801079 CrossRef PubMed.
- J. Yang, Z. Zhao, S. Wang, Y. Guo and Y. Liu, Insight into High-Performance Conjugated Polymers for Organic Field-Effect Transistors, Chem, 2018, 4, 2748–2785 CAS.
- A. C. Arias, J. D. MacKenzie, I. McCulloch, J. Rivnay and A. Salleo, Materials and applications for large area electronics: Solution-based approaches, Chem. Rev., 2010, 110, 3–24 CrossRef CAS PubMed.
- C. Wang, H. Dong, W. Hu, Y. Liu and D. Zhu, Semiconducting π-conjugated systems in field-effect transistors: A material odyssey of organic electronics, Chem. Rev., 2012, 112, 2208–2267 CrossRef CAS PubMed.
- Z. Lv, Y. Zhou, S. Han and V. A. L. Roy, From biomaterial-based data storage to bio-inspired artificial synapse, Mater. Today, 2018, 21, 537–552 CrossRef CAS.
- A. Tsumura, H. Koezuka and T. Ando, Macromolecular electronic device: Field-effect transistor with a polythiophene thin film, Appl. Phys. Lett., 1986, 49, 1210–1212 CrossRef CAS.
- H. Minemawari, T. Yamada, H. Matsui, J. Y. Tsutsumi, S. Haas, R. Chiba, R. Kumai and T. Hasegawa, Inkjet printing of single-crystal films, Nature, 2011, 475, 364–367 CrossRef CAS PubMed.
- I. Vladimirov, M. Kellermeier, T. Geßner, Z. Molla, S. Grigorian, U. Pietsch, L. S. Schaffroth, M. Kühn, F. May and R. T. Weitz, High-mobility, ultrathin organic semiconducting films realized by surface-mediated crystallization, Nano Lett., 2018, 18, 9–14 CrossRef CAS PubMed.
- G. Schweicher, V. Lemaur, C. Niebel, C. Ruzié, Y. Diao, O. Goto, W.-Y. Lee, Y. Kim, J. B. Arlin, J. Karpinska, A. R. Kennedy, S. R. Parkin, Y. Olivier, S. C. B. Mannsfeld, J. Cornil, Y. H. Geerts and Z. Bao, Bulky end-capped [1]benzothieno[3,2-b]benzothiophenes: Reaching high-mobility organic semiconductors by fine tuning of the crystalline solid-state order, Adv. Mater., 2015, 27, 3066–3072 CrossRef CAS PubMed.
- Y. Xiong, J. Tao, R. Wang, X. Qiao, X. Yang, D. Wang, H. Wu and H. Li, A Furan–Thiophene-Based Quinoidal Compound: A New Class of Solution-Processable High-Performance n-Type Organic Semiconductor, Adv. Mater., 2016, 28, 5949–5953 CrossRef CAS PubMed.
- H. R. Tseng, H. Phan, C. Luo, M. Wang, L. A. Perez, S. N. Patel, L. Ying, E. J. Kramer, N. Thuc-Quyen, G. C. Bazan and A. J. Heeger, High-mobility field-effect transistors fabricated with macroscopic aligned semiconducting polymers, Adv. Mater., 2014, 26, 2993–2998 CrossRef CAS PubMed.
- J. Dhar, U. Salzner and S. Patil, Trends in molecular design strategies for ambient stable n-channel organic field effect transistors, J. Mater. Chem. C, 2017, 5, 7404–7430 RSC.
- X. Gao and Y. Hu, Development of n-type organic semiconductors for thin film transistors: A viewpoint of molecular design, J. Mater. Chem. C, 2014, 2, 3099–3117 RSC.
- A. R. Murphy and J. M. J. Fréchet, Organic semiconducting oligomers for use in thin film transistors, Chem. Rev., 2007, 107, 1066–1096 CrossRef CAS PubMed.
- L. L. Chua, J. Zaumseil, J. F. Chang, E. C. W. Ou, P. K. H. Ho, H. Sirringhaus and R. H. Friend, General observation of n-type field-effect behaviour in organic semiconductors, Nature, 2005, 434, 194 CrossRef CAS PubMed.
- G. R. Hutchison, M. A. Ratner and T. J. Marks, Intermolecular charge transfer between heterocyclic oligomers. Effects of heteroatom and molecular packing on hopping transport in organic semiconductors, J. Am. Chem. Soc., 2005, 127, 16866–16881 CrossRef CAS PubMed.
- Y. Chang, M. Kuo, C. Chen, H. Lu and I. Chao, On the air stability of n-channel organic field-effect transistors: A theoretical study of adiabatic electron affinities of organic semiconductors, J. Mater. Chem. C, 2010, 114, 11595–11601 CAS.
- H. Usta, C. Risko, Z. Wang, H. Huang, M. K. Deliomeroglu, A. Zhukhovitskiy, A. Facchetti and T. J. Marks, Design, synthesis, and characterization of ladder-type molecules and polymers. Air-stable, solution-processable n-channel and ambipolar semiconductors for thin-film transistors via experiment and theory, J. Am. Chem. Soc., 2009, 131, 5586–5608 CrossRef CAS PubMed.
- H. E. Katz, A. J. Lovinger, J. Johnson, C. Kloc, T. Siegrist, W. Li, Y. Y. Lin and A. Dodabalapur, A soluble and air-stable organic semiconductor with high electron mobility, Nature, 2000, 404, 478 CrossRef CAS PubMed.
- X. Guo, A. Facchetti and T. J. Marks, Imide- and amide-functionalized polymer semiconductors, Chem. Rev., 2014, 114, 8943–9021 CrossRef CAS PubMed.
- J. D. Yuen, V. A. Pozdin, A. T. Young, B. L. Turner, I. D. Giles, J. Naciri, S. A. Trammell, P. T. Charles, D. A. Stenger and M. A. Daniele, Perylene-diimide-based n-type semiconductors with enhanced air and temperature stable photoconductor and transistor properties, Dyes Pigm., 2020, 174, 108014 CrossRef CAS.
- O. A. Melville, T. M. Grant and B. H. Lessard, Silicon phthalocyanines as N-type semiconductors in organic thin film transistors, J. Mater. Chem. C, 2018, 6, 5482–5488 RSC.
- S. M. Swick, T. Gebraad, L. Jones, B. Fu, T. J. Aldrich, K. L. Kohlstedt, G. C. Schatz, A. Facchetti and T. J. Marks, Building Blocks for High-Efficiency Organic Photovoltaics: Interplay of Molecular, Crystal, and Electronic Properties in Post-Fullerene ITIC Ensembles, ChemPhysChem, 2019, 20, 2608–2626 CrossRef CAS PubMed.
- C. Zhang, Y. Zang, E. Gann, C. R. McNeill, X. Zhu, C.-A. Di and D. Zhu, Two-dimensional π-expanded quinoidal terthiophenes terminated with dicyanomethylenes as n-type semiconductors for high-performance organic thin-film transistors, J. Am. Chem. Soc., 2014, 136, 16176–16184 CrossRef CAS PubMed.
- J. Dou, Y. Zheng, Z. Yao, Z. Yu, T. Lei, X. Shen, X. Luo, J. Sun, S. Zhang, Y. Ding, G. Han, Y. Yi, J. Wang and J. Pei, Fine-tuning of crystal packing and charge transport properties of BDOPV derivatives through fluorine substitution, J. Am. Chem. Soc., 2015, 137, 15947–15956 CrossRef CAS PubMed.
- N. A. Minder, S. Ono, Z. Chen, A. Facchetti and A. F. Morpurgo, Band-Like Electron Transport in Organic Transistors and Implication of the Molecular Structure for Performance Optimization, Adv. Mater., 2012, 24, 503–508 CrossRef CAS PubMed.
- B. A. Jones, A. Facchetti, M. R. Wasielewski and T. J. Marks, Tuning orbital energetics in arylene diimide semiconductors. Materials design for ambient stability of n-type charge transport, J. Am. Chem. Soc., 2007, 129, 15259–15278 CrossRef CAS PubMed.
- H. E. Katz, A. J. Lovinger, J. Johnson, C. Kloc, T. Siegrist, W. Li, Y. Y. Lin and A. Dodabalapur, A soluble and air-stable organic semiconductor with high electron mobility, Nature, 2000, 404, 478–481 CrossRef CAS PubMed.
- R. Schmidt, J. H. Oh, Y.-S. Sun, M. Deppisch, A.-M. Krause, K. Radacki, H. Braunschweig, M. Könemann, P. Erk, Z. Bao and F. Würthner, High-performance air-stable n-channel organic thin film transistors based on halogenated perylene bisimide semiconductors, J. Am. Chem. Soc., 2009, 131, 6215–6228 CrossRef CAS PubMed.
- B. J. Jung, J. Sun, T. Lee, A. Sarjeant and H. E. Katz, Low-temperature-processible, transparent, and air-operable n-channel fluorinated phenylethylated naphthalenetetracarboxylic diimide semiconductors applied to flexible transistors, Chem. Mater., 2009, 21, 94–101 CrossRef CAS.
- K. C. See, C. Landis, A. Sarjeant and H. E. Katz, Easily synthesized naphthalene tetracarboxylic diimide semiconductors with high electron mobility in air, Chem. Mater., 2008, 20, 3609–3616 CrossRef CAS.
- B. J. Jung, K. Lee, J. Sun, A. G. Andreou and H. E. Katz, Air-Operable, High-Mobility Organic Transistors with Semifluorinated Side Chains and Unsubstituted Naphthalenetetracarboxylic Diimide Cores: High Mobility and Environmental and Bias Stress Stability from the Perfluorooctylpropyl Side Chain, Adv. Funct. Mater., 2010, 20, 2930–2944 CrossRef CAS.
- D. Zhang, L. Zhao, Y. Zhu, A. Li, C. He, H. Yu, Y. He, C. Yan, O. Goto and H. Meng, Effects of p-(trifluoromethoxy)benzyl and p-(trifluoromethoxy)phenyl molecular architecture on the performance of naphthalene tetracarboxylic diimide-based air-stable n-type semiconductors, ACS Appl. Mater. Interfaces, 2016, 8, 18277–18283 CrossRef CAS PubMed.
- T. He, M. Stolte, C. Burschka, N. H. Hansen, T. Musiol, D. Kälblein, J. Pflaum, X. Tao, J. Brill and F. Würthner, Single-crystal field-effect transistors of new Cl2-NDI polymorph processed by sublimation in air, Nat. Commun., 2015, 6, 5954 CrossRef CAS PubMed.
- C. Liu, C. Xiao, Y. Li, W. Hu, Z. Li and Z. Wang, High performance, air stable n-type single crystal transistors based on core-tetrachlorinated perylene diimides, Chem. Commun., 2014, 50, 12462–12464 RSC.
- T. He, M. Stolte and F. Wuerthner, Air-Stable n-Channel Organic Single Crystal Field-Effect Transistors Based on Microribbons of Core-Chlorinated Naphthalene Diimide, Adv. Mater., 2013, 25, 6951–6955 CrossRef CAS PubMed.
- J. D. Heer, The electronic structure of conjugated sulfur compounds. I. Thiophene and isothianaphthene, J. Am. Chem. Soc., 1954, 76, 4802–4806 CrossRef.
- F. Wudl, M. Kobayashi and A. J. Heeger, Poly(isothianaphthene), J. Org. Chem., 1984, 49, 3382–3384 CrossRef CAS.
- X. Chen, Y. He, M. U. Ali, Y. He, Y. Zhu, A. Li, C. Zhao, I. F. Perepichka and H. Meng, Isothianaphthene diimide: an air-stable n-type semiconductor, Sci. China: Chem., 2019, 62, 1360–1364 CrossRef CAS.
- C. P. Yu, R. Kimura, T. Kurosawa, E. Fukuzaki, T. Watanabe, H. Ishii, S. Kumagai, M. Yano, J. Takeya and T. Okamoto, Air-Stable Benzo[c]thiophene Diimide n-Type π-Electron Core, Org. Lett., 2019, 21, 4448–4453 CrossRef CAS PubMed.
- D. Kumaki, T. Umeda and S. Tokito, Influence of H2O and O2 on threshold voltage shift in organic thin-film transistors: Deprotonation of SiOH on SiO2 gate-insulator surface, Appl. Phys. Lett., 2008, 92, 093309 CrossRef.
Footnote |
† Electronic supplementary information (ESI) available. CCDC 1998826. For ESI and crystallographic data in CIF or other electronic format see DOI: 10.1039/d0qm00137f |
|
This journal is © the Partner Organisations 2020 |