Diastereoselective construction of carbazole-based spirooxindoles via the Levy three-component reaction†
Received
15th September 2019
, Accepted 2nd December 2019
First published on 2nd December 2019
Abstract
The CuSO4 catalyzed three-component reaction of indole-2-acetate, aromatic aldehydes and 3-methyleneoxindoles in toluene at 130 °C afforded polysubstituted spiro[carbazole-3,3′-indolines] in good yields and with high diastereoselectivity. When isatylidene malononitriles were used as dienophiles, regio-isomeric spiro[carbazole-2,3′-indolines] were selectively obtained. A similar three-component reaction with 2-arylidene-1,3-indanediones resulted in polysubstituted spiro[carbazole-3,2′-indenes] in satisfactory yields and with high diastereoselectivity. The stereochemistry of the diastereoisomers of the spiro compounds was clearly elucidated by analysis of NMR spectra and determination of fourteen single crystal structures. The reaction mechanism included formation of reactive 2,3-dimethyleneindoline and a sequential Diels–Alder reaction.
Introduction
Spirooxindoles represent the core structure of a wide variety of medicinal agents and natural products with broad biological activities, ranging from antitumor, antimicrobial, and anti-HIV to antipyretic agents.1,2 As a consequence, considerable interest has been paid to the development of elegant synthetic methodologies for diverse spirooxindole derivatives.3,4 On the other hand, tetrahydrocarbazole is also a privileged heterocyclic ring system. It not only widely exists in various naturally occurring alkaloids and pharmacologically active compounds, but also was found in a large family of synthetic analogues exhibiting a wide spectrum of important bioactivities, such as anti-tumor activity and anti-protein kinase C activity.5 Additionally, carbazole derivatives have found various potential applications in organic light-emitting diodes and other functional materials.6 Therefore, many elegant methods for the preparation of various tetrahydrocarbazole derivatives have been developed in the past years.7–9 The [4 + 2] reactions of 3-vinylindolines or 2-vinylindolines with diverse dienophiles have been proven to be the most attractive strategy for the synthesis of many carbazole derivatives.10–12 The merge of tetrahydrocarbazole with spirooxindole might result in a privileged heterocyclic system with interesting biological activities. According to the relative position of the spiro carbon atom in the central cyclohexyl ring, there are four possible regio-isomeric spiro-carbazole-oxindoles. A literature survey showed that three kinds of spiro-carbazole-oxindoles have been successfully synthesized mainly on the basis of the Diels–Alder reaction. Barbas and coworkers successfully constructed spiro[carbazole-1,3′-oxindoline] containing three or four stereocenters by using a bisthiourea organocatalytic reaction between 3-vinylindolines and methyleneindolinones (eqn (1) in Scheme 1).13a Feng's group also achieved a chiral N,N′-dioxide-Ni(II) complex catalyzed reaction between 3-vinylindoles and 3-methyleneindolinones for enantioselective synthesis of spiro[carbazole-1,3′-oxindolines].13b Recently, we have successfully revealed an efficient and diastereoselective synthetic protocol for spiro[carbazole-1,3′-indolines] by employing a TfOH catalyzed one-pot domino reaction of indoles, acetophenones (cyclic ketones) and 3-methyleneoxindolines.13c,d Melchiorre and co-workers also developed a chiral amine catalyzed reaction of 3-methyleneindolinones with indole-2,3-quinodimethanes generated from α,β-unsaturated aldehydes for asymmetric synthesis of spiro[carbazole-3,3′-oxindolines] (eqn (2) in Scheme 1).14a Shi and Tu reported a chiral phosphoric acid catalyzed asymmetric reaction of 2-vinylindolines with 3-methyleneindolinones to efficiently construct spiro[carbazole-3,3′-oxindolines] in high yields and with excellent stereoselectivities.14b A similar cinchona-derived squaramide catalyzed asymmetric reaction of 2-vinylindoles with 3-methyleneindolinones has also been disclosed.14c,d Very recently, tetrahydrospiro[carbazole-4,3′-indoline] has been synthesized by an AgOTf catalyzed alkyne-annulation/Diels–Alder cascade reaction of N-tosyl-2-(but-3-en-1-yn-1-yl)aniline and 2-methyleneindolinones (eqn (3) in Scheme 1).15 In continuation of our aim to develop multicomponent reactions for construction of diverse spirooxindole systems,16,17 herein, we wish to report an efficient and diastereoselective construction of tetrahydrospiro[carbazole-2,3′-indoline] by using a Levy three-component reaction of indole-2-acetate, aromatic aldehydes and isatylidene malononitriles (eqn (4) in Scheme 1). A similar diastereoselective synthesis of spiro[carbazole-3,2′-indene] and spiro[carbazole-3,3′-oxindoline] derivatives was also developed by employing 3-methyleneindolinones and 2-arylidene-1,3-indanediones as active dienophiles in the three-component reaction.
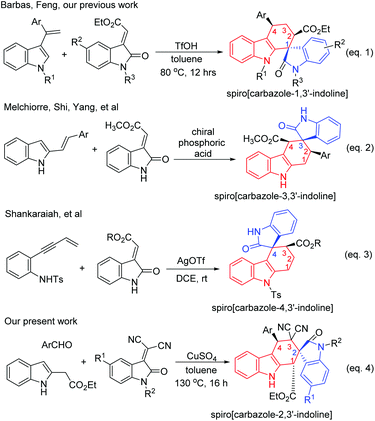 |
| Scheme 1 Synthesis of various isomeric spiro-carbazole-oxindoles. | |
Results and discussion
According to the Levy reaction conditions,18 a mixture of ethyl indole-2-acetate, benzaldehyde, and 3-phenacylideneoxindole in toluene in the presence of hydrated copper sulphate (CuSO4·5H2O) was heated at about 110 °C. The TLC monitor showed that the reaction was finished in 24 hours to give the expected spiro compound 1a in 42% yield. When the reaction was conducted at 130 °C, the yield of 1a was increased to 60%. When the reaction was carried out at high temperature such as 150–180 °C, the yield of the product 1a was decreased. Therefore, under these simple reaction conditions, various aromatic aldehydes and 3-methyleneoxindoles were employed in the reaction. The results are summarized in Table 1. All reactions proceeded smoothly to give the main products 1a–1m in 51–65% yields and the minor products in less than 10% yields. For convenience, only the main products were isolated by column chromatography and were fully characterized. In two cases, the minor products 1g′ and 1i′ were successfully isolated in 10% and 7% yields, respectively. It can be seen that aromatic aldehyde itself and those with methoxy, methyl, chloro and nitro groups gave similar yields of products, which indicated that both electron-rich and electron-poor aryl groups have a marginal effect on the reaction. On the other hand, both the benzoyl and alkoxycarbonyl substituted 3-methyleneoxindoles can be successfully employed in the reaction. These results showed that this three-component reaction has a widely variety of substrates. The obtained products were fully characterized by 1H NMR, 13C NMR, HRMS and IR spectroscopy. Because there are four stereogenic centers in spiro[carbazole-3,3′-indoline], a couple of diastereoisomers might be formed in the reaction. 1H and 13C NMR spectra of the obtained major products 1a–1m clearly showed only one diastereoisomer existing in the product. For elucidating the relative configuration, five single crystal structures 1a (Fig. 1), 1e, 1g, 1k and 1l (Fig. s1–s4†) were successfully determined. The same configuration was observed in the five single crystal structures. From Fig. 1, it can be seen that the aryl group, benzoyl group and phenyl group of the oxindole moiety exist in the cis-positions in the newly formed cyclohexenyl ring. The ethoxycarbonyl group exists in the trans-position. On the other hand, the middle cyclohexenyl ring adopts a half-chair conformation, in which the aryl group, benzoyl group and ethoxycarbonyl group all exist on the equatorial bond. This means that the major isomer belongs to the most stable conformation. It has been known that the benzoyl group and the phenyl group of the oxindole moiety exist in the cis-position in the starting (E)-3-methyleneoxindoles.19 Therefore, the cis-configuration of the two groups was retained in the reaction. The single crystal structures of the minor isomer 1g′ (Fig. 2) were also determined by the X-ray diffraction method. Compared to the single crystal structures of the above major isomers, it can be seen that all other substituents exist in the same position, and only the ethoxycarbonyl group stretches to the other direction in the minor isomer. In other words, the major isomer and minor isomer belong to epimers. Thus, on the basis of NMR data and single crystal structures, we could get a conclusion that the major products 1a–1m have this kind of relative configuration. These results also indicated that a concerted Diels–Alder reaction took place in the three-component reaction.
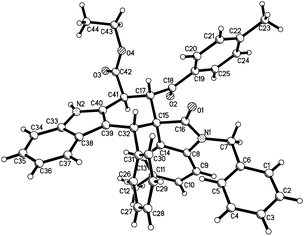 |
| Fig. 1 Single crystal structure of the spiro compound 1a. | |
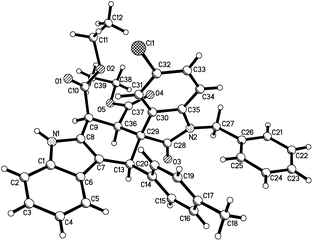 |
| Fig. 2 Single crystal structure of the spiro compound 1g′. | |
Table 1 Synthesis of spiro[carbazole-3,3′-indolines] 1a–1ma
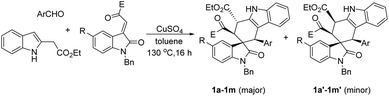
|
Entry |
Compd |
Ar |
R |
E |
Yieldb (%) |
Reaction conditions: Ethyl indole-2-acetate (0.5 mmol), aldehyde (0.6 mmol), 3-methyleneoxindole (0.5 mmol), CuSO4·5H2O (0.2 mmol), toluene (6.0 mL), 130 °C, 16 h.
Isolated yields.
The single crystal structure was determined by the X-ray diffraction method.
|
1 |
1a
|
C6H5 |
CH3 |
p-CH3C6H4 |
60c |
2 |
1b
|
C6H5 |
F |
p-CH3C6H4 |
62 |
3 |
1c
|
C6H5 |
Cl |
OCH2CH3 |
60 |
4 |
1d
|
p-CH3C6H4 |
CH3 |
p-CH3OC6H4 |
57 |
5 |
1e
|
p-CH3C6H4 |
F |
p-CH3C6H4 |
65c |
6 |
1f
|
p-CH3C6H4 |
Cl |
p-CH3C6H4 |
58 |
7 |
1g (1g′) |
p-CH3C6H4 |
Cl |
OCH2CH3 |
51c (10)c |
8 |
1h
|
p-CH3OC6H4 |
H |
p-CH3C6H4 |
60 |
9 |
1i (1i′) |
p-CH3OC6H4 |
CH3 |
p-CH3OC6H4 |
57 (7) |
10 |
1j
|
p-CH3C6H4 |
F |
OCH3 |
55 |
11 |
1k
|
p-ClC6H4 |
CH3 |
p-CH3OC6H4 |
55c |
12 |
1l
|
p-ClC6H4 |
CH3 |
OCH3 |
57c |
13 |
1m
|
p-NO2C6H4 |
Cl |
p-CH3OC6H4 |
60 |
For developing a variety of the substrates of the reaction, 2-arylidene-1,3-indanediones were employed in the reaction under same reaction conditions. The results are summarized in Table 2. It can be seen that all reactions proceeded smoothly to give the major products 2a–2l and minor products 2a′–2l′. The electron-rich and electron-poor aryl groups showed little effect on the yields of the products. All major products 2a–2l were obtained in moderate to good yields and some minor products 2b′, 2c′, and 2k′ were successfully isolated in lower yields. The structures of the obtained products were fully characterized by various spectroscopy techniques. The single crystal structures of the compounds 2a (Fig. s5†), 2b (Fig. 3), 2h and 2j (Fig. s6 and s7†) clearly indicated that both the aryl groups (Ar1 and Ar2) exist in the trans-position, while the ethoxycarbonyl group also exists in the trans-position to the neighboring aryl group (Ar2). The central cyclohexenyl ring still adopts a half-chair conformation. The ethoxycarbonyl group and the neighboring aryl group (Ar2) are in equatorial bonds, while the aryl group (Ar1) is in axial bonds. Due to the high steric repulsion, the planes of the two aryl groups and the whole scaffold of 1,3-indanedione stand perpendicularly to the cyclohexenyl ring. Additionally, the single crystal structure of the minor isomer 2b′ was also successfully determined (Fig. 4), in which the two aryl groups exist in the cis-position in the newly formed ring of cyclohexenyl ring. Thus, the relative configurations of both major isomers and minor isomers were confirmed by the X-ray single crystal diffraction method.
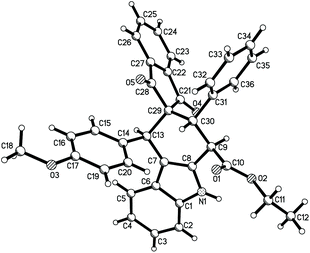 |
| Fig. 3 Single crystal structure of the spiro compound 2b. | |
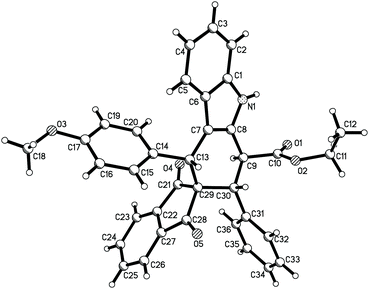 |
| Fig. 4 Single crystal structure of the spiro compound 2b′. | |
Table 2 Synthesis of spiro[carbazole-3,2′-indenes] 2a–2la
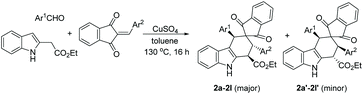
|
Entry |
Compd |
Ar1 |
Ar2 |
Yieldb (%) |
Reaction conditions: Ethyl indole-2-acetate (0.5 mmol), aldehyde (0.6 mmol), 2-arylidene-1,3-indanedione (0.5 mmol), CuSO4·5H2O (0.2 mmol), toluene (6.0 mL), 130 °C, 16 h.
Isolated yields.
The single crystal structure was determined by the X-ray diffraction method.
|
1 |
2a
|
p-CH3OC6H4 |
p-CH3C6H4 |
55c |
2 |
2b (2b′) |
p-CH3OC6H4 |
C6H4 |
55c (10)c |
3 |
2c (2c′) |
p-CH3OC6H4 |
p-ClC6H4 |
60 (8) |
4 |
2d
|
p-CH3C6H4 |
p-FC6H4 |
62 |
5 |
2e
|
p-CH3C6H4 |
m-NO2C6H4 |
53 |
6 |
2f
|
p-FC6H4 |
p-ClC6H4 |
55 |
7 |
2g
|
p-ClC6H4 |
m-CH3C6H4 |
50 |
8 |
2h
|
p-ClC6H4 |
p-CH3OC6H4 |
55c |
9 |
2i
|
p-ClC6H4 |
m-CH3C6H4 |
57 |
10 |
2j
|
p-ClC6H4 |
p-CH3C6H4 |
60c |
11 |
2k (2k′) |
p-NO2C6H4 |
p-CH3C6H4 |
52 (10) |
12 |
2l
|
p-NO2C6H4 |
p-CH3OC6H4 |
53 |
For demonstrating the synthetic values of this domino Diels–Alder reaction, isatylidene malononitriles were also employed in the reaction. Under similar reaction conditions, the three-component reaction of indole-2-acetate, aromatic aldehydes and isatylidene malononitriles in toluene in the presence of copper sulfate gave the expected spiro[carbazole-2,3′-indolines] 3a–3i in satisfactory yields (Table 3). In each case, the minor products were also produced in very lower yields. In one case, the minor product 3i′ was successfully isolated in 10% yield. It should be noticed that the reaction with isatylidene malononitrile afforded spiro[carbazole-2,3′-indoline], while the above reaction with 3-phencylideneoxindoles gave the regio-isomeric spiro[carbazole-3,3′-indolines]. Therefore, this reaction has a high regioselectivity. For elucidating the relative configuration of the major products, the single crystal structures of the spiro compounds 3b (Fig. 5), 3f (Fig. s8†) and 3h (Fig. s9†) were successfully determined by the X-ray diffraction method. The three single crystals have the same relative configuration. From Fig. 5, it can be seen that the aryl group and the ethoxycarbonyl group exist in the trans-position. The aryl group of the oxindole moiety stands in the cis-position to the neighbouring ethoxycarbonyl group.
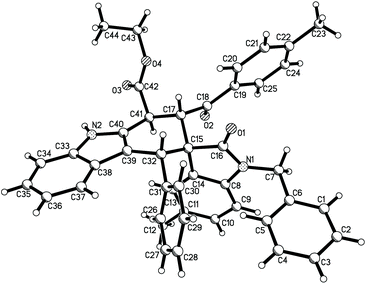 |
| Fig. 5 Single crystal structure of the spiro compound 3b. | |
Table 3 Synthesis of spiro[cazrbazole-2,3′-indolines] 3a–3ia
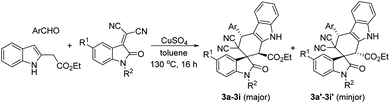
|
Entry |
Compd |
Ar |
R1 |
E |
R2 |
Yieldb (%) |
Reaction conditions: Ethyl indole-2-acetate (0.5 mmol), aldehyde (0.6 mmol), isatylidene malononitrile (0.5 mmol), CuSO4·5H2O (0.2 mmol), toluene (6.0 mL), 130 °C, 16 h.
Isolated yields.
The single crystal structure was determined by X-ray diffraction.
The relative configuration was still presumed.
|
1 |
3a
|
C6H5 |
H |
CN |
Bn |
60 |
2 |
3b
|
p-CH3C6H4 |
CH3 |
CN |
Bn |
71c |
3 |
3c
|
p-CH3C6H4 |
F |
CN |
Bn |
63 |
4 |
3d
|
p-CH3C6H4 |
Cl |
CN |
n-Bu |
62 |
5 |
3e
|
p-CH3OC6H4 |
F |
CN |
Bn |
58 |
6 |
3f
|
p-CH3OC6H4 |
Cl |
CN |
n-Bu |
63c |
7 |
3g
|
p-FC6H4 |
CH3 |
CN |
Bn |
61 |
8 |
3h
|
p-ClC6H4 |
H |
CN |
Bn |
66c |
9 |
3i (3i′) |
p-NO2C6H4 |
CH3 |
CN |
Bn |
52 (10)d |
For explanation of the formation of the spiro compounds, a plausible reaction mechanism was proposed in Scheme 2 on the basis of the previous studies13,18 and the present experiments. Firstly, the alkylation of indole with an aldehyde in the presence of a Lewis acid resulted in a 3-substituted indole (A), which in turn converted to an indole-2,3-quinodimethane (B) by acid catalyzed dehydration. Then, a Diels–Alder reaction between the indole-2,3-quinodimethane (B) and 3-methyleneoxindole afforded the spiro compound 1′ through a endo-type transition state. In the minor isomer 1′, the four larger substituents are in the cis-position on the newly formed cyclohexenyl ring, which has a relatively larger steric hindrance. Thus, the minor isomer can be isomerized to give the major isomer 1 through the epimerization of the ethoxycarbonyl group of the enol form (C) in the reaction system. Levy and coworkers have thoroughly investigated the epimerization mechanism of the different diastereoisomers in the Diels–Alder reaction of the in situ generated indole-2,3-quinodimethane with various chain and cyclic dienophiles.18 Lastly, the stable isomer 1 becomes the major product and the less stable isomer 1′ becomes the minor product. Because the isatylidene malononitrile has a similar steric pattern to that of 3-phenacylideneoxindole, it could be deduced that the formation of the spiro[carbazole-2,3′-indolines] 3 and 3′ obeyed a similar Diels–Alder addition and epimerization process described above. In the case of the three-component reaction with 2-arylidene-1,3-indanedione, a similar Diels–Alder reaction through the endo-type transition state gave the major isomer 2. Because 1,3-indanedione has a symmetric pattern, the energy difference between the endo-type transition state and the exo-type transition state in the Diels–Alder reaction is not very large. Therefore, the reaction through an exo-type transition state gave an intermediate (D), which in turn became the minor isomer 2′ through a similar epimerization process in the reaction system.
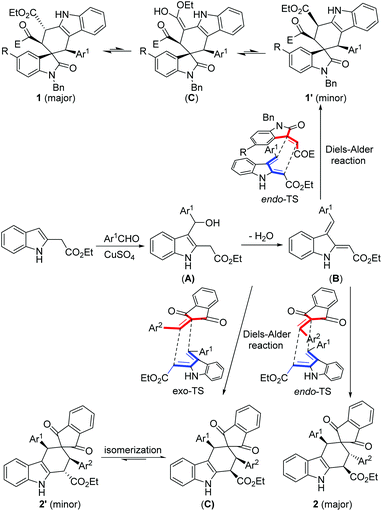 |
| Scheme 2 Proposed reaction mechanism for the three-component reaction. | |
Conclusions
In summary, we have investigated three-component reactions of ethyl indole-2-acetate and aromatic aldehydes with various cyclic dienophiles such as 3-methyleneoxindoles, 2-arylidene-1,3-indanediones and isatylidene malononitriles. This reaction successfully provided a convenient synthetic protocol for diverse polysubstituted spiro[carbazole-3,3′-indolines], spiro[carbazole-3,2′-indenes] and spiro[carbazole-2,3′-indolines] in high yields and with high diastereoselectivity. The stereochemistry of the obtained spiro compounds was clearly elucidated by determination of fourteen single crystal structures. The advantages of this reaction included using readily available starting materials, simple reaction conditions, a broad scope of substrates, satisfactory yields and high diastereoselectivity. The further synthetic applications of this multicomponent reaction in the construction of diverse carbazole derivatives are on the way from our laboratory.
Conflicts of interest
The authors declare no competing financial interest.
Acknowledgements
We are grateful to the National Natural Science Foundation of China (No. 21572196) and the Priority Academic Program Development of Jiangsu Higher Education Institutions (No. BK 2013016) for financial support.
References
-
(a) G. S. Singh and Z. Y. Desta, Chem. Rev., 2012, 112, 6104–6155 CrossRef CAS PubMed;
(b) L. Hong and R. Wang, Adv. Synth. Catal., 2013, 355, 1023–1052 CrossRef CAS;
(c) Y. Liu, H. Wang and J. Wan, Asian J. Org. Chem., 2013, 2, 374–386 CrossRef CAS;
(d) Z. Liu, N. Li, X. Huang, B. Wu, N. Li, C. Kwok, Y. Wang and X. Wang, Tetrahedron, 2014, 70, 2406–2415 CrossRef CAS;
(e) D. Q. Cheng, Y. Ishihara, B. Tan and C. F. Barbas, ACS Catal., 2014, 4, 743–762 CrossRef CAS.
-
(a) Y. T. Yang, J. F. Zhu, G. C. Liao, H. J. Xu and B. Yu, Med. Chem., 2018, 25, 2233–2244 CAS;
(b) N. Ye, H. Y. Chen, E. A. Wold, P. Y. Shi and J. Zhou, ACS Infect. Dis., 2016, 2, 382–392 CrossRef CAS PubMed;
(c) B. Yu, D. Q. Yu and H. M. Liu, Eur. J. Med. Chem., 2015, 97, 673–698 CrossRef CAS PubMed;
(d) B. Yu, Z. Q. Yu, P. P. Qi, D. Q. Yu and H. M. Liu, Eur. J. Med. Chem., 2015, 95, 35–40 CrossRef CAS PubMed;
(e) C. V. Galliford and K. A. Scheidt, Angew. Chem., Int. Ed., 2007, 46, 8748–8758 CrossRef CAS PubMed.
-
(a) L.-T. Shen, P.-L. Shao and S. Ye, Adv. Synth. Catal., 2011, 353, 1943–1948 CrossRef CAS;
(b) T.-Z. Li, Y. Jiang, Y.-Q. Guan, F. Sha and X.-Y. Wu, Chem. Commun., 2014, 50, 10790–10792 RSC;
(c) T.-P. Gao, J.-B. Lin, X.-Q. Hu and P.-F. Xu, Chem. Commun., 2014, 50, 8934–8936 RSC;
(d) Y. Que, T. Li, C. Yu, X.-S. Wang and C. Yao, J. Org. Chem., 2015, 80, 3289–3294 CrossRef CAS PubMed;
(e) J.-L. Han and C.-H. Chang, Chem. Commun., 2016, 52, 2322–2325 RSC;
(f) J. Yan, K. Shi, C. Zhao, L. Ding, S. Jiang, L. Yang and G. Zhong, Chem. Commun., 2018, 54, 1567–1570 RSC.
-
(a) G. J. Mei and F. Shi, Chem. Commun., 2018, 54, 6607–6621 RSC;
(b) G. J. Mei, D. Li, G. X. Zhou, Q. Shi and Z. Cao, Chem. Commun., 2017, 53, 10030–10033 RSC;
(c) J.-L. Wu, B.-X. Du, Y.-C. Zhang, Y.-Y. He, J.-Y. Wang, P. Wu and F. Shi, Adv. Synth. Catal., 2016, 358, 2777–2790 CrossRef CAS;
(d) Q.-N. Zhu, Y.-C. Zhang, M.-M. Xu, X.-X. Sun, X. Yang and F. Shi, J. Org. Chem., 2016, 81, 7898–7907 CrossRef CAS PubMed;
(e) K. Zhao, Y. Zhi, T. Shu, A. Valkonen, K. Rissanen and D. Enders, Angew. Chem., Int. Ed., 2016, 55, 12104–12108 CrossRef CAS PubMed;
(f) S. Abbaraju, N. Ramireddy, N. K. Rana, H. Arman and J. C. G. Zhao, Adv. Synth. Catal., 2015, 357, 2633–2638 CrossRef CAS.
-
(a) K. Thevissen, A. Marchand, P. Chaltin, E. M. K. Meert and B. P. A. Cammue, Curr. Med. Chem., 2009, 16, 2205–2211 CrossRef CAS PubMed;
(b) R. Hesse, O. Kataeva, A. W. Schmidt and H. J. Knolker, Chem. – Eur. J., 2014, 20, 9504–9509 CrossRef CAS PubMed;
(c) M. S. Shaikh, R. Karpoormath, N. Thapliyal, R. A. Rane, M. B. Palkar, A. M. Faya, H. M. Patel, W. S. Alwan, K. Jain and G. A. Hampannavar, Anti-Cancer Agents Med. Chem., 2015, 15, 1049–1065 CrossRef CAS PubMed;
(d) K. Dhara, T. Mandal, J. Das and J. Dash, Angew. Chem., Int. Ed., 2015, 54, 15831–15835 CrossRef CAS PubMed.
-
(a) Y. D. Zhang, T. Wada and H. J. Sasabe, Mater. Chem., 1998, 8, 809–828 RSC;
(b) H. J. Jiang, J. Sun and J. L. Zhang, Curr. Org. Chem., 2012, 16, 2014–2025 CrossRef CAS;
(c) K. T. Kamtekar, A. P. Monkman and M. R. Bryce, Adv. Mater., 2010, 22, 572–582 CrossRef CAS PubMed;
(d) M. K. Hong, M. K. Ravva, P. Winget and J. L. Bredas, Chem. Mater., 2016, 28, 5791–5798 CrossRef CAS.
-
(a) H.-J. Knolker and R. R. Kethiri, Chem. Rev., 2002, 102, 4303–4427 CrossRef PubMed;
(b) A. W. Schmidt, K. R. Reddy and H. J. Knlker, Chem. Rev., 2012, 112, 3193–3328 CrossRef CAS PubMed;
(c) S. Lancianesi, A. Palmieri and M. Petrini, Chem. Rev., 2014, 114, 7108–7149 CrossRef CAS PubMed;
(d) S. Z. Zhao and R. B. Andrade, J. Am. Chem. Soc., 2013, 135, 13334–13337 CrossRef CAS PubMed.
-
(a) V. P. Kumar, K. K. Gruner, O. Kataeva and H. J. Knolker, Angew. Chem., Int. Ed., 2013, 52, 11073–11077 CrossRef CAS PubMed;
(b) S. H. Cho, J. Yoon and S. Chang, J. Am. Chem. Soc., 2011, 133, 5996–6005 CrossRef CAS PubMed;
(c) A. C. Hernandez-Perez and S. K. Collins, Angew. Chem., Int. Ed., 2013, 52, 12696–12700 CrossRef CAS PubMed;
(d) H. Gao, Q. L. Xu, M. Yousufuddin, D. H. Ess and L. Kurti, Angew. Chem., Int. Ed., 2014, 53, 2701–2705 CrossRef CAS PubMed.
-
(a) C. Liu, X. Q. Han, X. Wang and R. A. Widenhoefer, J. Am. Chem. Soc., 2004, 126, 3700–3701 CrossRef CAS PubMed;
(b) N. Kuroda, Y. Takah ashi, K. Yoshinaga and C. Mukai, Org. Lett., 2006, 8, 1843–1845 CrossRef CAS PubMed;
(c) C. Liu and R. A. Widenhoefer, Org. Lett., 2007, 9, 1935–1938 CrossRef CAS PubMed;
(d) L. L. Anderson, M. A. Kroc, T. W. Reidl and J. W. Son, J. Org. Chem., 2016, 81, 9521–9529 CrossRef CAS PubMed;
(e) F. Zhao, N. Li, Y. F. Zhu and Z. Y. Han, Org. Lett., 2016, 18, 1506–1509 CrossRef CAS PubMed;
(f) S. Z. Zhao and R. B. Andrade, J. Org. Chem., 2017, 82, 521–531 CrossRef CAS PubMed.
-
(a) E. Rossi, G. Abbiati and V. Pirovano, Eur. J. Org. Chem., 2017, 4512–4529 CrossRef CAS;
(b) M. Abualnaja, P. G. Waddell, W. Clegg and M. J. Hall, Tetrahedron, 2016, 72, 5798–5806 CrossRef CAS;
(c) C. Gioia, A. Hauville, L. Bernardi, F. Fini and A. Ricci, Angew. Chem., Int. Ed., 2008, 47, 9236–9239 CrossRef CAS PubMed;
(d) Y. Tao, F. Zhang, C. Y. Tang, X. Y. Wu and F. Sha, Asian J. Org. Chem., 2014, 3, 1292–1301 CrossRef CAS;
(e) L. J. Zhou, B. Xu and J. L. Zhang, Angew. Chem., Int. Ed., 2015, 54, 9092–9096 CrossRef CAS PubMed;
(f) J. W. Ren, Z. F. Zhou, J. A. Xiao, X. Q. Chen and H. Yang, Eur. J. Org. Chem., 2016, 1264–1268 CrossRef CAS.
-
(a) J. Cowell, M. Abualnaja, S. Morton, R. Linder, F. Buckingham, P. G. Waddell, M. R. Probert and M. J. Hall, RSC Adv., 2015, 5, 16125–16152 RSC;
(b) L. J. Wu, H. Huang, P. Dang, Y. Liang and S. F. Pi, RSC Adv., 2015, 5, 64354–64357 RSC;
(c) C. W. Kuo, A. Konala, L. Lin, T. T. Chiang, C. Y. Huang, T. H. Yang, V. Kavala and C. F. Yao, Chem. Commun., 2016, 52, 7870–7873 RSC;
(d) K. S. Rathore, B. S. Lad, H. Chennamsetti and S. Katukojvala, Chem. Commun., 2016, 52, 5812–5815 RSC;
(e) P. Sharma, N. P. Kumar, N. H. Krishna, B. S. D. Prasanna and N. Shankaraiah, Org. Chem. Front., 2016, 3, 1503–1508 RSC.
-
(a) T. Wang and T. R. Hoye, J. Am. Chem. Soc., 2016, 138, 13870–13873 CrossRef CAS PubMed;
(b) F. Sha, Y. Tao, C. Y. Tang, F. Zhang and X. Y. Wu, J. Org. Chem., 2015, 80, 8122–8133 CrossRef CAS PubMed;
(c) W. Dai, X. L. Jiang, J. Y. Tao and F. Shi, J. Org. Chem., 2016, 81, 185–192 CrossRef CAS PubMed;
(d) S. Chen, Y. Li, P. Ni, H. Huang and G. J. Deng, Org. Lett., 2016, 18, 5384–5387 CrossRef CAS;
(e) S. P. Chen, Y. X. Li, P. H. Ni, B. C. Yang, H. W. Huang and G. J. Deng, J. Org. Chem., 2017, 82, 2935–2942 CrossRef CAS PubMed.
-
(a) B. Tan, G. Hernandez-Torres and C. F. Barbas, J. Am. Chem. Soc., 2011, 133, 12354–12357 CrossRef CAS PubMed;
(b) H. F. Zheng, P. He, Y. B. Liu, Y. L. Zhang, X. H. Liu, L. L. Lin and X. M. Feng, Chem. Commun., 2014, 50, 8794–8796 RSC;
(c) R. Y. Yang, J. Sun, Y. Tao, Q. Sun and C. G. Yan, J. Org. Chem., 2017, 82, 13277–13287 CrossRef CAS PubMed;
(d) R. Y. Yang, J. Sun, Q. Sun and C. G. Yan, J. Org. Chem., 2018, 83, 5909–5919 CrossRef CAS PubMed;
(e) F. Tan and H. G. Cheng, Chem. Commun., 2019, 55, 6151–6164 RSC.
-
(a) Y. K. Liu, M. Nappi, E. Arceo, S. Vera and P. Melchiorre, J. Am. Chem. Soc., 2011, 133, 15212–15218 CrossRef CAS PubMed;
(b) Y. Wang, M. S. Tu, L. Yin, M. Sun and F. Shi, J. Org. Chem., 2015, 80, 3223–3232 CrossRef CAS PubMed;
(c) J. W. Ren, J. Wang, J. A. Xiao, J. Li, H. Y. Xiang, X. Q. Chen and H. Yang, J. Org. Chem., 2017, 82, 6441–6449 CrossRef CAS PubMed;
(d) E. Siddalingamurthy, K. M. Mahadevan, J. N. Masagalli and H. N. Harishkumar, Tetrahedron Lett., 2013, 54, 5591–5596 CrossRef CAS;
(e) L. J. Huang, J. Weng, S. Wang and G. Lu, Adv. Synth. Catal., 2015, 357, 993–1003 CrossRef CAS.
-
(a) P. Sharma, N. P. Kumar, N. H. Krishna, D. Prasanna, B. Sridhar and N. Shankaraiah, Org. Chem. Front., 2016, 3, 1503–1508 RSC;
(b) Z. H. You, Y. H. Chen, Y. Tang and Y. K. Liu, Org. Lett., 2018, 20, 6682–6686 CrossRef CAS PubMed.
-
(a) J. Sun, Y. Sun, H. Gong, Y. J. Xie and C. G. Yan, Org. Lett., 2012, 14, 5172–5175 CrossRef CAS PubMed;
(b) J. Sun, Y. J. Xie and C. G. Yan, J. Org. Chem., 2013, 78, 8354–8365 CrossRef CAS;
(c) H. Gao, J. Sun and C. G. Yan, J. Org. Chem., 2014, 79, 4131–4136 CrossRef CAS PubMed;
(d) Y. Han, Y. J. Sheng and C. G. Yan, Org. Lett., 2014, 16, 2654–2657 CrossRef CAS PubMed;
(e) J. Sun, L. Chen, H. Gong and C. G. Yan, Org. Biomol. Chem., 2015, 13, 5905–5917 RSC;
(f) L. Chen, J. Sun, J. Xie and C. G. Yan, Org. Biomol. Chem., 2016, 14, 6497–6507 RSC.
-
(a) R. G. Shi, X. H. Wang, R. Liu and C. G. Yan, Chem. Commun., 2016, 52, 6280–6283 RSC;
(b) J. Cao, J. Sun and C. G. Yan, Org. Biomol. Chem., 2018, 16, 4170–4175 RSC;
(c) R. Z. Liu, R. G. Shi, J. Sun and C. G. Yan, Org. Chem. Front., 2017, 4, 354–357 RSC;
(d) J. Sun, Y. Zhang, R. G. Zhang and C. G. Yan, Org. Biomol. Chem., 2019, 17, 3978–3983 RSC;
(e) J. Cao, F. Yang, J. Sun, Y. Huang and C. G. Yan, J. Org. Chem., 2019, 84, 622–635 CrossRef CAS PubMed.
-
(a) K. Diker, M. Maindreville, D. Royer, F. Le Provost and J. Levy, Tetrahedron Lett., 1999, 40, 7463–7467 CrossRef CAS;
(b) D. Royer, Y. S. Wong, S. Ple, A. Chiaroni and K. Diker, Tetrahedron, 2008, 64, 9607–9618 CrossRef CAS;
(c) B. Laudet, V. Moucadel, R. Prudent, O. Filhol, Y. S. Wong, D. Royer and C. Cochet, Mol. Cell. Biochem., 2008, 316, 63–69 CrossRef CAS PubMed;
(d) L. Kroger and B. Wunsche, Eur. J. Org. Chem., 2018, 6297–6303 CrossRef.
-
(a) C. N. Reddy, V. L. Nayak, G. S. Mani, J. S. Kapure, P. R. Adiyala, R. A. Maurya and A. Kamal, Bioorg. Med. Chem. Lett., 2015, 25, 4580–4586 CrossRef CAS PubMed;
(b) S. J. Edeson, J. L. Jiang, S. Swanson, P. A. Procopiou, H. Adams, A. Meijer and J. Harrity, Org.
Biomol. Chem., 2014, 12, 3201–3210 RSC.
Footnote |
† Electronic supplementary information (ESI) available: The copies of the 1H and 13C NMR spectra for all new products. CCDC 1942765 (1a), 1942766 (1e), 1942767 (1g), 1942768 (1k), 1942769 (1l), 1942770 (2a), 1942771 (2b), 1942772 (2h), 1942773 (2j), 1942774 (3b), 1942775 (3f), 1942776 (3h), 1965666 (1g′) and 1964704 (2b′). For ESI and crystallographic data in CIF or other electronic format see DOI: 10.1039/c9ob02013f |
|
This journal is © The Royal Society of Chemistry 2020 |
Click here to see how this site uses Cookies. View our privacy policy here.