DOI:
10.1039/C9FO02815C
(Paper)
Food Funct., 2020,
11, 824-833
Citrus flavonoids suppress IL-5 and ROS through distinct pathways in PMA/ionomycin-induced EL-4 cells
Received
28th November 2019
, Accepted 26th December 2019
First published on 27th December 2019
Abstract
Interleukin-5 (IL-5) strongly initiates the asthmatic inflammatory response, which affects 300 million patients with asthma annually worldwide, through oxidative stress generation. Citrus flavonoids have beneficial properties, such as anti-inflammatory and antioxidant properties, but the precise molecular mechanism of the inhibition of the asthmatic inflammatory response is still unclear. This study aimed to investigate the underlying mechanisms of ROS and IL-5 reduction with citrus flavonoid treatment in PMA/ionomycin-induced EL-4 cells. Our results showed that hesperetin and gardenin A dramatically suppressed ROS and IL-5 production through distinct pathways. Interestingly, hesperidin induced HO-1 expression through the transcription factor Nrf2 coupled with the PI3K/AKT or ERK/JNK signaling pathway, consequently downregulating NFAT activity and IL-5 secretion. Likewise, gardenin A induced HO-1 expression and subsequently suppressed IL-5 production by reducing NFAT activity and upregulating PPARγ in EL-4 cells, suggesting that inducing HO-1 expression may inhibit asthmatic inflammation. Altogether, hesperidin and gardenin A have great potential for regulating the asthma-associated immune responses through antioxidant properties.
1. Introduction
It is well known that air pollution from electricity, fuel, and transportation is a permanent physical risk and contributes to the global burden of disease (GBD), such as asthma, which has over 300 million patients annually in the world.1–4 In addition to ambient air pollution, indoor endotoxin exposure is also linked to allergic rhinitis and asthma.5,6 Although investigators and clinicians are striving to find personalized prevention strategies through epidemiological studies on air pollution and asthma, the high incidence and prevalence of asthma still cannot be mitigated.7–9
How air pollution affects asthma and allergic rhinitis is unclear. Despite intensive research, scientists still cannot completely understand why some individuals develop antigen-specific cell-mediated immune responses. Nevertheless, it is certain that individual genetic susceptibility may affect an especially proinflammatory subtype of antigen-specific T helper 2 (Th2) cells, which are dominant in initiating and orchestrating the asthmatic inflammatory response pathways through the generation of oxidative stress.10–12 An overactive Th2 immune response is stimulated by allergens and typically produces characteristic effector cytokines such as interleukin-5 (IL-5).11 Th2 cytokine expression is posttranscriptionally regulated by Th2-related transcription factors, including nuclear factor of activated T cells (NFAT), signal transducer and activator of transcription 6 (STAT6), and activator protein-1 (AP-1).13,14
IL-5, a Th2 cell-derived cytokine, is involved in eosinophil development and differentiation from myeloid precursor cells in bone marrow.15 Dysregulation of IL-5 secretion by Th2 cells has been linked to eosinophilic inflammation in asthma.16 In addition, reactive oxygen species (ROS), such as nitric oxide (NO) and eosinophil extracellular traps (EETs), a cytotoxic granule protein, have been found to be involved in severe eosinophilic asthma.17,18 Mepolizumab, an anti-IL 5 humanized monoclonal antibody (trade name Nucala), has been documented as having therapeutic benefits and is a standard care for severe eosinophilic asthma patients.19 However, anti-IL-5 therapy with mepolizumab induces a sustained decrease in blood eosinophils with an increase in lymphocyte IL-5 and IL-5 receptor α production, suggesting that the endogenous IL-5 autoregulatory mechanism or IL-5-independent pathway exacerbates eosinophilic asthma processes and should be considered before asthma treatment.20–22 Therefore, finding a safe, effective, and economical alternative treatment strategy is important for allergic rhinitis and asthma control.
Flavonoids are the largest group of phytonutrients in the human diet and are ubiquitously found in foods, including tea, cocoa, vegetables, red wine, and fruits.23 Several epidemiological studies have examined the relationship between flavonoids in dietary intake and asthma.24 A previous study demonstrated that flavonoids from apples and red wine consumption show a protective effect on asthma through a powerful antioxidant capacity.25 A recent review summarized that flavonoids may be potential therapeutic agents for asthma treatment by targeting oxidizing molecules that conjugate immune mediators and sequence-specific DNA-binding factors.26 Moreover, a previous report demonstrated that fisetin, a flavonol, inhibits IL-5 cytokine production in the human KU812 basophil cell line.27 Likewise, hesperidin effectively treats asthma by reducing IL-5 cytokine levels.28 Citrus flavonoids, including nobiletin, tangeretin, 5-demethylnobiletin (5-DN), gardenin A, and hesperidin, have been shown to have powerful antioxidant activity and anti-inflammatory properties in promoting health.29–32 However, the underlying mechanisms by which citrus flavonoids suppress Th2 cell-derived IL-5 and ROS remain unclear. In this study, we aimed to uncover the signaling related to the downregulation of IL-5 and ROS expression by citrus flavonoid treatment in EL-4 lymphocyte cells.
2. Materials and methods
2.1. Chemicals
Dulbecco's modified Eagle's medium (DMEM), horse serum (HS), and penicillin−streptomycin (PS) antibiotic solution were obtained from Invitrogen (Carlsbad, CA, USA). Citrus flavonoids (nobiletin, 5-demethylnobiletin, tangeretin, and gardenin A) were kindly provided by Professor Chi-Tang Ho (Rutgers University, USA). Hesperidin, phorbol 12-myristate 13-acetate (PMA), ionomycin, dimethyl fumarate, retinoic acid, GW9662, and rosiglitazone were obtained from Sigma-Aldrich Co. (St Louis, MO, USA). AS1517499 was purchased from Axon Medchem (Axon Medchem (Groningen, The Netherlands). SR 11302 was purchased from Tocris Bioscience (Ellisville, MO, USA). NFAT inhibitor was obtained from Cayman Chemical (Ann Arbor, MI, USA). Anti-Nrf2 was purchased from Novus Biologicals (Littleton, CO, USA). Anti-HO-1 and anti-lamin B1 antibodies were obtained from BioVision (Milpitas, CA, USA). Anti-NFAT, anti-STAT6, anti-β-actin, anti-c-Jun, anti-c-Fos, anti-PI3k, anti-phospho-PI3k, anti-Akt, anti-phospho-Akt, anti-ERK, anti-phospho-ERK, anti-p38, anti-phospho-p38, anti-JNK, and anti-phospho-JNK were purchased from Cell Signaling Technology (Beverly, MA, USA). Anti-PPARγ was obtained from Merck Millipore (Billerica, MA, USA). Anti-PCNA was purchased from GeneTex (Irvine, CA, USA). PD98059, wortmannin, and SP600125 were obtained from Biosource (Camarillo, CA, USA).
2.2. Cell culture
The EL-4 murine T-lymphoma cell line (BCRC 60179) was obtained from the Bioresource Collection and Research Center (BCRC, Food Industry Research and Development Institute, Hsinchu, Taiwan). Cells were grown in DMEM and supplemented with 10% HS. Confluent cells were subcultured at a ratio of 1
:
3, and the medium was changed twice a week. Cells were cultured at 37 °C under a humidified atmosphere of 5% CO2.
2.3. Cell viability analysis
Cell viability analysis was performed according to our previous report.33 Briefly, after treatment, cells were then suspended in trypan blue solution for viability measurement.
2.4. Enzyme-linked immunosorbent assay (ELISA)
Quantification of IL-5 secretion by PMA/ionomycin-induced EL-4 cells was performed after treatment with or without citrus flavonoids (10 μM) for 24 h. The level of IL-5 was evaluated according to the ELISA kit instructions (R&D system Inc., Minneapolis, MN, USA).
2.5. Intracellular ROS determination
The 2,7-dichlorodihydrofluorescein diacetate (DCFH-DA) method was used to measure intracellular ROS production according to the method described by Cheng et al. (2019).34
2.6. Nuclear and cytosolic protein extraction
After citrus flavonoid (10 μM) or inhibitor stimulation, the nuclear and cytosolic proteins were extracted using a nuclear/cytosol fractionation kit (BioVision, Mountain View, CA, USA) and according to the method described by Ho et al. (2012).35
2.7. Western blotting
Western blotting was performed according to the report by Chang et al. (2019).36 The primary antibodies used in this study were NFAT, c-Jun, c-Fos, STAT6, Nrf2, PPARγ, HO-1, phospho-PI3k, PI3k, phospho-Akt, Akt, phospho-ERK, ERK, phospho-JNK, JNK, phospho-p38, p38, lamin B1, β-actin, and PCNA.
2.8. Statistical analysis
All results are presented as mean ± SD. Significant differences were evaluated by ANOVA followed by Duncan's test between multiple groups. A p value <0.05 was considered to be significant.
3. Results
3.1. Regulating IL-5 and ROS production through the transcription factors NFAT, STAT6, and AP-1 in PMA/ionomycin-induced EL-4 cells
Several articles have shown that PMA plus ionomycin enhances Th2 cytokine production in EL-4 cells.37–39 In the present study, we found that the maximum IL-5 and ROS production by EL-4 cells occurred with 0.1 μg mL−1 PMA combined with 400 nM ionomycin treatment for 24 h (Fig. 1A and B). The cell viability was not affected by 0.1 μg mL−1 PMA plus 400 nM ionomycin treatment compared to untreated cells (Fig. 1C). To validate the potent transcription factors involved in IL-5 and ROS production, nuclear protein expression of the Th2-associated NFAT, STAT6, and AP-1 family members c-Fos and c-Jun transcription factors were evaluated in PMA/ionomycin-induced EL-4 cells. As shown in Fig. 1D and E (quantification data), all the nuclear protein expression levels of these transcription factors were upregulated by PMA/ionomycin treatment in EL-4 cells. To confirm this result, PMA/ionomycin-induced EL-4 cells were pretreated with an NFAT inhibitor, STAT6 inhibitor (AS1517499), or AP1 inhibitor (SR11302) for 1 h. After stimulation, IL-5 secretion was significantly reduced by inhibitor treatment, particularly in NFAT inhibitor-treated cells (Fig. 1F). Likewise, ROS production was significantly inhibited by inhibitor treatment (Fig. 1G), indicating that not only IL-5 but also ROS production was regulated by NFAT, STAT6, and AP-1 transcription factors in PMA/ionomycin-treated EL-4 cells. In addition, cell viability was not affected in inhibitor-treated cells (Fig. 1H).
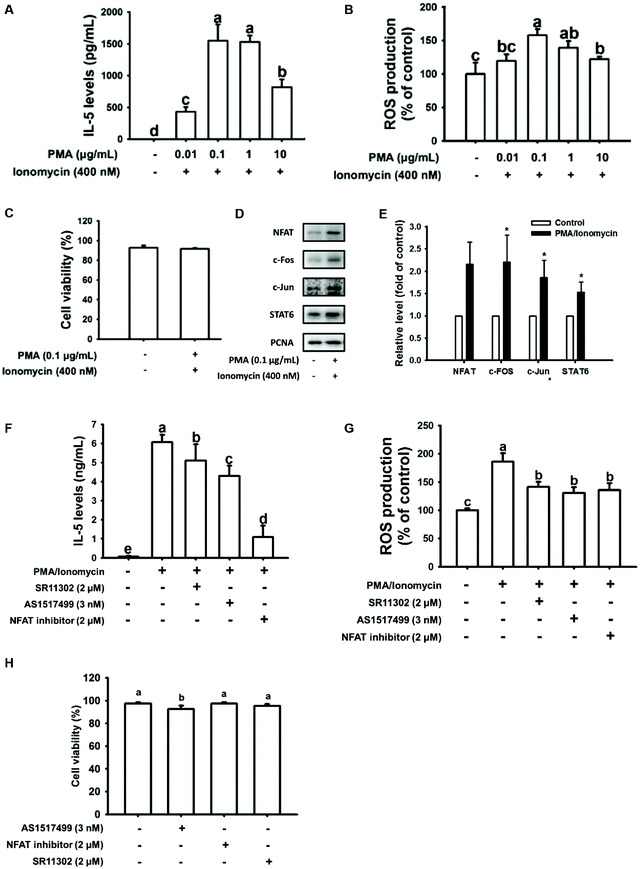 |
| Fig. 1 Regulating IL-5 and ROS production in PMA/ionomycin-induced EL-4 cells. (A) IL-5 and (B) ROS were induced by 0–10 μg mL−1 PMA combined with 400 nM ionomycin treatment for 24 h. (C) Cell viability was determined after treatment with 0.1 μg mL−1 PMA combined with 400 nM ionomycin for 24 h. (D) Th2-associated NFAT, c-FOS, c-Jun, and STAT6 transcription factor protein expression. (E) Quantification data of transcription factors were assessed. The relative level of protein is expressed as the fold change over the control to normalize against PCNA (loading control). The effects of transcription factor inhibitors on (F) IL-5, (G) ROS, and (H) cell viability were measured in PMA/ionomycin-induced EL-4 cells. The results are expressed as mean ± SD (n = 3). * indicates p < 0.05 compared with the control cells. Different letters indicate p < 0.05 compared with every other group. | |
3.2. Citrus flavonoids suppressed IL-5 and ROS production in PMA/ionomycin-treated EL-4 cells
It is well known that citrus flavonoids have excellent antioxidant activity and anti-inflammatory properties that promote health.29–31 Here, citrus flavonoids, including nobiletin, tangeretin, 5-DN, gardenin A, and hesperidin, were used to explore their effects on IL-5 and ROS production in EL-4 cells treated with PMA/ionomycin. As shown in Fig. 2A, gardenin A or hesperidin displayed an intensely suppressive effect on IL-5 secretion by PMA/ionomycin-treated EL-4 cells, respectively. Additionally, ROS production was inhibited by gardenin A or hesperidin treatment in PMA/ionomycin-incubated EL-4 cells (Fig. 2B). Surprisingly, 5-DN treatment dramatically increased IL-5 production and significantly decreased ROS expression in PMA/ionomycin-treated EL-4 cells (Fig. 2A and B). In addition, no cytotoxicity was found in 10 μM gardenin A- or hesperidin-treated cells compared with untreated cells (Fig. 2C). NFAT was the major transcription factor in regulating IL-5 secretion by PMA/ionomycin-induced EL-4 cells. NFAT protein expression was inhibited by gardenin A or hesperidin treatment in a dose-dependent manner (5 to 10 μM) in PMA/ionomycin-treated EL-4 cells (Fig. 2D and E). For the reasons given above, we chose those doses of citrus flavonoids to perform the study.
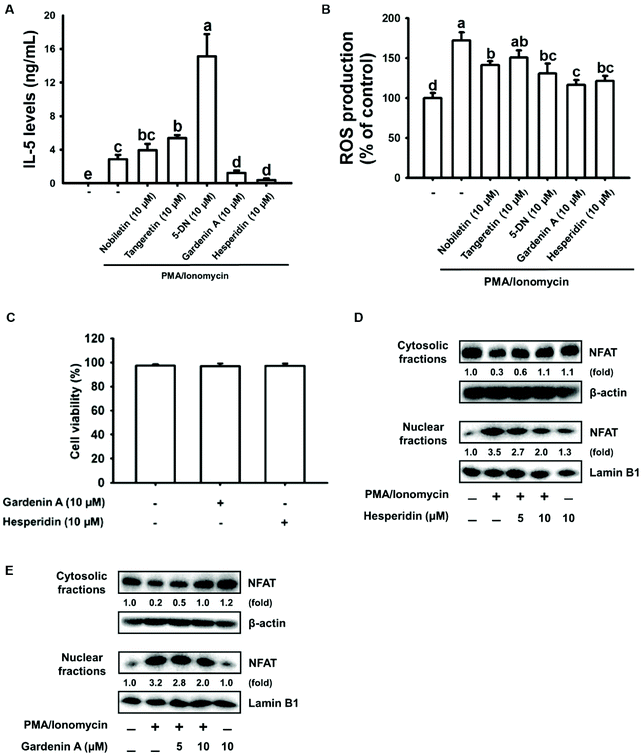 |
| Fig. 2 Citrus flavonoids inhibited IL-5 and ROS production in PMA/ionomycin-induced EL-4 cells. Cells were co-treated with citrus flavonoids and PMA/ionomycin for 24 h. The level of (A) IL-5 in the medium was evaluated by ELISA. (B) ROS level in cells was determined using the fluorescent probe DCFH-DA (20 μM). The cells were incubated with gardenin A or hesperidin for 24 h. (C) Cell viability was measured. (D and E) NFAT protein expression was regulated by hesperidin or gardenin A combined with PMA/ionomycin treatment in EL-4 cells for 24 h. The results are expressed as mean ± SD (n = 3). Different letters indicate p < 0.05 compared with each group. | |
3.3. Hesperidin induced HO-1 expression through the transcription factor Nrf2 coupled with the PI3K/AKT or ERK/JNK signaling pathway in EL-4 cells
Heme oxygenase-1 (HO-1) is upregulated in an ROS-dependent manner through the transcription factor nuclear factor-erythroid 2-related factor 2 (Nrf2) or peroxisome proliferator-activated receptor γ (PPARγ) in rosiglitazone-treated human pulmonary alveolar epithelial cells.40 As shown in Fig. 3A and B, upregulated Nrf2 nuclear protein expression and HO-1 antioxidant cytosolic protein levels were observed in EL-4 cells with hesperidin incubation for 2 h. Further investigation of the related kinases that modulate HO-1 expression found an increase in the phosphorylation of PI3K/Akt, ERK, and c-JNK in EL-4 cells with hesperidin treatment (Fig. 3C). However, kinase phosphorylation was impeded by PI3K inhibitor (Wortmannin), MAP kinase inhibitor (PD98059) or JNK inhibitor (SP600125) pretreatment in hesperidin-treated EL-4 cells (Fig. 3D).
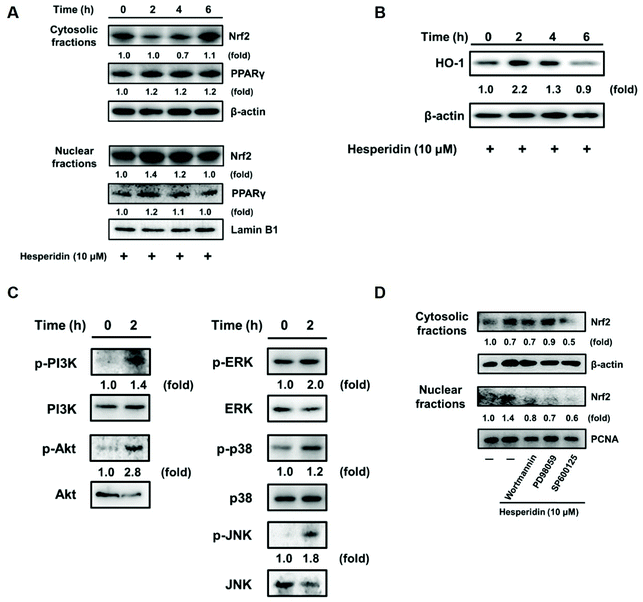 |
| Fig. 3 Hesperidin induced HO-1 expression via the transcription factor Nrf2 coupled with the PI3K/AKT or ERK/JNK signaling pathway in EL-4 cells. Cells were incubated with or without 10 μM hesperidin for 0, 2, 4, and 6 h. Protein expression of (A) transcription factors Nrf2 and PPARγ and (B) HO-1 was measured by western blot analysis. The cells were incubated with or without 10 μM hesperidin for 2 h. (C) PI3K, Akt, ERK, p38, and JNK protein expression was assessed. Cells were pretreated with or without wortmannin (20 μM), PD98059 (20 μM) or SP600125 (20 μM) for 1 h and then incubated with hesperidin (10 μM) for another 2 h. (D) Nrf2 protein expression was analyzed by western blot analysis. The results are expressed as mean ± SD (n = 3). The relative level of protein is expressed as the fold change over the control to normalize against the loading control (Lamin B1, β-actin or PCNA). | |
3.4. Hesperidin suppressed IL-5 production through the transcription factor NFAT in PMA/ionomycin-induced EL-4 cells
Previous evidence demonstrated the negative regulation of NFAT protein expression by the transcription factor Nrf2.41 We therefore assessed NFAT nuclear protein expression by pretreating EL-4 cells with or without an Nrf2 agonist (dimethyl fumarate, DMF) and an Nrf2 antagonist (retinoic acid, RA), followed by hesperidin coupled with PMA/ionomycin treatment. As shown in Fig. 4A, pretreatment with DMF slightly altered NFAT protein expression in hesperidin- and PMA/ionomycin-treated cells compared with PMA/ionomycin-treated cells. Interestingly, NFAT protein expression increased with RA pretreatment in PMA/ionomycin-treated EL-4 cells; however, this increase was abolished by hesperidin treatment in a dose-dependent manner (Fig. 4A). Furthermore, IL-5 levels were evaluated under DMF or RA pretreatment conditions in hesperidin- and PMA/ionomycin-treated EL-4 cells. Pretreatment with DMF significantly inhibited IL-5 secretion in hesperidin- and PMA/ionomycin-treated cells compared with non-DMF-stimulated cells (Fig. 4B). Additionally, elevated IL-5 production by RA pretreatment in PMA/ionomycin-treated EL-4 cells was significantly blocked by 5 or 10 μM hesperidin treatment (Fig. 4B). These results suggest that hesperidin and the signaling it initiates strongly affected NFAT and IL-5 and had suppressive effects even under Nrf2 antagonist stimulation (Fig. 4A and B). Hesperidin-initiated signaling in PMA/ionomycin-treated EL-4 cells was explored. As shown in Fig. 4C and D, the phosphorylation of PI3K, PKC, ERK, JNK, and p38 was promoted by PMA/ionomycin treatment, whereas hesperidin treatment reduced phosphorylation in a dose-dependent manner in PMA/ionomycin-treated EL-4 cells.
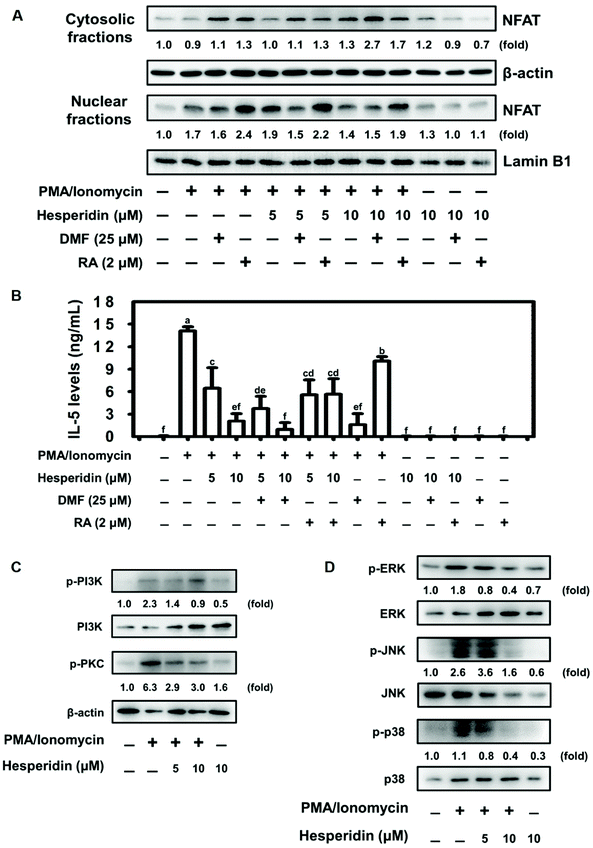 |
| Fig. 4 Hesperidin suppressed IL-5 production through the transcription factor NFAT in PMA/ionomycin-induced EL-4 cells. The cells were pretreated with or without DMF (25 μM) or RA (2 μM) for 1 h and then incubated with or without hesperidin (10 μM) and exposed to PMA/ionomycin for 24 h. After stimulation, the effect of DMF and RA on (A) NFAT protein expression and (B) IL-5 levels were assessed. Effect of hesperidin on (C) PI3K/PKC and (D) ERK/JNK/p38 protein expression in PMA/ionomycin-induced EL-4 cells after 24 h of incubation. The results are expressed as mean ± SD (n = 3). The relative level of protein is expressed as the fold change over the control to normalize against the loading control (Lamin B1 or β-actin). Different letters indicate p < 0.05 compared with each group. | |
3.5. Gardenin A induced HO-1 expression and suppressed IL-5 production through the transcription factor PPARγ in EL-4 cells
As previously mentioned, HO-1 may be regulated by the transcription factors Nrf2 and PPARγ.40 We found that the maximum PPARγ nuclear protein level and HO-1 protein expression were upregulated in EL-4 cells by 10 μM gardenin A treatment for 4 h (Fig. 5A and B). Phosphorylation of the related kinase ERK was increased in gardenin A-treated EL-4 cells (Fig. 5C). Moreover, IL-5 production by PMA/ionomycin-treated EL-4 cells was significantly reduced by gardenin A treatment in a dose-dependent manner (Fig. 5D). Interestingly, a decrease in IL-5 expression by PMA/ionomycin-incubated EL-4 cells that were treated with a PPARγ agonist (Rosiglitazone) coupled with gardenin A was observed (Fig. 5D). In contrast, the opposite result was observed with PPARγ antagonist (GW9662) treatment in PMA/ionomycin-treated EL-4 cells (Fig. 5D).
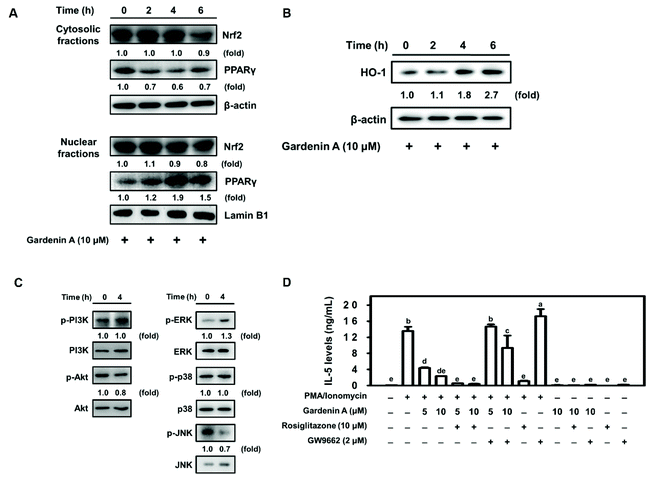 |
| Fig. 5 Gardenin A reduced HO-1 expression through the transcription factor PPARγ in EL-4 cells. Cells were incubated with or without 10 μM gardenin A for 0, 2, 4, and 6 h. Protein expression of (A) transcription factors Nrf2 and PPARγ and (B) HO-1 were measured by western blot analysis. The cells were incubated with or without 10 μM gardenin A for 4 h. (C) PI3K, Akt, ERK, p38, and JNK protein expression was assessed. The cells were pretreated with or without rosiglitazone (10 μM) and GW9662 (2 μM) for 1 h and then incubated with or without gardenin A (10 μM) and exposed to PMA/ionomycin for 24 h. (D) IL-5 levels were assessed. The results are expressed as mean ± SD (n = 3). The relative level of protein is expressed as the fold change over the control to normalize against the loading control (Lamin B1 or β-actin). | |
4. Discussion
The asthmatic-Th2 immune response typically produces the characteristic effector cytokine IL-5 and generates oxidative stress.10–12 Citrus flavonoids have been reported to have a variety of beneficial qualities, including impairing dengue virus replication, ameliorating cardiac hypertrophy, and preventing hepatic steatosis, improving hepatic ischemia-reperfusion injury, as well as antimicrobial activities and antiendotoxemic, anti-inflammatory and antioxidant properties.42–48 However, the effect of citrus flavonoids on the asthmatic inflammatory response is still unclear. Here, we found that citrus flavonoids, including hesperetin and gardenin A, dramatically prohibited ROS and IL-5 production in PMA/ionomycin-induced EL-4 cells.
In this study, citrus flavonoids, including nobiletin, tangeretin, 5-DN, hesperetin, and gardenin A, were used to investigate the suppressive effect on ROS and IL-5 production. IL-5 secretion was increased by nobiletin, tangeretin, and especially 5-DN treatment in PMA/ionomycin-incubated EL-4 cells (Fig. 2A). However, IL-5 has been demonstrated to repress the Th1 response and induction of antigen-specific CD4+CD25+ T regulatory cells,49,50 indicating that 5-DN could be a potential natural compound for preventing autoimmune disease.
NFAT, STAT6, and AP-1 family transcription factors c-Fos and c-Jun are involved in balancing the Th1 and Th2 immune responses.14 NFAT has been shown to be a master transcription factor in enhancing the Th2 response for choreographing the cytokine profile.51,52 In addition, a previous study demonstrated that the NFAT/AP-1 binding element plays an important role in IL-5 synthesis in peripheral T cells from patients with asthma.53 Consistently, our study found that IL-5 production was strongly regulated by the transcription factor NFAT in PMA/ionomycin-treated EL-4 cells (Fig. 1). Treatment with 10 μM hesperetin dramatically suppressed IL-5 secretion by downregulating NFAT nuclear protein expression (Fig. 2).
Increasing HO-1 expression suppressed the asthmatic immune response through MAPK, Nrf2, and PPARγ transcriptional signaling pathways for the therapeutic modulation of inflammation.54 Convincingly, our results demonstrated that hesperetin and gardenin A upregulated HO-1 production in PMA/ionomycin-treated EL-4 cells. Interestingly, hesperidin induced HO-1 expression via the transcription factor Nrf2 coupled with the PI3K/AKT or ERK/JNK signaling pathway in EL-4 cells (Fig. 3). Moreover, gardenin A induced HO-1 expression and suppressed IL-5 production through the transcription factor PPARγ in EL-4 cells (Fig. 5). Hesperetin and gardenin A upregulated HO-1 production through distinct pathways. However, both hesperetin and gardenin A significantly downregulated IL-5 secretion by the transcription factor NFAT (Fig. 2, 3 and 5), indicating that increasing HO-1 expression may inhibit asthmatic inflammation by suppressing IL-5 secretion.
Our study demonstrated that hesperidin induced the activation of Nrf2 and HO-1 via increasing the phosphorylation of the PI3K/AKT or ERK/JNK signaling pathway. These results played an important role in the inhibition of NFAT expression and consequently suppressed IL-5 secretion in PMA/ionomycin-treated EL-4 cells. In addition, gardenin A treatment showed a similar effect in inducing HO-1 expression and suppressing IL-5 production through the transcription factor PPARγ in EL-4 cells. Taken together, downregulating NFAT via the activation of Nrf2 and PPARγ contributed to the hesperidin- and gardenin A-mediated inhibitory effects on IL-5 and ROS production by PMA/ionomycin-incubated EL-4 cells, suggesting that stimulating HO-1 expression may inhibit asthmatic inflammatory effects by suppressing IL-5 secretion and ROS production. Our study showed that citrus flavonoid consumption may protect against asthma pathogenesis through antioxidant properties.
Abbreviations
5-DN | 5-Demethylnobiletin |
AP-1 | Activator protein-1 |
AP1 inhibitor | SR11302 |
DMF | Dimethyl fumarate (Nrf2 agonist) |
GBD | Global burden of disease |
HO-1 | Heme oxygenase-1 |
IL-5 | Interleukin-5 |
NFAT | Nuclear factor of activated T cells |
NO | Nitric oxide |
Nrf2 | Nuclear factor-erythroid 2 related factor 2 |
PMA | Phorbol 12-myristate 13-acetate |
PPARγ | Peroxisome proliferator activated receptor γ |
PPARγ agonist | Rosiglitazone |
PPARγ antagonist | GW9662 |
ROS | Reactive oxygen species |
RA | Retinoic acid (Nrf2 antagonist) |
STAT6 | Signal transducer and activator of transcription 6 |
STAT6 inhibitor | AS1517499 |
Th2 | T helper 2 |
Conflicts of interest
The authors declare no competing financial interest.
Acknowledgements
This research work was supported in part by the grant MOST 108-2320-B-005-001- from the Ministry of Science and Technology, Taiwan.
References
- GBD 2017 Disease and Injury Incidence and Prevalence Collaborators, Global, regional, and national incidence, prevalence, and years lived with disability for 354 diseases and injuries for 195 countries and territories, 1990–2017: a systematic analysis for the Global Burden of Disease Study 2017, Lancet, 2018, 392, 1789–1858 CrossRef.
- C. H. Fanta, Asthma, N. Engl. J. Med., 2009, 360, 1002–1014 CrossRef CAS PubMed.
- M. Guarnieri and J. R. Balmes, Outdoor air pollution and asthma, Lancet, 2014, 383, 1581–1592 CrossRef CAS.
- L. M. Wheatley and A. Togias, Clinical practice. Allergic rhinitis, N. Engl. J. Med., 2015, 372, 456–463 CrossRef PubMed.
- A. Mendy, J. Wilkerson, P. M. Salo, C. H. Weir, L. Feinstein, D. C. Zeldin and P. S. Thorne, Synergistic Association of House Endotoxin Exposure and Ambient Air Pollution with Asthma Outcomes, Am. J. Respir. Crit. Care Med., 2019, 200, 712–720 CrossRef PubMed.
- M. J. Abramson and Y. Guo, Indoor Endotoxin Exposure and Ambient Air Pollutants Interact on Asthma Outcomes, Am. J. Respir. Crit. Care Med., 2019, 200, 652–654 CrossRef PubMed.
- E. L. Stevens, F. Rosser, E. Forno, D. Peden and J. C. Celedon, Can the effects of outdoor air pollution on asthma be mitigated?, J. Allergy Clin. Immunol., 2019, 143, 2016–2018 CrossRef PubMed.
- R. Pawankar, Allergic diseases and asthma: a global public health concern and a call to action, World Allergy Organ. J., 2014, 7, 12 CrossRef PubMed.
- C. Brokamp, E. B. Brandt and P. H. Ryan, Assessing Exposure to Outdoor Air Pollution for Epidemiological Studies: Model-based and Personal Sampling Strategies, J. Allergy Clin. Immunol., 2019, 143, 2002–2006 CrossRef CAS PubMed.
- E. Wambre, V. Bajzik, J. H. DeLong, K. O'Brien, Q. A. Nguyen, C. Speake, V. H. Gersuk, H. A. DeBerg, E. Whalen, C. Ni, M. Farrington, D. Jeong, D. Robinson, P. S. Linsley, B. P. Vickery and W. W. Kwok, A phenotypically and functionally distinct human TH2 cell subpopulation is associated with allergic disorders, Sci. Transl. Med., 2017, 9, eaam9171 CrossRef PubMed.
- T. Nakayama, K. Hirahara, A. Onodera, Y. Endo, H. Hosokawa, K. Shinoda, D. J. Tumes and Y. Okamoto, Th2 Cells in Health and Disease, Annu. Rev. Immunol., 2017, 35, 53–84 CrossRef CAS PubMed.
- D. J. Cousins, Pinning allergies on pathogenic TH2 cells, Sci. Transl. Med., 2017, 9, eaao0392 CrossRef PubMed.
- K. P. Hoefig and V. Heissmeyer, Posttranscriptional regulation of T helper cell fate decisions, J. Cell Biol., 2018, 217, 2615–2631 CrossRef CAS PubMed.
- D. J. Cousins, J. McDonald and T. H. Lee, Therapeutic approaches for control of transcription factors in allergic disease, J. Allergy Clin. Immunol., 2008, 121, 803–809 CrossRef CAS PubMed.
- M. E. Rothenberg and S. P. Hogan, The eosinophil, Annu. Rev. Immunol., 2006, 24, 147–174 CrossRef CAS PubMed.
- B. N. Lambrecht and H. Hammad, The immunology of asthma, Nat. Immunol., 2015, 16, 45–56 CrossRef CAS PubMed.
- H. Iijima, A. Duguet, S. Y. Eum, Q. Hamid and D. H. Eidelman, Nitric oxide and protein nitration are eosinophil dependent in allergen-challenged mice, Am. J. Respir. Crit. Care Med., 2001, 163, 1233–1240 CrossRef CAS PubMed.
- Y. Choi, D. Le Pham, D. H. Lee, S. H. Lee, S. H. Kim and H. S. Park, Biological function of eosinophil extracellular traps in patients with severe eosinophilic asthma, Exp. Mol. Med., 2018, 50, 104 CrossRef PubMed.
- S. E. Wenzel, Eosinophils in asthma–closing the loop or opening the door?, N. Engl. J. Med., 2009, 360, 1026–1028 CrossRef CAS PubMed.
- M. L. Stein, J. M. Villanueva, B. K. Buckmeier, Y. Yamada, A. H. Filipovich, A. H. Assa'ad and M. E. Rothenberg, Anti-IL-5 (mepolizumab) therapy reduces eosinophil activation ex vivo and increases IL-5 and IL-5 receptor levels, J. Allergy Clin. Immunol., 2008, 121, 1473–1483 CrossRef CAS PubMed.
- P. S. Foster, S. P. Hogan, M. Yang, J. Mattes, I. G. Young, K. I. Matthaei, R. K. Kumar, S. Mahalingam and D. C. Webb, Interleukin-5 and eosinophils as therapeutic targets for asthma, Trends Mol. Med., 2002, 8, 162–167 CrossRef CAS PubMed.
- P. Haldar, C. E. Brightling, B. Hargadon, S. Gupta, W. Monteiro, A. Sousa, R. P. Marshall, P. Bradding, R. H. Green, A. J. Wardlaw and I. D. Pavord, Mepolizumab and exacerbations of refractory eosinophilic asthma, N. Engl. J. Med., 2009, 360, 973–984 CrossRef CAS PubMed.
- F. H. Messerli, Chocolate consumption, cognitive function, and Nobel laureates, N. Engl. J. Med., 2012, 367, 1562–1564 CrossRef CAS PubMed.
- V. Garcia, I. C. Arts, J. A. Sterne, R. L. Thompson and S. O. Shaheen, Dietary intake of flavonoids and asthma in adults, Eur. Respir. J., 2005, 26, 449–452 CrossRef CAS PubMed.
- S. O. Shaheen, J. A. Sterne, R. L. Thompson, C. E. Songhurst, B. M. Margetts and P. G. Burney, Dietary antioxidants and asthma in adults: population-based case-control study, Am. J. Respir. Crit. Care Med., 2001, 164, 1823–1828 CrossRef CAS PubMed.
- V. Mishra, J. Banga and P. Silveyra, Oxidative stress and cellular pathways of asthma and inflammation: Therapeutic strategies and pharmacological targets, Pharmacol. Ther., 2018, 181, 169–182 CrossRef CAS PubMed.
- S. Higa, T. Hirano, M. Kotani, M. Matsumoto, A. Fujita, M. Suemura, I. Kawase and T. Tanaka, Fisetin, a flavonol, inhibits TH2-type cytokine production by activated human basophils, J. Allergy Clin. Immunol., 2003, 111, 1299–1306 CrossRef CAS PubMed.
- S. H. Kim, B. K. Kim and Y. C. Lee, Antiasthmatic effects of hesperidin, a potential Th2 cytokine antagonist, in a mouse model of allergic asthma, Mediators Inflammation, 2011, 2011, 485402 Search PubMed.
- Z. Zou, W. Xi, Y. Hu, C. Nie and Z. Zhou, Antioxidant activity of Citrus fruits, Food Chem., 2016, 196, 885–896 CrossRef CAS PubMed.
- H. Parhiz, A. Roohbakhsh, F. Soltani, R. Rezaee and M. Iranshahi, Antioxidant and anti-inflammatory properties of the citrus flavonoids hesperidin and hesperetin: an updated review of their molecular mechanisms and experimental models, Phytother. Res., 2015, 29, 323–331 CrossRef CAS PubMed.
- D. Barreca, G. Gattuso, E. Bellocco, A. Calderaro, D. Trombetta, A. Smeriglio, G. Lagana, M. Daglia, S. Meneghini and S. M. Nabavi, Flavanones: Citrus phytochemical with health-promoting properties, BioFactors, 2017, 43, 495–506 CrossRef CAS PubMed.
- T. T. Xing, X. J. Zhao, Y. D. Zhang and Y. F. Li, Fast Separation and Sensitive Quantitation of Polymethoxylated Flavonoids in the Peels of Citrus Using UPLC-Q-TOF-MS, J. Agric. Food Chem., 2017, 65, 2615–2627 CrossRef CAS PubMed.
- Y. H. Chen, H. Y. Chen, C. L. Hsu and G. C. Yen, Induction of apoptosis by the Lactuca indica L. in human leukemia cell line and its active components, J. Agric. Food Chem., 2007, 55, 1743–1749 CrossRef CAS PubMed.
- Y. T. Cheng, J. A. Lin, J. J. Jhang and G. C. Yen, Protocatechuic acid-mediated DJ-1/PARK7 activation followed by PI3K/mTOR signaling pathway activation as a novel mechanism for protection against ketoprofen-induced oxidative damage in the gastrointestinal mucosa, Free Radicals Biol. Med., 2019, 130, 35–47 CrossRef CAS PubMed.
- C. Y. Ho, Y. T. Cheng, C. F. Chau and G. C. Yen, Effect of diallyl sulfide on in vitro and in vivo Nrf2-mediated pulmonic antioxidant enzyme expression via activation ERK/p38 signaling pathway, J. Agric. Food Chem., 2012, 60, 100–107 CrossRef CAS PubMed.
- H. Y. Chang, S. Y. Chen, C. H. Wu, C. C. Lu and G. C. Yen, Glycyrrhizin Attenuates the Process of Epithelial-to-Mesenchymal Transition by Modulating HMGB1 Initiated Novel Signaling Pathway in Prostate Cancer Cells, J. Agric. Food Chem., 2019, 67, 3323–3332 CrossRef CAS PubMed.
- S. Klein-Hessling, R. Rudolf, K. Muhammad, K. P. Knobeloch, M. A. Maqbool, P. Cauchy, J. C. Andrau, A. Avots, C. Talora, V. Ellenrieder, I. Screpanti, E. Serfling and A. K. Patra, A threshold level of NFATc1 activity facilitates thymocyte differentiation and opposes notch-driven leukaemia development, Nat. Commun., 2016, 7, 11841 CrossRef CAS PubMed.
- W. H. Hsu, B. H. Lee, Y. W. Hsu and T. M. Pan, Inhibition of Th2 cytokine production in T cells by monascin via PPAR-gamma activation, J. Agric. Food Chem., 2013, 61, 8126–8133 CrossRef CAS PubMed.
- A. S. Cheng, Y. H. Cheng and T. L. Chang, Scopoletin attenuates allergy by inhibiting Th2 cytokines production in EL-4 T cells, Food Funct., 2012, 3, 886–890 RSC.
- R. L. Cho, C. C. Yang, H. C. Tseng, L. D. Hsiao, C. C. Lin and C. M. Yang, Haem oxygenase-1 up-regulation by rosiglitazone via ROS-dependent Nrf2-antioxidant response elements axis or PPARgamma attenuates LPS-mediated lung inflammation, Br. J. Pharmacol., 2018, 175, 3928–3946 CrossRef CAS PubMed.
- S. Hyeon, H. Lee, Y. Yang and W. Jeong, Nrf2 deficiency induces oxidative stress and promotes RANKL-induced osteoclast differentiation, Free Radicals Biol. Med., 2013, 65, 789–799 CrossRef CAS PubMed.
- J. D. Toscano-Garibay, M. Arriaga-Alba, J. Sanchez-Navarrete, M. Mendoza-Garcia, J. J. Flores-Estrada, M. A. Moreno-Eutimio, J. J. Espinosa-Aguirre, M. Gonzalez-Avila and N. J. Ruiz-Perez, Antimutagenic and antioxidant activity of the essential oils of Citrus sinensis and Citrus latifolia, Sci. Rep., 2017, 7, 11479 CrossRef CAS PubMed.
- J. H. Park, H. J. Ku, J. K. Kim, J. W. Park and J. H. Lee, Amelioration of High Fructose-Induced Cardiac Hypertrophy by Naringin, Sci. Rep., 2018, 8, 9464 CrossRef PubMed.
- A. Mosqueda-Solis, J. Sanchez, B. Reynes, M. Palou, M. P. Portillo, A. Palou and C. Pico, Hesperidin and capsaicin, but not the combination, prevent hepatic steatosis and other metabolic syndrome-related alterations in western diet-fed rats, Sci. Rep., 2018, 8, 15100 CrossRef PubMed.
- X. Liu, N. Wang, S. Fan, X. Zheng, Y. Yang, Y. Zhu, Y. Lu, Q. Chen, H. Zhou and J. Zheng, The citrus flavonoid naringenin confers protection in a murine endotoxaemia model through AMPK-ATF3-dependent negative regulation of the TLR4 signalling pathway, Sci. Rep., 2016, 6, 39735 CrossRef CAS PubMed.
- S. Frabasile, A. C. Koishi, D. Kuczera, G. F. Silveira, W. A. Verri Jr., C. N. Duarte Dos Santos and J. Bordignon, Corrigendum: The citrus flavanone naringenin impairs dengue virus replication in human cells, Sci. Rep., 2017, 7, 43976 CrossRef CAS PubMed.
- T. Dusabimana, S. R. Kim, H. J. Kim, S. W. Park and H. Kim, Nobiletin ameliorates hepatic ischemia and reperfusion injury through the activation of SIRT-1/FOXO3a-mediated autophagy and mitochondrial biogenesis, Exp. Mol. Med., 2019, 51, 51 CrossRef PubMed.
- A. Anwar, A. Masri, K. Rao, K. Rajendran, N. A. Khan, M. R. Shah and R. Siddiqui, Antimicrobial activities of green synthesized gums-stabilized nanoparticles loaded with flavonoids, Sci. Rep., 2019, 9, 3122 CrossRef PubMed.
- G. T. Tran, S. J. Hodgkinson, N. M. Carter, N. D. Verma, K. M. Plain, R. Boyd, C. M. Robinson, M. Nomura, M. Killingsworth and B. M. Hall, IL-5 promotes induction
of antigen-specific CD4+CD25+T regulatory cells that suppress autoimmunity, Blood, 2012, 119, 4441–4450 CrossRef CAS PubMed.
- E. Guenova, R. Watanabe, J. E. Teague, J. A. Desimone, Y. Jiang, M. Dowlatshahi, C. Schlapbach, K. Schaekel, A. H. Rook, M. Tawa, D. C. Fisher, T. S. Kupper and R. A. Clark, TH2 cytokines from malignant cells suppress TH1 responses and enforce a global TH2 bias in leukemic cutaneous T-cell lymphoma, Clin. Cancer Res., 2013, 19, 3755–3763 CrossRef CAS PubMed.
- J. Fric, T. Zelante, A. Y. Wong, A. Mertes, H. B. Yu and P. Ricciardi-Castagnoli, NFAT control of innate immunity, Blood, 2012, 120, 1380–1389 CrossRef CAS PubMed.
- G. R. Crabtree and E. N. Olson, NFAT signaling: choreographing the social lives of cells, Cell, 2002, 109(Suppl), S67–S79 CrossRef CAS PubMed.
- K. Ogawa, O. Kaminuma, H. Okudaira, H. Kikkawa, K. Ikezawa, N. Sakurai and A. Mori, Transcriptional regulation of the IL-5 gene in peripheral T cells of asthmatic patients, Clin. Exp. Immunol., 2002, 130, 475–483 CrossRef CAS PubMed.
- C. Wang, Y. H. Choi, Z. Xian, M. Zheng, H. Piao and G. Yan, Aloperine suppresses allergic airway inflammation through NF-kappaB, MAPK, and Nrf2/HO-1 signaling pathways in mice, Int. Immunopharmacol., 2018, 65, 571–579 CrossRef CAS PubMed.
Footnote |
† These authors contributed equally to this work. |
|
This journal is © The Royal Society of Chemistry 2020 |