DOI:
10.1039/C9NA00760A
(Paper)
Nanoscale Adv., 2020,
2, 1352-1357
Porous nickel doped titanium dioxide nanoparticles with improved visible light photocatalytic activity
Received
6th December 2019
, Accepted 23rd February 2020
First published on 29th February 2020
Abstract
A green hydrothermal synthesis route to prepare a porous nickel doped titanium dioxide (Ni–TiO2) nanostructured photocatalyst has been developed in this research. The results show that Ni doping can greatly increase the visible light photocatalytic performance of TiO2 through the introduction of impurity bands in the band gap of TiO2. After 5 cycles of reuse, Ni–TiO2 nanoparticles still show stable photocatalytic activity for MB degradation. The Ni–TiO2 nanoparticles developed in the present study are expected to have great potential applications in wastewater treatment due to the advantages of strong visible light photocatalytic performance, a simple synthetic process and high cycle utilization performance.
1. Introduction
Due to the prosperity of modern industries, especially the ones dealing with plastics, paper and textile dying, a huge amount of wastewater with various kinds of effluents is discharged, resulting in a great crisis in the acquirement of fresh water.1 Organic dye pollutants, one of the main produced effluents, can seriously disturb and destroy the ecological balance, leaving a heavy negative impact on the living, both human beings and plants.2 To mitigate the above mentioned crisis, a great number of studies have focused on dye wastewater treatment, and various strategies have been developed, such as biodegradation,3 chlorination,4 electrochemical,5 photocatalytic6–10 and adsorption11,12 methods. As one of the most effective methods, heterogeneous photocatalysis can greatly facilitate the oxidation of the pollutants and the by-products of hazardous organic pollutants.12 These catalysts typically have an excellent capability to convert photon energy into chemical energy which is favorable for the decomposition of the main toxic organic contaminants. Among these catalysts, TiO2 has been proved to be the most effective one due to its first usage in heterogeneous photocatalysis under UV light irradiation by Fujishima and Honda in 1972.13 Afterwards, photocatalysis with TiO2 catalysts became a research hotspot to decay the harmful chemical effluents present in wastewater.14,15 After several decades of development, anatase TiO2 is now considered to be one of the most common photocatalysts with high photocatalytic activity.16
TiO2 has a favorable band gap, good chemical stability, good photostability, and high corrosion resistance.17,18 TiO2 is also one of the most noticeable photocatalysts with particular properties: it is a recoverable and reusable catalyst and can offer an eco-friendly and non-toxic approach for dye wastewater treatment. The photocatalytic activity of TiO2 is based on the mechanism of the formation of electron/hole (e−/h+) pairs under the illumination of light which can initiate chemical reactions by generating radical species on the surface of TiO2.19 However, its poor efficiency in response to visible light limits its application due to the hindrance of the large band gap to catalyst efficiency under natural sunlight illumination which mostly contains visible regions.20
Doping of TiO2 with different transition metals (Fe, Mn, Cu, Ni, etc.) can enhance the degradation under visible light irradiation, which has been successfully applied in wastewater treatment.21 The reducing of the band gap of the catalysts has been achieved by doping metals through different processes. Benjwal et al. reported that a Zn and Mn co-doped TiO2 photocatalyst showed high activity and excellent adsorption properties in low concentration aqueous solutions.22 Copper phthalocyanine (CuPc) doped TiO2 was confirmed to be an efficient and stable photocatalyst for degradation of methylene blue from aqueous solution under solar light irradiation.23 The doping of TiO2 with other transition metals such as Fe, Ni and Co has also been employed in various applications.24–26 However, the applications of doped TiO2 are still limited by their high cost and relatively low stability.
To obtain highly effective TiO2 photocatalysts, the synthesis techniques need to be well controlled. In this work, novel Ni–TiO2 nanoparticles have been developed using a green hydrothermal-synthesis route. Different from traditional TiO2 preparation techniques, this synthesis route is easy to be operated and could save time. Meanwhile, the novel Ni–TiO2 nanoparticles exhibit outstanding performance on adsorption of MB dye from aqueous solutions in darkness and high photocatalytic activity towards MB dye under visible light. The catalyst also exhibits extremely high cycle performance and recyclability. The synthesis strategy presented in this work can prepare materials with outstanding properties and will show potential application in water treatment systems.
2. Experimental
2.1 Materials
Butyl titanate ([CH3(CH2)3O]4Ti), absolute ethyl alcohol (C2H5OH), hydrochloric acid (HCl), ammonium hydroxide (NH3·H2O), nickel nitrate (Ni(NO3)2·6H2O), and methylene blue (MB), were all purchased from Sinopharm Reagent Co Ltd. All the chemicals were of analytical grade and used without further purification. Deionized water was used throughout for the preparation of all the experimental solutions.
2.2 Preparation of TiO2 and Ni–TiO2 nanoparticles
Tetrabutyl titanate (10 mL) and absolute ethyl alcohol (10 mL) were mixed to obtain solution A. Absolute ethyl alcohol (20 mL) and deionized water (100 mL) were mixed to obtain solution B. Solution A was then added into solution B dropwise under magnetic stirring for 30 min. Then, the pH value was adjusted to 9 by ammonium hydroxide. After homogenization for 30 min, the mixed solution was transferred into a Teflon-lined autoclave for crystallization at 140 °C for 4 h. The resulting product was washed with ethyl alcohol and deionized water 3 times each. Then the nanoparticles were separated from the liquid phase by centrifugation to remove the remaining compounds. The final product was dried at 80 °C overnight to obtain TiO2 powders. The synthetic steps for Ni-(3 wt%) TiO2 nanoparticles were little different from the above. In step three, solution A and a nickel nitrate solution (0.85 mL, 1 mol L−1) were added into solution B dropwise under magnetic stirring for 30 min.
2.3 Characterization
FT-IR spectra were recorded using a Shimadzu instrument (model 8400S) in the region 4000–400 cm−1. The phase analysis of the as-synthesized products was carried out using X-ray diffraction (XRD, DX-2700) with Cu-Kα radiation (λ = 1.5406 Å). UV-vis-NIR absorption spectra of the samples were recorded using a UV-1800 spectrophotometer (Shimadzu). SEM images were obtained using a S-4800 instrument (Hitachi, Japan). The specific surface area was calculated by the Brunauer–Emmett–Teller (BET) method, and the pore size distribution was obtained using the Barrett–Joyner–Halenda (BJH) model using a Micromeritics ASAP 2020 adsorption analyzer.
2.4. Photocatalytic experiments
Degradation of MB was used as an indicator for the photocatalytic activity of the TiO2 nanoparticles. The prepared TiO2 nanoparticles were immersed in 10 mg L−1 MB solution and were allowed to completely equilibrate with MB for 20 min in darkness. Then the system was irradiated by simulated solar light (Xe lamp, 300 W) or UV light. 10 mL of solution was taken and analysed at different reaction times (every 15 min) using a UV-1800 spectrophotometer.
3. Results and discussion
3.1 FTIR spectra
Fig. 1 shows the FTIR spectra of the TiO2 and Ni–TiO2 nanoparticles. The strong absorption bands at 662 and 704 cm−1 might be due to the Ti–O vibrations in the TiO2 lattice. Furthermore, a broad absorption band in the region of 3000–3500 cm−1 can be assigned to the surface-bound hydroxyl groups and their stretching vibration on the surface of TiO2.27 A second adsorption band at 1000–1700 cm−1 is assigned to surface-adsorbed water molecules (H–O–H bending, 1633 cm−1).28 It can confirm a strong interaction of water molecules with the TiO2 surface to form a number of broad OH– stretching vibrations.22 A broad intense vibration region at 1000–1200 cm−1 is credited to the Ti–O–Ti vibration. Moreover, occurrence of bands between 1300–1500 cm−1 for Ni–TiO2 nanoparticles indicates the presence of a small amount of organic material in the sample.29 With an increase in Ni concentration, the shift to lower wavenumbers of the Ti–O–Ti band could be attributed to the increase in powder particle size.30
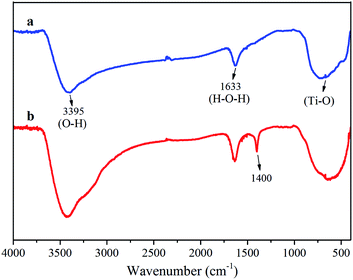 |
| Fig. 1 FT-IR spectral analysis of (a) TiO2 and (b) Ni–TiO2 nanoparticles. | |
3.2 Phase analysis and morphology
XRD patterns of TiO2 and Ni–TiO2 nanoparticles are shown in Fig. 2A. The Ni–TiO2 sample exhibits peaks at 25.28°, 37.80°, 48.05°, 53.89°, 55.06°, 62.69°, 70.31°, and 75.03°, corresponding to the anatase phase (JCPDF 21-1272), with no other phases. In addition, peaks corresponding to Ni oxides are not detected. These results further indicate that Ni ions have been successfully doped into TiO2 nanoparticles.2Fig. 2B shows the spherically shaped Ni doped TiO2 nanostructures. Compared with the pure TiO2 image (Fig. 2C), Ni–TiO2 shows homogeneous nanoparticles with sizes of 20–30 nm.
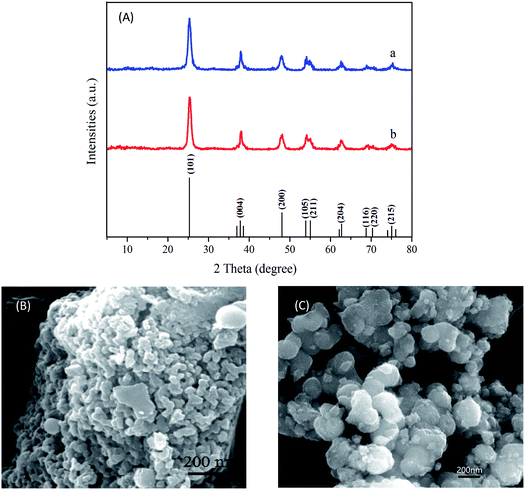 |
| Fig. 2 (A) XRD patterns of (a) TiO2 and (b) Ni–TiO2 nanoparticles; (B) morphology of Ni–TiO2; (C) morphology of TiO2. | |
3.3 UV-vis spectral analysis
The electronic structure of the samples that furnishes the optical properties (e.g., absorption and band gap) through the irradiating light intensity was determined by UV-vis spectral analysis,17 as shown in Fig. 3. In the absorption spectra, it is noticeable that the optical absorption edge of the pure TiO2 is at 400 nm. The band gap of TiO2 is 3.21 eV which is favorable to produce electron–hole pairs under the UV light irradiation. However, the pure TiO2 can not degrade dyes under visible light. Compared to pure TiO2, Ni–TiO2 nanoparticles exhibit a broad absorption covering the range as shown in Fig. 3b, which can be ascribed to the doping energy levels caused by the doped Ni in the band gap of TiO2.
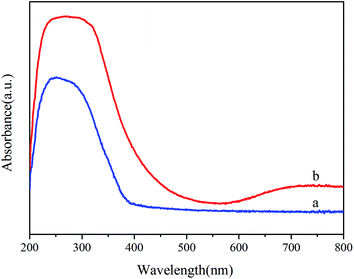 |
| Fig. 3 UV-visible absorption spectra of (a) TiO2 and (b) Ni–TiO2 samples. | |
3.4 Nitrogen adsorption–desorption isotherm of Ni–TiO2
The nitrogen adsorption–desorption isotherm and BJH pore size distribution curve (inset) of Ni–TiO2 are shown in Fig. 4, which displays a type-IV isotherm with a specific surface area of 124.02 m2 g−1. This implies that the pores within the materials are mainly within the mesoporous range. The pore size distribution is calculated using the BJH method (desorption curve).31 The pore-size distribution of Ni–TiO2 shows that the pore diameters distribution (Fig. 4 inset) has a peak at about 9 nm, indicating that Ni–TiO2 has a mesoporous structure. These small pores can enhance photocatalytic activities by favoring the adsorption of small dye molecules on the active surface.
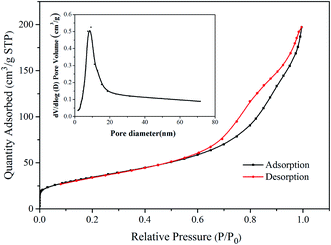 |
| Fig. 4 Nitrogen adsorption–desorption isotherms and pore size distribution curve of Ni–TiO2. | |
3.5 MB decomposition capacity under solar and UV light
Fig. 5 depicts the photocatalytic degradation of MB using TiO2 and Ni–TiO2 nanoparticles under UV and solar light irradiation as a function of time with an initial MB concentration of 10 mg L−1. The degradation efficiencies of Ni–TiO2 nanoparticles under solar and UV after 60 min irradiation are found to be 92.7% and 96.3%, respectively. However, the corresponding degradation efficiencies are only 85.9% and 27.7% for pure TiO2. It can be found that Ni doping can increase the visible light degradation performance greatly. The TiO2 catalyst shows very weak photocatalytic performance for MB degradation under solar light irradiation. After Ni doping, the visible light photocatalytic performance increases to a similar level compared with that under UV light. The result demonstrates that Ni doping can improve the photocatalytic activity of TiO2 nanoparticles under visible light.
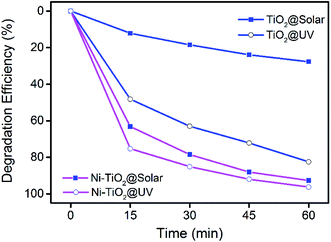 |
| Fig. 5 Photodegradation of MB dye using TiO2 and Ni–TiO2 nanoparticles under UV and solar light irradiation. | |
3.6 Photocatalytic performance stability
The stability is also important for the practical application of the photocatalyst. Therefore, the cyclic stability of Ni–TiO2 nanoparticles was investigated by monitoring the catalytic activity during successive cycles of degradation. As shown in Fig. 6, after a five cycles test, the Ni–TiO2 nanoparticles exhibit a very stable photocatalytic performance without any significant deactivation, thereby demonstrating high stability after multiple reuse cycles.
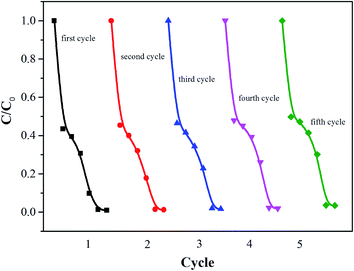 |
| Fig. 6 Photocatalytic stability test of Ni–TiO2 nanoparticles. | |
3.7 Photocatalytic mechanism of Ni–TiO2
The plausible mechanism of the photocatalytic activity of the synthesized Ni–TiO2 nanoparticles can be explained by the energy band gap structure of TiO2 shown in Fig. 7. The direct excitation of an electron from the valence band (VB) to the conduction band (CB) in the presence of visible light is not possible due to the broad band gap (3.21 eV) of pure TiO2. Through the incorporation of Ni ions into the TiO2 lattice, the band gap of TiO2 decreases due to the formation of impurity levels below the CB in the band gap, then the electrons can transfer from the VB to these energy levels. These electrons travel to the surface and are adsorbed by O2 and produce ˙O2 ions, which can further convert to the strong redox species ˙OH ions.32 These redox ions are responsible for the degradation of the surface adsorbed hazardous MB.22
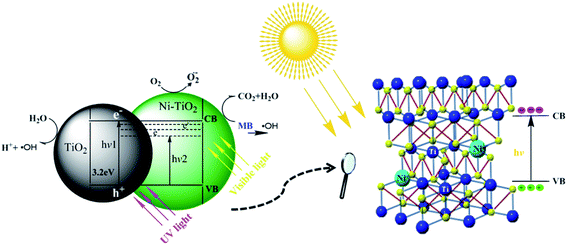 |
| Fig. 7 Possible mechanism of the MB degradation by Ni–TiO2 nanoparticles. | |
4. Conclusions
Ni–TiO2 nanoparticles were synthesized by a green hydrothermal-synthesis route and characterized in detail. The activities of the synthesized nanoparticles were studied through MB photocatalytic degradation. The results demonstrate that Ni doping can greatly increase the visible light photocatalytic performance of TiO2 through the introduction of impurity bands in the band gap of TiO2. After 5 cycles of reuse, Ni–TiO2 nanoparticles still show stable photocatalytic activity for MB degradation. Thus the Ni–TiO2 nanoparticles developed in the present study are expected to have great potential applications in wastewater treatment due to the advantages of strong visible light photocatalytic performance, a simple synthetic process and high cycle utilization performance.
Conflicts of interest
There are no conflicts to declare.
Acknowledgements
The authors gratefully acknowledge the support from National Natural Science Foundation of China (51478406, 51508293), 973 Program (2015CB6555100), 111 Program and Qingdao Applied Research Project (17-1-1-87-jch).
References
- T. M. David, P. Wilson, R. Mahesh, S. Dhanavel, S. Hussain, S. J. M. Boby, A. Stephen, C. Ramesh and P. Sagayaraj, Investigating the photocatalytic degradation property of Pt, Pd and Ni nanoparticles-loaded TiO2 nanotubes powder prepared via rapid breakdown anodization, Environ. Technol., 2017, 1, 1479–1487 Search PubMed.
- X. H. Li, Y. Wu, Y. H. Shen, Y. Sun, Y. Yang and A. J. Xie, A novel bifunctional Ni-doped TiO2 inverse opal with enhanced SERS performance and excellent photocatalytic activity, Appl. Surf. Sci., 2018, 427, 739–744 CrossRef CAS.
- C. Yang, J. S. Yu, Q. S. Li and Y. M. Yu, Facile synthesis of monodisperse porous ZnO nanospheres for organic pollutant degradation under simulated sunlight irradiation: the effect of operational parameters, Mater. Res. Bull., 2017, 87, 72–83 CrossRef CAS.
- S. Yaparatne, C. P. Tripp and A. Amirbahman, Photodegradation of taste and odor compounds in water in the presence of immobilized TiO2–SiO2 photocatalysts, J. Hazard. Mater., 2018, 346, 208–217 CrossRef CAS PubMed.
- S. Kumar, B. Ahmed, A. K. Ojha, J. Das and A. Kumar, Facile synthesis of CdO nanorods and exploiting its properties towards supercapacitor electrode materials and low power UV irradiation driven photocatalysis against methylene blue dye, Mater. Res. Bull., 2017, 90, 224–231 CrossRef CAS.
- S. Y. Guo, S. Han, B. Chi, J. Pu and J. Li, Structurally controlled ZnO/TiO2 heterostructures as efficient photocatalysts for hydrogen generation from water without noble metals: The role of microporous amorphous/crystalline composite structure, J. Power Sources, 2014, 245, 979–985 CrossRef CAS.
- S. Y. Guo, T. J. Zhao, J. Z. Quan and S. Han, Self-assembly synthesis of precious-metal-free 3D ZnO nano/micro spheres with excellent photocatalytic hydrogen production from solar water splitting, J. Power Sources, 2015, 293, 17–22 CrossRef CAS.
- S. Y. Guo, S. Han, B. Chi, J. Pu and J. Li, A facile low-temperature approach to designing controlled amorphous-based titania composite photocatalysts with excellent noble-metal-free photocatalytic hydrogen production, ACS Appl. Mater. Interfaces, 2014, 6, 4743–4751 CrossRef CAS PubMed.
- S. Y. Guo, S. Han, B. Chi, J. Pu and J. Li, Synthesis and characterization of nitrogen and phosphate codoped titanium dioxide with excellent visible-light photocatalytic activity, J. Alloys Compd., 2012, 544, 50–54 CrossRef CAS.
- A. A. Ismail, I. Abdelfattah, M. Faisal and A. Helal, Efficient photodecomposition of herbicide imazapyr over mesoporous Ga2O3–TiO2 nanocomposites, J. Hazard. Mater., 2018, 342, 519–526 CrossRef CAS PubMed.
- L. N. Liu, J. G. Dai, T. J. Zhao, S. Y. Guo, D. S. Hou, P. Zhang, J. Shang, S. Wang and S. Han, A novel Zn(II) dithiocarbamate/ZnS nanocomposite for highly efficient Cr6+ removal from aqueous solutions, RSC Adv., 2017, 7, 35075–35085 RSC.
- D. Zhao, G. Sheng, C. Chen and X. Wang, Enhanced photocatalytic degradation of methylene blue under visible irradiation on graphene@TiO2 dyade structure, Appl. Catal., B, 2012, 111–112, 303–308 CrossRef CAS.
- A. Fujishima and K. Honda, Photolysis-decomposition of water at surface of an irradiated semiconductor, Nature, 1972, 238, 37–38 CrossRef CAS PubMed.
- J. Schneider, M. Matsuoka, M. Takeuchi, J. Zhang, Y. Horiuchi, M. Anpo and D. W. Bahnemann, Understanding TiO2 photocatalysis: mechanisms and materials, Chem. Rev., 2014, 114, 9919–9986 CrossRef CAS PubMed.
- M. Ahmed and X. Guo, A review of metal oxynitrides for photocatalysis, Inorg. Chem. Front., 2016, 3, 578–590 RSC.
- R. W. Matthews, Photocatalytic oxidation of organic contaminants in water: an aid to environmental preservation, Pure Appl. Chem., 1992, 64, 1285–1290 CAS.
- A. A. Ismail, I. Abdelfattah, A. Helal, S. A. Al-Sayari, L. Robben and D. W. Bahnemann, Ease synthesis of mesoporous WO3–TiO2 nanocomposites with enhanced photocatalytic performance for photodegradation of herbicide imazapyr under visible light and UV illumination, J. Hazard. Mater., 2016, 307, 43–54 CrossRef CAS PubMed.
- X. Yang, L. Zhao, K. Lv, B. Dong and S. Wang, Enhanced efficiency for dye-sensitized solar cells with ZrO2 as a barrier layer on TiO2 nanofibers, Appl. Surf. Sci., 2019, 469, 821–828 CrossRef CAS.
- W. He, Z. Fang, K. Zhang, X. Li, D. Ji, X. Jiang, C. Qiu and K. Guo, Continuous synthesis of a co-doped TiO2 photocatalyst and its enhanced visible light catalytic activity using a photocatalysis microreactor, RSC Adv., 2015, 5, 54853–54860 RSC.
- W. H. Feng, J. Z. Fang, L. X. Zhang, S. Y. Lu, S. X. Wu, C. Cheng, Y. Chen, Y. Ling and Z. Q. Fang, Plasmonic metallic Bi deposited Bi12SiO20 crystals with rich oxygen vacancies for enhanced photocatalytic degradation of RhB and 2,4-DCP, Mater. Res. Bull., 2017, 94, 45–53 CrossRef CAS.
- S. N. R. Inturi, T. Boningari, M. Suidan and P. G. Smirniotis, Visible-light-induced photodegradation of gas phase acetonitrile using aerosol-made transition metal (V, Cr, Fe, Co, Mn, Mo, Ni, Cu, Y, Ce, and Zr) doped TiO2, Appl. Catal., B, 2014, 144, 333–342 CrossRef CAS.
- P. Benjwal and K. K. Kar, Removal of methylene blue from wastewater under a low power irradiation source by Zn, Mn co-doped TiO2 photocatalysts, RSC Adv., 2015, 5, 98166–98176 RSC.
- B. Cabir, M. Yurderi, N. Caner, M. S. Agirtas, M. Zahmakiran and M. Kaya, Methylene blue photocatalytic degradation under visible light irradiation on copper phthalocyanine-sensitized TiO2 nanopowders, Mater. Sci. Eng., B, 2017, 224, 9–17 CrossRef CAS.
- G. Murtaza, R. Ahmad, M. S. Rashid, M. Hassan, A. Hussnain, M. AzharKhan, M. E. Ul Haq, M. A. Shafiqu and S. Riaz, Structural and magnetic studies on Zr doped ZnO diluted magnetic semiconductor, Curr. Appl. Phys., 2014, 14, 176–181 CrossRef.
- W. Zhang, W. Zhou, J. H. Wright, Y. N. Kim, D. Liu and X. Xiao, Mn-doped TiO2 nanosheet-based spheres as anode materials for lithium-ion batteries with high performance at elevated temperatures, ACS Appl. Mater. Interfaces, 2014, 6, 7292–7300 CrossRef CAS PubMed.
- D. Das, D. Banerjee, B. Das, N. S. Das and K. K. Chattopadhyay, Effect of cobalt doping into graphitic carbon nitride on photo induced removal of dye from water, Mater. Res. Bull., 2017, 89, 170–179 CrossRef CAS.
- R. Sharma and K. K. Kar, Effects of structural disorder and nitrogen content on the oxygen reduction activity of polyvinylpyrrolidone-derived multi-doped carbon, J. Mater. Chem. A, 2015, 3, 11948–11959 RSC.
- W. C. Hung, S. H. Fu, J. J. Tseng, H. Chu and T. H. Ko, Study on photocatalytic degradation of gaseous dichloromethane using pure and iron ion-doped TiO2 prepared by the sol-gel method, Chemosphere, 2007, 66, 2142–2151 CrossRef CAS PubMed.
- P. Singla, O. P. Pandey and K. Singh, Study of photocatalytic degradation of environmentally harmful phthalate esters using Ni-doped TiO2 nanoparticles, Int. J. Environ. Sci. Technol., 2016, 13, 849–856 CrossRef CAS.
- H. Khojasteh, M. S. Niasari and S. M. Derazkola, Synthesis, characterization and photocatalytic properties of nickel-doped TiO2 and nickel titanate nanoparticles, J. Mater. Sci.: Mater. Electron., 2016, 27, 3599–3607 CrossRef CAS.
- A. Ajmal, I. Majeed, R. N. Malik, H. Idriss and M. A. Nadeem, Principles and mechanisms of photocatalytic dye degradation on TiO2 based photocatalysts: a comparative overview, RSC Adv., 2014, 4, 37003–37026 RSC.
- E. O. Oseghe, P. G. Ndungu and S. B. Jonnalagadda, Synthesis ofmesoporous
Mn/TiO2 nanocomposites and investigating the photocatalytic properties in aqueous systems, Environ. Sci. Pollut. Res., 2015, 22, 211–222 CrossRef CAS PubMed.
Footnote |
† These authors contributed equally. |
|
This journal is © The Royal Society of Chemistry 2020 |