DOI:
10.1039/C9PY01441A
(Paper)
Polym. Chem., 2020,
11, 75-83
A thermotropic poly(ether ketone) based on the p-quaterphenyl unit: evidence for a smectic C phase†
Received
24th September 2019
, Accepted 19th November 2019
First published on 20th November 2019
Abstract
High-temperature polycondensation of 4,4′-(hexafluoroisopropylidene)diphenol with the homologous series of bis(fluoroaryl)diketones FArCO[Ar]xCOArF (Ar = 1,4-phenylene, x = 1, 2, 3 or 4) affords polyetherketones [–OArC(CF3)2ArOArCOArxCOAr–]n. The polymers with x = 1–3 are conventional amorphous thermoplastics, but when x = 4 much more complex and unusual phase behaviour is seen. The thermophysical characteristics of this polymer have been investigated by DSC and by X-ray powder and fibre diffraction. The results are accounted for on the basis that cooling from the isotropic melt (which is present above 375 °C) leads to rapid formation of a smectic-C-type mesophase at around 330 °C, with higher levels of order developing more slowly over a broad temperature range between ca. 300 and 230 °C.
1. Introduction
Linear thermoplastics comprising mainly aromatic subunits have achieved high industrial significance as engineering materials over recent decades,1,2 as a consequence of their excellent resistance to thermal and oxidative degradation, and their retention of mechanical strength and toughness to much higher temperatures than their aliphatic counterparts.3,4 For amorphous materials such as the aromatic poly(ether sulfone)s5 and poly(ether-imide)s6 the limits of thermomechanical stability are set simply by the glass transition temperature, but for semi-crystalline polymers such as the poly(ether ketone)s7 this limit is a more complex function of both the glass transition temperature and the crystalline melting point, since polymer crystallites can act as “physical cross-links”,8 even well above the glass transition. Crystallisation thus leads to enhanced polymer properties,9 including retention of flexural stiffness and strength at higher temperatures and greatly increased resistance to solvent attack. For this reason there have been continuing attempts to develop semi-crystalline aromatic thermoplastics with ever-higher glass transition temperatures, while maintaining a crystalline melting point that is low enough for the polymer still to be processed in the melt.10
Some success has been achieved in polyimide chemistry, notably with the crystallisable thermoplastic polyimide 1 developed by NASA as LARC-CPI,11,12 and polyimide 2, commercialised by Mitsui as Aurum™ (Chart 1).13,14 The latter polymer has a Tg of 250 °C and a crystalline melting point Tm of 388 °C so that the ratio of Tg to Tm in kelvin, at 0.79, is very high although the crystallisation rates of polymers 1 and 2 are, as a consequence, extremely low.14 More recent reports in this area have described a further range of thermally crystallisable polyimides such as 315 and 4,16 but the issue of slow crystallisation persists.17 Moreover, although aromatic polyimides generally display very good stability towards thermal degradation, the imide group has only low hydrolytic stability, even at neutral pH if the temperature is sufficiently high.2 In contrast, thermally crystallisable aromatic poly(ether ketone)s such as PEEK (5) and PEK (6) are essentially inert towards hydrolysis18 and crystallise rapidly from the melt,19,20 but their thermomechanical stability is limited to some extent by their relatively low glass transition temperatures (typically ca. 140 to 170 °C).1
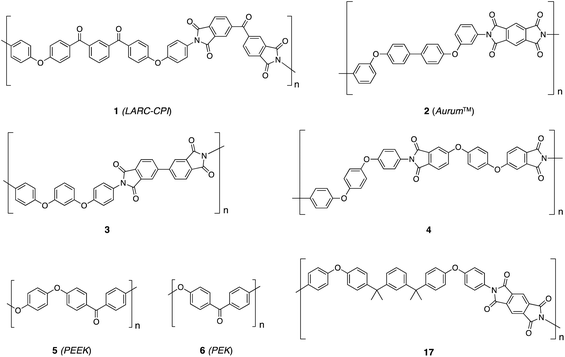 |
| Chart 1 Some thermally-crystallisable high-performance polymers (1–6) and a thermotropic polyimide (17). | |
In the present paper we report the discovery of an unusual poly(ether ketone), made during the course of a research programme aimed at developing more thermo-mechanically stable matrix polymers for advanced carbon fibre composites. Our approach was based on progressive replacement of the central 1,4-phenylene residue, Ar, in a known, non-crystallising polymer, [–OArC(CF3)2ArOArxCOArCOAr–]n, by more extended oligo-p-phenylene units [1,4-Ar]x (x = 2, 3 and 4). The intention was not only to enhance Tg by incorporation of a progressively higher proportion of rigid biaryl linkages but also ultimately, perhaps, to achieve thermal crystallisability. In practice, amorphous character is retained for x = 2 and 3, but at x = 4 the polymer shows much more complex phase behaviour, notably formation of a mesophase on cooling from the isotropic melt, followed by a series of less-well-defined ordering transitions at lower temperatures. The kinetics of mesophase formation on cooling from the melt are very fast, and this phase cannot be by-passed even at the highest available cooling rates. The mesophase is provisionally identified as smectic C on the basis of X-ray fibre and powder diffraction studies, but the lower-temperature phases whose formation is evident from DSC are not fully ordered and may best be described as smectic-crystalline.
2. Experimental section
2.1 Materials
Anhydrous aluminium chloride, 1,2-dichlorobenzene (DCB), 1,2,4-trichlorobenzene (TCB), N-methylpyrrolidinone (NMP), N,N-dimethylacetamide (DMAc) and N,N-dimethylformamide (DMF) were purchased from Acros, while hydrochloric (36%, w/w) and nitric acids were purchased from Fischer Scientific. Diphenyl sulfone (DPS), 4,4′-hexafluoroisopropylidene-diphenol (HFDP), nickel(II) chloride, zinc powder, triphenylphosphine, 4-fluorobenzoyl chloride and 1,3-phenylenebis((4-fluorophenyl)-methanone) were purchased from Aldrich. All materials were used as received except 4-bromobiphenyl (98%), which was purchased from Aldrich and recrystallised from methanol before use. The monomers 1,4-phenylenebis((4-fluorophenyl)methanone),21 [1,1′-biphenyl]-4,4′-diylbis((4-fluorophenyl)methanone),10 and [1,1′:4′,1′′-terphenyl]-4,4′′-diylbis((4-fluorophenyl)methanone)22 were synthesised as described in the literature.
2.2 Instrumentation and analysis
Proton and 13C NMR spectra were recorded on Bruker Nanobay 400 MHz and Avance III 700 MHz spectrometers and were referenced to residual solvent protons. Chemical shifts (δ) are reported in parts per million (ppm) relative to tetramethylsilane (0.00 ppm). Electrospray mass spectra were recorded using a ThermoFisher Orbitrap XL instrument. Solid-state FT-IR spectra were recorded on a PerkinElmer Spectrum 100 spectrometer. Differential scanning calorimetry was carried out under nitrogen using a TA Instruments Q2000 DSC at a standard heating rate of 20 °C min−1 and cooling rate of 10 °C min−1. Inherent viscosities (ηinh) were determined for 0.1 wt% solutions of polymer in either NMP or trifluoromethanesulfonic acid, using a semi-automatic Schott-Geräte CT-150 viscometer, with capillary 53110. An FEI Quanta FEG 600 Environmental Scanning Electron Microscope, operating at 20 kV was used to image cryogenically fractured polymer surfaces. X-ray powder diffraction data were collected at room temperature using a Bruker D8 powder diffractometer with Cu-Kα radiation (λ = 1.5418 Å) and at the (former) Daresbury Synchrotron Radiation Source using X-radiation of wavelength 1.54 Å. Fibre-diffraction data were obtained from uniaxially-oriented film samples (ca. 50 μm in thickness), drawn over a hot stage to ca. 5× their original length and annealed at constant length for 8 h at 300 °C. X-ray fibre diagrams were measured using a Rigaku/MSC FR-D X-ray source (Cu-Kα radiation, λ = 1.5418 Å) and a Saturn 92 CCD detector with the X-ray beam normal to the fibre direction. Small angle X-ray scattering studies were carried out on a Bruker AXS Nanostar system fitted with a Vantec 2000 photon-counting 2D detector and variable camera length. This system used a Cu-Kα (λ = 1.5418 Å) source at 45 kV with a compact 3-pinhole collimation system giving a spot size of 0.4 to 0.8 mm. SAXS patterns were integrated both radially and azimuthally to produce 1D intensity profiles. Molecular modelling was carried out using the Cerius2 suite of programs (v.3.5, Accelrys, San Diego). Visual observations were made using a Leica polarizing optical microscope equipped with a Mettler Toledo FP82HT hot-stage. Tensile testing was carried out on an Instron 3300 system at a true strain rate of 0.04 s−1.
2.3 Synthesis
Synthetic details for the p-quaterphenyl-based monomer 7 and its derived thermotropic poly(ether-ketone) 8 are given here. The syntheses of other intermediates and polymers are detailed in the ESI.†
2.3.1 [1,1′:4′,1′′:4′′,1′′′-Quaterphenyl]-4,4′′′-diylbis((4-fluorophenyl)methanone) (7).
A mixture of anhydrous NiCl2 (0.24 g, 1.83 mmol), triphenylphosphine (3.79 g, 14.47 mmol), powdered zinc (12.37 g, 36.28 mmol) tetrabutylammonium iodide (2.59 g, 7.01 mmol) and anhydrous DMAc (30 mL) was stirred at 60 °C under nitrogen for 45 min. To this was added, dropwise with stirring over 2 h, a solution of intermediate 9 (3.65 g, 10.26 mmol; see Scheme 2 and ESI†) in DMAc (36 mL). The resulting suspension was cooled to room temperature and the solid was filtered off and washed with acetone until the washings were colourless. The solid was then dried and extracted in aqueous nitric acid (300 mL, 10%), with stirring for 1 hour, to remove zinc and residual nickel. The residue was filtered off and the extraction repeated. The resulting solid was washed with water until it was acid-free, then with acetone (100 mL), and dried before being recrystallised from 1,2,4-trichlorobenzene (300 mL) to give [1,1′:4′,1′′:4′′,1′′′-quaterphenyl]-4,4′′′-diylbis((4-fluorophenyl)methanone) (7) as a white crystalline solid (1.86 g, 66%); mp. 401 °C (99.9% purity by DSC); 1H NMR (700 MHz, CF3SO3H with CDCl3 coaxial reference) δ 8.67–8.20 (br, 12H), 8.19–7.80 (br, 8H), 7.79–7.49 (br, 4H); IR (Vmax) 3070 (νCHAr), 1642 (νC
O), 1598 (νC–CArc), 1237 (νC–F); 13C NMR (125 MHz, CDCl3) δ (ppm) 203.3, 182.6, 182.5, 180.3, 179.5, 172.5, 170.9, 155.2, 142.7, 139.7, 137.3, 136.9, 134.2, 134.0, 133.1, 132.9, 132.8, 130.9, 128.5, 128.0, 126.2, 124.6.
2.3.2 Thermotropic homopolymer 8.
A mixture of 4,4′-hexafluoroisopropylidenediphenol (10, 1.780 g, 5.298 mmol), monomer 7 (3.068 g, 5.578 mmol, 5.0 mol% excess), anhydrous sodium carbonate (0.580 g, 5.57 mmol) and diphenyl sulfone (30 g) was stirred under nitrogen and heated from 190 °C to 300 °C over the course of an hour. The mixture was stirred for a further 2.5 hours at this temperature, and the mixture was then poured onto aluminium foil and allowed to cool. The resulting solid was milled to a coarse powder (particle size 0.1–0.5 mm). Extraction with acetone (200 mL), water (200 mL) and finally again with acetone (200 mL), all at reflux, afforded the off-white polymer 8 (4.08 g, 91%). The polymer was insoluble at room temperature in any organic solvent but dissolved readily, without decomposition, in trifluoromethanesulfonic (triflic) acid. Inherent viscosity measurements and NMR characterisation (with co-axial CDCl3 as external reference) were therefore carried out in triflic acid solution. 1H NMR (400 MHz, CF3SO3H) δ (ppm) 8.44 (br, 4H), 8.30 (br, 8H), 8.09 (br, 8H), 7.84 (br, 4H), 7.50 (br, 4H), 7.46 (br, 4H); 13C NMR (100 MHz, CF3SO3H) δ (ppm) 194, 160, 139, 133, 129, 66; IR (νmax) 3032 cm−1(νCHAr), 1651 cm−1 (νC
O), 1594 cm−1 (νC–CAr) and 1242 cm−1 (νC–F), 1172 cm−1 (νO–CAr); ηinh (CF3SO3H) = 0.61 dL g−1. Lowering the molar excess of 7 to 3% and 2% gave samples of polymer 8 with inherent viscosities (ηinh) of 0.67 and 0.91 dL g−1 respectively.
3. Results and discussion
3.1 Polymer synthesis
Our conceptual approach was to begin with the relatively low-Tg (171 °C onset) non-crystallisable poly(ether ketone) derived from 4,4′-hexafluoroisopropylidene-diphenol (10) and 1,4-bis(4′-fluorobenzoyl)benzene (11), and then to modify the difluoro-monomer by replacing the central 1,4-phenylene unit with more extended, linear, poly-p-phenylene residues using monomers 12, 13 and 7 (Scheme 1).
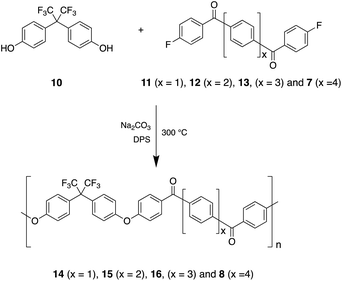 |
| Scheme 1 Synthesis of amorphous poly(ether-ketone)s 14–16 and the thermotropic polymer 8. | |
Polymers 14, 15 and 16 (Scheme 1) were thus obtained from monomers 11, 12 and 13 respectively by polycondensation with 4,4′-hexafluoroisopropylidene-diphenol. The synthesis of monomer 7 is outlined in Scheme 2.
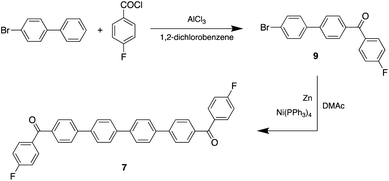 |
| Scheme 2 Synthesis of the quaterphenyl-based monomer 7via nickel-catalysed coupling of 9. | |
3.2 Thermal analysis
Polymers 14, 15 and 16, containing 1,4-phenylene, 4,4′-biphenylene and 4,4′′-terphenylene subunits, proved amorphous by DSC (Fig. 1), although their glass transition temperatures (onsets at 171, 185 and 205 °C respectively) did increase progressively with the length of the poly-p-phenylene residue. However, as shown in Fig. 1, polymer 8 (ηinh = 0.61 dL g−1) derived from the p-quaterphenyl-based monomer 7 behaved very differently on heating, with a broad, shallow endotherm from 243 °C and a sharp “melting” transition at ca. 355 °C (peak temperature; ΔH = 15.7 J g−1). Higher-viscosity samples of polymer 8 showed this transition at slightly lower temperature: 344 °C for ηinh = 0.67 dL g−1, and 342 °C for ηinh = 0.91 dL g−1.
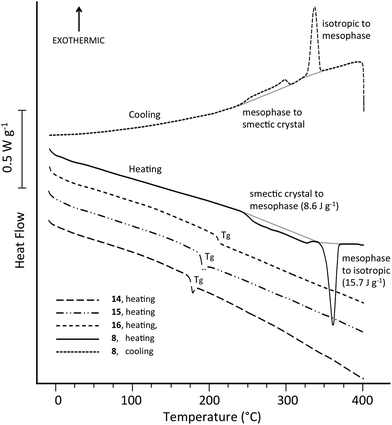 |
| Fig. 1 DSC thermograms (2nd scans) of polymers 14–16 and 8 showing the amorphous character of 14, 15 and 16, and the thermotropic behaviour of 8. Heating rate 20 °C min−1; cooling rate 10 °C min−1. Glass transitions are very evident for the first three polymers (onset temperatures of 171, 185 and 205 °C respectively). An apparently similar step-transition at 243 °C is seen for polymer 8, but the mesomorphic character of this polymer prevents its conclusive identification as a Tg. | |
These thermal transitions were reversible (Fig. 1) and, between cooling rates of 5 and 40 °C, there was only a ca. 2 °C change in the onset temperature of the high-temperature transition (see ESI†). Moreover, for the lowest-MW sample (ηinh = 0.61 dL g−1) even “ballistic” cooling of 8, from 400 °C into ice-water, produced no change in DSC behaviour on re-heating—all transitions previously observed (Fig. 1) were still present. This behaviour stands in sharp contrast to conventional thermoplastics such as PET and PEEK, for which crystallisation is completely suppressed on quenching from the isotropic melt.20 Even for the highest-MW sample of 8 (ηinh = 0.91 dL g−1), the sharp endothermic transition at 360 °C remained essentially unchanged on reheating after quenching, although the lower-temperature transitions were rather weaker.
Above 380 °C, polymer 8 was fluid and isotropic in the polarising microscope, but quenched samples were birefringent (see ESI†), strongly suggesting that the “unquenchable” transition on cooling represents the transition from an isotropic phase to a mesophase, which is “frozen” on quenching but at slower cooling rates undergoes further transitions to a more ordered solid state, as represented by the broad, low temperature endotherm. It is also possible that the onset-step (at 243 °C) of this endotherm on heating represents a glass transition, but at high cooling rates formation of a frozen mesophase (which does not exhibit a Tg), rather than an amorphous phase (which does), prevents conclusive identification of this feature.
There have been a number of reports of aromatic ether-ketone copolymers in which a thermotropic mesophase is accessed by disrupting the structure of a crystalline homopolymer. This has generally been achieved by introduction of a bulky and/or directionally-disordered co-monomer: indeed, all previous thermotropic poly(ether-ketone)s have adopted this design philosophy.23–31 Here, polymer 8 represents a very different type of thermotropic material, specifically in being a homopolymer rather than a copolymer, and being developed, conceptually, from an amorphous material rather than one with pre-existing order. It may be noted that the design of polymer 8, with its combination of a linear, rigid, p-quaterphenyl mesogen alternating with a non-linear and flexible hexafluoroisopropylidene-diphenol residue finds parallel in a thermotropic polyimide (polymer 17, Chart 1) based on a linear, rigid, diphenylpyromellitimide unit alternating with a non-linear, flexible diether residue.32,33
As shown in Fig. 1, the enthalpies of the observed DSC transitions of polymer 8 are relatively low, typically in the range 15–18 J g−1 for the main mesophase transition and 8–10 J g−1 overall for the broad series of lower-temperature transitions. These enthalpies are, however, in the range generally found for mesophase transitions in main-chain liquid crystal polymers.34 For comparison, the melting enthalpy of the conventionally crystalline poly(ether ketone) PEEK is typically around 50 J g−1, at a crystallinity level of ca. 40%.20
3.3 X-ray analysis
Laboratory WAXS studies (Fig. 2) of a sample of polymer 8, annealed at 300 °C for 8 h and then cooled to 25 °C, showed two sharp, low-angle reflections with d-spacings of 11.0 and 16.5 Å, and a series of strong but diffuse reflections at higher angles. Synchrotron data revealed an additional, very strong reflection at lower angle, at d = 33.0 Å (Fig. 2), and this peak was still evident, if substantially less intense, even in quenched samples of the polymer.
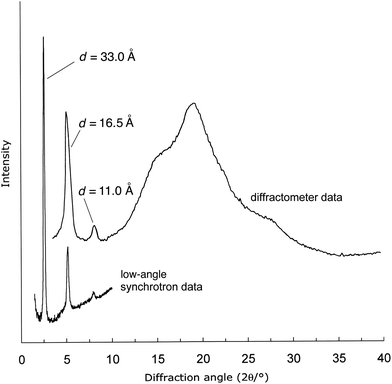 |
| Fig. 2 X-Ray powder diffractograms of an annealed sample of polymer 8. | |
The X-ray powder data are fully consistent with the presence in 8 of a smectic mesophase (or, after annealing, a more ordered smectic-crystalline phase), in which the sharp, low-angle reflections represent the first, second and third orders of diffraction from lamellae whose spacing (33.0 Å) is very well-defined in the direction of the polymer chain. The diffuse, higher-angle reflections then arise from disordered packing of polymer chains within the lamellae. The detailed nature of this structure was investigated by X-ray fibre diffraction.
Film samples of quenched polymer 8 were cut into strips and hot-drawn to five times their original length before being annealed at constant length for 8 h at 300 °C. The resulting X-ray fibre-diagram (Fig. 3a), revealed a striking “cross-ways” pattern of sharp reflections about the meridian, and strong but diffuse diffraction on the equator. Such a pattern is very characteristic of a smectic C phase,35 but not of a three-dimensionally-ordered crystalline phase. Both powder and fibre data thus suggest that, even after high-temperature annealing, the polymer adopts a disordered “smectic-crystalline” phase, i.e. a phase with only limited lateral correlations within the smectic layers,35 rather than a fully-crystalline material.
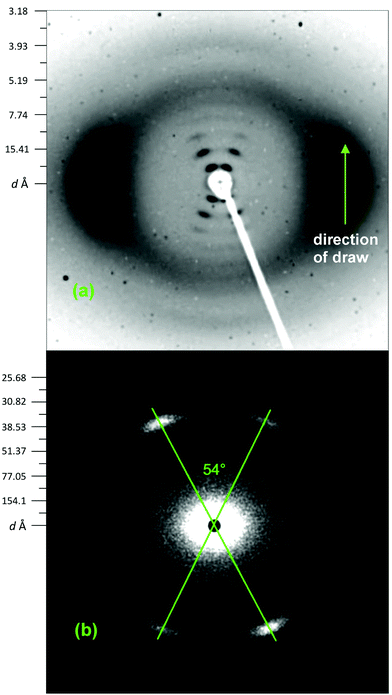 |
| Fig. 3 X-ray fibre diagrams of 8. (a) Wide angle diffractometer, and (b) small angle scattering instrument. The SAXS reflections correspond to the innermost spots visible in the wide-angle pattern. | |
Tensile testing of polymer 8 samples before and after uniaxial drawing (fivefold extension) showed an approximate doubling of tensile modulus, from 760 to 1480 MPa, and a corresponding reduction in extension to break, from 13% to 9%.
Small-angle scattering measurements on the same oriented sample as was used for WAXS analysis (Fig. 3b) confirmed that there were no diffraction peaks at lower angle than those observed in the original fibre diagram (Fig. 3a), and the SAXS study established both the smectic lamellar spacing (33.0 Å) and the tilt angle of the lamellae from the normal to the fibre direction (27.0°). The fact that the diffuse reflections lie on the equator (Fig. 3a) suggests that the polymer chains, overall, are arranged parallel to the fibre axis and thus to the direction of orientation.
A schematic of the proposed smectic C mesophase of polymer 8, based on the present fibre-diffraction data, is given in Fig. 4. Although the lamellar spacing is obviously very well-defined, in a smectic crystal there is substantial freedom for rotational and lateral disorder of chains within the lamellae. This proposed arrangement of chains aligns the polymer-repeat with the direction of fibre-orientation, and moreover it locates the carbonyl groups in near-linear alignment with one another (Fig. 4). Such an alignment of carbonyl groups is frequently observed in the crystal structures of aromatic polyetherketones,36–39 so that Fig. 4 is consistent with both the reported diffraction data and with the more general structural principles of poly(ether-ketone) chemistry. The repeat-distance for a fully-extended chain of polymer 8 was estimated by computational modelling at ca. 37.0 Å so that, as shown in Fig. 4, the observed lamellar thickness of 33.0 Å is fully consistent with the proposed tilting of the lamellae with respect to the chain-direction normal (37.0 × cos
27° = 33.0).
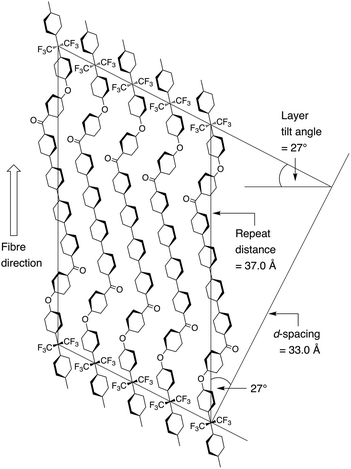 |
| Fig. 4 Proposed organisation of polymer chains in the smectic-crystalline phase of polymer 8. | |
3.4 Electron microscopy
Analysis by SEM of the fracture-surface of polymer 8 after slow cooling from 400 °C showed a texture comprising sheafs of material, invariably terminating in curious, sharply-pointed features that were highly reproducible between samples (Fig. 5). The origins of this unusual morphology are not clear, but certainly deserve further investigation.
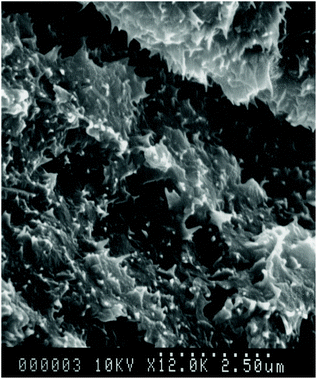 |
| Fig. 5 Morphology of polymer 8 at the fracture-surface. | |
3.5 Copolymer studies
Finally, we have explored the potential for the quaterphenyl-based monomer 7 to sustain smectic and/or smectic-crystalline behaviour in copolymers containing the potentially mesophase-disrupting monomer 18. Polycondensation of 4,4′-hexafluoroisopropylidene-diphenol (10) with 18 is known40 to give a purely amorphous poly(ether ketone) and so, in the present work, copolymers 19 and 20 were synthesised by reaction of 10 with 1
:
1 and 2
:
1 molar ratios of 7 and 18 respectively (Scheme 3). Copolymers 19 and 20 show thermal transitions by DSC that echo those of homopolymer 8, but the mesophase transition is much broadened, weakened, and shifted to lower temperature (Fig. 6). However, the lower-temperature transitions, though greatly broadened, are not significantly weaker than in homopolymer 8. All the observed transitions are still reversible, and an X-ray fibre diagram of copolymer 20, drawn and annealed as for homopolymer 8, again shows evidence of lamellar ordering.
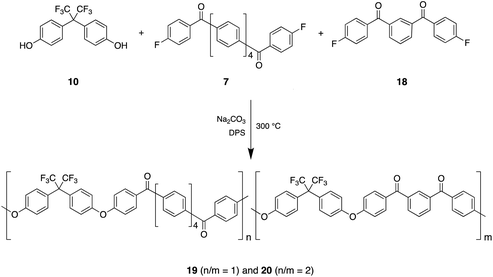 |
| Scheme 3 Synthesis of copolymers 19 and 20 using different ratios of monomers 7 and 18. | |
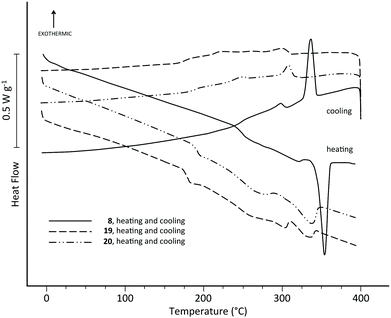 |
| Fig. 6 DSC thermograms of polymers 8, 19 and 20 showing destabilisation of the mesophase transition as the proportion of comonomer 18 is increased relative to the mesogenic monomer 7. | |
A series of diffuse but now on-meridional reflections is observed (Fig. 7) indicating a non-tilted lamellar structure which may have its origin in a mesophase of the smectic A type. The equatorial reflections are much better-resolved than in the fibre-diagram of homopolymer 8, even though the DSC results indicate an lower degree of ordering overall. The X-ray fibre pattern of copolymer 20 thus suggests that the mesophase transition observed by DSC leads to a different type of structure from that of homopolymer 8, and that, even if copolymer 20 does pass through a smectic (A) phase on cooling from the melt, its final, ordered phase has a lamellar structure that is more conventionally crystalline.
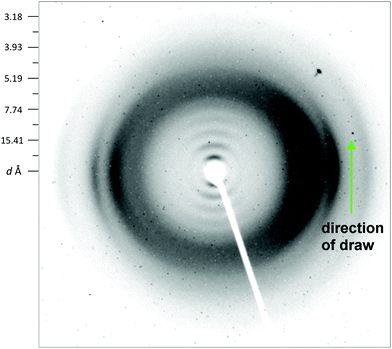 |
| Fig. 7 X-ray fibre diagram of copolymer 20 showing “smectic A type” reflections along the meridian, but with some resolution of equatorial reflections suggesting a higher degree of lateral ordering than is found in homopolymer 8 (cf. Fig. 3a). | |
4. Conclusions
A novel p-quaterphenyl-based poly(ether ketone) [–OArC(CF3)2ArOArCOAr4COAr–]n has been synthesised and its thermal and crystallographic properties are accounted for in terms of liquid crystalline behaviour. Cooling from the isotropic melt (ca. 400 °C) leads to rapid formation of a smectic C mesophase at around 330 °C, with higher levels of order, possibly in the form of a smectic-crystalline phase, developing more slowly over a broad temperature range between ca. 300 and 230 °C. The isotropic-to-mesophase transition cannot be by-passed, even at “ballistic” cooling rates, and in keeping with this, no glass transition can be identified on re-heating the sample. Homologous polymers containing the shorter p-terphenyl and biphenyl residues show only amorphous character.
Conflicts of interest
There are no conflicts of interest to declare.
Acknowledgements
This work was funded by the University of Reading and the Education Trust Fund of Nigeria (a PhD studentship to FO). We thank the University of Reading Chemical Analysis Facility for access to thermal and X-ray facilities. Our original work in this field was carried out in the research laboratories of the former ICI plc.41 We are grateful to Mrs Carol Connor for experimental assistance, Dr Nick Terrill and Mr Nick Seymour for help with X-ray analysis, and Drs Graeme Finlan and Peter Harris for assistance with electron microscopy. We thank the Leverhulme Trust (an Emeritus Fellowship to HMC, grant number EM-2018-0161/4) for support during the completion of this work.
References
-
D. J. Kemmish, Practical Guide to High Performance Engineering Plastics, iSmithers, Shawbury, 2011 Search PubMed.
-
D. Kemmish, High Performance Engineering Plastics, Rapra Technology, Shawbury, 1995 Search PubMed.
-
High Performance Polymers: Their Origin and Development, ed. R. B. Seymour and G. S. Kirshenbaum, Elsevier, New York, 1986 Search PubMed.
-
V. Mittal, High Performance Polymers and Engineering Plastics, Wiley, New York, 2011 Search PubMed.
-
R. J. Cotter, Engineering Plastics: A Handbook of Polyarylethers, Gordon and Breach, Basel, 1995 Search PubMed.
-
Polyimides, ed. D. Wilson, H. D. Stenzenberger and P. M. Hergenrother, Blackie, Glasgow, 1990 Search PubMed.
-
P. A. Staniland, Poly(ether ketones), in Comprehensive Polymer Science, ed. G. Allen and J. C. Bevington, Pergamon, Oxford, 1989, vol. 5, pp. 483–497 Search PubMed.
- A. Askadskii, M. Popova, T. Matseevich and E. Afanasyev, The influence of the degree of crystallinity on the elasticity modulus of polymers, Adv. Mater. Res., 2013, 864–867, 640–643 Search PubMed.
- P. B. Bowden and R. J. Young, Deformation mechanisms in crystalline polymers, J. Mater. Sci., 1974, 9, 2034–2051 CrossRef CAS.
- See for example: H. M. Colquhoun, C. C. Dudman, D. J. Blundell, A. Bunn, P. D. Mackenzie, P. T. McGrail, E. Nield, J. B. Rose and D. J. Williams, An aromatic polyether in which sequence-randomization leads to induction of crystallinity: X-ray structure of the crystalline phase [-OArCOArArCOAr-]n (Ar=1,4-phenylene), Macromolecules, 1993, 26, 107–111 CrossRef CAS.
- P. M. Hergenrother, N. T. Wakelyn and S. J. Havens, Polyimides containing carbonyl and ether connecting groups, J. Polym. Sci., Part A: Polym. Chem., 1987, 25, 1093–1103 CrossRef CAS.
- J. T. Muellerleile, B. G. Risch, D. E. Rodrigues and G. L. Wilkes, Crystallization behaviour and morphological features of LARC-CPI, Polymer, 1993, 34, 789–806 CrossRef CAS.
-
M. Ohta, S. Kawashima, Y. Sonobe, S. Tamai, H. Oikawa, K. Ohkoshi and A. Yamaguchi, Eur. Pat, EP0235294, 1991, to Mistui Toatsu Search PubMed.
- R. S. Tsai, D. K. Lee, Y. C. Liu and H. B. Tsai, Crystallization of a thermoplastic polyimide, J. Appl. Polym. Sci., 2003, 90, 2604–2608 CrossRef CAS.
-
Y. Ikunori, Y. Kayako, Y. Masaji, K. Tomohito and O. Hideaki, Japanese Pat, JP3688097, 1999, to Mitsui Chemicals Search PubMed.
- H. Zhang, W. Wang, G. Chen, A. Zhang and X. Fang, Melt-processable semicrystalline polyimides based on 1,4-bis(3,4-dicarboxyphenoxy) benzenedianhydride (HQDPA): synthesis, crystallization, and melting behavior, Polymers, 2017, 9, 420 CrossRef.
- V. Ratta, A. Ayambem, R. Young, J. E. McGrath and G. L. Wilkes, Thermal stability, crystallization kinetics and morphology of a new semicrystalline polyimide based on 1,3-bis(4-aminophenoxy) benzene and 3,3′,4,4′-biphenyltetracarboxylic dianhydride, Polymer, 2000, 41, 8121–8138 CrossRef CAS.
- L. O. Dandy, G. Oliveux, J. Wood, M. J. Jenkins and G. A. Leeke, Accelerated degradation of polyetheretherketone (PEEK) composite materials for recycling applications, Polym. Degrad. Stab., 2015, 112, 52–62 CrossRef CAS.
- D. J. Blundell and B. N. Osborn, The morphology of poly(aryl-ether-ether-ketone), Polymer, 1983, 24, 953–958 CrossRef CAS.
- L. Jin, J. Ball, T. Bremner and H. J. Sue, Crystallization behavior and morphological characterization of poly(ether ether ketone), Polymer, 2014, 55, 5255–5265 CrossRef CAS.
- K. A. Walker, L. J. Markoski and J. S. Moore, Processible poly(arylene ether ketone)s that can be cross-linked to high-performance networks, Macromolecules, 1993, 26, 3713–3716 CrossRef CAS.
- A. Ben-Haida, H. M. Colquhoun, P. Hodge and D. F. Lewis, Reduction of carbonyl groups in aromatic polyketones: synthesis and characterization of methylene-bridged polyaryl-ethers and –thioethers, Polymer, 1999, 40, 5173–5182 CrossRef CAS.
- G. S. Bennett and R. J. Farris, The synthesis and characterization of novel thermotropic liquid crystalline poly(aryl ether ketone)s, Polym. Eng. Sci., 1994, 34, 781–793 CrossRef CAS.
- S. J. Zhang, Y. Zheng, Z. Wu, M. Tian, D. Yang and R. Yosomiya, The new class of thermotropic liquid-crystalline polymers: poly(etherether ketone ketone)s based on a chloro side group, J. Mater. Sci. Lett., 1997, 16, 1813–1815 CrossRef CAS.
- S. J. Zhang, D. C. Yang, S. Z. D. Cheng, Y. B. Zheng, Z. W. Wu and R. Yosomiya, Novel poly(aryl ether ketone)s exhibiting liquid crystallinity, Acta Polym., 1998, 49, 198–200 CrossRef CAS.
- S. Zhang, L. Fu, J. Liu, D. Yang, Z. Gao, M. Jia, Y. Zheng and Z. Wu, Synthesis and thermotropic liquid crystalline behaviour of novel poly(aryl ether ketone)s with a lateral methoxy group, Macromol. Chem. Phys., 2000, 201, 649–655 CrossRef CAS.
- S. Zhang, L. Fu, J. Liu, D. Yang, Z. Gao, M. Jia, Y. Zheng and Z. Wu, Synthesis and thermotropic liquid-crystalline behaviour of novel main-chain poly(ether ether ketone ketone)s containing a lateral tert-butyl group, Polym. Int., 2000, 49, 1539–1542 CrossRef.
- W. Hu, B. Liu, D. Wang, Y. Yang, C. Chen, Z. Jiang, W. Zhang and Z. Wu, Synthesis and thermotropic liquid-crystalline behavior of novel main-chain poly(aryl ether ketones), J. Appl. Polym. Sci., 2003, 89, 1347–1350 CrossRef CAS.
- G. Wang, Z. Jiang, S. Zhang, C. Chen and Z. Wu, Synthesis and characterization of thermotropic liquid crystalline poly(aryl ether ketone) copolymers with pendant 3-(trifluoromethyl) phenyl groups, Polym. Int., 2006, 55, 657–661 CrossRef CAS.
- Q. Sun, J. Wang, K. Jin, G. Su, G. Li and X. Jian, Synthesis and thermotropic liquid crystalline behavior of novel main-chain poly(aryl ether ketones) copolymer containing phthalazinone moiety and biphenyl mesogen, Polym. J., 2008, 40, 46–49 CrossRef CAS.
- Y. J. Lin, S. Qin, B. Han, C. Gao and Z. L. Zhang, Preparation of poly(ether ether ketone)-based composite with high electrical conductivity, good mechanical properties and thermal stability, High Perform. Polym., 2017, 29, 205–210 CrossRef CAS.
- T. Asanuma, H. Oikawa, Y. Ookawa, W. Yamasita, M. Matsuo and A. Yamaguchi, Synthesis of thermotropic liquid crystal polyimide and its properties, J. Polym. Sci., Part A: Polym. Chem., 1994, 32, 2111–2118 CrossRef CAS.
- M. Tanaka, M. Konda, M. Miyamoto, Y. Kimura and A. Yamaguchi, High Perform. Polym., 1998, 10, 147–153 CrossRef CAS.
- N. Kahn, V. L. Patel, Z. Bashir and D. M. Price, Phase transitions in two polyesters containing a smectic diol, J. Polym. Sci., Part B: Polym. Phys., 1995, 33, 1957–1967 CrossRef.
-
A. M. Donald, A. H. Windle and S. Hanna, Liquid Crystalline Polymers, Cambridge University Press, Cambridge, 2nd edn, 2005, pp. 259 and 268 Search PubMed.
- P. C. Dawson and D. J. Blundell, X-ray data for poly(aryl ether ketones), Polymer, 1980, 21, 577–578 CrossRef CAS.
- D. J. Blundell and A. B. Newton, Variations in the crystal lattice of PEEK and related para-substituted aromatic polymers: 2. Effect of sequence and proportion of ether and ketone links, Polymer, 1991, 32, 308–313 CrossRef CAS.
- D. J. Blundell and V. Bayon, The crystal structure of poly(ether ketone) copolymers, Polymer, 1993, 34, 1354–1360 CrossRef CAS.
- H.
M. Colquhoun and D. J. Williams, Crystal and molecular simulation of high- performance polymers, Acc. Chem. Res., 2000, 33, 189–198 CrossRef CAS PubMed.
- F. Leroux, R. A. Bennett, D. F. Lewis and H. M. Colquhoun, Macromolecules, 2018, 51, 3415–3422 CrossRef CAS.
-
H. M. Colquhoun and D. F. Lewis, Eur. Pat. Appln, EP0322151, 1989, to ICI Search PubMed.
Footnote |
† Electronic supplementary information (ESI) available. See DOI: 10.1039/c9py01441a |
|
This journal is © The Royal Society of Chemistry 2020 |
Click here to see how this site uses Cookies. View our privacy policy here.