DOI:
10.1039/C9FO01461F
(Paper)
Food Funct., 2020,
11, 111-122
Pearl millet (Pennisetum glaucum) couscous breaks down faster than wheat couscous in the Human Gastric Simulator, though has slower starch hydrolysis†
Received
5th July 2019
, Accepted 20th November 2019
First published on 22nd November 2019
Abstract
Consumption of traditional West African pearl millet (Pennisetum glaucum) couscous delayed gastric emptying in our recent human study compared to other starch-based foods (white rice, boiled potatoes, pasta). The objective of this study was to determine whether physical properties of pearl millet couscous affect particle breakdown and starch hydrolysis during simulated gastric digestion to understand the basis of the slow gastric emptying. Starch fine structure and viscosity were analyzed for initial millet and wheat couscous samples by high performance size-exclusion chromatography and the Rapid Visco Analyzer, respectively. Couscous samples were subjected to simulated gastric digestion using the Human Gastric Simulator (HGS), a dynamic model of human gastric digestion. Digesta was collected from the HGS at 30 min intervals over 180 min. Particle size and percent starch hydrolysis of couscous in the digesta were evaluated at each time point. The number of particles per gram of dry mass substantially increased over digestion time for millet couscous (p < 0.05), while changed little for the wheat couscous samples. Millet couscous showed lower starch hydrolysis per unit surface area of particles than wheat couscous (p < 0.05). Slower starch hydrolysis was associated with smaller (p < 0.05) amylose chain length for millet (839–963 DP) than for wheat (1225–1563 DP), which may enable enable a denser packing of millet starch molecules that impedes hydrolysis. We hypothesize that the slow gastric emptying rate of millet couscous observed in humans may be explained by its slow starch hydrolysis property that could activate the ileal brake system, independent of high particle breakdown rate in the stomach.
Introduction
Increasing prevalence of obesity is indicative of dysregulation in the control of appetite and energy homeostasis. Thus, there is a need for foods with satiating properties that can better promote health. Slow gastric emptying is associated with increased satiety.1,2 Our recent human study showed that couscous made from pearl millet (Pennisetum glaucum), a traditional West African food, substantially delayed gastric emptying rate compared to foods typically consumed as part of the Western diet (white rice, boiled potatoes, and pasta).3 However, the underlying cause of the observed difference was not well understood. Previous research has identified the importance of rate of food breakdown in the stomach and suggested that foods which resist breakdown have a slower gastric emptying rate.4–7 It is also hypothesized that a slow starch hydrolysis property of pearl millet-based foods, including couscous, plays a role in their slow gastric emptying through activation of the ileal brake feedback mechanism in the body.8
The emptying of food from the stomach is a complex process, as it depends on food breakdown, physical properties of digesta, and physiological regulation.9–11 It has been found that food particles larger than approximately 2 mm in size are retained by the pyloric valve through a phenomenon called gastric sieving, which contributes to longer stomach retention times for foods that resist particle breakdown.12 Accordingly, foods which break down more slowly in the stomach have been found to delay gastric emptying.13 However, gastric emptying rate is also controlled by the presence of macronutrients, including starch and partially hydrolysed starch in the distal small intestine, triggering the ileal brake feedback response.14–16
The objective of this work was to determine how pearl millet couscous, which will hereafter be referred to as millet couscous, breaks down in a simulated gastric environment and whether it is resistant to breakdown; and to determine starch hydrolysis rate related to potential ileal brake activation. In both procedures, millet was compared to wheat couscous. Three types of millet couscous (self-made with large and small final particle sizes as well as a commercial type from Senegal) were studied along with two types of wheat couscous (self-made small and a commercial type). Initial flour particle size was controlled and the final couscous particle size was matched in three types of couscous so that effects due to the differences in particle breakdown, and not differences in flour or initial particle size, could be elucidated. Starch fine structural features and rheological properties of initial couscous samples were analyzed.
Materials and methods
Raw material preparation
Couscous materials.
Whole grain pearl millet (Pennisetum glaucum) grain was obtained as intact kernels (Alif Group, Dakar, Senegal) to be made into couscous. Commercially prepared millet couscous from Dakar, Senegal was also acquired and evaluated to represent couscous typically consumed in Western Africa (Mme. Deme of Free Work Services, Dakar, Senegal). Wheat flour was obtained from a commercial source (Bob's Red Mill, Milwaukie, OR, USA) and was made into wheat couscous. Commercial wheat couscous was purchased as a comparator (Riviana Foods Inc., Houston, TX, USA).
Couscous pre-processing.
Millet grain was decorticated using an abrasive decorticator (15% loss in mass, resulting in bran removal). The kernels and bran were separated using a sieve shaker (Smico Corp., Oklahoma City, OK, USA) such that particles greater than approximately 1 mm were retained as decorticated kernels and were milled to flour using a pin mill operated at 5.5 rpm (Alpine, 160 Z, Augsburg, Germany). The millet flour was then separated into different particle size fractions using a sieve shaker (Model RX-24, W.S. Tyler Inc., Mentor, OH, USA). Millet flour particles between 300–495 μm were used to make the millet couscous.
Flour for wheat couscous was obtained commercially, so no decortication or milling was necessary. Wheat flour was separated into the same particle size fractions as the millet flour using the procedure already described. Wheat flour particles between 300–495 μm were used to make the wheat couscous.
Couscous preparation.
Couscous was prepared according to a traditional West African method with the expertise of a Senegalese native with more than 15 years of experience preparing couscous. Briefly, flour (500 g wet weight) was weighed and water (283 ± 11 mL) was gradually added as the mixture was rolled continuously by hand into small couscous particulate spheres. These spheres were then passed through a 1.70 mm or 2.36 mm sieve to constitute the small and large couscous samples, respectively. All retained couscous spheres were sieved again before being steamed in a couscoussier for 14 min over boiling water (100–120 °C). For uniformity and storage, samples were dried at 50 °C for 240 min following steaming. Prior to simulated gastric digestion, all couscous samples were hydrated with water (2.5
:
1 g couscous
:
mL water, wet basis) and steamed 10 min, after which they were immediately used for simulated digestion. In total, five types of couscous were used in this study (Table 1).
Table 1 Types of couscous. Source of each couscous is given in addition to its initial (before digestion, dry) particle size as determined by sieving
Couscous type |
Source |
Initial particle size (mm) |
Wheat small |
Flour from Bob's Red Mill, Milwaukie, OR, USA |
0.60–1.70 |
Couscous self-made at Purdue University, IN, USA |
Wheat commercial |
Riviana Foods Inc., Houston, TX, USA |
0.60–2.36 |
Millet small |
Grain from Alif Group, Dakar, Senegal |
0.60–1.70 |
Couscous self-made at Purdue University, IN, USA |
Millet large |
Grain from Alif Group, Dakar, Senegal |
1.70–2.36 |
Couscous self-made at Purdue University, IN, USA |
Millet commercial |
Mme. Deme of Free Work Services, Dakar, Senegal |
0.30–2.36 |
Couscous characterization
Rapid Visco Analyzer analysis.
Pasting profiles of couscous (small and large millet couscous combined, small wheat combined with an additional large wheat couscous treatment) without the second steaming step, and raw flours, were determined using a Rapid Visco Analyzer (RVA; model RVA-4, Perten Instruments Instrumentvägen 29, SE-126 53 Hägersten, Sweden) using the Standard 1 protocol supplied with the instrument. This protocol consists of holding at 50 °C for 1 min, heating at a rate of 12 °C min−1 to 95 °C, equilibrating at 95 °C for 2.5 min, cooling at a rate of 12 °C min−1 to 50 °C, and holding at 50 °C for 2 min. The RVA mixing paddle speed was 960 rpm for the first 10 s and then 160 rpm for the remainder of the experiment. Slurries were made for each sample (3 g dry basis) with water (25 mL for millet flour and wheat flour; 15 mL for millet couscous and wheat couscous).
Starch fine structure.
Molecular size and unit chain length distribution of amylose and amylopectin (starch structure) were characterized for all couscous samples along with the flour starting materials for the self-made couscous by high performance size-exclusion chromatography (HPSEC) following the procedure of Roman et al.17
Light microscopy.
Light microscopy was also performed as in Roman et al.17 for all initial flour and couscous samples.
Simulated oral and gastric digestion
Simulated saliva formulation.
Simulated saliva was prepared according to Bornhorst and Singh.18 Briefly, mucin (1 g L−1, Sigma-Aldrich, St Louis, MO, USA), α-amylase (from Bacillus subtilis, 1.18 g L−1, MP Biomedicals, Catalog Number 100447, 160
000 BAU g−1 activity, Santa Ana, CA, USA), NaCl (0.117 g L−1, Avantor Performance Materials, Radnor, PA, USA), KCl (0.149 g L−1, ThermoFisher Scientific, Waltham, MA, USA) and NaHCO3 (2.10 g L−1, ThermoFisher Scientific, Waltham, MA, USA) were mixed in deionized water. The concentration of α-amylase was set to reflect its activity in vivo.19
Simulated gastric juice formulation.
Simulated gastric juice was prepared according to Mennah-Govela and Bornhorst.20 Mucin (1.5 g L−1, Sigma-Aldrich, St Louis, MO, USA), NaCl (8.78 g L−1, Sigma-Aldrich, St Louis, MO, USA), and pepsin from porcine pancreas (1.0 g L−1, Sigma-Aldrich, St Louis, MO, USA) were mixed in deionized water (acidified to pH 1.8 using 3 M HCl). After all components were dissolved, pH was adjusted to 1.8 using 3 M HCl. Pepsin concentration was chosen to provide an activity of 2000 U mL−1 in simulated gastric juice,21,22 and pH was set to 1.8 to simulate the fasted pH of gastric juice.23
Simulated oral and gastric digestion procedure.
Following steaming, 300 g cooked couscous was weighed, and 60 mL of simulated saliva was added (0.2 mL g−1) and mixed for 30 s to represent the oral phase.24
Simulated gastric digestions were conducted using the HGS to simulate the peristaltic movement of the human stomach (Fig. 1). The HGS has been described in detail previously.25,26 Briefly, the HGS utilizes rollers to apply a simulated peristaltic wave (3 contractions per minute) to the food and simulated gastric juice,13,27,28 which are contained in a flexible plastic bag. The temperature is maintained at 37 °C.
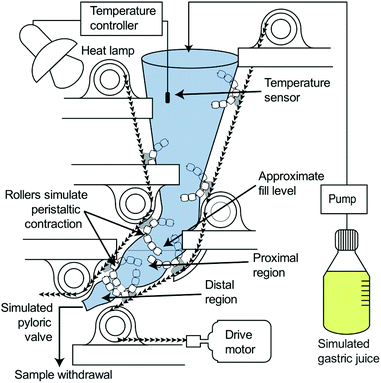 |
| Fig. 1 Diagram of the human gastric simulator (HGS). | |
Simulated gastric digestions were initiated immediately after the oral phase by placing the test meal into the HGS which was preloaded with 75 mL gastric juice preheated to 37 °C. A peristaltic pump (Model 13-876-2, ThermoFisher Scientific, Waltham, MA, USA) was used to continuously secrete simulated gastric juice at 2.5 mL min−1.12,29 Simulated gastric digestions were carried out for 180 min, with 90 mL samples of digesta (approximately 100 g wet weight) collected from the simulated pyloric valve every 30 min. The simulated pyloric valve consisted of a small opening (1 cm) in the antral region of the simulated stomach chamber, which released the sample at each predetermined time point. Due to the small initial size of the couscous particles (1.70–2.36 mm), the pyloric valve did not prevent the passage of larger particles. After collection, samples of digesta were analyzed for moisture content, pH, particle size, and reducing sugar content. Digestions were conducted in triplicate except for small millet couscous which was done in quadruplicate.
Moisture content
Digesta was collected at each time point and 3 g, weighed into pre-dried aluminum pans, was dried in a vacuum oven (Lindberg Blue M, Thermo Scientific, Waltham, MA, USA) for 20 h at 120 °C (AACCI Method 44-40.01).
pH measurement
Digesta from each time point was placed in a 50 mL conical tube for pH measurement (IQ Scientific IQ150-77 ISFET, Cole-Parmer, Vernon Hills, IL, USA).
Particle size analysis
Image analysis was used to determine the size distribution of couscous particles in the digesta according to a previously described method with minor modifications.30 Following collection of digesta from the HGS, an aliquot of 0.48 ± 0.01 g was dispersed into several Petri dishes, each containing 20 mL deionized water. Multiple dishes were used for each sample in order to minimize particle overlap. Lugol's iodine solution (5–10 μL) was added to each Petri dish to enhance the contrast between the light field and the particles. Petri dishes were illuminated from underneath using a lightbox (AGPtek HL0163, Brooklyn, NY, USA; color temperature 6000 K). A reference object (ABFO No. 2 photomacrographic standard reference scale) was included in all images for spatial calibration. One image of each dish was captured using a Canon EOS Rebel SL1 digital camera (18 Mega Pixels, APS-C CMOS sensor, Canon USA, INC. San Jose, CA, USA) that was fixed to a vertical support and triggered using a computer interface. The camera settings were: no flash, 35 mm focal length, aperture F8.0, ISO 100, and shutter speed 0.1 s. Images were analyzed using MATLAB (MathWorks, Natick, MA, USA) to determine the total number of particles in each image and the area of each particle. Particles per gram of dry mass was defined as the number of particles in a sample of digesta divided by the dry mass of the sample, as determined by moisture content analysis. The particle size distribution was analyzed by fitting the cumulative area percentage of the particles in each sample to the Rosin-Rammler model:31 | 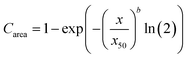 | (1) |
where Carea is the cumulative area percentage of each particle (0 to 100%), x50 is the median particle area (mm2), and b is the distribution breadth constant (dimensionless). Smaller b values represent a broader distribution spread. This model has been used by previous researchers to describe the size changes of solid food particles during oral and gastric digestion using image analysis.4,30,32
Starch hydrolysis analysis
Aliquots of digesta samples from each time point were centrifuged at 1000g, upon which the supernatant was mixed with 0.3 M sodium carbonate (200 μL) and stored until analysis was performed. Reducing sugar content was then quantified using the dinitrosalicylic acid (DNS) method33 and expressed as percent starch hydrolysis. Due to differences in surface area resulting from the different particle breakdown profiles observed during the simulated digestion experiments, percent starch hydrolysis at each time point was then divided by the total area of couscous particles from image analysis to obtain a value of starch hydrolysis per unit area of digested particles (% starch hydrolysis per mm2), similar to the approach used by Ratanpaul et al.34
Relative gastric emptying of solids from the HGS
Samples of digesta were subjected to gravimetric moisture content analysis as described above to determine the solid matter content. The relative gastric emptying of solids was expressed as the remaining dry matter in the HGS at each time point divided by the initial dry matter. Since the overall emptying rate of digesta (90 mL every 30 min) was held constant for all types of couscous, the relative gastric emptying of solids reflected the solids content of digesta samples that were withdrawn from the HGS. For each type of couscous, the curve of relative gastric emptying of solids was fit to a modified power-exponential model used by previous researchers to fit gastric emptying data:5,35,36 | y(t) = 1 − (1 − e−kt)β | (2) |
where y(t) is the percent of initial dry matter retained in the HGS at time t, t is digestion time (min), k is the emptying rate parameter (min−1), and β is the extrapolated y-intercept from the terminal portion of the curve (dimensionless). The fit was conducted using nonlinear least squares in MATLAB (MathWorks, Natick, MA, USA). The half-time for relative gastric emptying of solids was estimated using eqn (3): | 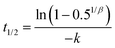 | (3) |
where t1/2 is the estimated time at which 50% of the dry mass present at time zero was emptied from the HGS (min), and the parameters k and β were from eqn (2).
Statistical analysis
Statistical analysis was conducted using SAS Enterprise Guide 7.1 (SAS Institute, Cary NC, USA). Initial properties of couscous were analyzed using a single factor ANOVA with type of couscous as the factor. Variables that were measured at each time point during simulated gastric digestion (median particle area, particles per gram, moisture content, pH, and starch hydrolysis per unit area) were analyzed using a two factor ANOVA with repeated measures (PROC MIXED). If the F value of the overall model was significant (p < 0.05), post hoc tests were conducted using Tukey's HSD and significance was taken at p < 0.05.
Results
Couscous properties
Rapid Visco Analyzer analysis.
To determine if initial material properties (i.e. viscosity) of couscous could help explain their behavior during simulated gastric digestion, analysis using the Rapid Visco Analyzer (RVA) was conducted. Viscosity profiles obtained using the RVA are shown in Fig. 2. Wheat couscous exhibited an initial increase in viscosity before heating (0–250 s), referred to as cold swelling, while millet couscous did not. Peak viscosity (at ∼320 s and 95 °C) was lower for millet couscous than wheat couscous (2791 ± 51 cP and 4084 ± 137 cP, respectively, p < 0.01). The final viscosity measured during RVA was 8206 ± 116 cP for wheat couscous, 2761 ± 28 cP for wheat flour, 5793 ± 22 cP for millet couscous, and 3887 ± 11 cP for millet flour. All final viscosity values were statistically different from each other (p < 0.01).
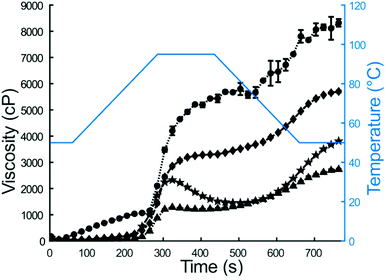 |
| Fig. 2 RVA profiles of wheat couscous (●), wheat flour (▲), millet couscous (◆), and millet flour (★). Error bars represent the standard deviation of two runs and in some cases may be too small to be seen. Viscosity was recorded every 4 seconds, but for clarity a symbol has been placed on every fifth data point (every 20 s). | |
Starch fine structure.
Starch fine structure was analyzed using HPSEC (Table 2). No significant differences were observed in amylopectin chain lengths between wheat and millet samples. However, there were statistically significant differences in amylose chain length between the wheat and millet flours and couscous [degree of polymerization (DP), p < 0.05]. Notably, wheat flour had the highest amylose chain length as represented by DP (1563.3 ± 38.5 DP), while small and large millet couscous samples had the lowest amylose chain lengths (839.0 ± 42.2 DP and 865.8 ± 24.9 DP, respectively). All millet samples had smaller amylose chain lengths than wheat samples, although statistically significant differences were not observed across all samples (Table 2).
Table 2 Starch structural characterization of initial (undigested) couscous as well as for flour starting materials used for self-made couscous types. Values in each column that do not share a letter (abc) represent significant differences (p < 0.05) between different types of couscous or flour. DP = degree of polymerization. If no letter is shown, there were no statistically significant differences
|
Amylopectin short chain length (DP) |
Amylopectin long chain length (DP) |
Amylose chain length (DP) |
Molar ratio of long to short amylopectin chains |
Amylose ratio (%) |
Wheat flour |
13.1 ± 0.1 |
38.8 ± 0.5 |
1563.3 ± 38.5a |
0.541 ± 0.012 |
33.5 ± 2.6 |
Millet flour |
14.7 ± 0.1 |
38.9 ± 0.2 |
962.6 ± 26.5bc |
0.532 ± 0.002 |
29.9 ± 2.7 |
Small wheat couscous |
13.3 ± 0.2 |
39.1 ± 0.5 |
1224.9 ± 105.7b |
0.550 ± 0.004 |
34.7 ± 0.2 |
Commercial wheat couscous |
16.1 ± 3.0 |
37.2 ± 2.4 |
1250.5 ± 123.2ab |
0.593 ± 0.042 |
35.6 ± 0.3 |
Small millet couscous |
14.9 ± 0.1 |
38.7 ± 0.1 |
839.0 ± 42.2c |
0.540 ± 0.009 |
33.8 ± 4.1 |
Large millet couscous |
14.9 ± 0.1 |
39.1 ± 0.3 |
865.8 ± 24.9c |
0.533 ± 0.004 |
32.6 ± 3.2 |
Commercial millet couscous |
14.6 ± 0.2 |
38.0 ± 1.6 |
939.0 ± 47.1bc |
0.598 ± 0.122 |
38.2 ± 9.3 |
Light microscopy.
Light micrographs of initial wheat and millet flour and couscous samples showed intact cell structures within the particles (Fig. S1†). Average millet cell diameter was estimated to be ∼0.4 mm; wheat cells differed more in shape but were of a similar dimension.
Particle size analysis
The Rosin-Rammler model (eqn (1)) was fit to the cumulative distribution of particle areas as measured using image analysis. It was found that the Rosin-Rammler function provided a good fit to the data as evidenced by a high coefficient of determination, with a minimum R2 of 0.945 across all types of couscous and gastric sampling time points, and the average R2 across all treatments of 0.980. Median particle area, as represented by Rosin-Rammler x50 (mm2), was significantly influenced by digestion time (p < 0.01), type of couscous (p < 0.01), and their interaction (p < 0.01). The particle area of couscous experienced a large and statistically significant decrease throughout the simulated digestion for all types of couscous (p < 0.05) (Table 3). For example, x50 of commercial millet after 30 s of simulated oral phase was 1.62 mm2 and decreased to 0.2 mm2 for the sample that underwent 180 min of simulated gastric digestion in the HGS.
Table 3 Median particle size, quantified by Rosin-Rammler x50 (eqn (1)), and particle size distribution spread parameter, quantified by Rosin-Rammler b (eqn (1)), for particles in digesta withdrawn from the HGS at different time points and for different types of couscous. All values are means of multiple runs in the HGS (n = 3 runs, except for millet small which was n = 4) ± standard deviation. Values in each column that do not share a letter (abc) represent significant differences (p < 0.05) within a certain type of couscous across different digestion times. Values in each row that do not share a letter (zyx) represent significant differences (p < 0.05) within a certain time point across different types of couscous. If no letter is shown, there were no statistically significant differences
|
x
50 (mm2) |
b (dimensionless) |
Digestion time (min) |
Wheat small |
Wheat commercial |
Millet small |
Millet large |
Millet commercial |
Wheat small |
Wheat commercial |
Millet small |
Millet large |
Millet commercial |
0.5 |
2.09 ± 0.73a,yx |
3.77 ± 0.11a,z |
1.45 ± 0.09a,w |
2.39 ± 0.30a,y |
1.62 ± 0.52a,xw |
1.33 ± 0.03a,yx |
1.72 ± 0.36a,z |
1.47 ± 0.12a,zy |
1.06 ± 0.26,x |
1.09 ± 0.21,x |
30 |
0.75 ± 0.19b,y |
2.27 ± 0.63bc,z |
0.15 ± 0.02b,y |
0.16 ± 0.02b,y |
0.42 ± 0.10b,y |
1.10 ± 0.15ab,z |
0.82 ± 0.02b,zy |
1.03 ± 0.05b,zy |
0.99 ± 0.04,zy |
0.79 ± 0.01y |
60 |
0.56 ± 0.06b,y |
1.53 ± 0.41de,z |
0.14 ± 0.02b,y |
0.15 ± 0.03b,y |
0.25 ± 0.05b,y |
1.08 ± 0.14ab |
0.84 ± 0.07b |
1.09 ± 0.01b |
1.10 ± 0.05 |
0.88 ± 0.03 |
90 |
0.59 ± 0.03b,y |
1.61 ± 0.65ce,z |
0.16 ± 0.03b,y |
0.14 ± 0.03b,y |
0.23 ± 0.04b,y |
1.07 ± 0.07ab |
0.90 ± 0.17b |
1.02 ± 0.04b |
1.11 ± 0.03 |
0.90 ± 0.04 |
120 |
0.57 ± 0.09b,y |
2.50 ± 0.34b,z |
0.13 ± 0.03b,y |
0.12 ± 0.03b,y |
0.19 ± 0.01b,y |
1.11 ± 0.10ab |
0.86 ± 0.11b |
1.03 ± 0.05b |
1.10 ± 0.05 |
0.92 ± 0.05 |
150 |
0.76 ± 0.21b,y |
1.80 ± 0.84bcde,z |
0.27 ± 0.15b,y |
0.11 ± 0.03b,y |
0.19 ± 0.04b,y |
1.01 ± 0.10ab |
0.92 ± 0.33b |
0.90 ± 0.12b |
1.09 ± 0.08 |
0.89 ± 0.04 |
180 |
0.85 ± 0.19b,zy |
1.06 ± 0.76de,z |
0.18 ± 0.12b,x |
0.17 ± 0.07b,x |
0.20 ± 0.09b,yx |
0.98 ± 0.06b |
0.81 ± 0.08b |
1.02 ± 0.25b |
0.95 ± 0.09 |
0.85 ± 0.08 |
The spread of the particle size distribution is represented by Rosin-Rammler b (dimensionless), where a smaller value of b indicates a wider distribution of particle sizes and a larger value of b indicates a narrower distribution of particle sizes. Rosin-Rammler b was significantly influenced by digestion time (p < 0.01), type of couscous (p < 0.01), and their interaction (p < 0.01) (Table 3). Post-hoc tests showed that statistically significant differences in the value of b between different types of couscous were only present during the first two time points (after 30 s oral phase, and after 30 s oral phase followed by 30 min gastric digestion, respectively). This suggests that the spread of the particle size distribution was different between types of couscous at the beginning of simulated digestion, but that after 60 min of simulated digestion in the HGS the differences in distribution spread between different types of couscous were no longer significant.
Particle size analysis also allowed for quantification of the number of particles per gram of dry mass in the digesta. Particles per gram of dry mass in the digesta was significantly influenced by digestion time (p < 0.01), type of couscous (p < 0.01), and their interaction (p < 0.01) (Fig. 3). For all types of millet couscous there was a large and statistically significant increase in particles per gram of dry mass during simulated digestion. For example, small millet couscous had 7.0 × 103 particles per gram after 30 s of simulated oral phase, but after 180 min in the HGS had 88.8 × 103 particles per gram (p < 0.05). Results showed that higher particle breakdown occurred during simulated gastric digestion of millet couscous leading to a substantial and statistically significant increase in the number of particles per gram dry mass, whereas in wheat couscous there was not a statistically significant increase in the number of particles per gram of dry mass.
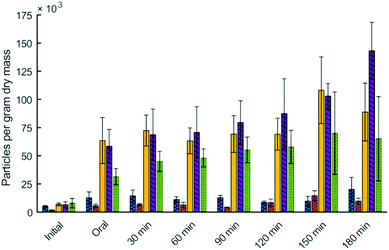 |
| Fig. 3 Particles per gram dry matter of for digesta of small wheat couscous ( ), commercial wheat couscous ( ), small millet couscous ( ), large millet couscous ( ), and commercial millet couscous ( ) during vitro gastric digestion (initial = no digestion; oral = 30 s simulated oral digestion; 30–180 min = corresponding simulated gastric digestion time). Error bars represent standard deviation of either three or four digestion in the HGS. | |
Starch hydrolysis in the HGS
Reducing sugar content in the HGS was measured in the liquid phase of centrifuged digesta over the course of the simulated digestion and the percent of starch hydrolysis was divided by the total area of particles at the same time point; the resulting values were expressed as percent starch hydrolysis per unit area of digested particles. Expressing this data on a unit area basis was necessary due to the differences in surface area resulting from particle breakdown during simulated digestion. The percent starch hydrolysis values (without being expressed per unit area) are included in Table S1.† Starch hydrolysis per unit area in the HGS was significantly influenced by digestion time (p < 0.01) and type of couscous (p < 0.01), but not their interaction (p > 0.05) (Fig. 4). There was a significant increase in percent starch hydrolysis in the total digestion time per unit area of digested particles for all types of couscous (p < 0.05), except for small millet couscous (p > 0.05). At the final time point of the simulated gastric digestion (180 min), percent starch hydrolysis per unit area for large millet couscous (16.8 ± 5.0 × 10−3 % mm−2) and for small millet couscous (11.8 ± 1.9 × 10−3 % mm−2) were significantly lower (p < 0.05) than for commercial wheat couscous (26.3 ± 3.9 × 10−3 % mm−2).
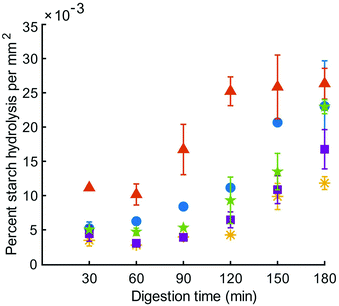 |
| Fig. 4 Percent starch hydrolysis per unit area of particles in the HGS for small wheat couscous ( ), commercial wheat couscous ( ), small millet couscous ( ), large millet couscous ( ), and commercial millet couscous ( ). Error bars represent the standard error of three runs except for millet small (four runs). | |
Moisture content
Moisture content (dry basis) was significantly influenced by digestion time (p < 0.01), but not by type of couscous (p > 0.05) (Table 4). Moisture content of digesta was not significantly different between the five types of couscous from oral phase up to 150 min of simulated gastric digestion. Moisture content of digesta after 180 min ranged from 4.09 ± 0.58 g moisture per g dry mass (wheat small) to 7.62 ± 1.79 (millet small) g moisture per g dry mass.
Table 4 Moisture content (dry basis, g water per g dry mass) and pH of digesta withdrawn from the HGS at different time points and for different types of couscous. All values are means of multiple runs in the HGS (n = 3 runs, except for millet small which was n = 4) ± standard deviation. Values in each column that do not share a letter (abc) represent significant differences (p < 0.05) within a certain type of couscous across different digestion times. Values in each row that do not share a letter (zyx) represent significant differences (p < 0.05) within a certain time point across different types of couscous. If no letter is shown, there were no statistically significant differences
|
Moisture content (g moisture per g dry mass) |
pH |
Digestion time (min) |
Wheat small |
Wheat commercial |
Millet small |
Millet large |
Millet commercial |
Wheat small |
Wheat commercial |
Millet small |
Millet large |
Millet commercial |
0.5 |
0.84 ± 0.09b |
0.82 ± 0.03b |
0.80 ± 0.09b |
0.90 ± 0.10c |
0.89 ± 0.05c |
6.38 ± 0.51a |
6.44 ± 0.32a |
6.29 ± 0.09a |
6.48 ± 0.05a |
5.83 ± 0.17a |
30 |
2.66 ± 0.35ab |
2.93 ± 0.22ab |
1.80 ± 0.04b |
1.81 ± 0.04c |
1.86 ± 0.08c |
4.88 ± 0.17bc |
5.01 ± 0.15bc |
5.35 ± 0.13b |
5.23 ± 0.09bc |
4.98 ± 0.06ab |
60 |
2.36 ± 0.11ab |
2.83 ± 0.23ab |
1.70 ± 0.06b |
1.68 ± 0.05c |
1.74 ± 0.12c |
5.36 ± 0.16b |
5.14 ± 0.26b |
5.63 ± 0.10ab |
5.63 ± 0.11ab |
5.29 ± 0.07ab |
90 |
2.51 ± 0.18ab |
3.01 ± 0.09ab |
1.93 ± 0.60b |
1.76 ± 0.11c |
1.66 ± 0.07c |
5.30 ± 0.43b |
4.92 ± 0.32bc |
5.63 ± 0.24ab |
5.62 ± 0.06ab |
5.36 ± 0.10a |
120 |
2.92 ± 0.12ab |
3.45 ± 0.10ab |
2.26 ± 0.45b |
2.03 ± 0.20c |
2.41 ± 1.08c |
4.76 ± 0.80bcd |
4.58 ± 0.19bcd |
5.37 ± 0.22b |
5.43 ± 0.14bc |
4.96 ± 0.48ab |
150 |
3.61 ± 0.21ab |
4.21 ± 0.11a |
5.15 ± 1.83a |
3.24 ± 0.44c |
3.21 ± 0.31bc |
4.69 ± 0.38bcd |
4.18 ± 0.11cd |
3.88 ± 0.94c |
4.49 ± 0.41c |
4.37 ± 0.24b |
180 |
4.09 ± 0.58a,y |
5.16 ± 0.29a,zy |
7.62 ± 1.79a,z |
7.53 ± 1.82b,z |
6.14 ± 1.53ab,zy |
4.21 ± 0.25cd |
3.84 ± 0.25d |
2.91 ± 0.32d |
2.87 ± 0.39d |
3.24 ± 0.32c |
pH
pH of digesta was significantly influenced by digestion time (p < 0.01), but not by type of couscous (p > 0.05) (Table 4). The pH of digesta decreased throughout the simulated digestion due to the secretion of additional gastric juice. For example, the pH of small millet couscous was 6.29 ± 0.09 after 30 s of simulated oral phase and decreased to 2.91 ± 0.32 after 180 min simulated gastric digestion.
Relative gastric emptying of solids from the HGS
Relative gastric emptying of solids in the HGS was fit to eqn (2), and results are shown in Fig. 5. The model provided a good fit to the data as evidenced by high coefficient of determination (R2 minimum = 0.997) (Table 5). The emptying rate parameter, k (min−1), was significantly influenced by couscous type (p < 0.05). Large millet couscous had a significantly higher k value than commercial wheat couscous (p < 0.05). The extrapolated y-intercept from the terminal portion of the curve, β, was not significantly influenced by type of couscous (p > 0.05). The half-emptying time t1/2 (min) was significantly influenced by type of couscous (p < 0.01). The highest half-emptying times were for commercial wheat couscous and small wheat couscous (240 min and 189 min, respectively). This reflects the slower emptying of solids from the HGS during simulated gastric digestions of commercial wheat couscous and small wheat couscous. It is important to note that these results indicate the emptying of solids as the overall gastric emptying rate of digesta was held constant at 3 mL min−1 for all types of couscous. The more rapid emptying of solids from millet couscous coincided with a greater degree of breakdown as described in the previous section, indicating that the HGS measures gastric emptying based on particle breakdown.
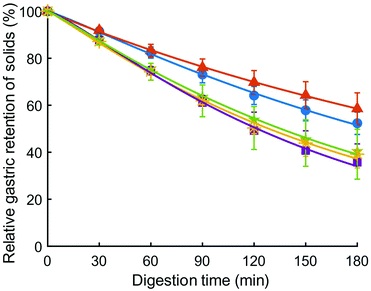 |
| Fig. 5 Relative solids remaining in the HGS (%), representative of relative gastric emptying, for small wheat couscous ( ), commercial wheat couscous ( ), small millet couscous ( ), large millet couscous ( ), and commercial millet couscous ( ). Error bars represent the standard deviation of three digestions except for small millet couscous (four digestions). Lines represent the model fit (eqn (2)). | |
Table 5 Parameters of the modified power-exponential model (eqn (2) and (3)) for gastric emptying. Values in each column that do not share a letter (abc) represent significant differences (p < 0.05) between different types of couscous
|
k × 103 (min−1) |
β
|
t
1/2 (min) |
R
2
|
Wheat small |
4.20 ± 0.59ab |
1.14 ± 0.07 |
189 ± 25ab |
0.999 ± 0.00 |
Wheat commercial |
2.97 ± 0.86bc |
0.99 ± 0.07 |
240 ± 49a |
0.999 ± 0.00 |
Millet small |
5.28 ± 2.12ab |
1.06 ± 0.19 |
146 ± 29bc |
0.997 ± 0.003 |
Millet large |
7.03 ± 0.60a |
1.25 ± 0.06 |
122 ± 6bc |
0.998 ± 0.001 |
Millet commercial |
5.76 ± 1.09ab |
1.13 ± 0.15 |
137 ± 10bc |
0.998 ± 0.001 |
Discussion
In this study, the Human Gastric Simulator was used to quantify the physical and chemical breakdown of couscous samples during simulated gastric digestion. Previously, other starch-based carbohydrates, such as white and brown rice, have been studied for their physicochemical breakdown properties during gastric digestion.6,37,38 Millet couscous and other products made from millet have previously shown slow gastric emptying and digestion properties in vivo3,39 and in vitro,40,41 yet there is a lack of knowledge of the basis of this response. The objective of this work was to determine how pearl millet couscous breaks down in a simulated gastric environment and quantify the starch hydrolysis of the gastric breakdown products.
Initial properties of couscous samples and flour (for self-made couscous) were characterized by RVA analysis and HPSEC. RVA analysis is a rheological method to characterize suspensions containing starch and water during heating and cooling.42 Notable factors with impact on peak viscosity include the extent of amylose leaching, amylose-lipid complex formation, starch granule swelling, friction between swollen granules, and competition for free water between leached amylose and remaining ungelatinized granules.43,44 For the current study, lower water amount was used for couscous samples than for flour samples (25 mL for flours, 15 mL for couscous), because couscous samples were pregelatinized and thus did not absorb water and swell like the intact starch granules in flours. Furthermore, millet couscous did not show a cold swelling property, which could be indicative of either immediate breakdown in the RVA or incomplete gelatinization of starch in millet couscous after the initial 14 min steaming period of preparation. However, it is important to note that for simulated digestions, all couscous samples were steamed an additional 10 min immediately prior to experimentation. Final viscosities from RVA results were compared due to relevance to foods prepared for consumption. Previous researchers found final viscosity to be the most sensitive measurement of intermolecular interactions in maize starch.45 Results indicated that final viscosity was higher for millet flour than for wheat flour (3887 ± 11 vs. 2761 ± 28 cP, p < 0.01). Given its higher viscosity, starch granules in millet flour exhibited greater amylose retrogradation than those in wheat flour.45 No significant amylose-lipid complex formation was evident for any of the samples as determined by differential scanning calorimetry (data not shown), so this would not have impacted the RVA profiles. In contrast to flour samples, wheat couscous exhibited higher final viscosity than millet couscous (8206 ± 116 vs. 5793 ± 22 cP, p < 0.01), indicating higher breakdown of millet couscous to smaller particles. Accordingly, millet couscous appeared paste-like, whereas wheat couscous particles remained intact.
HPSEC was used to determine starch fine structural features, as it was hypothesized that they could help explain the slower starch hydrolysis rate that was found for millet samples. Millet samples had smaller amylose chain length (839–963 DP) than wheat samples (1225–1563 DP; p < 0.05; Table 2). Amylose of intermediate chain length (667 DP) was previously shown to exhibit a higher propensity to form intermolecular interactions.46 Although the amylose chain lengths measured for millet samples were somewhat larger than 667 DP, it can be hypothesized that they also could exhibit increased intermolecular interactions, causing denser matrices that impede starch hydrolysis.47 To the authors’ knowledge, starch fine structural characteristics have not been previously elucidated for the particular type of pearl millet studied (grown in Senegal), however, the characteristics for wheat are similar to those found by Martinez et al.48
Along with initial property measurements of couscous, the particle size of couscous in digesta emptied from the HGS was assessed. It was found that all types of couscous experienced a statistically significant reduction in particle size throughout simulated digestion, however, breakdown of millet couscous was substantial and significantly (p < 0.05) higher than that of wheat couscous. For example, after 180 min simulated gastric digestion, large millet couscous particles had a median size of 0.17 mm2, whereas commercial wheat couscous particles had a median size of 1.06 mm2 (p < 0.05). A range of diameters of 0.37 to 0.59 mm was found for small, large, and commercial millet couscous after back-calculation from the obtained particle areas, assuming 2D circles, from 60 to 180 min in the HGS. This was similar to the initial millet cell diameter estimated from the light micrographs to be about 0.4 mm, suggesting that the simulator reduced the size of the millet couscous to that of individual cells. Additionally, the differences between breakdown of millet couscous and wheat couscous may be due to the presence of gluten in wheat,49 which acts to provide structure in many gluten-containing foods, and may help wheat-based foods resist breakdown. Previous research has shown a decrease in particle size of white and brown rice during gastric processing in vitro50 and in vivo.4 To the authors’ knowledge, the particle breakdown of couscous has not previously been studied in vitro or in vivo.
Starch hydrolysis per unit area of digested particles increased over time for all types of couscous (p < 0.05). To control for increases in surface area that occurred due to particle breakdown in the HGS, starch digestion was reported as percent starch hydrolysis per unit area of digested particles. Percent starch hydrolysis per unit area at the final time point in digestion was significantly lower (p < 0.05) for large millet couscous (16.8 ± 5.0 × 10−3 % mm−2) and small millet couscous (11.8 ± 1.9 × 10−3 % mm−2) than for commercial wheat couscous (26.3 ± 3.9 × 10−3 % mm−2). Interestingly, starch hydrolysis per unit area increased by only 4% in the final hour of simulated gastric digestion for commercial wheat couscous, whereas there was an 88% increase over the same time period for large millet couscous and 85% increase for commercial millet couscous. This suggests that the millet starch became more susceptible to hydrolysis in the last hour of digestion, whereas the wheat couscous starch was fully accessible by the end of the second hour of digestion. This finding suggests that changes to millet couscous resulting in an increased rate of starch hydrolysis occur only after two hours of simulated digestion. Previous researchers have found that millet has a slow starch hydrolysis property,39–41 lending support for the current results. Since pH profiles were not significantly different among the types of couscous, differences in hydrolysis between different types of couscous cannot be attributed to differences in residual salivary α-amylase activity during simulated gastric digestion.
Differences in starch hydrolysis could have been due to retention of intact cells in millet couscous after breakdown as well as differences in chemical and/or structural composition of the couscous. The integrity of cell wall structure is a factor that could influence starch hydrolysis. Previous researchers have shown using wheat and sorghum that intact cells slow enzymatic hydrolysis of the inside starch due to hindered transport of enzymes through the cell walls.52,53 Given that the millet couscous particle sizes obtained from 60 to 180 min of simulated digestion were in a similar size range as the initial intact cell, it seems plausible that the lower millet hydrolysis was affected by cell walls. However, cell wall structures were also observed in wheat flour particles that could affect digestion. It is possible that millet cell wall structures are more rigid than wheat, thus impeding starch hydrolysis more. Another possibility is that intermediate amylose chain lengths (841–970 DP) for millet samples could have allowed for increased intermolecular interactions46 which might have promoted the formation of denser matrices that are more resistant to hydrolysis.47 Previous researchers have proposed that microstructural and starch granule features of starchy foods can influence starch hydrolysis.51
pH of the digesta decreased over time for all types of couscous due to the secretion of gastric juice during simulated digestion. Due to the constant secretion rate of gastric juice, the lack of significant differences in pH between the different couscous samples indicates that they had similar buffering capacity. The relatively high pH values (highest value among treatments at 30 min = 6.48; highest value at 180 min = 4.21) throughout gastric digestion create an environment where activity of salivary α-amylase could be partially retained.
Relative gastric emptying of solids was greater for millet couscous than wheat couscous, as analyzed by calculating the percentage of initial dry matter that remained in the HGS at each time point. Since the overall emptying rate was held constant at 3 mL min−1, differences in relative gastric emptying of solids reflected the solids content of samples withdrawn from the HGS and the relative breakdown of particles that occurred. It is hypothesized that the more rapid breakdown of millet couscous in comparison to wheat couscous led to increased packing density in the antral region of the simulated stomach chamber, allowing a greater amount of solids from millet couscous to be emptied at each time point. Curves representing relative emptying of solids were fit to a modified power-exponential model (eqn (2) and (3)) which was used to describe the emptying profile in terms of a kinetic value, k (min−1), an extrapolated y intercept, β, and an emptying half-time, t1/2 (min). It was found that large millet couscous emptied the most rapidly, with k value of 7.03 min−1 and t1/2 of 122 min. Commercial wheat couscous emptied more slowly than large millet couscous, with k value of 2.97 min−1 and t1/2 of 146 min (p < 0.05). Gastric emptying half-times in this study (122–240 min) were comparable in magnitude to those reported by previous researchers for in vivo gastric emptying of brown and white rice meals using the growing pig as a model for the adult human (229 and 227 min, respectively).5 In our recent human study conducted in Mali, we found that millet couscous had very slow gastric half-emptying time (5.3 h).3 It appears that the discrepancy between gastric emptying times of millet couscous measured in vivo and in the HGS reflects that gastric emptying in vivo is affected by factors other than particle breakdown solely. In the HGS, millet couscous broke down into smaller, more numerous particles than wheat couscous and had a more rapid gastric emptying rate of solids. However, physiological feedback and control mechanisms of gastric emptying in vivo are not reproduced by current in vitro models. Thus, the results of this study lend support to the hypothesis that physiological controls of gastric emptying may better explain the slow gastric emptying of millet couscous in vivo, and that hydrolysis-resistant particles of millet couscous reaching the distal small intestine may activate the ileal brake. The ileal brake mechanism has been shown to delay gastric emptying rate in a dose-dependent manner54 and when administered as a preload.55 Of note, higher delay in gastric emptying has been related to greater length of small intestine exposure to glucose.56 In this study, percent starch hydrolysis of couscous was expressed per unit surface area of particles, and demonstrated that increased surface area by greater particle breakdown of millet couscous still did not increase starch hydrolysis. Thus, millet particles exiting the stomach could reach the distal small intestine, potentially activating the ileal brake and contributing to the long gastric emptying times for these foods.3 It is also possible that millet couscous, which broke down into smaller particles, could have experienced strong particle–particle interactions, forming a viscous paste in the stomach that slowed gastric emptying in the human study, but was not measured in the HGS. Meals higher in viscosity have been shown to delay gastric emptying rate in vivo.57,58 An area of future research is the measurement of viscosity of foods in the stomach as they undergo breakdown. This study indicates there are future opportunities to understand the mechanisms of gastric emptying and the means by which it is controlled by the body, which could be investigated using a combination of in vivo and in vitro methods.
Conclusions
In this study, three types of millet couscous and two types of wheat couscous were digested using the Human Gastric Simulator, a device which simulates the peristaltic motion, continuous secretion of gastric juice, and intermittent emptying of the human stomach. It was found that millet couscous broke down into smaller and more numerous particles than wheat couscous. Millet couscous samples also showed lower percent starch hydrolysis per unit area of digested particles. It is hypothesized that the slow gastric emptying rate of millet couscous observed in humans may be explained by slow hydrolysis in the small intestines that activates the ileal brake mechanism. It was suggested that densely packed starch matrices with intact cell wall structure exist in gastric processed millet couscous particles that are slowly digesting and reach the ileum. This study provides new understanding of the slow gastric emptying of millet couscous, which could provide strategies to make processed foods with this quality for appetite control and extended nutrient (energy) delivery to the body after food consumption.
Conflicts of interest
There are no conflicts to declare.
Acknowledgements
This research was made possible in part by the support of the American People provided to the Feed the Future Innovation Lab for Collaborative Research on Sorghum and Millet through the United States Agency for International Development (USAID). The contents are the sole responsibility of the authors and do not necessarily reflect the views of USAID or the United States Government. Program activities are funded by USAID under Cooperative Agreement No. AID-OAA-A-13-00047. This research was also supported by the Whistler Center for Carbohydrate Research.
References
- H. Halawi, M. Camilleri, A. Acosta, M. Vazquez-Roque, I. Oduyebo, D. Burton, I. Busciglio and A. R. Zinsmeister, Relationship of gastric emptying or accommodation with satiation, satiety, and postprandial symptoms in health, Am. J. Physiol.: Gastrointest. Liver Physiol., 2017, 313, G442–G447 CrossRef PubMed.
- P. M. Hellström and E. Näslund, Interactions between gastric emptying and satiety, with special reference to glucagon-like peptide-1, Physiol. Behav., 2001, 74, 735–741 CrossRef.
- F. Cisse, D. P. Erickson, A. M. R. Hayes, A. R. Opekun, B. L. Nichols and B. R. Hamaker, Traditional malian solid foods made from sorghum and millet have markedly slower gastric emptying than rice, potato, or pasta, Nutrients, 2018, 10, 124 CrossRef PubMed.
- G. M. Bornhorst, K. Kostlan and R. P. Singh, Particle size distribution of brown and white rice during gastric digestion measured by image analysis, J. Food Sci., 2013, 78, E1383–E1391 CrossRef CAS.
- G. M. Bornhorst, L. Q. Chang, S. M. Rutherfurd, P. J. Moughan and R. P. Singh, Gastric emptying rate and chyme characteristics for cooked brown and white rice meals in vivo, J. Sci. Food Agric., 2013, 93, 2900–2908 CrossRef CAS PubMed.
- G. M. Bornhorst, N. Ströbinger, S. M. Rutherfurd, R. P. Singh and P. J. Moughan, Properties of gastric chyme from pigs fed cooked brown or white rice, Food Biophys., 2013, 8, 12–23 CrossRef.
- G. M. Bornhorst, M. J. Roman, S. M. Rutherfurd, B. J. Burri, P. J. Moughan and R. P. Singh, Gastric digestion of raw and roasted almonds in vivo, J. Food Sci., 2013, 78, H1807–H1813 CrossRef CAS PubMed.
- A. Fardet, A shift toward a new holistic paradigm will help to preserve and better process grain products’ food structure for improving their health effects, Food Funct., 2015, 6, 363–382 RSC.
- R. Gopirajah, K. P. Raichurkar, R. Wadhwa and C. Anandharamakrishnan, The glycemic response to fibre rich foods and their relationship with gastric emptying and motor functions: An MRI study, Food Funct., 2016, 7, 3964–3972 RSC.
- G. Zhang, L. Y. Hasek, B.-H. Lee and B. R. Hamaker, Gut feedback mechanisms and food intake: a physiological approach to slow carbohydrate bioavailability, Food Funct., 2015, 6, 1072–1089 RSC.
- S. Gallier, S. M. Rutherfurd, P. J. Moughan and H. Singh, Effect of food matrix microstructure on stomach emptying rate and apparent ileal fatty acid digestibility of almond lipids, Food Funct., 2014, 5, 2410–2419 RSC.
- Q. Guo, A. Ye, M. Lad, M. Ferrua, D. Dalgleish and H. Singh, Disintegration kinetics of food gels during gastric digestion and its role on gastric emptying: An in vitro analysis, Food Funct., 2015, 6, 756–764 RSC.
- L. Marciani, P. A. Gowland, A. Fillery-Travis, P. Manoj, J. Wright, A. Smith, P. Young, R. Moore and R. C. Spiller, Assessment of antral grinding of a model solid meal with echo-planar imaging, Am. J. Physiol.: Gastrointest. Liver Physiol., 2001, 280, G844–G849 CrossRef CAS PubMed.
- L. Y. Hasek, R. J. Phillips, G. Zhang, K. P. Kinzig, C. Y. Kim, T. L. Powley and B. R. Hamaker, Dietary slowly digestible starch triggers the gut-brain axis in obese rats with accompanied reduced food intake, Mol. Nutr. Food Res., 2018, 62, 1700117 CrossRef PubMed.
- S. D. Poppitt, H. S. Shin, A. T. McGill, S. C. Budgett, K. Lo, M. Pahl, J. Duxfield, M. Lane and J. R. Ingram, Duodenal and ileal glucose infusions differentially alter gastrointestinal peptides, appetite response, and food intake: A tube feeding study, Am. J. Clin. Nutr., 2017, 106, 725–735 CAS.
- J. Schirra, M. Katschinski, C. Weidmann, T. Schäfer, U. Wank, R. Arnold and B. Göke, Gastric emptying and release of incretin hormones after glucose ingestion in humans, J. Clin. Invest., 1996, 97, 92–103 CrossRef CAS PubMed.
- L. Roman, M. Gomez, C. Li, B. R. Hamaker and M. M. Martinez, Biophysical features of cereal endosperm that decrease starch digestibility, Carbohydr. Polym., 2017, 165, 180–188 CrossRef CAS PubMed.
- G. M. Bornhorst and R. P. Singh, Kinetics of in vitro bread bolus digestion with varying oral and gastric digestion parameters, Food Biophys., 2013, 8, 50–59 CrossRef.
- D. A. Mackie and R. M. Pangborn, Mastication and its influence on human salivary flow and alpha-amylase secretion I, Physiol. Behav., 1990, 47, 593–595 CrossRef CAS.
- Y. A. Mennah-Govela, G. M. Bornhorst and R. P. Singh, Acid diffusion into rice boluses is influenced by rice type, variety, and presence of α-amylase, J. Food Sci., 2015, 80, E316–E325 CrossRef CAS PubMed.
- M. Minekus, M. Alminger, P. Alvito, S. Ballance, T. Bohn, C. Bourlieu, F. Carrière, R. Boutrou, M. Corredig, D. Dupont, C. Dufour, L. Egger, M. Golding, S. Karakaya, B. Kirkhus, S. Le Feunteun, U. Lesmes, A. Macierzanka, A. Mackie, S. Marze, D. J. McClements, O. Ménard, I. Recio, C. N. Santos, R. P. Singh, G. E. Vegarud, M. S. J. Wickham, W. Weitschies and A. Brodkorb, A standardised static in vitro digestion method suitable for food - an international consensus, Food Funct., 2014, 5, 1113–1124 RSC.
- E. Capuano, N. Pellegrini, E. Ntone and C. V. Nikiforidis, In vitro lipid digestion in raw and roasted hazelnut particles and oil bodies, Food Funct., 2018, 9, 2508–2516 RSC.
- L. Sams, J. Paume, J. Giallo and F. Carrière, Relevant pH and lipase for in vitro models of gastric digestion, Food Funct., 2016, 7, 30–45 RSC.
- E. B. Ozvural and G. M. Bornhorst, Chemical and structural characteristics of frankfurters during in vitro gastric digestion as influenced by cooking method and severity, J. Food Eng., 2018, 229, 102–108 CrossRef CAS.
- D. Dupont, M. Alric, S. Blanquet-Diot, G. Bornhorst, C. Cueva, A. Deglaire, S. Denis, M. Ferrua, R. Havenaar, J. Lelieveld, A. R. Mackie, M. Marzorati, O. Menard, M. Minekus, B. Miralles, I. Recio and P. Van den Abbeele, Can dynamic in vitro digestion systems mimic the physiological reality?, Crit. Rev. Food Sci. Nutr., 2019, 59, 1546–1562 CrossRef CAS PubMed.
-
D. M. Phinney, Design, construction, and evaluation of a reactor designed to mimic human gastric digestion, University of California, Davis, 2013 Search PubMed.
- M. Hocke, U. Schöne, H. Richert, P. Görnert, J. Keller, P. Layer and A. Stallmach, Every slow-wave impulse is associated with motor activity of the human stomach, Am. J. Physiol.: Gastrointest. Liver Physiol., 2009, 296, G709–G716 CrossRef CAS PubMed.
- C. Swackhamer, Z. Zhang, A. Y. Taha and G. M. Bornhorst, Fatty acid bioaccessibility and structural breakdown from in vitro digestion of almond particles, Food Funct., 2019, 10, 5174–5187 RSC.
- Q. Guo, A. Ye, M. Lad, D. Dalgleish and H. Singh, Effect of gel structure on the gastric digestion of whey protein emulsion gels, Soft Matter, 2014, 10, 1214–1223 RSC.
- S. K. Gebauer, J. A. Novotny, G. M. Bornhorst and D. J. Baer, Food processing and structure impact the metabolizable energy of almonds, Food Funct., 2016, 7, 4231–4238 RSC.
- P. Rosin and E. Rammler, The laws governing the fineness of powdered coal, J. Inst. Fuel, 1933, 7, 29–36 CAS.
- L. Hedjazi, S. Guessasma, C. Yven, G. Della Valle and C. Salles, Preliminary analysis of mastication dynamics and fragmentation during chewing of brittle cereal foods, Food Res. Int., 2013, 54, 1455–1462 CrossRef.
- G. L. Miller, Use of dinitrosalicylic acid reagent for determination of reducing sugar, Anal. Chem., 1959, 31, 426–428 CrossRef CAS.
- V. Ratanpaul, B. A. Williams, J. L. Black and M. J. Gidley, Apparent amylase diffusion rates in milled cereal grains determined in vitro: potential relevance to digestion in the small intestine of pigs, J. Cereal Sci., 2018, 82, 42–48 CrossRef CAS.
- J. A. Siegel, J. L. Urbain, L. P. Adler, N. D. Charkes, A. H. Maurer, B. Krevsky, L. C. Knight, R. S. Fisher and L. S. Malmud, Biphasic nature of gastric emptying, Gut, 1988, 29, 85–89 CrossRef CAS PubMed.
- J. L. C. Urbain, J. A. Siegel, N. D. Charkes, A. H. Maurer, L. S. Malmud and R. S. Fisher, The two-component stomach: effects of meal particle size on fundal and antral emptying, Eur. J. Nucl. Med., 1989, 15, 254–259 CrossRef CAS PubMed.
- G. M. Bornhorst, M. J. Ferrua, S. M. Rutherfurd, D. R. Heldman and R. P. Singh, Rheological properties and textural attributes of cooked brown and white rice during gastric digestion in vivo, Food Biophys., 2013, 8, 137–150 CrossRef.
- K. C. Drechsler and G. M. Bornhorst, Modeling the softening of carbohydrate-based foods during simulated gastric digestion, J. Food Eng., 2018, 222, 38–48 CrossRef CAS.
- J. Alyami, N. Ladd, S. E. Pritchard, C. L. Hoad, A. A. Sultan, R. C. Spiller, P. A. Gowland, I. A. Macdonald, G. P. Aithal, L. Marciani and M. A. Taylor, Glycaemic, gastrointestinal and appetite responses to breakfast porridges from ancient cereal grains: A MRI pilot study in healthy humans, Food Res. Int., 2019, 118, 49–57 CrossRef CAS PubMed.
- K. S. Sandhu and A. K. Siroha, Relationships between physicochemical, thermal, rheological and in vitro digestibility properties of starches from pearl millet cultivars, LWT–Food Sci. Technol., 2017, 83, 213–224 CrossRef CAS.
- G. A. Annor, M. Marcone, M. Corredig, E. Bertoft and K. Seetharaman, Effects of the amount and type of fatty acids present in millets on their in vitro starch digestibility and expected glycemic index (eGI), J. Cereal Sci., 2015, 64, 76–81 CrossRef CAS.
- C. W. Walker, A. S. Ross, C. W. Wrigley and G. J. McMaster, Accelerated starch-paste characterization with the Rapid Visco-Analyzer, Cereal Foods World, 1988, 33, 491–494 CAS.
- H. D. Almeida-Dominguez, E. L. Suhendro and L. W. Rooney, Factors affecting Rapid Visco Analyser curves for the determination of maize kernel hardness, J. Cereal Sci., 1997, 25, 93–102 CrossRef.
- M. A. Fitzgerald, M. Martin, R. M. Ward, W. D. Park and H. J. Shead, Viscosity of rice flour: A rheological and biological study, J. Agric. Food Chem., 2003, 51, 2295–2299 CrossRef CAS PubMed.
- R. Juhász and A. Salgó, Pasting behavior of amylose, amylopectin and their mixtures as determined by RVA curves and first derivatives, Starch/Stärke, 2008, 60, 70–78 CrossRef.
- J.-L. Jane and J.-F. Chen, Effect of amylose molecular size and amylopectin branch chain length on paste properties of starch, Cereal Chem., 1992, 69, 60–65 CAS.
- B. Zhang, S. Dhital and M. J. Gidley, Densely packed matrices as rate determining features in starch hydrolysis, Trends Food Sci. Technol., 2015, 43, 18–31 CrossRef CAS.
- M. M. Martinez, C. Li, M. Okoniewska, I. Mukherjee, D. Vellucci and B. Hamaker, Slowly digestible starch in fully gelatinized material is structurally driven by molecular size and A and B1 chain
lengths, Carbohydr. Polym., 2018, 197, 531–539 CrossRef CAS.
- J. A. Delcour, I. J. Joye, B. Pareyt, E. Wilderjans, K. Brijs and B. Lagrain, Wheat gluten functionality as a quality determinant in cereal-based food products, Annu. Rev. Food Sci. Technol., 2012, 3, 469–492 CrossRef CAS.
- F. Kong and R. P. Singh, A Human Gastric Simulator (HGS) to study food digestion in human stomach, J. Food Sci., 2010, 75, E627–E635 CrossRef CAS PubMed.
- L. Roman, M. Sahagun, M. Gomez and M. M. Martinez, Nutritional and physical characterization of sugar-snap cookies: effect of banana starch in native and molten states, Food Funct., 2019, 10, 616–624 RSC.
- R. R. Bhattarai, S. Dhital, A. Mense, M. J. Gidley and Y. C. Shi, Intact cellular structure in cereal endosperm limits starch digestion in vitro, Food Hydrocolloids, 2018, 81, 139–148 CrossRef CAS.
- K. Korompokis, N. De Brier and J. A. Delcour, Differences in endosperm cell wall integrity in wheat (Triticum aestivum L.) milling fractions impact on the way starch responds to gelatinization and pasting treatments and its subsequent enzymatic in vitro digestibility, Food Funct., 2019, 10, 4674–4684 RSC.
- L. J. Miller, J. R. Malagelada, W. F. Taylor and V. L. Go, Intestinal control of human postprandial gastric function: the role of components of jejunoileal chyme in regulating gastric secretion and gastric emptying, Gastroenterology, 1981, 80, 763–769 CrossRef CAS.
- F. Cisse, E. A. Pletsch, D. P. Erickson, M. Chegeni, A. M. R. Hayes and B. R. Hamaker, Preload of slowly digestible carbohydrate microspheres decreases gastric emptying rate of subsequent meal in humans, Nutr. Res., 2017, 45, 46–51 CrossRef CAS.
- H. C. Lin, J. E. Doty, T. J. Reedy and J. H. Meyer, Inhibition of gastric emptying by glucose depends on length of intestine exposed to nutrient, Am. J. Physiol., 1989, 256, G404–G411 CAS.
- L. Marciani, P. A. Gowland, R. C. Spiller, P. Manoj, R. J. Moore, P. Young and A. J. Fillery-Travis, Effect of meal viscosity and nutrients on satiety, intragastric dilution, and emptying assessed by MRI, Am. J. Physiol.: Gastrointest. Liver Physiol., 2001, 280, G1227–G1233 CrossRef CAS PubMed.
- L. Marciani, P. A. Gowland, R. C. Spiller, P. Manoj, R. J. Moore, P. Young, S. Al-Sahab, D. Bush, J. Wright and A. J. Fillery-Travis, Gastric response to increased meal viscosity assessed by echo-planar magnetic resonance imaging in humans, J. Nutr., 2000, 130, 122–127 CrossRef CAS PubMed.
Footnote |
† Electronic supplementary information (ESI) available: Table S1 and Fig. S1. See DOI: 10.1039/c9fo01461f |
|
This journal is © The Royal Society of Chemistry 2020 |
Click here to see how this site uses Cookies. View our privacy policy here.