DOI:
10.1039/C9NA00671K
(Communication)
Nanoscale Adv., 2020,
2, 166-170
Regioselective thiocyanation of corroles and the synthesis of gold nanoparticle–corrole assemblies†‡
Received
23rd October 2019
, Accepted 20th November 2019
First published on 21st November 2019
Abstract
Herein we demonstrate a synthetic protocol for the regioselective thiocyanation of corroles. To the best of our knowledge, thiocyanato appended corrole has never been reported earlier. The resulting thiocyanato appended corrole turned out to be a good corrole based precursor for the facile synthesis of thiol protected gold nanoparticles (Au NPs). The ligand system acts as a good bidentate framework and passivates the gold surface. A strong electronic interaction between the corrole and the gold nanoparticles is manifested by their unique photo physical properties and it also confirms that the binding through β-substitutions has a more pronounced effect even though the corrole rings are face-off to the gold surface.
Gold nanoparticles (Au NPs) are extensively used in various areas like catalysis,1 medicine,2 sensors,3 fuel cells,4 surface enhanced Raman spectroscopy,5 probes for transmission electron microscopy,6 and antibiotics.7 One can easily coat the surface of Au NPs with various molecules, either small or big, and thus can fine tune their physical and chemical properties.8 In this context, Au NPs bearing π-conjugated molecules are ideal platforms for various nanoelectronic devices.9 Thus there is widespread research interest in studying porphyrinoid coated gold nanoparticles.10 Feringa et al. demonstrated that porphyrins having π-conjugation can coordinate with the Au (111) surface via intramolecular interactions (via linker groups) and also via interfacial interactions (via inner nitrogens).11 Corrole, a ring contracted version of porphyrin, has interesting and different photo physical properties in comparison to porphyrin.12 Thus, it is expected that corrole coated Au NPs will show great promise in designing newer varieties of functional materials having diverse applications. The importance of the multi-dentate ligand in binding the surface of the Au NPs in a specific orientation was demonstrated independently by Ohyama et al.13 and Teranishi et al.14 Very few research papers indeed highlight the importance of polydentate ligands as binders of gold nanoparticles.15 Tour et al. reported that organic thiocyanates can easily form assemblies on gold surfaces without the help of any external agent and have several advantages over free thiols or other thiol based precursors.16 Their observations have prompted us to choose the thiocyanato appended corrole as a possible precursor for the synthesis of gold nanoparticle–corrole assemblies. The existing literature reports that meso-substituted porphyrinoids are used exclusively as binders for gold nanoparticles, and the related β-substituted derivatives are rarely used for this purpose. As β-substitution has a more pronounced effect on the electronic structure, we have chosen β-substitution at the corrole periphery. In order to obtain the thiocyanato appended corrole derivative, we have developed a simple and novel protocol for the regio-selective thiocyanation of corrole. To the best of our knowledge, the thiocyanato group has never been inserted at the corrole periphery. We have synthesized corrole coated gold nanoparticles by using a one-phase strategy.13 Corrole and HAuCl4 solutions were mixed together, and then a NaBH4 solution was added and stirred for ∼3 hours. No co-stabilizers (citrate, TOAB, etc.) were used for this purpose. The thiocyanato appended corrole, 3,17-bis(thiocyanato)–5,10,15-triphenylcorrole (1), was prepared by following a strategy developed by our group (Scheme 1).
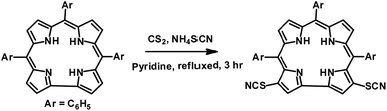 |
| Scheme 1 Synthetic route to 3,17-bis(thiocyanato)–5,10,15-triphenylcorrole, 1. | |
5,10,15-triphenylcorrole (TPC) in a carbon disulfide solution was treated with an excess amount of NH4SCN and excess pyridine. Refluxing for 3 hours resulted in the formation of 1 in good yields (see the ESI‡ and Scheme 1). The newly synthesized thiocyanato appended corrole (1) has been fully characterized using several spectroscopic techniques like CHN analysis, FT-IR analysis, 1H NMR, ESI-mass spectrometry, XPS and single crystal XRD (see the ESI; Fig. S1–S6 and Table S1, S2‡). Considering thiocyanate to be a potent nucleophile, this reaction should be considered a unique class of the nucleophilic aromatic substitution reaction in corrole.17 Paolesse et al. described this class of reactions as nucleophilic aromatic substitution of hydrogens, occurring via two steps. The first step is the formation of a σ-adduct by simple addition of the nucleophile to the corrole ring and the second step is the elimination of hydride. Pyridine is suspected to be a hydride ion abstractor here. The 1H NMR spectrum of 1 exhibits normal chemical shifts in accordance with the ‘twenty one’ aromatic protons in the region δ, ∼8.91–7.78 ppm. Negligible shielding is observed in 1 in comparison with 5,10,15-triphenylcorrole (see ESI Fig. S4‡). The FT-IR spectrum of 1 as a KBr pellet shows a peak at 2154 cm−1 due to C
N group stretching (see ESI Fig. S3‡). The crystal structure of 1 is shown in Fig. 1.
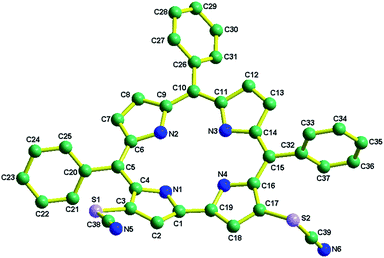 |
| Fig. 1 Perspective view of 1. Hydrogen atoms are omitted for clarity. | |
The crystal system is triclinic, and the unit cell contains two molecules of 1. Important crystallographic parameters for compound 1 are shown in Table S1.‡ Bond distances and angles of 1 are in agreement with those of other FB corrole derivatives.12 Pyrrolic nitrogen atoms have shown deviations from the mean corrole plane (considering the 19-carbon atom plane to be the mean corrole plane) by distances varying from 0.488 to −0.052 Å in 1. The corresponding deviations in 5,10,15-triphenylcorrole (TPC) are 0.128 to −0.195 Å.12v The dihedral angles between the planes of the phenyl rings (meso-substituted phenyl group) and the 19-carbon mean corrole plane vary significantly and are 43.74–78.37°. The corresponding dihedral angles in TPC are 41.74–50.33°.12v Thus, it appears that the insertion of two thiocyanate groups at the β-pyrrolic position induced significant saddling of the corrole framework. The two C
N (for N (5)–C (38) and N (6)–C (39)) bond distances of 1 are 1.13 Å and 1.14 Å and the two S–C
N bond angles (for S (1)–C (38)–N (5) and S (2)–C (39)–N (6)) are 177.66° and 178.85°.
Gold nanoparticles coated with 1 were prepared by addition of NaBH4 to a premixed mixture of 1 and HAuCl4 (see the ESI‡). The newly synthesized 1-Au NPs were characterized by CHN, EDS and FT-IR analysis (see the ESI, Fig. S3 and S7‡). The EDS spectrum of 1-Au NPs shows the presence of all the constituent elements (Au, N, and S). From CHN analysis (see ESI‡), we can roughly estimate that there are 200 corrole ligands on a single Au NP surface. This implies that there are many physisorbed corrole molecules at the surface which do not have direct contact with the gold surface. The UV-Vis spectra of the solid samples of 1 (very broad and red shifted) clearly indicate extensive aggregate formation (Fig. S1‡). The FT-IR spectrum of Au NP–corrole assemblies obtained using a KBr pellet shows the absence of C
N group stretching at 2154 cm−1 (see the ESI, Fig. S3‡). This confirms that the ligand moiety loses the C
N group while binding with the Au surface. Fig. 2 shows the time evolution of the UV-Vis spectra for mixtures of 1 (∼10−6M), NaBH4 (∼10−4M), and HAuCl4 (∼10−5 M) in DMF. The initial spectrum (black colour, colour online) was for pure 1 in the DMF solution. It was observed that the positions of the Soret and Q bands in 1 are negligibly changed upon addition of NaBH4. However, both bands are drastically different in intensity upon gradual addition of the HAuCl4 solution. The red coloured final spectrum (colour online) was obtained after the addition of optimal concentration of the HAuCl4 (∼2 × 10−5M) solution. The final spectrum obtained after 2.5 hours shows a clear signature of the combination of the characteristic Soret band of corrole 1 (as a weak shoulder) and the strong plasmon band of gold nanoparticles. The surface plasmon resonance (SPR) band appears at 525 nm.13,14 The intensity of the Q bands of 1 is drastically reduced but it still remains as a shoulder at around 655 nm. However, the Soret bands are broadened but the shifts of the bands are negligible. A direct comparison of these UV-Vis spectra with that of the FB corrole 1 (prior to Au–thiol contact formation) is not possible because after the interaction of FB corrole 1 with the gold nanoparticles, the thiocyanate group loses the cyanide fragment (after Au–thiol contact formation) and thus the ligand electronic structure is perturbed.
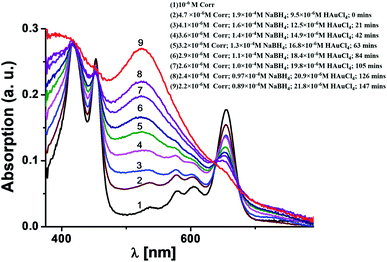 |
| Fig. 2 Time evolution UV-vis spectra of a mixture of 1 (∼10−6M), NaBH4 (∼10−4M), and HAuCl4 (∼10−5 M) in DMF. | |
These results are in complete agreement with those for previously reported porphyrin thioester protected gold nano-particles.14 In line with the previous report by Teranishi et al., our absorption spectra also suggest that a strong electronic interaction exists between the corrole and the gold surface (Fig. 3).14 However, in contrast to them, we believe that in our case the parallel binding mode of corrole to the gold surface is not feasible due to the steric hindrance of the meso-aryl groups. Thus a face-off binding mode of corrole (due to the lack of one meso-carbon) is proposed here. Fig. 4 shows the time evolution fluorescence spectra of a mixture of 1 (∼10−6 M), NaBH4 (∼10−4 M), and HAuCl4 (∼10−5 M) in DMF.
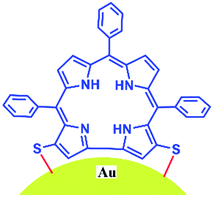 |
| Fig. 3 Plausible structure of gold nano-corrole assemblies. | |
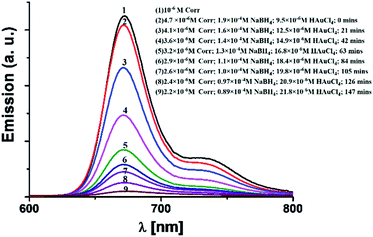 |
| Fig. 4 Time evolution emission spectra of a mixture of 1 (∼10−6 M), NaBH4 (∼10−4M), and HAuCl4 (∼10−5 M) in DMF. | |
The initial spectrum (black colour, colour online) was for pure 1 in the DMF solution. The position of the emission bands is negligibly changed upon addition of NaBH4. However, a drastic change of the corrole fluorescence intensity is observed upon gradual addition of the HAuCl4 solution. The fluorescence quenching is obvious as the corrole molecules transfer their excitation energy to the gold nanoparticles.18 The excited state lifetimes of 1 and 1- Au NPs were found to be 3.69 ns and 1.19 ns, respectively (Fig. 5). Considering that only static quenching is viable,18a the change in the lifetime of corrole 1 on binding with the Au surface is attributed to the change of the ligand structure in 1-Au NPs (the two thiocyanate groups are converted to thiol groups on binding with Au).
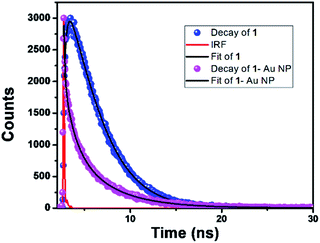 |
| Fig. 5 Fluorescence decay profiles of (a) 1 (—); λem = 672 nm and (b) 1-Au NPs ( ); λem = 672 nm. The red line represents the IRF. | |
A representative TEM image is shown in Fig. 6. One can easily visualize the diffraction contrast fringes and thus it can confirm the crystalline nature of the 1 coated gold nanoparticles.13 Particle size analysis indicates that the mean diameter of the 1 coated gold nanoparticles is ∼6.5 nm.
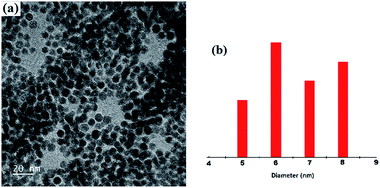 |
| Fig. 6 (a) TEM image of the nanoparticles (the inset shows the diffraction contrast fringes) and (b) histograms of the particle size. | |
Gold nanoparticles coated with 1 are stable under ambient conditions for a few weeks as concluded from the characteristic surface plasmon resonance (SPR) band at 525 nm. This is in contrast with the stability of gold nanoparticles alone obtained through NaBH4 reduction without the use of the stabilizing corrole molecule, 1.13
1-Au NPs were also studied by X-ray photoelectron spectroscopy (XPS). The composition of the organic contributions is 85 at% carbon, 11 at% nitrogen and 4 at% sulphur, approximately matching the expected ratio of 87 : 9 : 4 for 1 with cleaved CN groups. The N 1s signal (Fig. 7) consists of three components which can be assigned to imine nitrogen (398.2 eV, 1.3 at%), amine nitrogen (399.9 eV, 9.6 at%) and amide nitrogen (400.3 eV).
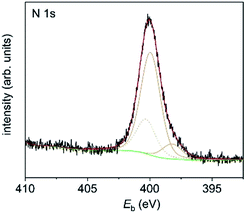 |
| Fig. 7 N 1s XP spectrum of 1-Au NPs on a Si substrate. Measured data are in black, fitted components are in orange, the background is in green and the sum is in red. | |
The latter is most probably due to the residual DMF which is left after synthesis and was therefore removed from the calculation of at% for the organic components. The imine and amine nitrogen contributions can be assigned to the four nitrogen atoms in the corrole framework.19 However, the ratio between amine and imine nitrogen is much higher than expected for pure corrole and might be an indication of extensive π–π stacking. The S 2p signal (Fig. 8) is also composed of three signals, which can be assigned to thiol bound to the gold surface (162.0 eV, 0.8 at%), free thiol (164.0 eV, 1.7 at%) and oxidized thiol (168.1 eV, 1.2 at%).20 As the ratio of corrole ligands per Au NP has been estimated from CHN analysis to be 200
:
1, it is obvious that only a minor number of corrole ligands can bind directly to the gold surface. Thus, many ligands are only adsorbed by π–π stacking and thus have free thiol groups which can easily be oxidized.
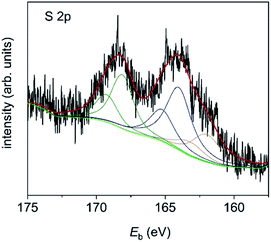 |
| Fig. 8 S 2p spectrum of 1-Au NPs on a Si substrate. Measured data are in black, the fit for bound thiol is in orange, the fit for free thiol is in blue, the fit for oxidized thiol is in dark green, the background is in green and the sum is in red. | |
In conclusion, we have developed a simple and novel protocol for the regio-selective thiocyanation of corrole. The thiocyanato appended corrole 1 acts as a stabilizer for gold nanoparticles. A unique face-off binding mode in corrole (due to the lack of one meso-carbon) is proposed here which is not at all feasible in porphyrins. The corrole 1 and the Au nanoparticle hybrid exhibits interesting photo physical properties. The changes in absorption and emission spectra are remarkable and these also clearly indicate that a strong electronic interaction exists between the corrole and the Au nanoparticles. These studies will certainly be helpful in designing various multi-dentate corrole based ligands in order to stabilize Au nanoparticles with a specific size and functions depending on the choice of corrole framework.
Conflicts of interest
There are no conflicts of interest to declare.
Acknowledgements
Financial support received from the Department of Atomic Energy, (India) is gratefully acknowledged. Authors acknowledge NISER-Bhubaneswar for providing infrastructure and instrumental supports. The authors acknowledge the financial support provided by SERB (Science & Engineering Research Board), India (Grant No: EMR/2016/005484) to carry out this research work. Funding from the DFG, Germany under grant INST 184/106-1 FUGG is acknowledged. The XPS spectrometer is co-financed by the German Science Foundation (INST 184/106-1 FUGG). We would like to acknowledge Dr M. Sadhukhan for TEM Measurement.
Notes and references
-
(a) M.-C. Daniel and D. Astruc, Chem. Rev., 2004, 104, 293–346 CrossRef CAS PubMed;
(b) A. Corma and H. Garcia, Chem. Soc. Rev., 2008, 37, 2096–2126 RSC;
(c) C. T. Campbell, Science, 2004, 306, 234–235 CrossRef CAS PubMed.
-
(a) D. A. Giljohann, D. S. Seferos, W. L. Daniel, M. D. Massich, P. C. Patel and C. A. Mirkin, Angew. Chem., Int. Ed., 2010, 49, 3280–3294 CrossRef CAS PubMed;
(b) E. C. Dreaden, A. M. Alkilany, X. Huang, C. J. Murphy and M. A. El-Sayed, Chem. Soc. Rev., 2012, 41, 2740–2779 RSC;
(c) J. J. Li, L. Zou, D. Hartono, C. N. Ong, B. H. Bay and L. Y. Lanry Yung, Adv. Mater., 2008, 20, 138–142 CrossRef CAS.
-
(a) S. Chah, M. R. Hammond and R. N. Zare, Chem. Biol., 2005, 12, 323–328 CrossRef CAS PubMed;
(b) G. Peng, U. Tisch, O. Adams, M. Hakim, N. Shehada, Y. Y. Broza, S. Billan, R. Abdah-Bortnyak, A. Kuten and H. Haick, Nat. Nanotechnol., 2009, 4, 669 CrossRef CAS PubMed.
-
(a) Y.-C. Lu, Z. Xu, H. A. Gasteiger, S. Chen, K. Hamad-Schifferli and Y. Shao-Horn, J. Am. Chem. Soc., 2010, 132, 12170–12171 CrossRef CAS PubMed;
(b) R. Muszynski, B. Seger and P. V. Kamat, J. Phys. Chem. C, 2008, 112, 5263–5266 CrossRef CAS;
(c) J. Luo, L. Wang, D. Mott, P. N. Njoki, Y. Lin, T. He, Z. Xu, B. N. Wanjana, I. I. S. Lim and C. J. Zhong, Adv. Mater., 2008, 20, 4342–4347 CrossRef CAS.
-
(a) C. E. Talley, J. B. Jackson, C. Oubre, N. K. Grady, C. W. Hollars, S. M. Lane, T. R. Huser, P. Nordlander and N. J. Halas, Nano Lett., 2005, 5, 1569–1574 CrossRef CAS PubMed;
(b) K. L. Wustholz, A.-I. Henry, J. M. McMahon, R. G. Freeman, N. Valley, M. E. Piotti, M. J. Natan, G. C. Schatz and R. P. Van Duyne, J. Am. Chem. Soc., 2010, 132, 10903–10910 CrossRef CAS PubMed;
(c) N. Félidj, J. Aubard, G. Lévi, J. R. Krenn, A. Hohenau, G. Schider, A. Leitner and F. R. Aussenegg, Appl. Phys. Lett., 2003, 82, 3095–3097 CrossRef.
-
(a) T. A. Taton, C. A. Mirkin and R. L. Letsinger, Science, 2000, 289, 1757–1760 CrossRef CAS PubMed;
(b) J. J. Storhoff, R. Elghanian, R. C. Mucic, C. A. Mirkin and R. L. Letsinger, J. Am. Chem. Soc., 1998, 120, 1959–1964 CrossRef CAS;
(c) S. G. Penn, L. He and M. J. Natan, Curr. Opin. Chem. Biol., 2003, 7, 609–615 CrossRef CAS PubMed.
- I. Yacoby and I. Benhar, Infect. Disord.: Drug Targets, 2007, 7, 221–229 CrossRef CAS PubMed.
-
(a) J. S. Lee, M. S. Han and C. A. Mirkin, Angew. Chem., Int. Ed., 2007, 46, 4093–4096 CrossRef CAS PubMed;
(b) G. H. Woehrle, L. O. Brown and J. E. Hutchison, J. Am. Chem. Soc., 2005, 127, 2172–2183 CrossRef CAS PubMed;
(c) S.-Y. Lin, S.-W. Liu, C.-M. Lin and C.-h. Chen, Anal. Chem., 2002, 74, 330–335 CrossRef CAS PubMed;
(d) K.-S. Kim, D. Demberelnyamba and H. Lee, Langmuir, 2004, 20, 556–560 CrossRef CAS PubMed.
-
(a) B. C. Sih and M. O. Wolf, Chem. Commun., 2005, 3375–3384 RSC;
(b) M. Ikeda, N. Tanifuji, H. Yamaguchi, M. Irie and K. Matsuda, Chem. Commun., 2007, 1355–1357 RSC;
(c) J. van Herrikhuyzen, S. J. George, M. R. Vos, N. A. Sommerdijk, A. Ajayaghosh, S. C. Meskers and A. P. Schenning, Angew. Chem., Int. Ed., 2007, 119, 1857–1860 CrossRef;
(d) K. Matsuda, H. Yamaguchi, T. Sakano, M. Ikeda, N. Tanifuji and M. Irie, J. Phys. Chem. C, 2008, 112, 17005–17010 CrossRef CAS.
-
(a) T. Hasobe, H. Imahori, P. V. Kamat, T. K. Ahn, S. K. Kim, D. Kim, A. Fujimoto, T. Hirakawa and S. Fukuzumi, J. Am. Chem. Soc., 2005, 127, 1216–1228 CrossRef CAS PubMed;
(b) T. Akiyama, M. Nakada, N. Terasaki and S. Yamada, Chem. Commun., 2006, 395–397 RSC;
(c) D. Conklin, S. Nanayakkara, T.-H. Park, M. F. Lagadec, J. T. Stecher, M. J. Therien and D. A. Bonnell, Nano Lett., 2012, 12, 2414–2419 CrossRef CAS PubMed;
(d) A. Satake, M. Fujita, Y. Kurimoto and Y. Kobuke, Chem. Commun., 2009, 1231–1233 RSC.
- N. Katsonis, J. Vicario, T. Kudernac, J. Visser, M. M. Pollard and B. L. Feringa, J. Am. Chem. Soc., 2006, 128, 15537–15541 CrossRef CAS PubMed.
- For selected examples see:
(a) E. Vogel, S. Will, A. S. Tilling, L. Neumann, J. Lex, E. Bill, A. X. Trautwein and K. Wieghardt, Angew. Chem., Int. Ed., 1994, 33, 731–735 CrossRef;
(b) Z. Gross, J. Biol. Inorg Chem., 2001, 6, 733–738 CrossRef CAS PubMed;
(c) R. Paolesse, S. Nardis, M. Stefanelli, F. R. Fronczek and M. G. H. Vicente, Angew. Chem., Int. Ed., 2005, 44, 3047–3050 CrossRef CAS PubMed;
(d) R. S. Czernuszewicz, V. Mody, A. Czader, M. Galezowski and D. T. Gryko, J. Am. Chem. Soc., 2009, 131, 14214–14215 CrossRef CAS PubMed;
(e) M. Autret, S. Will, E. V. Caemelbecke, J. Lex, J.-P. Gisselbrecht, M. Gross, E. Vogel and K. M. Kadish, J. Am. Chem. Soc., 1994, 116, 9141–9149 CrossRef CAS;
(f) J. H. Palmer, A. C. Durrell, Z. Gross, J. R. Winkler and H. B. Gray, J. Am. Chem. Soc., 2010, 132, 9230–9231 CrossRef CAS PubMed;
(g) H.-Y. Liu, Y. Fei, Y.-T. Xie, X.-Y. Li and C. K. Chang, J. Am. Chem. Soc., 2009, 131, 12890–12891 CrossRef CAS PubMed;
(h) B. Brizet, N. Desbois, A. Bonnot, A. Langlois, A. Dubois, J.-M. Barbe, C. P. Gros, C. Goze, F. Denat and P. D. Harvey, Inorg. Chem., 2014, 53, 3392–3403 CrossRef CAS PubMed;
(i) S. Kuck, G. Hoffmann, M. Broering, M. Fechtel, M. Funk and R. Wiesendanger, J. Am. Chem. Soc., 2008, 130, 14072–14073 CrossRef CAS PubMed;
(j) K. E. Thomas, H. Vazquez-Lima, Y. Fang, Y. Song, K. J. Gajnon, C. M. Beavers, K. M. Kadish and A. Ghosh, Chem.–Eur. J., 2015, 21, 16839–16847 CrossRef CAS PubMed;
(k) M. Bröring, F. Bregier, E. Cónsul Tejero, C. Hell and M. C. Holthausen, Angew. Chem., Int. Ed., 2007, 46, 445–448 CrossRef PubMed;
(l) A. Ghosh, Chem. Rev., 2017, 117, 3798–3881 CrossRef CAS PubMed;
(m) R. Orłowski, D. Gryko and D. T. Gryko, Chem. Rev., 2017, 117, 3102–3137 CrossRef PubMed;
(n) Y. Fang, Z. Ou and K. M. Kadish, Chem. Rev., 2017, 117, 3377–3419 CrossRef CAS PubMed;
(o) K. Fujino, Y. Hirata, Y. Kawabe, T. Morimoto, A. Srinivasan, M. Toganoh, Y. Miseki, A. Kudo and H. Furuta, Angew. Chem., Int. Ed., 2011, 50, 6855–6859 CrossRef CAS PubMed;
(p) S. Hiroto, K. Furukawa, H. Shinokubo and A. Osuka, J. Am. Chem. Soc., 2006, 128, 12380–12381 CrossRef CAS PubMed;
(q) A. Mahammed, I. Giladi, I. Goldberg and Z. Gross, Chem.–Eur. J., 2001, 7, 4259–4265 CrossRef CAS PubMed;
(r) S. Hirabayashi, M. Omote, N. Aratani and A. Osuka, Bull. Chem. Soc. Jpn., 2012, 85, 558–562 CrossRef CAS;
(s) B. koszarna and D. T. Gryko, Chem. Commun., 2007, 2994–2996 RSC;
(t) B. Koszarna and D. T. Gryko, J. Org. Chem., 2006, 71, 3707–3717 CrossRef CAS PubMed;
(u) H. L. Buckley, W. A. Chomitz, B. Koszarna, M. Tasior, D. T. Gryko, P. J. Brothers and J. Arnold, Chem. Commun., 2012, 48, 10766–10768 RSC;
(v) C. F. Zipp, J. P. Michael, M. A. Fernandes and H. M. Marques, S. Afr. J. Chem., 2013, 66, 158–166 CAS;
(w) W. Sinha, M. G. Sommer, N. Deibel, F. Ehret, M. Bauer, B. Sarkar and S. Kar, Angew. Chem., Int. Ed., 2015, 54, 13769–13774 CrossRef CAS PubMed;
(x) W. Sinha, M. G. Sommer, N. Deibel, F. Ehret, B. Sarkar and S. Kar, Chem.–Eur. J., 2014, 20, 15920–15932 CrossRef CAS PubMed;
(y) W. Sinha, M. G. Sommer, L. Hettmanczyk, B. Patra, V. Filippou, B. Sarkar and S. Kar, Chem.–Eur. J., 2017, 23, 2396–2404 CrossRef CAS PubMed;
(z) B. Patra, S. Sobottka, W. Sinha, B. Sarkar and S. Kar, Chem.–Eur. J., 2017, 23, 13858–13863 CrossRef CAS PubMed;
(a
a) A. Garai, S. Sobottka, R. Schepper, W. Sinha, M. Bauer, B. Sarkar and S. Kar, Chem.–Eur. J., 2018, 24, 12613–12622 CrossRef CAS PubMed;
(a
b) X.-S. Ke, Y. Hong, V. M. Lynch, D. Kim and J. L. Sessler, J. Am. Chem. Soc., 2018, 140, 7579–7586 CrossRef CAS PubMed.
- J. Ohyama, Y. Hitomi, Y. Higuchi, M. Shinagawa, H. Mukai, M. Kodera, K. Teramura, T. Shishido and T. Tanaka, Chem. Commun., 2008, 6300–6302 RSC.
- M. Kanehara, H. Takahashi and T. Teranishi, Angew. Chem., Int. Ed., 2008, 47, 307–310 CrossRef CAS PubMed.
- B. C. Mei, K. Susumu, I. L. Medintz and H. Mattoussi, Nat. Protoc., 2009, 4, 412 CrossRef CAS PubMed.
- J. W. Ciszek, M. P. Stewart and J. M. Tour, J. Am. Chem. Soc., 2004, 126, 13172–13173 CrossRef CAS PubMed.
-
(a) M. Stefanelli, F. Mandoj, S. Nardis, M. Raggio, F. Fronczek, G. T. McCandless, K. Smith and R. Paolesse, Org. Biomol. Chem., 2015, 13, 6611–6618 RSC;
(b) S. Hiroto, N. Aratani, N. Shibata, Y. Higuchi, T. Sasamori, N. Tokitoh, H. Shinokubo and A. Osuka, Angew. Chem., 2009, 121, 2424–2426 CrossRef.
-
(a) A. J. Shaikh, F. Rabbani, T. A. Sherazi, Z. Iqbal, S. Mir and S. A. Shahzad, J. Phys. Chem. A, 2015, 119, 1108–1116 CrossRef CAS PubMed;
(b) A. Ishida and Y. Sakata, Chem. Commun., 1998, 57–58 RSC.
-
(a) H. Aldahhak, M. Paszkiewicz, F. Allegretti, D. A. Duncan, S. Tebi, P. S. Deimel, P. Casado Aguilar, Y.-Q. Zhang, A. C. Papageorgiou, R. Koch, J. V. Barth, W. G. Schmidt, S. Müllegger, W. Schöfberger, F. Klappenberger, E. Rauls and U. Gerstmann, J. Phys. Chem. C, 2017, 121, 2192–2200 CrossRef CAS;
(b) E. Steene, A. Dey and A. Ghosh, J. Am. Chem. Soc., 2003, 125, 16300–16309 CrossRef CAS PubMed.
- M. S. Boeckl, A. L. Bramblett, K. D. Hauch, T. Sasaki, B. D. Ratner and J. W. Rogers Jr, Langmuir, 2000, 16, 5644–5653 CrossRef CAS.
Footnotes |
† This contribution is dedicated to the occasion of the 60th birthday of Professor Goutam Kumar Lahiri. |
‡ Electronic supplementary information (ESI) available: Experimental section, NMR, mass spectrometry, FT-IR, UV-Vis, emission and X-ray data. See DOI: 10.1039/c9na00671k |
|
This journal is © The Royal Society of Chemistry 2020 |