DOI:
10.1039/C9FO01230C
(Paper)
Food Funct., 2020,
11, 1110-1121
Anti-tumor and immunomodulatory activity of the aqueous extract of Sarcodon imbricatus in vitro and in vivo
Received
10th June 2019
, Accepted 4th November 2019
First published on 11th December 2019
Abstract
Sarcodon imbricatus (S. imbricatus), a well-known edible mushroom, is one of the most commonly consumed wild mushrooms in China because of its nutritional value. Previous studies have demonstrated that S. imbricatus has immunoregulatory activity. We previously described the potential anti-tumor activity of several types of mushrooms, including S. imbricatus. In this study, the results demonstrate that an aqueous extract of S. imbricatus (SIE) effectively inhibits the growth, migration, and invasion properties of breast cancer cells in vitro and reduces tumor growth in vivo. In addition, the SIE increased serum concentrations of interleukin (IL)-2, IL-6 and tumor necrosis factor-α, natural killer cell activity and the viability of splenocytes and reduced the expression of programmed cell death-Ligand 1 (PD-L1) in 4T1 tumor-bearing mice. Collectively, these results are the first demonstration that the SIE has anti-tumor and immunomodulatory effects in the 4T1 mouse breast cancer model. These findings provide a scientific rationale for the potential therapeutic use of S. imbricatus in breast cancer patients.
1. Introduction
Breast cancer is the most common primary malignancy and the second most common cause of cancer deaths in females.1,2 There were an estimated 2 million new cases and 630
000 deaths from breast cancer every year.3 Despite improvements in early detection and treatment4, breast cancer remains a public health crisis worldwide, due in large part to its aggressive nature and resistance to conventional therapies such as chemotherapy and radiotherapy5. Therefore, there is a pressing need to identify and develop more effective therapeutic agents.
In recent years, mushrooms have attracted attention not only due to their nutritional value6 but also as a potential source of therapeutic compounds for a variety of diseases7, including cancer8. Sarcodon imbricatus (L. Fr.) Karst (S. imbricatus), also locally known as Hei Hu Zhang, is one of the most common edible mushrooms in China. Studies in recent years have found that S. imbricatus has anti-oxidant and immunomodulatory functions. It has a high ratio (3.03
:
1) of unsaturated to saturated fatty acids, and the free radical scavenging rate for 2,2-diphenyl-1-picrylhydrazyl is 81.25%, with a half-maximal inhibitory concentration of 0.054 mg mL−1.9 Methanol extracts of S. imbricatus show anti-oxidant activity, reducing power, metal chelating ability, inhibition of linoleic acid peroxidation, and superoxide, peroxide and hydrogen peroxide scavenging effects.10,11 Furthermore, S. imbricatus extracts have been shown to inhibit the formation of bacterial biofilms12 and enhance the immunity of cyclophosphamide-immunosuppressed mice by neutralizing oxidative stress and increasing serum concentrations of immunoglobulin and other factors.13
Natural killer (NK) cells are prototype innate lymphoid cells endowed with potent cytolytic function that provide host defence against microbial infection and tumours. Current checkpoint blockade agents used to activate T cells in cancer patients, such as antibodies against programmed cell death 1 (PD-1), may also target NK cells. Checkpoint blockade targeting inhibitory receptors shared by NK cells and T cells are currently under clinical evaluation.14 Increased programmed cell death-Ligand 1 (PD-L1) expression in tumor cells led to decreased T cell proliferation and increased apoptosis. So far, immunotherapy targeting PD-1/PD-L1 has been on the way to clinical trials for breast cancer.15
Previous experiments have shown that S. imbricatus aqueous extract (SIE) possessed anti-tumor activity in vitro.16 In the present study, we sought to extend those findings by investigating the effects of the SIE on breast cancer cells in vitro and in vivo. It was found that the SIE effectively inhibited the proliferation of breast cancer cells in vitro and the growth of tumors in vivo by regulating immunity and reducing the expression of PD-L1 in tumors.
2. Materials and methods
2.1. Preparation and analysis of extracts
The dried fruiting bodies of S. imbricatus (Fig. 1) were provided by Guangdong Yuewei Edible Fungi Technology Co., Ltd (Guangzhou, China). A total of 200 g dried S. imbricatus was crushed into a powder, soaked in 10 volumes of distilled water for 30 min, and then extracted in boiling water for 1 h. The extract was cooled, filtered, evaporated under vacuum, and lyophilized using a rotary vacuum freeze dryer. The yield of the SIE from the dried powder was 20.27% (w/w). The phenol-sulfuric acid assay was used to determine the polysaccharide content of the SIE which was 3.41 ± 0.1% (w/w).
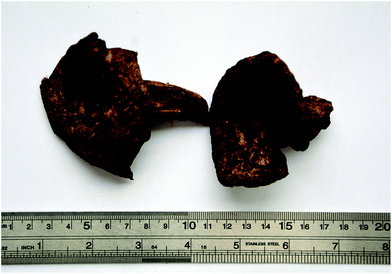 |
| Fig. 1
Sarcodon imbricatus (L. Fr.) Karst fruiting body. | |
2.2. Cell culture
Human (MDA-MB-231, MDA-MB-468, and MCF-7) and mouse (4T1) breast cancer cell lines were obtained from the American Type Culture Collection (Manassas, VA, USA). The human breast cancer cell line MT-1 was presented by Professor Burton B. Yang from the University of Toronto, Canada. Cells were cultured in RPMI-1640 or high glucose DMEM (4.5 g L−1, Gibco) supplemented with 10% (v/v) fetal bovine serum (FBS; Life Technologies, USA) and were maintained at 37 °C in a humidified 5% CO2 incubator.
2.3. Giemsa staining and cytotoxicity assay
Logarithmic phase cells were seeded at 1 × 105 per well in 24-well flat-bottom plates, allowed to adhere to the wells, and then incubated with various concentrations of the SIE for 72 h. The wells were washed with phosphate-buffered saline (PBS), fixed in methanol for 15 min, and incubated with 0.5 mL Giemsa (Nanjing Jiancheng, China, D010-1-3) stain for 20 min. The wells were then washed with distilled water and imaged using an inverted microscope (Kantao Technology, China).
Cell viability was assessed using Trypan blue dye exclusion. Wells were washed with PBS and cells were dislodged and dispersed by addition of 0.1 mL 0.25% (w/v) trypsin/0.53 mM EDTA solution. Complete growth medium (0.4 mL per well) was added to the wells, and the cells were harvested, resuspended in Trypan blue (Solarbio, C0040), and counted. The cells were plated and counted using an automated cell counter. Cell viability was calculated as
2.4. Wound-healing assay and transwell invasion assay
The effect of the SIE on MCF-7 and 4T1 migration was assessed using a wound-healing assay, as previously described.17 In brief, logarithmic phase cells were harvested, washed twice with PBS, resuspended in medium, and seeded in 6-well flat-bottom plates at 4 × 105 per well. The cells were incubated at 37 °C for 24 h, and a scratch was made across the center of each well using a pipet tip. Non-adherent cells were washed away with PBS, and the remaining cell monolayer was imaged using an inverted microscope. Various concentrations of the SIE were added to the wells, and the plates were incubated at 37 °C for 48 h. The wells were photographed at 0 and 48 h.
Cell invasion was assessed using a transwell migration assay.18 4T1 or MCF-7 cells were added to the upper chambers of Transwells at a density of 5 × 104 per 100 μL and the SIE was added to a total volume of 200 μL per well. Complete growth medium (500 μL per well) was added to the lower chambers. The plates were then incubated for 16 h at 37 °C. The non-migrated cells on the top surface of the filter membrane were scraped away with a cell scraper and the membrane was fixed in methanol and stained with Giemsa. Migrated cells on the under surface of the membrane were counted using an inverted microscope. Percentage invasion was calculated as
2.5. Mouse breast cancer model
Female BALB/c mice (4–6 weeks old, 16–18 g body weight) were obtained from the Medical Laboratory Animal Center of Guangdong Province (Approval No. SCXK [Yue] 2013-0092, Foshan, China). Mice were acclimated for 5 days prior to the experiments and were housed in a temperature-controlled room (24 ± 2 °C) on a 12 h/12 h light/dark cycle. Food and water were available ad libitum. This study was performed in strict accordance with the NIH guidelines for the care and use of laboratory animals (NIH Publication No. 85-23 Rev. 1985) and approved by the Animal Experimentation Ethics Committee of the Center of Laboratory Animals of the Guangdong Institute of Microbiology (Guangzhou, China).
The mouse breast cancer model was established as previously described.19 Briefly, female BALB/c mice were randomly divided into 4 groups of 12 mice each. The Control group was subcutaneously injected with 100 μL serum-free RPMI-1640 medium and was orally administered distilled water once daily. The Model, SIE and paclitaxel (PDX) groups were subcutaneously injected with 4T1 cells (2 × 104 in 100 μL medium). The mice were orally administered distilled water once daily (Model group), orally administered the SIE at 200 mg kg−1 once daily (SIE group), and intraperitoneally injected with PDX at 10 mg kg−1 once weekly (PDX group) for 15 days. Body weights and tumor volumes were monitored every 2 days. On day 16, mice were bled retro-orbitally and sacrificed by cervical dislocation. The tumors were excised and the thymuses and spleens were collected.
2.6. Tumor histology
Freshly excised tumors were fixed in neutral-buffered formalin, embedded in paraffin, and sectioned. The sections were then stained with hematoxylin and eosin (H&E) by standard methods20 and visualized using a microscope (Kantao Technology, China).
2.7. Preparation of splenocytes
Spleens were weighed and pressed through a nylon mesh (200 μm pore size) with a sterile glass syringe piston. The cell suspension was centrifuged at 250g for 5 min, and the pellet was incubated with 1 mL red blood cell lysis buffer (Shanghai Gefan Biotechnology Co., Ltd, GM02) to remove red blood cells. The pellet was washed with PBS, centrifuged at 250g for 5 min, and resuspended in complete RPMI-1640 medium supplemented with 10% (v/v) FBS and penicillin–streptomycin.
2.8. Natural killer cell assay
To assess natural killer (NK) cell activity in the splenocyte suspension, lysis of the NK cell-specific target cell YAC-1 was measured with quantifying the release of lactate dehydrogenase (LDH). Aliquots of splenocytes (2 × 106 cells per 100 μL) were mixed with YAC-1 cells (4 × 104 cells per 100 μL, splenocyte to target cell ratio of 50
:
1) in triplicate wells of a 96-well plate and incubated for 4 h at 37 °C. Positive control cells (4 × 104 YAC-1 cells + 1% Nonidet P40 in 200 μL RPMI-1640) and negative control cells (4 × 104 YAC-1 cells in 200 μL RPMI-1640) were also plated in triplicate. At the end of the 4 h incubation, the plates were centrifuged (1500 rpm, 5 min), and 100 μL of the supernatant was removed and placed in fresh wells, mixed with 100 μL of LDH conjugate, and incubated for 15 min. The reaction was stopped by addition of 30 μL 1 M HCl per well and the optical density (OD) at 490 nm was measured using a spectrophotometer. NK cell activity was determined using the formula
2.9. Spleen lymphocyte proliferation assay
Spleen lymphocyte proliferation was measured with the MTT ([3-(4,5-dimethylthiazol-2-yl)-2,5-diphenyltetrazolium bromide]) assay. When T lymphocytes are stimulated by Concanavalin A, the progenitor cell proliferation reaction occurs. MTT are decomposed by mitochondrial hydrolase of the proliferating cells to develop color, and the optical density value can reflect the proliferation of the cells. In brief, splenocytes were resuspended at 6 × 106 cells per mL in complete RPMI-1640 medium and added to triplicate wells of a 96-well plate at 100 μL per well. Positive and negative control wells contained an equal volume of Concanavalin A (3 μg mL−1) or medium, respectively. The plates were incubated for 72 h at 37 °C. At the end of the incubation, MTT reagent (5 mg mL−1) was 50 μl per well, and incubation was continued for 4 h for measurement. The OD at 490 nm was measured using a microplate reader. The stimulation index was calculated using the formula
2.10. Serum IL-2, IL-6, TNF-α, and IFN-γ ELISAs
Blood samples were collected retro-orbitally from mice at sacrifice, and serum was separated by centrifugation at 3500 rpm at 4 °C for 15 min. The serum samples were stored at −80 °C until analysis. Interleukin-2 (IL-2), IL-6, tumor necrosis factor-alpha (TNF-α), and Immunoglobulin G (IgG) levels were measured using Mouse IL-2, IL-6, TNF-α, and IgG ELISA kits (Cheng lin, Beijing, China) according to the manufacturer's instructions.
2.11. Immunohistochemistry of CD56 and immunofluorescence of PD-L1
For immunohistochemistry, formalin-fixed paraffin-embedded tumor tissues were cut into 4 μm sections. Tumor sections were deparaffinized through a graded series of dimethylbenzene and ethanol, and antigens were retrieved by incubation in Tris/EDTA buffer (pH 9.0) at 100 °C for 20 min. The sections were incubated with 3% H2O2 for 20 min to block endogenous peroxidase activity and then with 1% goat serum albumin for 30 min to block nonspecific binding. The sections were incubated overnight at 4 °C with the following primary antibodies diluted in PBS: anti-NCAM1 antibody (CD56, ab237708, 1
:
800). After washing, the sections were incubated with goat anti-rabbit IgG antibody (ab6721, 1
:
1000 in PBS) for 50 min at room temperature. The sections were then washed again, incubated with 3,3-diaminobenzidine (Solarbio, DA1010), washed and counterstained with hematoxylin. The sections were finally mounted and imaged using a microscope (Kantao Technology, China).
For immunofluorescence, additional sections were deparaffinized through a graded series of dimethylbenzene and ethanol, and antigens were retrieved by incubation in citric acid buffer (pH 6.0) at 100 °C for 20 min. The sections were incubated with 3% H2O2 for 20 min to block endogenous peroxidase activity and then with 1% goat serum albumin for 30 min to block nonspecific binding. The sections were incubated overnight at 4 °C with the following primary antibodies (all from Abcam) diluted in PBS: anti-PD-L1 antibody (ab213480, 1
:
800). After washing, the sections were incubated with goat anti-rabbit IgG antibody (F(ab′)2 Fragment, Alexa Fluor® 488 Conjugate, Cell Signaling Technology, 4412S) for 50 min at room temperature. After washing, DAPI (Servicebio, G1012) was used to stain the nuclei for 5 min, and images were captured using an inverted fluorescence microscope (EVOS™ FL Auto 2 Imaging System).
2.12. Statistical analysis
All data are expressed as mean ± standard deviation (SD). Statistical analysis was performed via one-way analysis of variance (ANOVA) followed by the least-significant-difference test using SPSS 19.0 software (IBM, Armonk, NY, USA). The level of statistical significance was set at p < 0.05.
3. Results
3.1. Cytotoxicity of the SIE on breast cancer cells in vitro
To determine whether the SIE was cytotoxic for breast cancer cells, the human cell lines MCF-7, MDA-MB-468, MDA-MB-231, and MT-1 and the mouse cell line 4T1 were incubated with different SIE concentrations for 72 h. Giemsa staining (Fig. 2A) and trypan blue exclusion assay (Fig. 2B) were used to assess the cell morphology and cell viability. The results showed a dose-dependent cytotoxic effect of the SIE on all of the cell lines between 200 and 1000 μg mL−1. MCF-7 and 4T1 were the most sensitive cell lines, followed by MDA-MB-231, MDA-MB-468, and MT-1 (Fig. 2B).
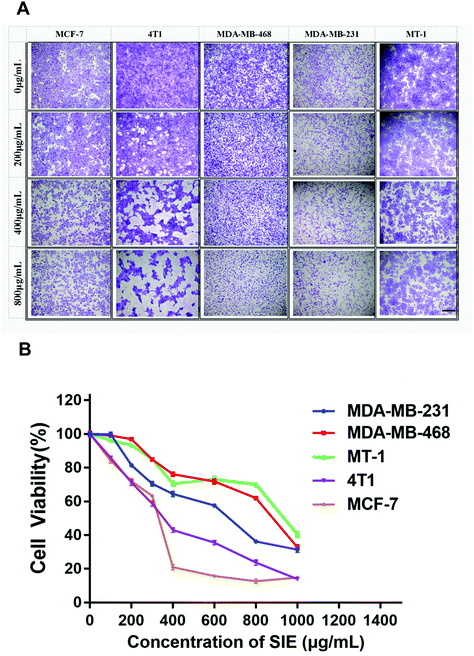 |
| Fig. 2 Cytotoxicity of the SIE towards breast cancer cell lines. MCF-7, 4T1, MDA-MB-468, MDA-MB-231, and MT-1 breast cancer cell lines were incubated with the indicated concentrations of the SIE for 72 h. (A) Giemsa staining. Images are representative of triplicate wells. (B) Trypan blue viability assay. Data are the mean of 6 wells. Scale bars = 1.0 mm. Magnification ×4. | |
3.2. Inhibitory effect of the SIE on 4T1 and MCF-7 cell migration and invasion
Wound-healing and transwell invasion assays were used to assess the effect of the SIE on 4T1 and MCF-7 cell migration and invasion in vitro. As shown in Fig. 3, 4T1 and MCF-7 migration at 48 h was effectively inhibited by 200–1000 μg mL−1 and 200–600 μg mL−1, respectively. Similarly, the SIE significantly reduced the invasive capacity of 4T1 and MCF-7 cells (Fig. 4A). The SIE at 100, 200, and 400 μg mL−1 reduced 4T1 cell invasion by 21.5%, 43.9% and 71.0% respectively (Fig. 4B), and the same concentrations of the SIE reduced MCF-7 cell invasion by 22.4%, 35.7% and 51.0% respectively (Fig. 4C).
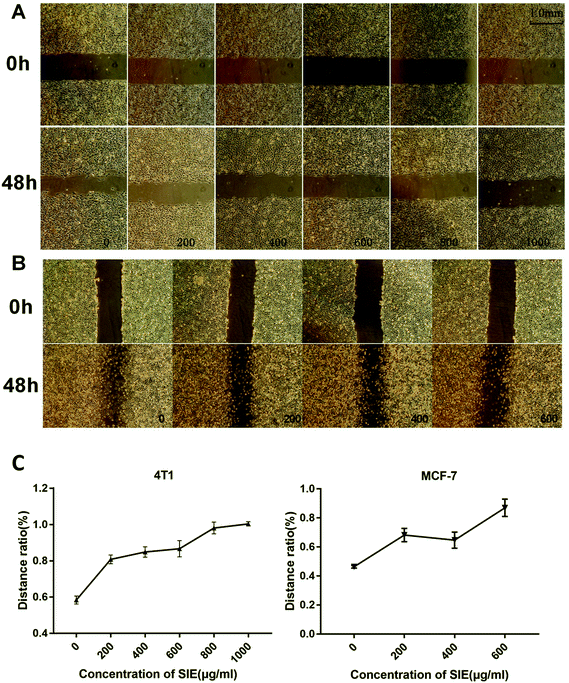 |
| Fig. 3 Effect of the SIE on 4T1 and MCF-7 cell migration. (A, B) Representative images of the wound-healing assay. 4T1 cells (A) and MCF-7 cells (B). Distance ratio of 4T1 cells and MCF-7 cells (C). Monolayers were wounded and incubated with up to 1000 μg mL−1 SIE for 48 h. Photographs were taken at 0 h and 48 h. Scale bars = 1.0 mm. Magnification ×10. | |
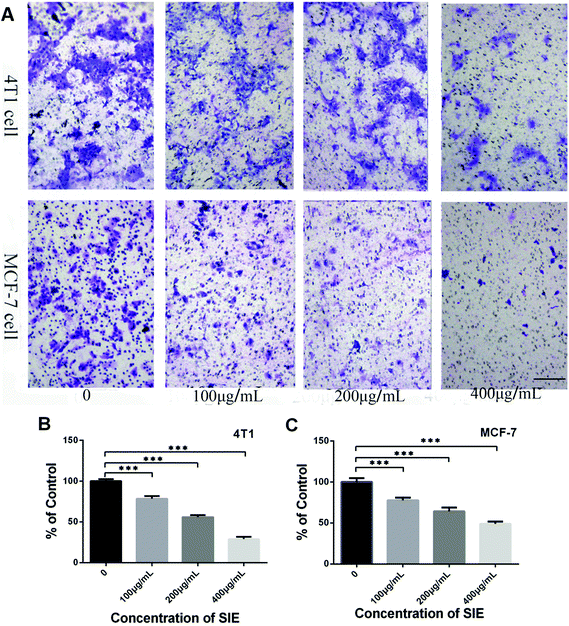 |
| Fig. 4 Effect of the SIE on 4T1 and MCF-7 cell invasion. (A) Representative images of a Transwell migration assay. 4T1 and MCF-7 cells were incubated with 0, 100, 200 or 400 μg mL−1 SIE. Photographs were taken after 16 h. (B, C) Quantification of the invasion assay with 4T1 cells (B) and MCF-7 cells (C). Data are presented as mean ± SD (n = 4). ***p < 0.001. Scale bars = 1.0 mm. Magnification ×10. | |
3.3. SIE inhibition of 4T1 cell growth in a mouse tumor model
To determine whether the SIE can inhibit the growth of breast cancer cells in vivo, BALB/c mice were injected with the mouse breast cancer cell line 4T1, and administered the SIE orally at 200 mg kg−1 once daily for 15 days. As negative and positive controls, additional groups of mice were orally administered water once daily or intraperitoneally injected with 10 mg kg−1 PDX once weekly, respectively. As shown in Fig. 5A, there was no significant loss of body weight attributable to the SIE during the treatment period, even as the tumor volumes increased. In the control animals treated with water (Model), tumor growth was evident by day 8 and continued until the end of the experiment (Fig. 5B and C). Notably, the SIE significantly reduced tumor growth and showed efficacy comparable to that of PDX (Fig. 5B and C). On day 15, the average weight of tumors excised from the SIE- and PDX-treated groups was significantly lower than that from the model group (p < 0.01, Fig. 5C and D). Consistent with this, H&E staining of tumor tissue sections from the SIE and PDX groups, but not the Model group, showed extensive cell death (Fig. 5E). These results suggest that the SIE can effectively inhibit the growth of breast cancer cells in mice. The SIE had no overt toxic effects on the animals during the experimental period.
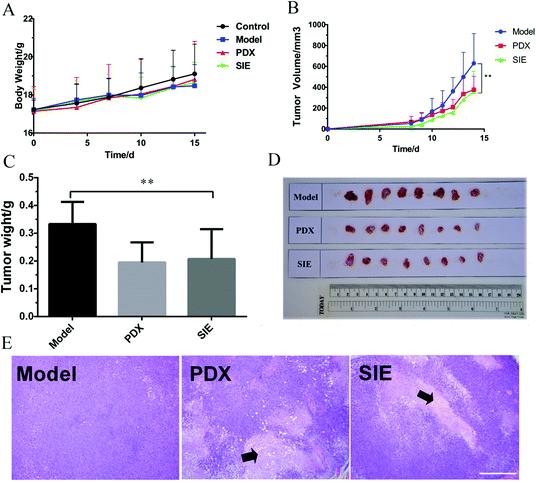 |
| Fig. 5 Anti-tumor effect of the SIE in the 4T1 mouse breast cancer model. Animals were injected with vehicle (Control) or 4T1 cells (all other groups) and treated with water (Model), 10 mg kg−1 paclitaxel weekly (PDX), or 100 mg kg−1 SIE daily for 2 weeks. (A) Body weights. (B) Tumor volumes. (C) Tumor weights on day 15. (D) Representative images of tumors on day 15. (E) Representative H&E-stained tumor sections. The arrows indicate extensive cell death. Data are presented as mean ± SD (n = 10 mice per group). **p < 0.01. Scale bars = 1.0 mm. Magnification ×4. | |
3.4. Immunomodulatory activity of the SIE in the 4T1 mouse tumor model
To determine whether the SIE influences breast cancer cell growth in vivo by modulating the immune system, the ratio of thymus and spleen weights to the total body weight (spleen/thymus indexes) was examined. As shown in Fig. 6A, there were no significant differences in the thymus index of non-tumor-bearing and 4T1-bearing mice at day 15. Unexpectedly, the tumor-bearing mice showed a marked increase in the spleen index compared with the non-tumor-bearing mice (control vs. model, p < 0.01, Fig. 6B). The spleen index of SIE-treated mice was significantly less than that of the untreated tumor-bearing mice (p < 0.05, Fig. 6B), suggesting that, while tumor growth severely decreased the spleen weight, the SIE had a modest protective effect.
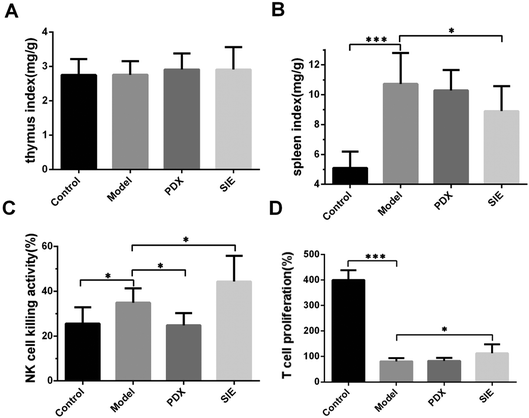 |
| Fig. 6 Effect of the SIE on the immune status in the 4T1 mouse breast cancer model. Mice were treated as described in Fig. 5. On day 15, the thymuses and spleens were harvested and weighed. Splenocytes were prepared and assayed for natural killer (NK) cell activity or proliferation. (A, B) Ratio of mean thymus (A) and spleen (B) weights to the mean body weight of each mouse group. (C) NK cell activity. (D) T cell proliferation. Groups are as described in Fig. 5. Data are presented as mean ± SD (n = 10 mice per group). *p < 0.05 and ***p < 0.001. | |
To explore this further, the ability of splenocytes to kill the mouse NK cell target YAC-1 was examined. Tumor injection significantly increased splenocyte NK cell activity (p < 0.05, Fig. 6C) and this was further increased by SIE treatment and decreased by PDX treatment. The results of the proliferative capacity of splenic lymphocyte T cells taken from these animals showed that all tumor bearing mice showed reduced proliferative power compared to control mice (Fig. 6D). Here, SIE treatment showed a small but significant improvement, resulting in a slight increase in T cell proliferative power stimulated by CoA protein (p < 0.05, Fig. 6D).
Expression of pro-inflammatory cytokines can have a major effect on the initiation and progression of many tumors.21 To determine whether the SIE affects cytokine production in the mouse model, serum levels of IL-2, IL-6, TNF-α, and IgG were measured at day 15 after tumor injection. As shown in Fig. 7, serum levels of IL-2, IL-6, and TNF-α were significantly reduced in the model group compared with the control group (p < 0.05). Interestingly, both SIE and PDX increased serum IL-2 (Fig. 7A) and TNF-α (Fig. 7C) levels, whereas only SIE resulted in elevation of IL-6 (Fig. 7B). In contrast, the PDX group reduced serum IgG levels compared to the model group and (p < 0.05) there was no significant change in the SIE group (Fig. 7D).
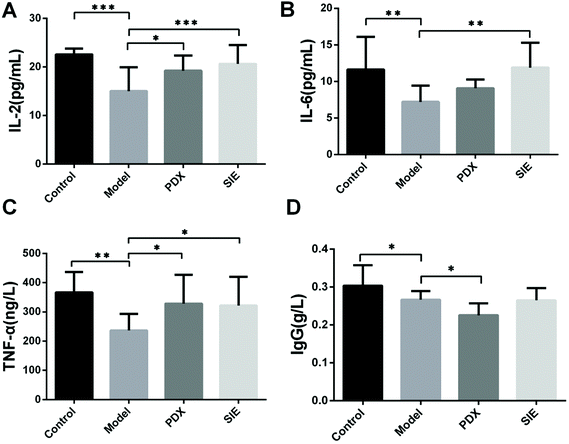 |
| Fig. 7 Effect of the SIE on serum cytokine levels in the 4T1 mouse breast cancer model. (A–D) Mice were treated as described in Fig. 5. On day 15, serum samples were prepared and assayed for IL-2 (A), IL-6 (B), TNF-α (C), and IgG (D) by ELISA. Groups are as described in Fig. 5. Data are presented as mean ± SD (n = 10 mice per group). *p < 0.05, **p < 0.01, and ***p < 0.001. | |
The results in Fig. 6 indicate that the SIE enhances the activity and proliferative capacity of spleen NK cells and T cells. Immunohistochemistry and immunofluorescence were used to label NK cells and PD-L1 in tumor tissues. As shown in Fig. 8, expression of CD56 increased in the SIE-treated groups (Fig. 8A). Meanwhile, the SIE significantly reduced the expression of PD-L1 in tumor tissues (Fig. 8B and C).
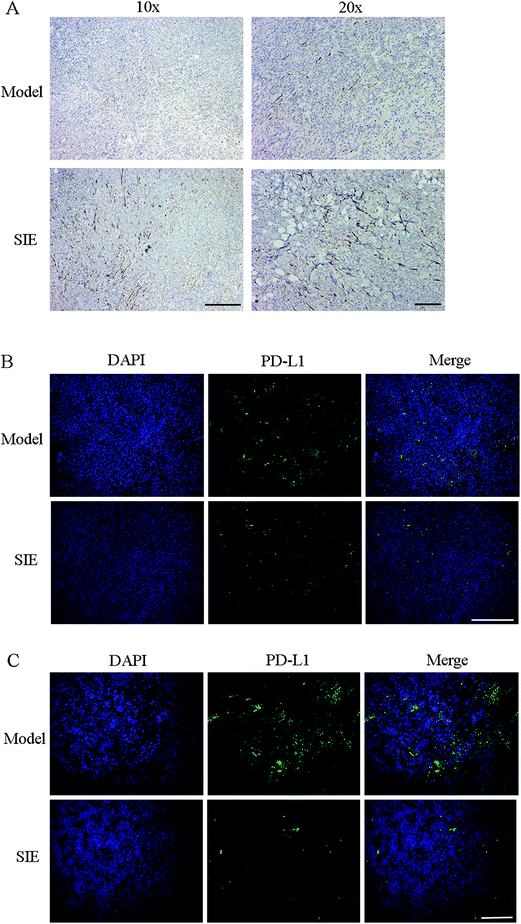 |
| Fig. 8 Effect of the SIE on CD56 and PD-L1 in the 4T1 mouse breast cancer model. (A) Immunohistochemical staining of tumor sections indicating CD56. (B, C) Fluorescence micrographs of tumor sections indicating PD-L1 (green). The sections were also stained with DAPI (blue) to visualize nuclei. (B) Scale bars = 500 μm. Magnification ×10. (C) Scale bars = 150 μm. Magnification ×20. | |
4. Discussion
S. imbricatus is a commonly consumed wild mushroom in China, and it was shown to have anti-oxidative,9–11 immunomodulatory,12 and bacteriostatic13 properties. Previous experiments have shown that a water extract of S. imbricatus was cytotoxic towards cancer cells.16 However, there have been few studies examining whether S. imbricatus retains its anti-cancer activity when orally administered in vivo. The result suggested that the anti-cancer activity of the SIE may be through immune regulation mechanisms.
Inhibition of tumor cell proliferation is a common assay for screening anti-cancer drugs, and several edible fungi with anti-cancer properties have been shown to have anti-proliferative and/or cytotoxic effects on tumor cells. For instance, a polysaccharide from Lentinus giganteus can inhibit the proliferation of HepG-2 tumor cells.22 Similarly, aqueous extracts of Lentinula edodes and Pleurotus sajor-caju have been reported to be cytotoxic towards tumor cells.23 In this study, the SIE had various dose-dependent effects on the breast cancer cell lines MDA-MB-231, MDA-MB-468, MT-1, MCF-7, and 4T1 in vitro, including suppression of viability, migration, and invasion. The SIE may also have the effect of inhibiting tumor metastasis. Through research and this experiment, it is speculated that the active ingredient of the SIE may be polysaccharides.
Most preclinical mouse models for testing anti-cancer reagents with human cancer cell lines are conducted in immunodeficient mice.24 However, these mice are not suitable for testing drugs and herbal medicines that may exert their anti-cancer properties via effects on the immune system.25 The results suggested that the anti-cancer effects of S. imbricatus may be related to its immunomodulatory properties. BALB/c mice were commonly used for tumor and immunological studies. Here, female BALB/c mice were used for testing SIE effects on the mouse breast cancer cell line 4T1, which was originally isolated from BALB/c mice. In this model, the SIE effectively inhibited 4T1 tumor growth, and H&E staining of tumor sections revealed extensive intra-tumoral cell death.
Increasing evidence points to the importance of the immune system in breast cancer,26 and primary immune organs, such as the spleen and thymus, play crucial roles in regulating anti-tumor immunity.27,28 The results demonstrated that the spleen index was significantly reduced by 4T1 tumor growth, whereas the thymus index was unaffected. NK cell activity29 and T cell proliferation were measured to assess the immunocompetence of the splenocytes from these mice. Here, the SIE promoted both of these properties in tumor-bearing mice, suggesting that the SIE may inhibit tumor growth via effects on the immune system.
Cytokines are known to play crucial roles in regulating the growth of breast cancer cells.30 Using IL-2 has been considered as a possible method of treating breast cancer31,32 since it can induce tumor regression by enhancing the cytotoxicity of NK cells and lymphokine-activated killer cells.33,34 Furthermore, TNF-α upregulates the secretion of numerous anti-proliferative, anti-angiogenic cytokines, which is particularly beneficial in halting the progression of breast cancer, including triple-negative breast cancer.35,36 Although IL-6 has been proposed to be a prognostic marker of breast cancer, this is controversial.37,38 In the present study, the result showed that the SIE upregulated serum levels of IL-2, IL-6, and TNF-α in the 4T1 mouse model. However, the SIE had no effect on serum IgG levels in tumor-bearing mice. Therefore, it is possible that the anti-tumor effect of the SIE may be due to the induction of cytokines such as IL-2, IL-6, and TNF-α and subsequent activation of cytotoxic cells.
Cancer immunotherapy involves blocking the interactions between the PD-1/PD-L1 immune checkpoints and antibodies.39 This has shown unprecedented positive outcomes in clinics. Particularly, PD-L1 antibody therapy has shown efficiency in blocking membrane PD-L1 and efficacy in treating some advanced carcinomas.40 Immunofluorescence results showed that the SIE significantly reduced the expression of PD-L1 in tumors, which also suggested why the expression of CD56 was increased in the tumors of the SIE-treated group.
5. Conclusion
In conclusion, the present study demonstrated that SIE inhibited the proliferation and invasive properties of breast cancer cells in vitro and significantly decreased the tumor volume and weight in mice, which may be achieved by regulating the expression of PD-L1 and increasing NK cell viability in tumors. Collectively, this study provides supporting evidence for the use of the SIE as a health supplement in breast cancer patients.
Abbreviations
FBS | Fetal bovine serum |
H&E | Hematoxylin and eosin |
IgG | Immunoglobulin G |
IL-2 | Interleukin-2 |
IL-6 | Interleukin-6 |
OD | Optical density |
PBS | Phosphate-buffered saline |
PDX | Paclitaxel |
SI |
Sarcodon imbricatus
|
SIE |
Sarcodon imbricatus aqueous extract |
TNF-α | Tumor necrosis factor-α |
PD-1 | Programmed cell death 1 |
PD-L1 | Programmed cell death-Ligand 1 |
Author contributions
Y. Z. X. conceived and designed the work; Y. Z. X. and C. W. J. coordinated technical support and funding; X. P. T. and W. C. wrote the manuscript; X. P. T. and H. J. L. performed the experiments and collected the samples; Y. J. L. and H. Y. acquired, analyzed, and interpreted the data; and C. Y. H., J. M. C and X. W. M. participated in data collection and analysis. All authors read and approved the final manuscript.
Conflicts of interest
The authors declare no conflicts of interest.
Acknowledgements
The authors acknowledge the Innovation and Entrepreneurship Leading Talent of Guangzhou Development Zone (2017-l181) and the Funding of Introducing Innovation and Entrepreneurship Team Project in Zhaoqing High-tech Zone.
References
- Q. Xia, F. Geng, F. F. Zhang, C. L. Liu, P. Xu, Z. Z. Lu, B. Sun, H. Wu, B. Yu, W. Kong, X. H. Yu and H. H. Zhang, Cyclophosphamide enhances anti-tumor effects of a fibroblast activation protein α-based DNA vaccine in tumor-bearing mice with murine breast carcinoma, Immunopharmacol. Immunotoxicol., 2017, 39(1), 37–44 CrossRef CAS PubMed.
- C. Chen, Z. Nong, Q. Xie, J. He, W. Cai, X. Tang, X. Chen, R. Huang and Y. Gao, 2-Dodecyl-6-methoxycyclohexa-2,5-diene-1,4-dione inhibits the growth and metastasis of breast carcinoma in mice, Sci. Rep., 2017, 7(1), 6704 CrossRef.
- J. Ferlay, M. Colombet and I. Soerjomataram, Estimating the global cancer incidence and mortality in 2018: GLOBOCAN sources and methods, Int. J. Cancer, 2019, 144(8), 1941–1953 CrossRef CAS.
- E. Iwabuchi, Y. Miki, K. Ono, Y. Onodera, T. Suzuki, H. Hirakawa, T. Ishida, N. Ohuchi and H. Sasano, In situ detection of estrogen receptor dimers in breast carcinoma cells in archival materials using proximity ligation assay (PLA), J. Steroid Biochem. Mol. Biol., 2017, 165(Pt B), 159–169 CrossRef CAS PubMed.
- P. Zhang, X. Liu, H. Li, Z. Chen, X. Yao, J. Jin and X. Ma, TRPC5-induced autophagy promotes drug resistance in breast carcinoma via CaMKKβ/AMPKα/mTOR pathway, Sci. Rep., 2017, 7(1), 3158 CrossRef PubMed.
- J. B. Guimarães, E. C. Dos Santos, E. S. Dias, A. G. Bertechini, C. L. da Silva Ávila and F. S. Dias, Performance and meat quality of broiler chickens that are fed diets supplemented with Agaricus brasiliensis mushrooms, Trop. Anim. Health Prod., 2014, 46(8), 1509–1514 CrossRef PubMed.
- B. Muszyńska, A. Grzywacz-Kisielewska, K. Kała and J. Gdula-Argasińska, Anti-inflammatory properties of edible mushrooms: A review, Food Chem., 2018, 243, 373–381 CrossRef PubMed.
- D. Sohretoglu and S. Huang,
Ganoderma lucidum Polysaccharides as An Anti-cancer Agent, Anticancer Agents Med. Chem., 2018, 18(5), 667–674 CrossRef CAS PubMed.
- Y. Luo, Y. Huang, X. Yuan, L. Zhang, X. Zhang and P. Gao, Evaluation of Fatty Acid Composition and Antioxidant Activity of Wild-Growing Mushrooms from Southwest China, Int. J. Med. Mushrooms, 2017, 19(10), 937–947 CrossRef PubMed.
- T. Ozen and I. Türkekul, Antioxidant activities of Sarcodon imbricatum wildly grown in the Black Sea Region of Turkey, Pharmacogn. Mag., 2010, 6(22), 89–97 CrossRef CAS PubMed.
- L. Barros, P. Baptista, D. M. Correia, J. S. Morais and I. C. Ferreira, Effects of conservation treatment and cooking on the chemical composition and antioxidant activity of Portuguese wild edible mushrooms, J. Agric. Food Chem., 2007, 55(12), 4781–4788 CrossRef CAS.
- M. J. Alves, I. C. Ferreira, I. Lourenço, E. Costa, A. Martins and M. Pintado, Wild mushroom extracts as inhibitors of bacterial biofilm formation, Pathogens, 2014, 3(3), 667–679 CrossRef PubMed.
- F. Meng, P. Xu, X. Wang, Y. Huang, L. Wu, Y. Chen, L. Teng and D. Wang, Investigation on the immunomodulatory activities of Sarcodon Imbricatus extracts in a cyclophosphamide (CTX)-induced immunosuppressanted mouse model, Saudi Pharm. J., 2017, 25(4), 460–463 CrossRef.
- M. G. Morvan and L. L. Lanier, NK cells and cancer: you can teach innate cells new tricks, Nat. Rev. Cancer, 2016, 16(1), 7–19 CrossRef CAS.
- F. Bertucci and A. Gonçalves, Immunotherapy in Breast Cancer: the Emerging Role of PD-1 and PD-L1, Curr. Oncol. Rep., 2017, 19(10), 64 CrossRef.
- C. Jiao, Y. Z. Xie, X. Yang, H. Li, X. M. Li, H. H. Pan, M. H. Cai, H. M. Zhong and B. B. Yang, Anticancer activity of Amauroderma rude, PLoS One, 2013, 8(6), e66504 CrossRef CAS.
- C. H. Wu, F. C. Liu, C. H. Pan, M. T. Lai, S. J. Lan, C. H. Wu and M. J. Sheu, Suppression of Cell Growth, Migration and Drug Resistance by Ethanolic Extract of Antrodia cinnamomea in Human Lung Cancer A549 Cells and C57BL/6J Allograft Tumor Model, Int. J. Mol. Sci., 2018, 19(3), 1–15 Search PubMed.
- Z. Guo, W. Li, Y. Yuan, K. Zheng, Y. Tang, K. Ma, C. Cui, L. Wang, B. He and Q. Zhang, Improvement of chemosensitivity and inhibition of migration via targeting tumor epithelial-to-mesenchymal transition cells by ADH-1-modified liposomes, Drug Delivery, 2018, 25(1), 112–121 CrossRef CAS.
- N. Gajovic, M. Jurisevic, J. Pantic, G. Radosavljevic, N. Arsenijevic, M. L. Lukic and I. Jovanovic, Attenuation of NK cells facilitates mammary tumor growth in streptozotocin-induced diabetes in mice, Endocr.-Relat. Cancer, 2018, 25(4), 493–507 CAS.
- J. Sakata, K. Yamana, R. Yoshida, Y. Matsuoka, K. Kawahara, H. Arita, Y. Nagao, A. Hiraki, M. Shinohara, R. Toya, R. Murakami and H. Nakayama, Tumor budding as a novel predictor of occult metastasis in cT2N0 tongue squamous cell carcinoma, Hum. Pathol., 2018, 17, 30492–30496 Search PubMed.
- D. Trivanović, A. Jauković, J. Krstić, S. Nikolić, I. Okić Djordjević, T. Kukolj, H. Obradović, S. Mojsilović, V. Ilić, J. F. Santibanez and D. Bugarski, Inflammatory cytokines prime adipose tissue mesenchymal stem cells to enhance malignancy of MCF-7 breast cancer cells via transforming growth factor-β1, IUBMB Life, 2016, 68(3), 190–200 CrossRef.
- Y. Tian, Y. Zhao, H. Zeng, Y. Zhang and B. Zheng, Structural characterization of a novel neutral polysaccharide from Lentinus giganteus and its antitumor activity through inducing apoptosis, Carbohydr. Polym., 2016, 154, 231–240 CrossRef CAS.
- T. CFinimundy, G. Gambato, R. Fontana, M. Camassola, M. Salvador, S. Moura, J. Hess, J. A. Henriques, A. J. Dillon and M. Roesch-Ely, Aqueous extracts of Lentinula edodes and Pleurotus sajor-caju exhibit high antioxidant capability and promising in vitro antitumor activity, Nutr. Res., 2013, 33(1), 76–84 CrossRef.
- J. Yang, F. Han, W. Liu, H. Chen, X. Hao, X. Jiang, L. Yin, Y. Huang, J. Cao, H. Zhang and J. Liu, ALX4, an epigenetically down regulated tumor suppressor, inhibits breast cancer progression by interfering Wnt/β-catenin pathway, J. Exp. Clin. Cancer Res., 2017, 36(1), 1–15 CrossRef PubMed.
- H. Pan, Y. Han, J. Huang, X. Yu, C. Jiao, X. Yang, P. Dhaliwal, Y. Xie and B. B. Yang, Purification and identification of a polysaccharide from medicinal mushroom Amauroderma rude with immunomodulatory activity and inhibitory effect on tumor growth, Oncotarget, 2015, 6(19), 17777–17791 CrossRef.
- J. Milovanović, N. Todorović-Raković and M. Radulovic, Interleukin-6 and interleukin-8 serum levels in prognosis of hormone-dependent breast cancer, Cytokine, 2019, 118, 93–98 CrossRef.
- D. R. Beckford Vera, C. C. Smith, L. M. Bixby, D. M. Glatt, S. S. Dunn, R. Saito, W. Y. Kim, J. S. Serody, B. G. Vincent and M. C. Parrott, Immuno-PET imaging of tumor-infiltrating lymphocytes using zirconium-89 radiolabeled anti-CD3 antibody in immune-competent mice bearing syngeneic tumors, PLoS One, 2018, 13(3), e0193832 CrossRef.
- G. Shu, S. Jiang, J. Mu, H. Yu, H. Duan and X. Deng, Antitumor immunostimulatory activity of polysaccharides from Panax japonicus C. A. Mey: Roles of their effects on CD4+ T cells and tumor associated macrophages, Int. J. Biol. Macromol., 2018, 111, 430–439 CrossRef CAS.
- Y. Wang, M. Huang, R. Sun and L. Pan, Extraction, characterization of a Ginseng fruits polysaccharide and its immune modulating activities in rats with Lewis lung carcinoma, Carbohydr. Polym., 2015, 127, 215–221 CrossRef CAS PubMed.
- H. Senju, A. Kumagai, Y. Nakamura, H. Yamaguchi, K. Nakatomi, S. Fukami, K. Shiraishi, Y. Harada, M. Nakamura, H. Okamura, Y. Tanaka and H. Mukae, Effect of IL-18 on the Expansion and Phenotype of Human Natural Killer Cells: Application to Cancer Immunotherapy, Int. J. Biol. Sci., 2018, 14(3), 331–340 CrossRef CAS PubMed.
- X. B. Hu, L. Z. Ouyang and L. L. Tang, Interleukin-2 gene polymorphisms and prognosis of breast cancer, Genet. Test. Mol. Biomarkers, 2013, 17(6), 453–457 CrossRef CAS.
- S. G. Semushina, D. A. Aronov and E. V. Moiseeva, Local Interleukin-2 Immunotherapy of Breast Cancer: Benefit and Risk in a Spontaneous Mouse Model, Pathol. Oncol. Res., 2018, 6, 1007–1025 Search PubMed.
- Y. R. Suryawanashi, T. Zhang, H. M. Woyczesczyk, J. Christie, E. Byers, S. Kohler, R. Eversole, C. Mackenzie and K. Essani, T-independent response mediated by oncolytic tanapoxvirus recombinants expressing interleukin-2 and monocyte chemoattractant protein-1 suppresses human triple negative breast tumors, Med. Oncol., 2017, 34(6), 112–121 CrossRef.
- X. Li, P. Lu, B. Li, W. Zhang, R. Yang, Y. Chu and K. Luo, Interleukin 2 and interleukin 10 function synergistically to promote CD8+ T cell cytotoxicity, which is suppressed by regulatory T cells in breast cancer, Int. J. Biochem. Cell Biol., 2017, 87, 1–7 CrossRef.
- H. Allen, N. Shraga-Heled, M. Blumenfeld, T. Dego-Ashto, D. Fuchs-Telem, A. Gilert, Z. Aberman and R. Ofir, Human Placental-Derived Adherent Stromal Cells Co-Induced with TNF-α and IFN-γ Inhibit Triple-Negative Breast Cancer in Nude Mouse Xenograft Models, Sci. Rep., 2018, 8(1), 670 CrossRef.
- I. Martínez-Reza, L. Díaz and R. García-Becerra, Preclinical and clinical aspects of TNF-α and its receptors TNFR1 and TNFR2 in breast cancer, J. Biomed. Sci., 2017, 24(1), 90 CrossRef.
- M. Esquivel-Velázquez, P. Ostoa-Saloma, M. I. Palacios-Arreola, K. E. Nava-Castro, J. I. Castro and J. Morales-Montor, The role of cytokines in breast cancer development and progression, J. Interferon Cytokine Res., 2015, 35(1), 1–16 CrossRef PubMed.
- D. Conze, L. Weiss, P. S. Regen, A. Bhushan, D. Weaver, P. Johnson and M. Rincón, Autocrine production of interleukin 6 causes multidrug resistance in breast cancer cells, Cancer Res., 2001, 61(24), 8851–8858 CAS.
- M. Feng, G. Xiong, Z. Cao, G. Yang, S. Zheng, X. Song, L. You, L. Zheng, T. Zhang and Y. Zhao, PD-1/PD-L1 and immunotherapy for pancreatic cancer, Cancer Lett., 2017, 407, 57–65 CrossRef CAS.
- A. G. Sacher and L. Gandhi, Biomarkers for the Clinical Use of PD-1/PD-L1 Inhibitors in Non-Small-Cell Lung Cancer: A Review, JAMA Oncol., 2016, 2(9), 1217–1222 CrossRef.
|
This journal is © The Royal Society of Chemistry 2020 |
Click here to see how this site uses Cookies. View our privacy policy here.