DOI:
10.1039/D0QI00792G
(Research Article)
Inorg. Chem. Front., 2020,
7, 4070-4076
Constructing [CoII6] hexagon-centered heterometallic {Ln6Co6} (Ln = Y, Eu and Dy) clusters with a calix[8]arene ligand†
Received
4th July 2020
, Accepted 2nd September 2020
First published on 7th September 2020
Abstract
Three isostructural 3d–4f polynuclear clusters {(LnIII6CoII6)(C8A)3} (Ln = Y (CIAC-248), Eu (CIAC-249), Dy (CIAC-250); C8A = p-tert-butylcalix[8]arene) were synthesized under solvothermal conditions. These three compounds feature some tripod-like entities in which three Ln2-C8A secondary building units acting as the legs bolster a [Co6] hexagon. Magnetic study indicated that the complex {(EuIII6CoII6)(C8A)3} (CIAC-249) exhibits spin glass behaviour at ≈9 K, and the complex {(DyIII6CoII6)(C8A)3} (CIAC-250) shows slow relaxation of magnetization.
Introduction
Heterometallic 3d–4f clusters have attracted considerable attention due to their fascinating structures, interesting magnetic properties such as single-molecule magnet (SMM) behaviour, and potential applications in high-density information storage and quantum computing.1–3 Commonly, the syntheses of these types of clusters are based on some shielded or multidentate ligands which can act as the magnetic coupling mediator. Calixarenes have been proven to be excellent multidentate ligands to construct single-molecule magnets due to their polyphenolic nature, which can not only induce ferromagnetic or antiferromagnetic coupling efficiently but also assume diverse coordination modes. To date, there have been some reports on the construction of paramagnetic molecular clusters with calixarenes. For example, Dalgarno and Brechin4–7 applied methylene-bridged calix[4]arene in the construction of SMMs and magnetic coolers. We8,9 and others10–13 used thia- and sulfonyl-bridged calix[4]arenes for the preparation of polynuclear clusters possessing interesting magnetic properties. However, to the best of our knowledge, polynuclear clusters based on larger calixarenes such as p-tert-butylcalix[8]arene with SMM properties and ferromagnetic ordering behaviours are rare.14–16 Compared to calix[4]arene, calix[8]arene, which has more coordination sites, variable conformation and higher flexibility, may generate novel materials with interesting properties.17,18 In addition, a carbonate anion is also a good bridge due to its various bridging modes, such as μ2-CO32−,19,20 μ3-CO32−,21,22 μ4-CO32−,23,24 and μ6-CO32−.25–27 Furthermore, we found that calix[8]arene adopting a double cone conformation can form a Ln2-C8A secondary building unit (SBU) when reacting with lanthanide ions and the Ln2-C8A SBUs can bond some transition metals (TM) to form heterometallic 3d–4f clusters. Here, we present the crystal structures and magnetic properties of three {(LnIII6CoII6)(C8A)3} compounds (Ln = Y (CIAC-248), Eu (CIAC-249), Dy (CIAC-250); C8A = p-tert-butylcalix[8]arene) incorporating a central μ6-carbonate anion. These compounds exhibit ferromagnetic long-range ordering or SMM properties.
Experimental
Starting materials
All starting materials were of analytical reagent grade and used as received without further purification. p-tert-Butylcalix[8]arene was prepared according to the literature method.28
Syntheses of compounds CIAC-248–250
{[(Y6Co6)(C8A)3(CH3OH)5(CH3O−)3(CO32−)(DMF)6(CH3COO−)2]}·2.3H2O 0.35CH3OH·[(C2H5)3NH+] (CIAC-248).
Blue block crystals were obtained by the solvothermal reaction of a mixture of C8A (0.065 g, 0.04 mmol), Co(CH3COO)2·4H2O (0.038 g, 0.15 mmol), Y(CH3COO)3·6H2O (0.04 g, 0.39 mmol), CH3OH (6 mL), triethylamine (1.1 mL) and several drops of DMF in a 20 mL Teflon-lined autoclave which was kept at 150 °C for 3 days and then slowly cooled to room temperature at about 4 °C h−1. The crystals were isolated by filtration and washed with methanol and dried under air. Yield: 36% with respect to Y. Elemental analysis (%): calcd for [C292H390Y6Co6N6O45] (excluding disordered solvent molecules): C, 62.73; N, 1.50; H, 6.98. Found: C, 62.23; N, 1.31; H, 6.31. FT-IR (KBr pellet, cm−1): 3527(w), 2955(s), 1650(s), 1569(w), 1454(s), 1387(m), 1360(m), 1293(s), 1212(s), 1125(w), 1044(m), 909(w), 875(w), 822(m), 754(m), 674(w).
{[(Eu6Co6)(C8A)3(CH3OH)5(CH3O−)3(CO32−)(DMF)6(CH3COO−)2]}·2H2O·[(C2H5)3NH+] (CIAC-249).
The procedure was similar to the synthesis of CIAC-249 except that Y(CH3COO)3·6H2O was substituted with Eu(CH3COO)3·6H2O. The crystals were isolated as blue block crystals in 32% yield based on Eu. Elemental analysis (%): calcd [C292H390Eu6Co6N6O45] (excluding disordered solvent molecules): C, 58.76; N, 1.41; H, 6.54. Found: C, 58.49; N, 1.32; H, 6.65. FT-IR (KBr pellet, cm−1): 3528(w), 2953(s), 1656(s), 1466(s), 1396(m), 1358(m), 1293(s), 1207(s), 1125(w), 1027(m), 908(w), 876(w), 821(m), 745(m), 685(w).
{[(Dy6Co6)(C8A)3(CH3OH)5(CH3O−)3(CO32−)(DMF)6(CH3COO−)2]}·3H2O·CH3OH·[(C2H5)3NH+] (CIAC-250).
The procedure was similar to the synthesis of CIAC-248 except that Y(CH3COO)3·6H2O was substituted by Dy(CH3COO)3·6H2O. The crystals were isolated as blue block crystals in 32% yield based on Dy. Elemental analysis (%): calcd for [C292H390Dy6Co6N6O45] (excluding disordered solvent molecules): C, 58.14; N, 1.39; H, 6.49. Found: C, 58.02; N, 1.28; H, 6.39. FT-IR (KBr pellet, cm−1): 3528(w), 2953(s), 1656(s), 1467(s), 1396(m), 1358(m), 1293(s), 1207(s), 1119(w), 1038(m), 914(w), 870(w), 815(m), 750(m), 674(w).
X-ray structure determination
The X-ray intensity data for compounds CIAC-248–250 were collected on a Bruker D8 QUEST system with Cu Kα radiation (λ = 0.15418 nm) operated at 50 W (50 kV, 1 mA). The crystal structures were solved by means of direct methods and refined employing full-matrix least squares on F2 (SHELXTL-97).29 The large R1 and wR2 factors of compounds CIAC-248–250 might be due to the weak high-angle diffractions and the disorder of p-tert-butyl groups. Hydrogen atoms of the organic ligands were generated theoretically onto the specific atoms and refined isotropically with fixed thermal factors. CCDC 2013506–2013508† contain the supplementary crystallographic data for this paper.
Physical measurements
Elemental analysis of C, N, and H was performed using a Vario EL instrument. TGA measurement was performed using a NETZSCH STA 449F3. FT-IR spectra were obtained with a Bruker Vertex 70 spectrometer. Magnetic susceptibility measurements were carried out with a Quantum Design MPMS-XL7 SQUID magnetometer. Freshly prepared crystalline samples were embedded in eicosane to avoid any field-induced crystal reorientation. Diamagnetic corrections were applied for eicosane and for the molecule, the latter being calculated from the Pascal constants. X-ray powder diffraction (XRD) measurements were performed on a D8 Focus diffractometer (Bruker) ranging from 0°–40° with Cu Kα radiation (λ = 0.15418 nm).
Results and discussion
Single-crystal X-ray diffraction analysis reveals that compounds CIAC-248–250 are isostructural and feature a tripod-like unit composed of a wrapped {Co6} hexagon and three Ln2-C8A SBUs (Fig. 1). So compound CIAC-250 is described in detail as an example. Complex CIAC-250 crystallizes in the monoclinic system with the space group C2/c. In the structure, three p-tert-butylcalix[8]arene molecules adopt the double cone conformation. Each conic subunit of the calix[8]arene molecule binds a Dy atom through its oxygen atoms at the lower rim so that a Dy2-C8A secondary building unit (SBU) is formed, which is a common unit found in the compounds of 4f/5f metals and calix[8]arene. Six adjacent Co atoms are bonded to the same μ6-CO32− anion in situ generated from the decomposition of DMF to form a {Co6} hexagonal plate. Furthermore, this hexagon is bolstered by three Dy2-C8A SBUs to form a tripod-like {(LnIII6CoII6)(C8A)3} entity (Fig. 2 and 3). It should be noted that all three calix[8]arene molecules in an isolated entity are nearly perpendicular to the planar {Co6} hexagon (Fig. S3†). The extended structure of compound CIAC-250 is stacked by these dodecanuclear heterometallic units through supramolecular interactions (Fig. S4†).
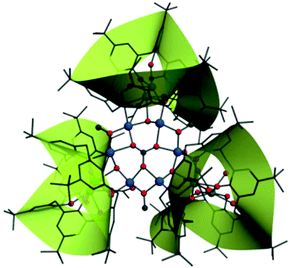 |
| Fig. 1 Molecular structure of compound CIAC-250. Hydrogen atoms and solvent molecules are omitted for clarity. Blue: Co atoms; green: Dy atoms; red: O atoms; gray: C atoms. | |
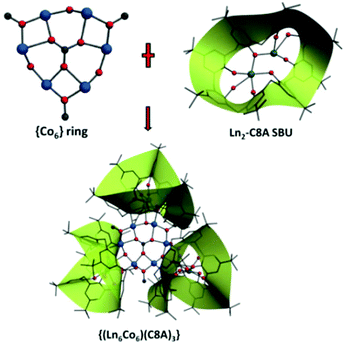 |
| Fig. 2 Scheme of the assembly of compound CIAC-250. | |
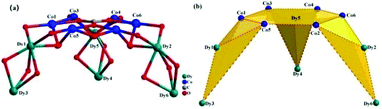 |
| Fig. 3 Arrangement of the metal ions of compound CIAC-250. | |
For the LnIII6CoII6 core, there are twelve crystallographically independent metal sites which have three types of coordination. Each Co atom is five coordinated in a distorted trigonal bipyramid coordination geometry. The coordination geometries of Dy(III) ions were calculated by SHAPE analysis (Table S2†).30,31 Site Dy1 is seven coordinated in a capped octahedron with C3v local symmetry. Sites Dy2, Dy4 and Dy5 are also seven coordinated, but in a capped trigonal prism with C2v local symmetry. Sites Dy3 and Dy6 are eight coordinated in a biaugmented trigonal prism J50 with C2v local symmetry. The distances of the Dy–O and Co–O bonds are in the range of 2.18–2.89 Å and 1.93–2.13 Å, respectively. The O–Dy–O and O–Co–O angles fall in the ranges of 52.7–159.7° and 74.8–178.6°. It is noted that the 3d–4f heterometallic compounds having a μ6-CO32− bridging-[Co6] ring are documented for the first time.
Magnetic properties
The variable-temperature direct current (dc) magnetic susceptibility data for all compounds were collected under a 1 kOe applied field at the temperature range of 2–300 K (Fig. 4), and the field (H) dependence of the magnetizations (M) for these three complexes was measured up to 7 T at low temperatures (Fig. S6–S8†). The χT value for CIAC-248 is 19.88 emu K mol−1 at room temperature, which is larger than the spin-only value (11.25 emu K mol−1) expected for six uncoupled Co(II) ions (S = 3/2, g = 2.0), owing to the contribution from the orbital angular momentum. Upon cooling, the χT value decreases slowly and reaches 5.75 emu K mol−1 at 2 K, presumably corresponding to the depopulation of higher Kramers doublets of the Co(II) centers and possible antiferromagnetic coupling between Co(II) ions. The magnetization for CIAC-248 increases slowly with the field and reaches a maximum value of 6.30 μB at 2 K and 7 T.
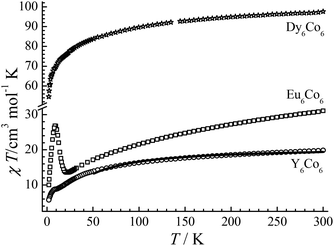 |
| Fig. 4 The χT versus T plots for CIAC-248, 249 and 250 under a 1 kOe dc field. The solid line is the best fit. | |
For CIAC-249, although the Eu(III) ion (J = 0, 7F0) possesses a diamagnetic ground state, the χT value at 300 K (31.10 emu K mol−1) is much larger than that of CIAC-248. Such a large value is due to a certain amount of magnetic moment from the excited states (e.g., 7F1) of the Eu(III) ion. The χT value is about 1.87 emu K mol−1 for each Eu ion at room temperature based on complex CIAC-248, which is close to the reported value.32 The χT values decrease slowly as the temperature decreases to 12.56 emu K mol−1 at 22 K, followed by a sharp increase to a maximum value of 26.81 emu K mol−1 at 8.7 K, which indicates ferromagnetic interactions in the {Eu6Co6} cluster, and then drop to 10.03 emu K mol−1 at 2 K. The field dependence of magnetizations for CIAC-249 gives a small value of 5.98 μB at 2 K and 7 T, which is close to those of CIAC-248, in accordance with the diamagnetic ground state of Eu(III) ions.
For CIAC-250, the χT value at room temperature (97.56 emu K mol−1) is consistent with the expected value of six uncoupled Dy(III) ions (S = 5/2, L = 5, g = 4/3, 6H15/2) and six isolated Co(II) ions. The χT value is smaller than the expected value with the orbital angular momentum from Co(II) ions, owing to the antiferromagnetic coupling between Dy(III) and Co(II). The χT values decrease gradually from 300 to 50 K and much rapidly below 50 K. At 2 K, the χT value is 54.55 emu K mol−1 and the maximum M value is 34.82 μB at 7 T. This unsaturation M value indicates the presence of magnetic anisotropy and/or low-lying excited states in the system. The program PHI was used to fit the magnetic susceptibility of CIAC-248 with a minimal model (eqn (1)),33 which can reproduce the temperature-dependent susceptibility at the temperature range from 50 K to 300 K (Fig. 4) and provide a reasonable estimate of the intramolecular magnetic exchange coupling (J) between the Co(II) ions. The best simulation gives J = −3.15 cm−1 with g = 2.73. Attempts for fitting the lower temperature data failed, probably due to the interplay and competition of spin-orbital coupling and magnetic interactions. The alternating current (ac) susceptibility measurement of CIAC-248 at the frequency of 1488 Hz with a zero dc field displays a paramagnetic property (Fig. S9†), thus showing neither magnetic ordering nor slow relaxation of the magnetization above 2 K.
|  | (1) |
As complex CIAC-249 shows a peak at 8.7 K in its χT–T plots, further magnetic investigation was performed. The zero-field cooled (ZFC) and field-cooled (FC) magnetic susceptibility for CIAC-249 was recorded under a field of 50 Oe. Both the peak of the ZFC curve and the divergence of the ZFC and FC curves appear at about 9.2 K (Fig. 5), indicating a transition from a paramagnetic state to either a long-range ordering, a spin-glass state or a superparamagnetic state.34 Magnetic hysteresis measurements show a clear hysteresis loop at 2 K with a coercivity field of 580 Oe and a remnant magnetization of 0.38μB, and a closed hysteresis loop at 9 K, indicative of a magnet behavior (inset in Fig. 5 and Fig. S13†).
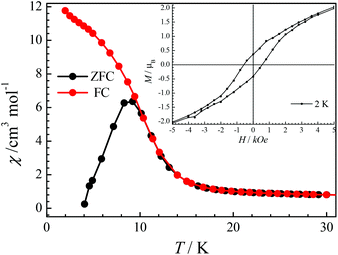 |
| Fig. 5
χ–T plots of CIAC-249 under field-cooled (FC) and zero-field-cooled (ZFC) conditions with a field of 50 Oe. Inset: Field-dependent magnetizations for complex CIAC-249 from 0 to 50 kOe at 2 K. | |
Ac magnetic susceptibility measurements for CIAC-249 were further carried out under a zero dc field. Wide peaks of the in-phase (χ′) and out-of-phase (χ′′) signals were observed between 8 K and 10 K with a very weak frequency dependence, supporting the existence of spin glassy behavior (Fig. 6). The parameter φ = (ΔTp/Tp)/(Δlog
f) was estimated as 0.02, which falls into the range of a spin glass and excludes a superparamagnet.35,36 We tried to fit the relaxation times at various temperatures via the Arrhenius law (τ = τ0
exp(Ueff/kBT), where τ0 is a pre-exponential factor and Ueff is the energy barrier), and obtained ΔE = 1176 K and τ0 = 2.43 × 10−57 s (Fig. S10†). This rather fast τ0 also excludes the possibility of an SMM. Those behaviours are similar to other Co clusters with spin glass behaviours.37,38 For CIAC-249, the spin glassy behavior probably is attributed to the weak magnetic moment from Eu(III) ions that modified the magnetic coupling between Co(II) ions. Every Eu2 C8A SUB bridges two Co(II) ions (Co2 dimer), and three Co2 dimers result in a triangular structure. Such a triangular geometry often leads to spin frustration with spin-glass ground state.
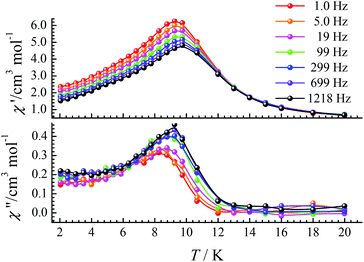 |
| Fig. 6 Temperature dependence of the in-phase (χ′) and out-of-phase (χ′′) ac susceptibility signals under a zero dc field at the indicated frequencies for CIAC-249. | |
The magnetic dynamics for CIAC-250 was studied using ac magnetic susceptibility measurements under a zero dc field. As shown in Fig. 7, χ′ signals diverge at nearly 30 K without reaching the maximum (except for high frequencies) and splitting tails of χ′′ signals were observed above 2 K, indicating the slow relaxation of magnetization, a characteristic SMM behaviour, but with the existence of fast quantum tunneling of magnetization (QTM). The parameter φ is calculated as 0.51, which is larger than the value for a spin glass, but close to a superparamagnetic behaviour. The relaxation times (τ) at different temperatures for CIAC-250 are evaluated from variable-frequency data via a generalized Debye model (Fig. S11 and S12†).39 The obtained ln(τ) values remain linear between 5.4 and 3.5 K, showing a thermally activated process, which can be fitted by the Arrhenius law, giving Ueff = 41.5 K and τ0 = 8.50 × 10−8 s (inset in Fig. 7). A slight deviation occurs as the temperature further decreases, which could be attributed to strong QTM at low temperature.
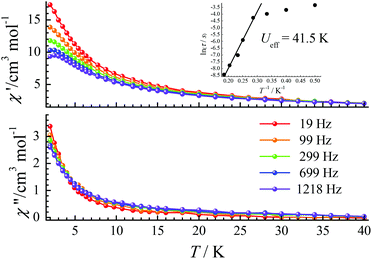 |
| Fig. 7 Temperature dependence of the in-phase (χ′) and out-of-phase (χ′′) ac susceptibility signals under a zero dc field at the indicated frequencies for CIAC-250. The lines are visual guides only. Inset: Plots of ln(τ) versus 1/T for CIAC-250. The solid line is the best fit. | |
To suppress the QTM, ac magnetic susceptibilities were measured under a dc field of 500 Oe from 2 K to 12 K (Fig. 8), which was fitted with a generalized Debye model (Fig. 9).35 The α parameters range from 0.50 to 0.64, indicating a wide contribution for the relaxation times. The obtained ln(τ) values remain linear above 6 K and with slight curvatures at low temperature, but do not become temperature independent. The linear regime at high temperature is often known as the Orbach relaxation process, while for low temperature it can be regarded as the Raman relaxation process because it is not temperature independent. So the Orbach process was combined with the Raman process to fit the relaxation times, giving C = 3.0(5) s−1 Kn, n = 2.6(1), τ0 = 3(2) × 10−6 s and Ueff = 56(9) K (Fig. 9).
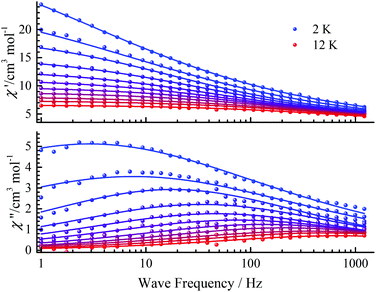 |
| Fig. 8 Frequency dependence of χ′ and χ′′ ac susceptibility signals under a 500 Oe dc field at the indicated temperatures for CIAC-250. The solid lines are the best fits. | |
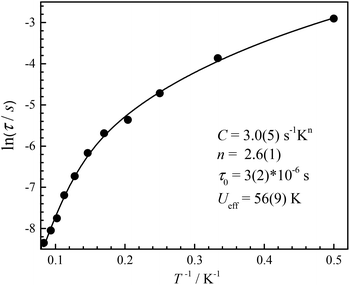 |
| Fig. 9 Plots of ln(τ) versus 1/T for CIAC-250 under a 500 Oe dc field. The solid line is the best fit. | |
Conclusions
In conclusion, we have synthesized {(LnIII6CoII6) (C8A)3} clusters featuring a wrapped [Co6] hexagon linked to three calix[8]arene molecules in a double-cone conformation. Interestingly, the {Eu6Co6} cluster shows spin glass behaviours below ≈ 9 K, while the {Dy6Co6} cluster exhibits SMM behaviour with Ueff of 41.5 K under a zero dc field (56(9) K under a 500 Oe dc field). This result emphasized that larger calix[n]arene (n = 6, 7, 8) with more coordination sites and larger molecules will provide further opportunities for controlling the self-assembly and tuning of the magnetic properties. Exploration of another transition metal, Ln, and mixed transition-metal/Ln clusters supported by methylene-bridged calix[8]arenes is underway to generate other novel functional materials.
Conflicts of interest
There are no conflicts to declare.
Acknowledgements
We thank the National Natural Science Foundation of China (no. 21971233, 21620102002, 21503155 and 21473129), the Science and Technology Development Plan of Jilin Province (20150520009JH), the “National 1000 Young Talents” Program, and the Wuhan National High Magnetic Field Center (2015KF06).
Notes and references
- L. R. Piquer, S. Dey, L. C. Amorós, S. J. Teat, J. Cirera, G. Rajaraman and E. C. Sañudo, Microwave assisted synthesis of heterometallic 3d–4f M4Ln complexes, Dalton Trans., 2019, 48, 12440 Search PubMed.
- V. Das, R. Kaushik and F. Hussain, Heterometallic 3d-4f polyoxometalates: An emerging field with structural diversity to multiple applications, Coord. Chem. Rev., 2020, 143, 213271 Search PubMed.
- J. Wang, M. Feng, M. N. Akhtar and M. L. Tong, Recent advance in heterometallic nanomagnets based on TMxLn4−x cubane subunits, Coord. Chem. Rev., 2019, 387, 129 Search PubMed.
- G. Karotsis, S. J. Teat, W. Wernsdorfer, S. Piligkos, S. J. Dalgarno and E. K. Brechin, Calix[4]arene-Based Single-Molecule Magnets, Angew. Chem., Int. Ed., 2009, 48, 8285 Search PubMed.
- G. Karotsis, S. Kennedy, S. J. Teat, C. M. Beavers, D. A. Fowler, J. J. Morales, M. Evangelisti, S. J. Dalgarno and E. K. Brechin, [MnIII4LnIII4] calix[4]arene clusters as enhanced magnetic coolers and molecular magnets, J. Am. Chem. Soc., 2010, 132, 12983 Search PubMed.
- G. Karotsis, M. Evangelisti, S. J. Dalgarno and E. K. Brechin, A calix[4]arene 3d/4f magnetic cooler, Angew. Chem., Int. Ed., 2009, 48, 9928 Search PubMed.
- S. M. Taylor, G. Karotsis, R. D. McIntosh, S. Kennedy, S. J. Teat, C. M. Beavers, W. Wernsdorfer, S. Piligkos, S. J. Dalgarno and E. K. Brechin, A family of calix[4]arene-supported [MnIII2MnII2] clusters, Chem. – Eur. J., 2011, 17, 7521 Search PubMed.
- Y. F. Bi, X. T. Wang, B. W. Wang, W. P. Liao, X. F. Wang, H. J. Zhang, S. Gao and D. Q. Li, Two MnII2LnIII4 (Ln=Gd, Eu) hexanuclear compounds of p-tert-butylsulfinylcalix[4]arene, Dalton Trans., 2009, 2250 Search PubMed.
- Y. F. Bi, Y. L. Li, W. P. Liao, H. J. Zhang and D. Q. Li, A unique Mn2Gd2 tetranuclear compound of p-tert-butylthiacalix [4]arene, Inorg. Chem., 2008, 47, 9733 Search PubMed.
- C. M. Liu, D. Q. Zhang, X. Hao and D. B. Zhu, Syntheses, crystal structures, and magnetic properties of two p-tert-butylsulfonylcalix[4]arene-supported cluster complexes with a totally disordered Ln4(OH)4 cubane core, Cryst. Growth Des., 2012, 12, 2948 Search PubMed.
- R. Zairov, S. Pizzanelli, A. P. Dovzhenko, I. Nizameev, A. Orekhov, N. Arkharova, S. N. Podyachev and S. Sudakova, Paramagnetic relaxation enhancement in hydrophilic colloids based on Gd(III) complexes with tetrathia-and calix[4]arenes, J. Phys. Chem., 2020, 124(7), 4320 Search PubMed.
- J. Y. Ge, X. Duan, J. P. Ma, S. Huang, J. Du and P. Wang, Thiacalix[4]arene-supported tetranuclear TbIII and EuIII compounds: Synthesis, structure, luminescence, and magnetism, Z. Anorg. Allg. Chem., 2019, 645, 416 Search PubMed.
- R. O. Fuller, G. A. Koutsantonis and M. I. Ogden, Magnetic properties of calixarene-supported metal coordination clusters, Coord. Chem. Rev., 2020, 402, 213066 Search PubMed.
- R. McLellan, S. M. Taylor, R. D. McIntosh, E. K. Brechin and S. J. Dalgarno, Complementary ligands direct the formation of a calix[8]arene-supported ferromagnetic MnIVMnIII dimer, Dalton Trans., 2013, 42, 6697 Search PubMed.
- S. M. Taylor, S. Sanz, R. D. McIntosh, C. M. Beavers, S. J. Teat, E. K. Brechin and S. J. Dalgarno,
p-tert-Butylcalix[8]arene: An extremely versatile platform for cluster formation, Chem. – Eur. J., 2012, 18, 16014 Search PubMed.
- H. S. Wang, Y. Chen, Z. B. Hu, Q. Q. Long, C. L. Yin, Z. C. Zhang and Z. Q. Pan, A Dy-based complex with the magnetic relaxation behavior regulated by enclosing one DyIII ion into a Calix[8]arene ligand, Inorg. Chem. Commun., 2019, 105, 76 Search PubMed.
- H. T. Han, X. L. Li, X. F. Zhu, G. S. Zhang, S. T. Wang, X. X. Hang, J. K. Tang and W. P. Liao, Single-molecule magnet behavior in a calix[8]arene-capped {Tb6IIICrIII} cluster, Eur. J. Inorg. Chem., 2017, 14, 2088 Search PubMed.
- H. T. Han, X. L. Li, X. F. Zhu, G. S. Zhang, X. X. Hang, J. K. Tang and W. P. Liao, Single molecule magnet behavior in a calix[8]arene-capped heterometallic {DyIII4CoII4} square antiprismatic cluster, Eur. J. Inorg. Chem., 2017, 41, 4879 Search PubMed.
- S. Haldara, G. Vijaykumar, L. Carrella, G. T. Musie and M. Bera, Structure and properties of a novel staircase-like decanuclear [Cu II10] cluster supported by carbonate and carboxylate bridges, New J. Chem., 2018, 42, 1276 Search PubMed.
- K. S. Brzostek, M. Terlecki, K. Sokołowski and J. Lewiński, Chemical fixation and conversion of CO2 into cyclic and cage-type metal carbonates, Coord. Chem. Rev., 2017, 334, 199 Search PubMed.
- B. Das, M. Bhadbhade, A. Thapper, C. D. Ling and S. B. Colbran, A new trinuclear Cu-carbonate cluster utilizing CO2 as a C1-building block -reactive intermediates, a probable mechanism, and EPR and magnetic studies, Dalton Trans., 2019, 48, 3576 Search PubMed.
- W. Meng, H. F. Ye, S. Liu, F. Xu and W. J. Xu, Emergence of complex chiral coordination clusters {Cu II48Na12} by using multiple ligands under oxidizing conditions, Dalton Trans., 2019, 48, 3204 Search PubMed.
- E. Guarda, K. Bader, J. V. Slagerenb and P. Alborés, {NiII8LnIII6} (Ln=Gd, Dy) rod-like nano-sized heteronuclear coordination clusters with a double carbonate bridge skeleton and remarkable MCE behaviour, Dalton Trans., 2016, 45, 8566 Search PubMed.
- H. Q. Tian, L. Zhao and J. K. Tang, Exploiting miraculous atmospheric CO2 fixation in the design of dysprosium single-molecule magnets, Cryst. Growth Des., 2018, 18, 1173 Search PubMed.
- X. M. Zhang, P. Li, W. Gao, J. P. Liu and E. Q. Gao, A new cube-based dodecanuclear cobalt(II) cluster with azide and tetrazolate ligands exhibiting ferromagnetic ordering, Dalton Trans., 2015, 44, 13581 Search PubMed.
- S. Schmitz, J. V. Leusen, A. Ellern, P. Kögerler and K. Y. Monakhov, Thioether-terminated nickel(II) coordination clusters with {Ni6} horseshoe-and {Ni8} rollercoaster-shaped cores, Inorg. Chem. Front., 2016, 3, 523 Search PubMed.
- G. J. Cooper, G. N. Newton, P. Kögerler, D. L. Long, L. Engelhardt, M. Luban and L. Cronin, Structural and compositional control in {M12} cobalt and nickel coordination clusters detected magnetochemically and with cryospray mass spectrometer, Angew. Chem., Int. Ed., 2007, 46, 1340 Search PubMed.
- C. D. Gutsche, B. Dhawan, K. H. No and R. Muthukrishnan, Calixarenes. 4. The synthesis, characterization, and properties of the calixarenes from p-tert-butylphenol, J. Am. Chem. Soc., 1981, 103, 3782 Search PubMed.
-
G. M. Sheldrick, SHELXS-97, A computer program for the solution of crystal structures, PC version, University of Göttingen, Göttingen, Germany, 1997 Search PubMed.
- D. Casanova, P. Alemany, J. M. Bofill and S. Alvarez, Shape and symmetry of hepta coordinate transition-metal complexes: structural trends, Chem. – Eur. J., 2003, 9, 1281 Search PubMed.
- D. Casanova, M. Llunell, P. Alemany and S. Alvarez, The rich stereochemistry of eight-vertex polyhedra: a continuous shape measures study, Chem. – Eur. J., 2005, 11, 1479 Search PubMed.
- Y. H. Wan, L. P. Zhang, L. P. Jin, S. Gao and S. Z. Lu, High-dimensional architectures from the self-assembly of lanthanide ions with benzenedicarboxylates and 1,10-phenanthroline, Inorg. Chem., 2003, 42, 4985 Search PubMed.
- N. F. Chilton, R. P. Anderson, L. D. Turner, A. Soncini and K. S. Murray, PHI: A powerful new program for the analysis of anisotropic monomeric and exchange-coupled polynuclear d- and f-block complexes, J. Comput. Chem., 2013, 34, 1164 Search PubMed.
- N. A. Chernova, Y. N. Song, P. Y. Zavalij and M. S. Whittingham, Solitary excitations and domain-wall movement in the two-dimensional canted antiferromagnet (C2N2H10)1/2FePO4(OH), Phys. Rev. B: Condens. Matter Mater. Phys., 2004, 70, 144405 Search PubMed.
-
J. A. Mydosh, Spin Glasses: An Experimental Introduction, Taylor & Francis, London, 1993 Search PubMed.
- M. A. Novak, Magnetic relaxation in molecular nanostructures, J. Magn. Magn. Mater., 2004, 272–276, E707 Search PubMed.
- Y. Z. Yu, Y. H. Guo, Y. R. Zhang, X. J. Tian and X. M. Zhang, Tetrahedral μ4-chloride and in situ generated octahedral μ6-sulfide templating Co8 complexes with different distortions of the cube, Chem. Commun., 2020, 56, 4236–4239 Search PubMed.
- Q. Chen, M. H. Zeng, L. Q. Wei and M. Kurmoo, A multifaceted cage cluster, [CoII6O12 ⊃X]− (X=Cl− or F−): Halide template effect and frustrated magnetism, Chem. Mater., 2010, 22, 4328 Search PubMed.
- Y. N. Guo, G. F. Xu, Y. Guo and J. K. Tang, Relaxation dynamics of dysprosium(III) single molecule magnets, Dalton Trans., 2011, 40, 9953 Search PubMed.
Footnotes |
† Electronic supplementary information (ESI) available: Additional figures, UV-vis spectra, TG/DSC curves and FT-IR spectra. CCDC 2013506–2013508. For ESI and crystallographic data in CIF or other electronic format, see DOI: 10.1039/d0qi00792g |
‡ These authors contributed equally. |
|
This journal is © the Partner Organisations 2020 |
Click here to see how this site uses Cookies. View our privacy policy here.