DOI:
10.1039/C9GC02657F
(Paper)
Green Chem., 2020,
22, 118-122
The clean preparation of multisubstituted pyrroles under metal- and solvent-free conditions†
Received
29th July 2019
, Accepted 21st October 2019
First published on 29th November 2019
Abstract
A practical and sustainable protocol for the clean preparation of various multisubstituted pyrroles through an iodine-catalyzed multicomponent reaction under metal- and solvent-free conditions was developed. In the gram-scale synthesis, the desired pyrroles can be easily isolated in high-purity through simple extraction.
Introduction
With the increasing awareness of sustainable development, chemistry has been driven to minimize chemical waste in order to be in line with the 12 principles of Green Chemistry over the past few decades.1 The main petroleum hydrocarbons consumed in a traditional chemical process are the organic solvents employed as the reaction media, which account for 80–90% of mass utilization in a pharmaceutical/fine chemical process.2 In this context, solvent-free reactions represent the ideal strategy because they are in conformity with at least two of the principles of Green Chemistry: “Principle 1, Prevent Waste” and “Principle 5, Safer Solvents and Auxiliaries”. But, the high concentration of chemicals under neat reaction conditions not only reduces the reaction efficiency but also causes undesired side reactions.
Heterocyclic compounds account for about 60% of all drugs in the pharmaceutical and pesticide industries, among which nitrogen-containing heterocyclic compounds are the most important.3 Multisubstituted pyrroles, in particular, are widely represented in numerous natural products, synthetic pharmaceuticals and organic functional-materials.4 Given their inherently important value, the development of novel methods to construct such molecules is a fundamental objective in synthesis chemistry. There are a lot of efficient protocols that solve this challenge, including classical methods (Hantzsch reaction,5 Knorr reaction,6 and Paal–Knorr condensation reaction7), cycloaddition reaction,8 multicomponent reaction9 and several others.10 Among the established synthesis methods, the multicomponent reaction is the best choice for attaining atom- and step economy, and is thus a popular approach for developing ideal synthesis procedures for multisubstituted pyrroles. Despite these significant progresses, it is also true that there is a lack of transition-metal-free multicomponent reactions under solvent-free conditions, which would very likely be more environmentally benign.
We were aware that only one example of the synthesis of multisubstituted N-methylpyrroles through a copper salt-catalyzed multicomponent reaction under neat conditions was reported by Shao-Hua Wang in 2016.9a However, the environmental friendliness and practicability of this reaction are seriously compromised by the requirement of a large excess of reductants (2 equiv. of TMSCN and 15 equiv. of N,N-dimethylformamide) and oxidant (2 equiv. of toxic 2,3-dichloro-5,6-dicyano-1,4-benzoquinone), plus the inevitable generation of plenty of undesired side-products and the tedious chromatographic purification. Moreover, a large amount of mixed solvents (n-heptane/Et2NCOMe) was necessary for the synthesis of other N-substituted pyrroles. On the other hand, molecular iodine has already been developed as an eco-friendly, cheap and readily available catalyst for numerous catalytic reactions over the past few years.11 For example, very recently, Gu and Li reported the iodine-catalyzed cycloaddition reaction of enolizable aldehyde and aliphatic primary amines.8l Therefore, molecular iodine may be a suitable substitute for environment damaging transition metals.
As our research was guided by the 12 principles of Green Chemistry,12 herein we disclose a sustainable and practical method for the clean preparation of multisubstituted pyrroles through a molecular iodine-catalyzed (Principle 9) multicomponent reaction (Principle 8) of equimolar amounts of alkynes, TMSCN, and N,N-disubstituted formamides (Principle 1 and 2) under transition-metal- and solvent-free (Principle 1, 3 and 5) conditions. In the large-scale synthesis, the targeted products are easily isolated in good to excellent yields with high purity through simple extraction (Principle 1) (Scheme 1b). The present protocol eliminates the employment of large excess amounts of reactants, harmful transition-metal salts and toxic organic oxidants, and offers conditions leading to a high molar efficiency and atom economy, a very low EcoScale and PMI, which makes it ideal for the eco-friendly pharmacy industry.
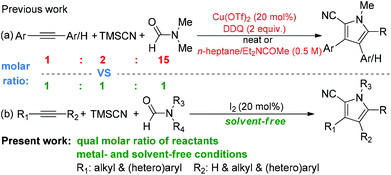 |
| Scheme 1 Synthesis of multisubstituted pyrroles. | |
Results and discussion
We initially investigated the reaction of phenylacetylene (1a) with equal equiv. of TMSCN (2) and DMF (3a), which was performed at 80 °C with 20 mol% of KI as the catalyst under solvent-free conditions. To our delight, the expected product 1-methyl-3-phenyl-1H-pyrrole-2-carbonitrile (4aa) was formed in 44% NMR yield after 2 h (Table 1, entry 1). Next, a series of iodine reagents were screened (entries 2–5) and the experimental results revealed that molecular iodine (entry 4) was the best choice for the present catalytic reaction. Varying the loading of molecular iodine and the reaction temperature did not improve the reaction efficiency (entries 6–9). No effect in the reaction efficiency was observed when the reaction was performed under a nitrogen atmosphere (entry 10). No reaction was observed in the absence of molecular iodine (entry 11), and the reaction substrates were quantitatively recovered.
Table 1 Optimization of reaction conditionsa
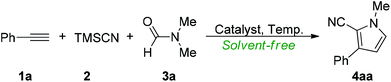
|
Entry |
Catalyst (mol) |
Temp. |
Yieldb |
Conditions: 1a (0.1 mmol), TMSCN (0.1 mmol), DMF (0.1 mmol), catalyst.
Estimated by 1H NMR using diethyl phthalate as an internal reference.
Under a nitrogen atmosphere. N.R.: no reaction.
|
1 |
KI (20%) |
80 °C |
44% |
2 |
NH4I (20%) |
80 °C |
58% |
3 |
Bu4NI (20%) |
80 °C |
27% |
4
|
I
2
(20%)
|
80 °C
|
100%
|
5 |
PhI(OAc)2 (20%) |
80 °C |
Trace |
6 |
I
2 (25%) |
80 °C |
100% |
7 |
I
2 (15%) |
80 °C |
90% |
8 |
I
2 (20%) |
100 °C |
100% |
9 |
I
2 (20%) |
60 °C |
91% |
10c |
I
2 (20%) |
80 °C |
100% |
11 |
— |
80 °C |
N.R. |
Having the optimal reaction conditions in hand (Table 1, entry 4), the substrate scope of the reaction was investigated with respect to both alkynes and N,N-disubstituted formamides (Table 2). We were pleased to find that the present reaction system was suitable for a variety of alkynes (4aa–4za). Both electron-rich and electron-poor functional-groups on the phenyl ring of phenylacetylene 1 were well-tolerated under the optimal reaction conditions leading to the generation of the expected products 4 in good to excellent yields (4aa–4ra). Notably, neither the nature nor the position of the substituents had notable influence on the reaction efficiency. Polycyclic- and hetero-aromatic terminal alkynes underwent the reactions efficiently to give the targeted products (4sa–4ua). However, performing the reaction of 2-ethynylfuran resulted in the formation of a complex reaction mixture. The reaction of aliphatic terminal alkynes such as 1-octyne (1v), 3-phenylpropyne (1w), and 4-phenylbut-3-ene-1-yne (1x) delivered the expected products (4va–4xa) in good yields. Moreover, thermodynamically more stable internal alkynes were also effective to provide the desired products (4ya and 4za). Subsequently, the substrate scope of the reaction with respect to the N,N-disubstituted formamides was investigated. A range of N,N-diethylformamide, N,N-dibutylformamide, N-methyl-N-phenylformamide, and piperidine-1-carbaldehyde substrates (3b–3e) were determined to be suitable reaction partners for this transformation and provided the desired products (4ab–4ae) in 71–81% yields. Importantly, in the gram-scale preparation of pyrroles, the products (4aa–4ha) were simply isolated in analytical purity through extraction without the tedious and costly chromatographic purifications.
Conditions: 1 (0.3 mmol), TMSCN (0.3 mmol), 3 (0.3 mmol), I2 (0.06 mmol), 80 °C, 2 h.
Conditions: 1 (7 mmol), TMSCN (7 mmol), 3 (7 mmol), I2 (1.4 mmol), 80 °C, 2 h.
|
|
In order to show the practicability of the developed process, both the large-scale preparation of multisubstituted pyrroles and one-pot transformations were performed. Phenylacetylenes 1a and 1f each reacted with TMSCN and DMF at a 30 mmol scale, and the desired products were easily isolated in excellent yields through simple extraction (Scheme 2a, see the ESI Fig. S1 and S2†). Decreasing the loading of I2 to 15 mol% led to a slightly lower yield of 4aa (72%). This clean multicomponent reaction (equal molar ratio of reactants and solvent-free conditions as well as high efficiency) offered a great opportunity for the exploration of one-pot sequential transformation from readily available starting materials. Without a tedious workup process, the crude reaction-mixture efficiently underwent hydration13 (1a → 5aa) or reduction14 (1a → 5ab) reaction in good yields (Scheme 2b and c).
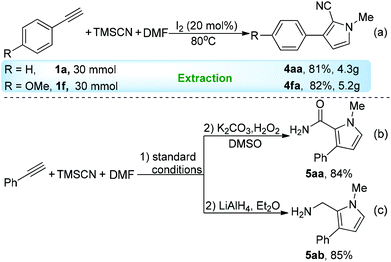 |
| Scheme 2 Large-scale synthesis of 3 and one-pot transformation. | |
To evaluate and benchmark the eco-friendly character of the present protocol, EcoScale,15 molar efficiency (Mol. E%),16 atom economy (AE) and process mass intensity (PMI)17 were calculated for 1-methyl-3-phenyl-1H-pyrrole-2-carbonitrile 4aa. As illustrated in Scheme 3, the present synthesis method presented a 2.1-fold increase in EcoScale, a 7.5-fold increase in Mol. E%, a 6-fold increase in AE, and an 85.7-fold decrease in PMI.
 |
| Scheme 3 Comparison of green metrics (calculated on the reaction of 7 mmol 1a). | |
To unveil the reaction mechanism, some control experiments were conducted (Scheme 4). The addition of 2 equiv. of radical scavengers (TEMPO or BHT) into the reaction mixture delivered the desired product 4aa in excellent yield (Scheme 4a), revealing the impossibility of a radical process (Scheme 4a). Considering that this type of reaction may involve the 2-(dimethylamino)malononitrile (6a) intermediate, we synthesized 6a.18 However, the reaction of 1a with 6a under the standard reaction conditions gave a trace amount of 4aa. Combined with the fact that the same amount of reactants were utilized, the possibility of 6a as an active intermediate in the present reaction could be ruled out (Scheme 4b). This result also indicated a different mechanism as compared to that reported in Wang's work.9a Performing the reaction with 20% mol NH4I as the catalyst under a nitrogen and air atmosphere resulted in the generation of 4aa in 0% (Scheme 4c) and 58% yields (Table 1, entry 2), respectively. These results suggested that the iodide was oxidized by molecular oxygen (in air) to generate molecular iodine.
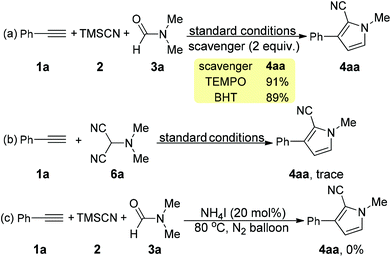 |
| Scheme 4 Control experiments. | |
A plausible reaction mechanism for the formation of multisubstituted pyrroles based on the control experiments and the related literature8d,9a is shown in Scheme 5. The multicomponent reaction was initiated by the direct formation of azomethine ylide IM1 through the molecular iodine catalyzed cyanation of DMF. The intermediate MI1
19 subsequently underwent cycloaddition to activated alkyne 1 to generate the desired product 4.
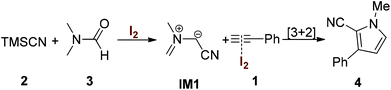 |
| Scheme 5 Possible mechanism. | |
Conclusions
In summary, we have developed a sustainable and practical approach for the clean preparation of various multisubstituted pyrroles (30 examples, 63–98%, >5 gram) through an iodine-catalyzed multicomponent reaction under metal- and solvent-free conditions. In the large-scale synthesis, only simple extraction is needed, and high purity target products can be obtained without time-consuming and laborious chromatographic purification. The present protocol is ideally suited for one-pot sequential synthesis, owing to its clean reaction conditions and high atom economy. Moreover, this methodology is much greener than the previously reported protocol with a 2.1-fold increase in EcoScale, a 7.5-fold increase in Mol. E%, a 6-fold increase in AE and an 85.7-fold decrease in PMI. Given the readily available materials, the clean reaction conditions, operational simplicity and high scalability, this protocol is expected to be ideal for industrial processing.
Conflicts of interest
There are no conflicts to declare.
Acknowledgements
We are grateful for financial support from the Hunan Provincial Natural Science Foundation of China (No. 2018JJ3215 and 2019JJ20008) and the Research Foundation of Education Bureau of Hunan Province (18C0147).
Notes and references
-
(a)
P. T. Anastas and J. C. Warner, Green Chemistry: Theory and Practice, Oxford University Press, 1998 Search PubMed;
(b) C.-J. Li and B. M. Trost, Proc. Natl. Acad. Sci. U. S. A., 2008, 105, 13197 CrossRef CAS PubMed.
-
(a) D. J. C. Constable, C. Jimenez-Gonzalez and R. K. Henderson, Org. Process Res. Dev., 2007, 11, 133 CrossRef CAS;
(b) H. C. Erythropel, J. B. Zimmerman, T. M. de Winter, L. Petitjean, F. Melnikov, C. H. Lam, A. W. Lounsbury, K. E. Mellor, N. Z. Janković, Q. Tu, L. N. Pincus, M. M. Falinski, W. Shi, P. Coish, D. L. Plata and P. T. Anastas, Green Chem., 2018, 20, 1929 RSC.
-
(a) X.-P. Ma, K. Li, S.-Y. Wu, C. Liang, G.-F. Su and D.-L. Mo, Green Chem., 2017, 19, 5761 RSC;
(b) X. Zhang, G.-P. Lu, Z.-B. Xu and C. Cai, ACS Sustainable Chem. Eng., 2017, 5, 9279 CrossRef CAS;
(c) L. Wang, D. Xiong, L. Jie, C. Yu and X. Cui, Chin. Chem. Lett., 2018, 29, 907 CrossRef CAS;
(d) C.-H. Chen, Q.-Y. Wu, C. Wei, C. Liang, G.-F. Su and D.-L. Mo, Green Chem., 2018, 20, 2722 RSC;
(e) Y. Gu, W. Huang, S. Chen and X. Wang, Org. Lett., 2018, 20, 4285 CrossRef CAS;
(f) J. Chen, D. Chang, F. Xiao and G.-J. Deng, Green Chem., 2018, 20, 5459 RSC;
(g) W. Wei, L. Wang, H. Yue, P. Bao, W. Liu, C. Hu, D. Yang and H. Wang, ACS Sustainable Chem. Eng., 2018, 6, 17252 CrossRef CAS;
(h) W. Xie, Y. Wu, J. Zhang, Q. Mei, Y. Zhang, N. Zhu, R. Liu and H. Zhang, Eur. J. Med. Chem., 2018, 145, 35 CrossRef CAS PubMed;
(i) K. Chen, W.-J. Hao, S.-J. Tu and B. Jiang, Green Chem., 2019, 21, 675 RSC;
(j) L. Wang, Y. Zhang, M. Zhang, P. Bao, X. Lv, H.-G. Liu, X. Zhao, J.-S. Li, Z. Luo and W. Wei, Tetrahedron Lett., 2019, 60, 1845 CrossRef CAS;
(k) J. Jiang, H. Huang and G.-J. Deng, Green Chem., 2019, 21, 986 RSC;
(l) L. Wang, M. Zhang, Y. Zhang, Q. Liu, X. Zhao, J.-S. Li, Z. Luo and W. Wei, Chin. Chem. Lett., 2019 DOI:10.1016/j.cclet.2019.05.041;
(m) Y. Liu, L. Chen, Z. Wang, P. Liu, Y. Liu and B. Dai, J. Org. Chem., 2019, 84, 204 CrossRef CAS;
(n) X. Zhang, S. Dong, Q. Ding, X. Fan and G. Zhang, Chin. Chem. Lett., 2019, 30, 375 CrossRef CAS;
(o) H. Huang, Z. Qu, X. Ji and G.-J. Deng, Org. Chem. Front., 2019, 6, 1146 RSC;
(p) S.-Y. Wu, W.-L. Chen, X.-P. Ma, C. Liang, G.-F. Su and D.-L. Mo, Adv. Synth. Catal., 2019, 361, 965 CrossRef CAS;
(q) X. Gong, G. Li, Z. Gan, Q. Yan, X. Dou and D. Yang, Asian J. Org. Chem., 2019, 8, 1472 CrossRef CAS;
(r) M. Li, X. Dong, N. Zhang, F. Jérôme and Y. Gu, Green Chem., 2019, 21, 4650 RSC;
(s) Z. Xu, H. Huang, H. Chen and G.-J. Deng, Org. Chem. Front., 2019, 6, 3060 RSC.
-
(a) H. Fan, J. Peng, M. T. Hamann and J.-F. Hu, Chem. Rev., 2008, 108, 264 CrossRef CAS PubMed;
(b) I. S. Young, P. D. Thornton and A. Thompson, Nat. Prod. Rep., 2010, 27, 1801 RSC;
(c) R. Khajuria, S. Dham and K. K. Kapoor, RSC Adv., 2016, 6, 37039 RSC.
- A. W. Trautwein, R. D. Süßmuth and G. Jung, Bioorg. Med. Chem. Lett., 1998, 8, 2381 CrossRef CAS PubMed.
- C. Paal, Ber. Dtsch. Chem. Ges., 1885, 18, 367 CrossRef.
- A. Balakrishna, A. Aguiar, P. J. M. Sobral, M. Y. Wani, J. Almeida e Silva and A. J. F. N. Sobral, Catal. Rev., 2019, 61, 84 CrossRef CAS.
-
(a) X. Li, M. Chen, X. Xie, N. Sun, S. Li and Y. Liu, Org. Lett., 2015, 17, 2984 CrossRef CAS PubMed;
(b) X. Wu, K. Li, S. Wang, C. Liu and A. Lei, Org. Lett., 2016, 18, 56 CrossRef CAS;
(c) T. Li, H. Yan, X. Li, C. Wang and B. Wan, J. Org. Chem., 2016, 81, 12031 CrossRef CAS PubMed;
(d) B.-Y. Cheng, Y.-N. Wang, T.-R. Li, L.-Q. Lu and W.-J. Xiao, J. Org. Chem., 2017, 82, 12134 CrossRef CAS PubMed;
(e) Z. Tian, J. Xu, B. Liu, Q. Tan and B. Xu, Org. Lett., 2018, 20, 2603 CrossRef CAS;
(f) H.-C. Chiu and I. A. Tonks, Angew. Chem., Int. Ed., 2018, 57, 6090 CrossRef CAS PubMed;
(g) S. M. Gillbard, C.-H. Chung, S. N. Karad, H. Panchal, W. Lewis and H. W. Lam, Chem. Commun., 2018, 54, 11769 RSC;
(h) N. N. Kumar Reddy, D. Rawat and S. Adimurthy, J. Org. Chem., 2018, 83, 9412 CrossRef CAS;
(i) J. Cen, Y. Wu, J. Li, L. Huang, W. Wu, Z. Zhu, S. Yang and H. Jiang, Org. Lett., 2019, 21, 2090 CrossRef CAS PubMed;
(j) K. Kawakita, E. P. Beaumier, Y. Kakiuchi, H. Tsurugi, I. A. Tonks and K. Mashima, J. Am. Chem. Soc., 2019, 141, 4194 CrossRef CAS PubMed;
(k) D. Chen, Y. Shan, J. Li, J. You, X. Sun and G. Qiu, Org. Lett., 2019, 21, 4044 CrossRef CAS;
(l) W. Huang, S. Chen, Z. Chen, M. Yue, M. Li and Y. Gu, J. Org. Chem., 2019, 84, 5655 CrossRef CAS PubMed.
-
(a) X.-Q. Mou, Z.-L. Xu, L. Xu, S.-H. Wang, B.-H. Zhang, D. Zhang, J. Wang, W.-T. Liu and W. Bao, Org. Lett., 2016, 18, 4032 CrossRef CAS;
(b) J. Peng, Y. Gao, C. Zhu, B. Liu, Y. Gao, M. Hu, W. Wu and H. Jiang, J. Org. Chem., 2017, 82, 3581 CrossRef CAS;
(c) M. Fleige and F. Glorius, Chem. – Eur. J., 2017, 23, 10773 CrossRef CAS;
(d) Y. Liu, X. Yi, X. Luo and C. Xi, J. Org. Chem., 2017, 82, 11391 CrossRef CAS;
(e) L. Li, Q. Chen, X. Xiong, C. Zhang, J. Qian, J. Shi, Q. An and M. Zhang, Chin. Chem. Lett., 2018, 29, 1893 CrossRef CAS;
(f) Y. E. Jad, S. K. Gudimella, T. Govender, B. G. de la Torre and F. Albericio, ACS Comb. Sci., 2018, 20, 187 CrossRef CAS PubMed;
(g) M. Sun, J. Jiang, J. Chen, Q. Yang and X. Yu, Tetrahedron, 2019, 75, 130456 CrossRef;
(h) Z.-W. Chen, L. Zheng and J. Liu, Eur. J. Org. Chem., 2019, 3051 CrossRef CAS;
(i) H. Cui, X. Liu, W. Wei, D. Yang, C. He, T. Zhang and H. Wang, J. Org. Chem., 2016, 81, 2252 CrossRef CAS PubMed;
(j) W. Wei, P. Bao, H. Yue, S. Liu, L. Wang, Y. Li and D. Yang, Org. Lett., 2018, 20, 5291 CrossRef CAS PubMed.
-
(a) G. Cheng, W. Lv and L. Xue, Green Chem., 2018, 20, 4414 RSC;
(b) B. Ge, W. Lv, J. Yu, S. Xiao and G. Cheng, Org. Chem. Front., 2018, 5, 3103 RSC.
-
(a) Y. Sun, A. Abdukader, D. Lu, H. Zhang and C. Liu, Green Chem., 2017, 19, 1255 RSC;
(b) Y. Xu, B. Li, X. Zhang and X. Fan, J. Org. Chem., 2017, 82, 9637 CrossRef CAS;
(c) W. Wei, H. Cui, D. Yang, X. Liu, C. He, S. Dai and H. Wang, Org. Chem. Front., 2017, 4, 26 RSC;
(d) W.-K. Fu, K. Sun, C. Qu, X.-L. Chen, L.-B. Qu, W.-Z. Bi and Y.-F. Zhao, Asian J. Org. Chem., 2017, 6, 492 CrossRef CAS;
(e) W. Wu, Y. An, J. Li, S. Yang, Z. Zhu and H. Jiang, Org. Chem. Front., 2017, 4, 1751 RSC;
(f) P. Sun, D. Yang, W. Wei, X. Sun, W. Zhang, H. Zhang, Y. Wang and H. Wang, Tetrahedron, 2017, 73, 2022 CrossRef CAS;
(g) Z. Zhang, C. Pi, H. Tong, X. Cui and Y. Wu, Org. Lett., 2017, 19, 440 CrossRef CAS PubMed;
(h) S. Cao, Y. Liu, C. Hu, C. Wen and J.-P. Wan, ChemCatChem, 2018, 10, 5007 CrossRef;
(i) L. Liu, Q. Sun, Z. Yan, X. Liang, Z. Zha, Y. Yang and Z. Wang, Green
Chem., 2018, 20, 3927 RSC;
(j) H. Li, P. Zhou, F. Xie, J.-Q. Hu, S.-Z. Yang, Y.-J. Wang, W.-J. Hao, S.-J. Tu and B. Jiang, J. Org. Chem., 2018, 83, 13335 CrossRef CAS PubMed;
(k) P. Bao, L. Wang, H. Yue, Y. Shao, J. Wen, D. Yang, X. Zhao, H. Wang and W. Wei, J. Org. Chem., 2019, 84, 2976 CrossRef CAS PubMed;
(l) Y. Guo, G. Wang, L. Wei and J.-P. Wan, J. Org. Chem., 2019, 84, 2984 CrossRef CAS PubMed;
(m) Z. Zhang, X. Li, Y. Li, Y. Guo, X. Zhao, Y. Yan, K. Sun and G. Zhang, Tetrahedron, 2019, 75, 3628 CrossRef CAS;
(n) H. Y. Yu, C. Pi, Y. Wang, X. L. Cui and Y. J. Wu, Chin. J. Org. Chem., 2018, 38, 124 CrossRef CAS;
(o) C. Wang, X. Geng, P. Zhao, Y. Zhou, Y.-D. Wu, Y.-F. Cui and A.-X. Wu, Chem. Commun., 2019, 55, 8134 RSC;
(p) W.-H. Bao, M. He, J.-T. Wang, X. Peng, M. Sung, Z. Tang, S. Jiang, Z. Cao and W.-M. He, J. Org. Chem., 2019, 84, 6065 CrossRef CAS PubMed;
(q) M.-L. Zhi, B.-Z. Chen, W. Deng, X.-Q. Chu, T.-P. Loh and Z.-L. Shen, J. Org. Chem., 2019, 84, 3017 CrossRef CAS PubMed;
(r) R. Fu, M.-F. Li, P. Zhou, W.-J. Hao, S.-J. Tu and B. Jiang, Adv. Synth. Catal., 2019, 361, 2280 CrossRef CAS.
-
(a) L.-Y. Xie, Y. Duan, L.-H. Lu, Y.-J. Li, S. Peng, C. Wu, K.-J. Liu, Z. Wang and W.-M. He, ACS Sustainable Chem. Eng., 2017, 5, 10407 CrossRef CAS;
(b) C. Wu, L.-H. Lu, A.-Z. Peng, G.-K. Jia, C. Peng, Z. Cao, Z. Tang, W.-M. He and X. Xu, Green Chem., 2018, 20, 3683 RSC;
(c) L.-Y. Xie, S. Peng, F. Liu, G.-R. Chen, W. Xia, X. Yu, W.-F. Li, Z. Cao and W.-M. He, Org. Chem. Front., 2018, 5, 2604 RSC;
(d) W.-H. Bao, Z. Wang, X. Tang, Y.-F. Zhang, J.-X. Tan, Q. Zhu, Z. Cao, Y.-W. Lin and W.-M. He, Chin. Chem. Lett., 2019 DOI:10.1016/j.cclet.2019.06.052;
(e) H. Jiang, X. Tang, Z. Xu, H. Wang, K. Han, X. Yang, Y. Zhou, Y.-L. Feng, X.-Y. Yu and Q. Gui, Org. Biomol. Chem., 2019, 17, 2715 RSC;
(f) Z. Cao, Q. Zhu, Y.-W. Lin and W.-M. He, Chin. Chem. Lett., 2019 DOI:10.1016/j.cclet.2019.09.041;
(g) S. Peng, Y.-X. Song, J.-Y. He, S.-S. Tang, J.-X. Tan, Z. Cao, Y.-W. Lin and W.-M. He, Chin. Chem. Lett., 2019 DOI:10.1016/j.cclet.2019.08.002;
(h) K.-J. Liu, Z.-H. Duan, X.-L. Zeng, M. Sun, Z. Tang, S. Jiang, Z. Cao and W.-M. He, ACS Sustainable Chem. Eng., 2019, 7, 10293 CrossRef CAS;
(i) L.-Y. Xie, L.-L. Jiang, J.-X. Tan, Y. Wang, X.-Q. Xu, B. Zhang, Z. Cao and W.-M. He, ACS Sustainable Chem. Eng., 2019, 7, 14153 CrossRef CAS.
- A. R. Katritzky, B. Pilarski and L. Urogdi, Synthesis, 1989, 949 CrossRef CAS.
- L. H. Amundsen and L. S. Nelson, J. Am. Chem. Soc., 1951, 73, 242 CrossRef CAS.
- K. Van Aken, L. Strekowski and L. Patiny, Beilstein J. Org. Chem., 2006, 2, 3 Search PubMed.
- F. I. McGonagle, H. F. Sneddon, C. Jamieson and A. J. B. Watson, ACS Sustainable Chem. Eng., 2014, 2, 523 CrossRef CAS.
-
(a) C. Jimenez-Gonzalez, C. S. Ponder, Q. B. Broxterman and J. B. Manley, Org. Process Res. Dev., 2011, 15, 912 CrossRef CAS;
(b) T. W. Bousfield, K. P. R. Pearce, S. B. Nyamini, A. Angelis-Dimakis and J. E. Camp, Green Chem., 2019, 21, 3675 RSC.
- X.-Q. Mou, L. Xu, S.-H. Wang and C. Yang, Tetrahedron Lett., 2015, 56, 2820 CrossRef CAS.
- We have tried to capture the intermediate IM1 by GC-MS and real-time NMR, but all efforts failed. Further studies on the detailed reaction mechanism are currently in progress.
Footnote |
† Electronic supplementary information (ESI) available. See DOI: 10.1039/c9gc02657f |
|
This journal is © The Royal Society of Chemistry 2020 |