DOI:
10.1039/C9SC01657K
(Edge Article)
Chem. Sci., 2019,
10, 6553-6559
Ligand and counteranion enabled regiodivergent C–H bond functionalization of naphthols with α-aryl-α-diazoesters†
Received
4th April 2019
, Accepted 25th May 2019
First published on 27th May 2019
Abstract
Here, an unprecedented ligand and counteranion-controlled and site-selectivity switchable direct C–H bond functionalization of unprotected naphthols with α-aryl-α-diazoesters was developed. In this transformation, site selectivities are realized by turning on/off the coordination between metal complexes and hydroxy groups. The preliminary mechanism revealed that the interaction between the hydroxy group and gold catalyst plays a key role in switching the site-selectivity of gold-carbene. This protocol potentially provides a novel design for C–H bond functionalization.
Compared to traditional convergent synthesis, divergent synthesis can generate molecule libraries with a high level of structural diversity in an efficient manner, which is of great importance in modern organic synthesis and drug discovery.1 Although recent years have witnessed significant achievements in this field,2 the development of a new strategy to achieve divergent synthesis with high chemo- and site-selectivity is still highly desirable.
Recently, site-selective C(sp2)–H bond functionalization of aromatic rings has emerged as one of the most effective and powerful methods to construct C–C bonds in organic synthesis. One useful and popular strategy to ensure high selectivity entails the use of a coordinating directing group (DG) on arenes (Scheme 1a).3–5 This chelation-assisted approach has been significantly exploited successfully for ortho C(sp2)–H bond functionalization,3 in some cases for meta selectivity,4 and in few cases for para selectivity5 (Scheme 1a). However, the site-selectivity of C–H bond functionalization is normally dependent on the length and structure of the DG, which limit the utilization of this approach in organic synthesis. The development of controllable site-divergent C–H bond functionalization without the installation of an additional DG is urgent and challenging.6
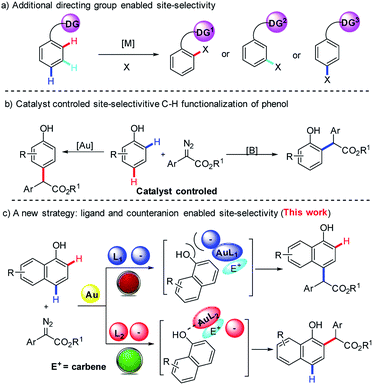 |
| Scheme 1 Different strategies to site-selective C(sp2)–H bond functionalization. | |
Naphthols and their derivatives are widely found in natural products, dyes, pharmaceuticals, bioactive compounds, materials, privileged ligands and so on (Fig. 1).7 Besides, they also represent one class of ideal and versatile starting materials and building blocks in organic synthesis. Thus, the development of straightforward access to naphthol derivatives via site-selective C(sp2)–H bond functionalization of naphthols is highly attractive to the synthetic community. Recently, Shi8 and our group9a independently developed gold-catalyzed highly site-selective para C(sp2)–H bond functionalization of phenols with α-aryl-α-diazoesters. Later on, our group also realized the more challenging highly selective ortho C(sp2)–H bond functionalization of phenols on catalysis by (C6F5)3B (Scheme 1b).9b As an ongoing interest in C(sp2)–H bond functionalization,9 we wanted to achieve direct and site-divergent C(sp2)–H bond functionalization of 1-naphthol via diazo compounds.10,11 However, our target is a very challenging issue. First, the free naphthol hydroxyl group is very reactive towards diazo compounds, typically resulting in O–H functionalization rather than C–H functionalization. To date, only the O–H insertion reactions of naphthols with diazo compounds have been reported.12 Second, the functionalization of ortho C–H bonds is more difficult than that of the para position due to similar nucleophilicity but more steric hindrance at the ortho position.13 Third, 1-naphthol is more reactive toward electrophiles than phenol;14 thus the control of the site-selectivity easily becomes more challenging. Indeed, we found that the reaction of 1-naphthol 1a and phenyl diazoester 2a gave a mixture of products with low site-selectivity on catalysis by a gold/phosphite catalyst and a low yield of ortho-substituted products in the presence of (C6F5)3B, respectively (Scheme 2). These results indicated that exploring an alternative strategy was highly necessary.
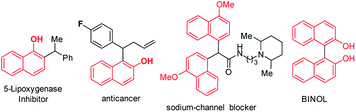 |
| Fig. 1 Naphthol derivatives in bioactive molecules and ligands. | |
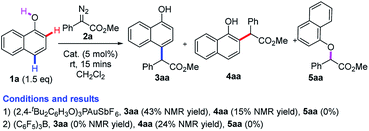 |
| Scheme 2 Initial studies under our previous conditions. | |
Recently, Yamaguchi15 and Yi16 reported ortho C–H bond alkenylation and alkylation via the coordination between the hydroxyl group and metal. Inspired by their nice findings, we envisioned that ortho-selectivity might be observed when the catalyst can turn on the coordination between the directing group and metal. In contrast, the para-substituted product becomes dominant if the catalyst can turn off the coordination between the directing group and metal, and meanwhile prevent the intermolecular electrophilic attack of carbene (Scheme 1c).
We envisioned that a bulky ligand might stop the coordination between gold complexes17,18 and hydroxyl groups and thus favor para-selective C–H bond functionalization. But unfortunately, the use of bulky X-phos and tBuXPhos derived gold complexes still delivered a similar selectivity to the tris(2,4-di-tert-butylphenyl)phosphite/gold complex (Table 1, entries 1 and 2). These results indicate that increasing only the steric hindrance at the phosphorus center of the phosphine ligand is not enough to suppress the coordination between gold and 1-naphthol because of the linear coordination geometry of the gold complex.19 In 2013, an elegant study of the Echavarren group disclosed that congested chloride-bridged digold complexes were formed when JohnphosAuCl was treated with AgOTf.20 Inspired by this result, we conceived that this complex was an ideal catalyst for para-selectivity. Indeed, we did obtain exclusively para-substituted products in 70% NMR yield (Table 1, entry 3). The NMR yield increased to 90% via switching the solvent to THF (Table 1, entry 4). In contrast, we envisioned that enhancing the coordination between the catalyst and hydroxyl group might elevate ortho-selectivity by using a weakly coordinated tetrakis[3,5-bis (trifluoro methyl)phenyl]borate (NaBArF) anion instead of the chloride one (Table 1, entry 5). Inspired by the work of Echavarren,21 we speculated that 1-naphthol could also coordinate with the NHC–gold complex using BarF− as a counteranion. Subsequently, we tested the combination of the NHC ligand (IPr) and NaBArF, affording the ortho-selective product 4aa in 82% isolated yield with 88
:
12 ratio of ortho- vs. para-selectivity (Table 1, entry 5). In addition, the yield and selectivity of the ortho-substituted product would be easily elevated via switching the R substituent on the ester group of diazo compounds 2 (Table 1, entries 6–8). It should be noted that other metals, such as Rh, Cu, Pd,10 which were widely used in diazo chemistry, could not give comparable results (for more details, please see the ESI, Table S1†).
Table 1 Variation of reaction parameters
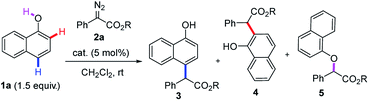
|
Entry |
R (2) |
Cat. (5 mol%) |
Yielda (%) |
3/4/5 |
NMR yield, the number in parentheses is the isolated yield.
THF as the solvent. XPhos = 2-dicyclohexylphosphino-2′,4′,6′-triisopropylbiphenyl; tBuXPhos = 2-di-t-butylphosphino-2′,4′,6′-tri-i-propyl-1,1′-bipheny; Johnphos = 2-(di-tert-butylphosphino)biphenyl; and IPr = 1,3-bis(2,6-di-ipropyl-phenyl)imidazol-2-ylidene. NaBArF = sodium tetrakis[3,5-bis (trifluoro methyl)phenyl]borate.
|
1 |
Me (2a) |
X-PhosAuCl/AgSbF6 |
50/31/0 |
2 |
Me (2a) |
t
BuXPhosAuCl/AgSbF6 |
49/27/0 |
3 |
Me (2a) |
JohnphosAuCl/AgOTf |
70/0/0 |
4b |
Me (2a) |
JohnphosAuCl/AgOTf |
90(87)/0 |
5 |
Me (2a) |
JohnphosAuCl/NaBArF |
26/54 |
6 |
Me (2a) |
IPrAuCl/NaBArF |
13/90(82)/0 |
7 |
Et (2b) |
IPrAuCl/NaBArF |
8/(87)/0 |
8 |
iPr (2c) |
IPrAuCl/NaBArF |
4/(91)/0 |
9 |
t
Bu (2d) |
IPrAuCl/NaBArF |
3/(90)/0 |
Under the optimal reaction conditions in hand, we next investigated the scope of this gold-catalyzed para-selective C–H functionalization of 1-naphthol. Gratifyingly, our protocol was applicable to a series of α-aryl-α-diazoacetates for the para-selective C–H functionalization of 1-naphthols. The reactions between 1-naphthol and α-aryl-α-diazoacetates bearing electron-donating or electron-withdrawing groups in the phenyl ring could deliver the site-specific products 3ab–3ao in good to excellent yields (72% to 99%). Moreover, a range of substituted 1-naphthols were also applicable to para-selective C–H bond functionalization, delivering the corresponding products 3ba–3ia in moderate to excellent yields (52% to 97%). However, C5-substituted 1-naphthol was incompatible with this transformation due to the bulky allylic 1,3-strain. It is well known that cyclopropanation22 would take place when an alkene is treated with a diazo compound using metal catalysts. However, no cyclopropanation product was detected in our reaction because of the low reactivity of this double bond (Table 2, 3ia). Finally, this para-selective C–H functionalization reaction could be used for the late-stage modification of β-blockers (propranolol), delivering the desired product in 94% yield (Table 2, 3ja). It is noteworthy that the diastereoselectivity was perfect for this transformation and the dr ratio was from the inversion isomer of the nitrogen atom. The structure of the product was further confirmed by single-crystal X-ray diffraction analysis of 3ah.23
Table 2 Gold(I)-catalyzed para-selective and ortho-selective C–H bond functionalization of 1-naphthols

|
Isolated yield of a single isomer.
2.0 equiv. diazo compound was used.
|
|
Subsequently, we turned to investigate the scope of gold-catalyzed ortho-selective C–H bond functionalization of 1-naphthols. All the reactions proceeded smoothly, affording the desired ortho-selective C–H functionalization products in moderate to excellent yields (52% to 88%) with up to 100
:
0 site-selectivity. Besides, the installation of strong electron-donating or electron-withdrawing groups in the phenyl ring of diazo compounds would decrease the regioselectivity (4ao and 4ap). In general, substituted 1-naphthols would increase the regioselectivity of the desired products. To our surprise, no C–H bond functionalization occurred at the ortho position to the methoxyl group (4dd and 4ed) in this transformation. The structure of ortho-selective products was also determined by single-crystal X-ray crystallography of 4al.23
Finally, we also studied the ortho C–H bond functionalization of 2-naphthols and the corresponding ortho-selective products were obtained in 77% to 99% yields in the presence of IPrAuCl and NaBArF (Table 3). Furthermore, the late-stage modification of naproxen was achieved, which is an important nonsteroidal anti-inflammatory drug (Table 3, 7k, 87%). We found that the steric hindrance played a key role in the late-stage modification of naproxen, but we could not obtain the desired product when more bulky isopropyl 2-diazo-2-phenyl-acetate was used. This result could also rationalize why C–H bond functionalization did not take place at the ortho position to the methoxyl group in the aforementioned cases (4dd and 4ed). It should be mentioned that all reactions had shown high chemo- and site-selectivity, and no O–H insertion product and other regioisomers via C–H bond functionalization were detected.
Table 3
Ortho-C–H functionalization of 2-naphthols
To our delight, this regiodivergent transformation was easy to scale-up to a gram-scale with even 0.5 mol% catalyst loading, affording the desired products 3ac (1.76 g, 92%) and 4ac (1.81 g, 94%), respectively. Moreover, C–H bond functionalization products could serve as versatile synthons. For example, a series of different groups could be incorporated into the phenyl ring via coupling reactions, such as phenyl groups (8b, 86%), alkynyl groups (8c, 91%) and ester groups (8d, 74%). The bromination of 3ac afforded 8e in 97% yield. The reduction of 3ac with LiAlH4 led to an alcohol (8f, 97%). TFA-catalyzed lactonization of 4ac could deliver synthetically valuable lactone 9 in 99% yield, whose structure was also confirmed by single-crystal X-ray diffraction analysis (Scheme 3).23
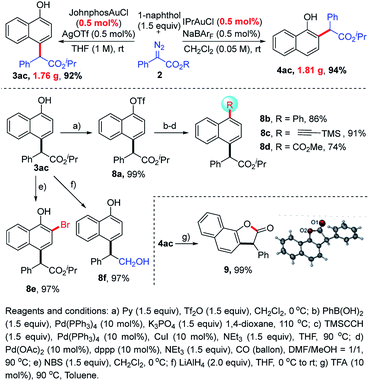 |
| Scheme 3 Gram-scale reactions and synthetic applications. | |
Furthermore, an array of related control experiments were then performed to gain insight into the reaction mechanism. First, we wanted to know whether a weak coordination interaction between the hydroxy group and gold catalyst played a key role in ortho-selectivity or not.24 Treatment of 1-methoxynaphthalene with the diazo compound only delivered para-selective C–H bond functionalization products (Scheme 4, eqn (1)), indicating that the ortho-selectivity indeed arose from the weak interaction between the hydroxy group and gold catalyst. Meanwhile, the 31P-NMR titration experiments (Scheme 4, eqn (2)) had shown that a new species was generated when PPh3AuOTf was mixed with 1-naphthol, disclosing that the gold catalyst could ligate to the hydroxy group. Besides, NMR titration experiments disclosed that no hydrogen bonding interactions existed between 1-naphthol and the gold catalyst (see the ESI†). On the other hand, further 19F-NMR and 11B-NMR experiments showed that there was no weak interactions between the hydroxyl group and boron atom (see the ESI†). Based on the above results, it is reasonable that the coordination between the hydroxy group and gold catalyst played a key role in ortho-selectivity. Further studies showed that a low concentration would facilitate ortho C–H bond functionalization, which was consistent with our conclusion that ortho C–H bond functionalization was a weak interaction enabled intramolecular process (Scheme 4, eqn (3)).24 Later on, deuterium labeling experiments disclosed that the C–H bond functionalization of 1-naphthol did not proceeded via a [1,2]-H shift25 but a possible water assisted [1,3]-H shift9g (Scheme 4, eqn (4) and (5)). Control experiments also demonstrated that no enolization of the product took place (Scheme 4, eqn (6)). Further experiments indicated that the C–H bond functionalization of 2-naphthols also proceeded via a similar process (see the ESI†). Besides, we also studied the silver effect20,26 and the results indicated that there is no silver effect in para C–H bond functionalization (Scheme 4, eqn (7)).
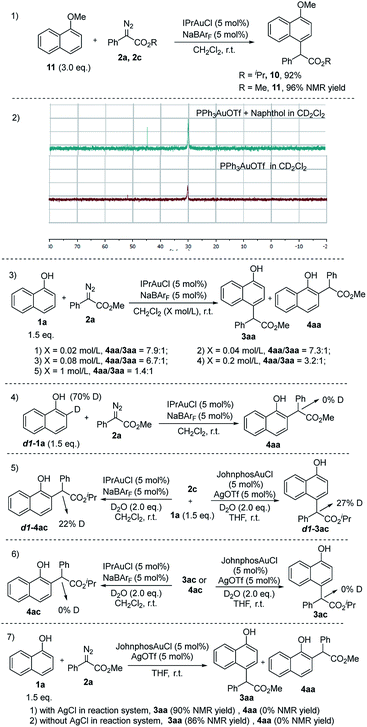 |
| Scheme 4 Preliminary mechanistic study. | |
In conclusion, we have described the first unprecedented and efficient example of gold-catalyzed regiodivergent and chemoselective direct C–H bond functionalization of naphthols with α-aryl-α-diazoesters, which has potential applications in the synthesis of bioactive compounds, natural products and late-stage modification of medicines containing a naphthol skeleton. Our research demonstrated that the control of the interactions between the hydroxy group and gold catalyst can enable the site-selectivities of this reaction. This work, we believe, would not only deepen the understanding of carbene chemistry, but also open a new window to directed C–H bond functionalization.
Conflicts of interest
There are no conflicts to declare.
Acknowledgements
We are grateful to the National Natural Science Foundation of China (No. 21572065, 21772042, 21425205), the Science and Technology Commission of Shanghai Municipality (18JC1412300) and the Program for Changjiang Scholars and Innovative Research Team in University (IRT_16R25) for financial support.
Notes and references
-
(a) B. M. Trost, Science, 1983, 219, 245–250 CrossRef CAS;
(b) S. L. Schreiber, Science, 2000, 287, 1964–1969 CrossRef CAS;
(c) J. Mahatthananchai, A. M. Dumas and J. W. Bode, Angew. Chem., Int. Ed., 2012, 51, 10954–10990 CrossRef CAS PubMed;
(d) W. R. J. D. Galloway, A. Isidro-Llobet and D. R. Spring, Nat. Commun., 2010, 1, 80 CrossRef PubMed.
- For recent examples, see:
(a) C. Guo, M. Fleige, D. Janssen-Müller, C. G. Daniliuc and F. Glorius, Nat. Chem., 2015, 7, 842–847 CrossRef CAS PubMed;
(b) R. Mose, G. Preegel, J. Larsen, S. Jakobsen, E. H. Iversen and K. A. Jørgensen, Nat. Chem., 2016, 9, 487–492 CrossRef PubMed;
(c) Z. Zhou, Z.-X. Wang, Y.-C. Zhou, W. Xiao, Q. Ouyang, W. Du and Y.-C. Chen, Nat. Chem., 2017, 9, 590–594 CrossRef CAS PubMed;
(d) Q.-Q. Cheng, J. Yedoyan, H. Arman and M. P. Doyle, J. Am. Chem. Soc., 2016, 138, 44–47 CrossRef CAS PubMed;
(e) Q.-Q. Cheng, M. Lankelma, D. Wherritt, H. Arman and M. P. Doyle, J. Am. Chem. Soc., 2017, 139, 9839–9842 CrossRef CAS;
(f) Y. Deng, L. A. Massey, Y. A. Rodriguez Núñez, H. Arman and M. P. Doyle, Angew. Chem., Int. Ed., 2017, 56, 12292 CrossRef CAS;
(g) J.-Y. Liao, P.-L. Shao and Y. Zhao, J. Am. Chem. Soc., 2015, 137, 628–631 CrossRef CAS;
(h) P. A. Donets and N. Cramer, Angew. Chem., Int. Ed., 2015, 54, 633 CAS;
(i) T. Hashimoto, H. Nakatsu and K. Maruoka, Angew. Chem., Int. Ed., 2015, 54, 4617–4621 CrossRef CAS PubMed;
(j) D. Ding, T. Mou, M. Feng and X. Jiang, J. Am. Chem. Soc., 2016, 138, 5218–5221 CrossRef CAS PubMed;
(k) S. Okumura, T. Komine, E. Shigeki, K. Semba and Y. Nakao, Angew. Chem., Int. Ed., 2018, 57, 929–932 CrossRef CAS PubMed;
(l) S. Xu, Z.-M. Zhang, B. Xu, B. Liu, Y. Liu and J. Zhang, J. Am. Chem. Soc., 2018, 140, 2278–2283 Search PubMed;
(m) L. Dell'Amic, G. Rassu, V. Zambrano, A. Sartori, C. Curti, L. Battistini, G. Pelosi, G. Casiraghi and F. Zanardi, J. Am. Chem. Soc., 2014, 136, 11107–11114 CrossRef PubMed;
(n) L. Liu and J. Zhang, Angew. Chem., Int. Ed., 2009, 48, 6093–6096 CrossRef CAS PubMed;
(o) C. Guo, B. Sahoo, C. G. Daniliuc and F. Glorius, J. Am. Chem. Soc., 2014, 136, 17402–17405 CrossRef CAS PubMed.
- For selected reviews, see:
(a) C. Cheng and J. F. Hartwig, Chem. Rev., 2015, 115, 8946–8975 CrossRef CAS PubMed;
(b) G. Song and X. Li, Acc. Chem. Res., 2015, 48, 1007–1020 CrossRef CAS PubMed;
(c) B. Ye and N. Cramer, Acc. Chem. Res., 2015, 48, 1308–1318 CrossRef CAS PubMed;
(d) K. Shin, H. Kim and S. Chang, Acc. Chem. Res., 2015, 48, 1040–1052 CrossRef CAS PubMed;
(e) O. Daugulis, J. Roane and L. D. Tran, Acc. Chem. Res., 2015, 48, 1053–1064 CrossRef CAS PubMed;
(f) Y. Segawa, T. Maekawa and K. Itami, Angew. Chem., Int. Ed., 2015, 54, 66–81 CrossRef CAS PubMed;
(g) T. W. Lyons and M. S. Sanford, Chem. Rev., 2010, 110, 1147–1169 CrossRef CAS PubMed;
(h) D. A. Colby, R. G. Bergman and J. A. Ellman, Chem. Rev., 2010, 110, 624–655 CrossRef CAS PubMed;
(i) P. B. Arockiam, C. Bruneau and P. H. Dixneuf, Chem. Rev., 2012, 112, 5879–5918 CrossRef CAS PubMed;
(j) B.-J. Li and Z.-J. Shi, Chem. Soc. Rev., 2012, 41, 5588–5598 RSC;
(k) K. M. Engle, T.-S. Mei, M. Wasa and J.-Q. Yu, Acc. Chem. Res., 2011, 45, 788–802 CrossRef PubMed.
- For selected meta-selective C–H functionalization, see:
(a) D. Leow, G. Li, T.-S. Mei and J.-Q. Yu, Nature, 2012, 486, 518–522 CrossRef CAS PubMed;
(b) L. Wan, N. Dastbaravardeh, G. Li and J.-Q. Yu, J. Am. Chem. Soc., 2013, 135, 18056–18059 CrossRef CAS PubMed;
(c) N. Hofmann and L. Ackermann, J. Am. Chem. Soc., 2013, 135, 5877–5884 CrossRef CAS PubMed;
(d) Y. Kuninobu, H. Ida, M. Nishi and M. Kanai, Nat. Chem., 2015, 7, 712–717 CrossRef CAS PubMed;
(e) X.-C. Wang, W. Gong, L.-Z. Fang, R.-Y. Zhu, S. Li, K. M. Engle and J.-Q. Yu, Nature, 2015, 519, 334–338 CrossRef CAS PubMed;
(f) Z. Dong, J. Wang and G. Dong, J. Am. Chem. Soc., 2015, 137, 5887–5890 CrossRef CAS PubMed;
(g) Z. Fan, J. Ni and A. Zhang, J. Am. Chem. Soc., 2016, 138, 8470–8475 CrossRef CAS PubMed.
- For para-selective C–H functionalization, see:
(a) S. Bag, T. Patra, A. Modak, A. Deb, S. Maity, U. Dutta, A. Dey, R. Kancherla, A. Maji, A. Hazra, M. Bera and D. Maiti, J. Am. Chem. Soc., 2015, 137, 11888–11891 CrossRef CAS PubMed;
(b) T. Patra, S. Bag, R. Kancherla, A. Mondal, A. Dey, S. Pimparkar, S. Agasti, A. Modak and D. Maiti, Angew. Chem., Int. Ed., 2016, 55, 7751–7755 CrossRef CAS PubMed;
(c) C. Yuan, L. Zhu, R. Zeng, Y. Lan and Y. Zhao, Angew. Chem., Int. Ed., 2018, 57, 1277–1281 CrossRef CAS PubMed.
- J. F. Hartwig and M. A. Larsen, ACS Cent. Sci., 2016, 2, 281–292 CrossRef CAS PubMed.
-
(a)
F. H. Fiege, H.-W. Voges, T. Hamamoto, S. Umemura; T. Iwata, H. Miki, Y. Fujita, H.-J. Buysch, D. Garbe and W. Paulus, Phenol derivatives, in Ullmann's Encyclopedia of Industrial Chemistry, Wiley VCH, Weinheim, 2000 Search PubMed;
(b) J. M. Brunel, Chem. Rev., 2005, 105, 857–897 CrossRef CAS PubMed;
(c) B. Das, C. R. Reddy, J. Kashanna, S. K. Mamidyala and C. G. Kumar, Med. Chem. Res., 2012, 21, 3321–3325 CrossRef CAS;
(d) I. Roufos, S. J. Hays, J. D. J. Dooley, R. D. Schwarz, G. W. Campbell and A. W. Probert Jr, J. Med. Chem., 1994, 37, 268–274 CrossRef CAS;
(e) D. G. Batt, G. D. Maynard, J. J. Petraitis, J. E. Shaw, W. Galbraith and R. R. Harris, J. Med. Chem., 1990, 33, 360–370 CrossRef CAS;
(f) R. J. Altenbach, L. A. Black, M. I. Strakhova, A. M. Manelli, T. L. Carr, K. C. Marsh, J. M. Wetter, E. J. Wensink, G. C. Hsieh, P. Honore, T. R. Garrison, J. D. Brioni and M. D. Cowart, J. Med. Chem., 2010, 53, 7869–7873 CrossRef CAS PubMed;
(g) E. S. Tan, M. Miyakawa, J. R. Bunzow, D. K. Grandy and T. S. Scanlan, J. Med. Chem., 2007, 50, 2787–2798 CrossRef CAS PubMed.
- Y. Xi, Y. Su, Z. Yu, B. Dong, E. J. McClain, Y. Lan and X. Shi, Angew. Chem., Int. Ed., 2014, 53, 9817–9821 CrossRef CAS PubMed.
-
(a) Z. Yu, B. Ma, M. Chen, H.-H. Wu, L. Liu and J. Zhang, J. Am. Chem. Soc., 2014, 136, 6904–6907 CrossRef CAS PubMed;
(b) Z. Yu, Y. Li, J. Shi, B. Ma, L. Liu and J. Zhang, Angew. Chem., Int. Ed., 2016, 55, 14807–14811 CrossRef CAS PubMed;
(c) Z. Yu, H. Qiu, L. Liu and J. Zhang, Chem. Commun., 2016, 52, 2257–2260 RSC;
(d) B. Ma, Z. Wu, B. Huang, L. Liu and J. Zhang, Chem. Commun., 2016, 52, 9351–9354 RSC;
(e) B. Ma, Z. Chu, B. Huang, Z. Liu, L. Liu and J. Zhang, Angew. Chem., Int. Ed., 2017, 56, 2749–2753 CrossRef CAS PubMed;
(f) B. Ma, J. Wu, L. Liu and J. Zhang, Chem. Commun., 2017, 53, 10164–10167 RSC;
(g) Y. Liu, Z. Yu, J. Z. Zhang, L. Liu, F. Xia and J. Zhang, Chem. Sci., 2016, 7, 1988–1995 RSC.
- For selected reviews, see:
(a)
M. P. Doyle and M. A. McKervey, in Modern Catalytic Methods for Organic Synthesis with Diazo Compounds, Wiley, New York, 1998 Search PubMed;
(b) M. P. Doyle, R. Duffy, M. Ratnikov and L. Zhou, Chem. Rev., 2010, 110, 704–724 CrossRef CAS PubMed;
(c) H. M. L. Davies and Y.-J. Lian, Acc. Chem. Res., 2012, 45, 923–935 CrossRef CAS PubMed;
(d) H. M. L. Davies and D. Morton, Chem. Soc. Rev., 2011, 40, 1857–1869 RSC;
(e) M. M. Díaz-Requejo and P. J. Pérez, Chem. Rev., 2008, 108, 3379–3394 CrossRef PubMed;
(f) T. Ye and M. A. McKervey, Chem. Rev., 1994, 94, 1091–1160 CrossRef CAS;
(g) A. Ford, H. Miel, A. Ring, C. N. Slattery, A. R. Maguire and M. A. McKervey, Chem. Rev., 2015, 115, 9981 CrossRef CAS PubMed;
(h) Y. Xia, D. Qiu and J. Wang, Chem. Rev., 2017, 117, 13810–13889 CrossRef CAS PubMed.
- For selected examples for aromatic C(sp2)–H bond functionalization with diazo compounds, see:
(a) W.-W. Chan, S.-F. Lo, Z. Zhou and W.-Y. Yu, J. Am. Chem. Soc., 2012, 134, 13565–13568 CrossRef CAS PubMed;
(b) T. K. Hyster, K. E. Ruhl and T. Rovis, J. Am. Chem. Soc., 2013, 135, 5364–5367 CrossRef CAS PubMed;
(c) H. Qiu, M. Li, L.-Q. Jiang, F.-P. Lv, L. Zan, C.-W. Zhai, M. P. Doyle and W.-H. Hu, Nat. Chem., 2012, 4, 733–738 CrossRef CAS PubMed;
(d) B. Xu, M.-L. Li, X.-D. Zuo, S.-F. Zhu and Q.-L. Zhou, J. Am. Chem. Soc., 2015, 137, 8700–8703 CrossRef CAS PubMed;
(e) D. Best, D. J. Burns and H. W. Lam, Angew. Chem., Int. Ed., 2015, 54, 7410–7413 CrossRef CAS PubMed;
(f) A. Conde, G. Sabenya, M. Rodríguez, V. Postils, J. M. Luis, M. M. Díaz-Requejo, M. Costas and P. J. Pérez, Angew. Chem., Int. Ed., 2016, 55, 6530–6534 CrossRef CAS PubMed.
- For reviews, see:
(a) D. Gillingham and N. Fei, Chem. Soc. Rev., 2013, 42, 4918–4931 RSC;
(b) X. Guo and W. Hu, Acc. Chem. Res., 2013, 46, 2427–2440 CrossRef CAS PubMed;
(c) S.-F. Zhu and Q.-L. Zhou, Acc. Chem. Res., 2012, 45, 1365–1377 CrossRef CAS PubMed;
(d) D. J. Miller and C. J. Moody, Tetrahedron, 1995, 51, 10811–10843 CrossRef CASFor O–H insertion of 1-naphthol, see:
(e) C. Chen, S.-F. Zhu, B. Liu, L.-X. Wang and Q.-L. Zhou, J. Am. Chem. Soc., 2007, 129, 12616–12617 CrossRef CAS PubMed;
(f) T. Osako, D. Panichakul and Y. Uozumi, Org. Lett., 2012, 14, 194–197 CrossRef CAS PubMedFor O–H insertion of 2-naphthol, see:
(g) X.-L. Xie, S.-F. Zhu, J.-X. Guo, Y. Cai and Q.-L. Zhou, Angew. Chem., Int. Ed., 2014, 53, 2978–2981 CrossRef CAS PubMed;
(h) X. Gao, B. Wu, W.-X. Huang, M.-W. Chen and Y.-G. Zhou, Angew. Chem., Int. Ed., 2015, 54, 11956–11960 CrossRef CAS PubMed;
(i) Y. Zhang, Y. Yao, L. He, Y. Liu and L. Shi, Adv. Synth. Catal., 2017, 359, 2754–2761 CrossRef CAS.
- In general, para-substituted product is the major one when 1-naphthol is treated with electrophile without intermolecular weak interaction. The references please see:
(a) M. C. Carreño, J. L. G. Ruano, G. Sanz, M. A. Toledo and A. Urbano, Synlett, 1997, 11, 1241–1242 CrossRef;
(b) P. Bovonsombat, J. Leykajarakul, C. Khan, K. Pla-on, M. M. Krause, P. Khanthapura, R. Ali and N. Doowa, Tetrahedron Lett., 2009, 50, 2664–2667 CrossRef CAS;
(c) H. Kajita and A. Togni, ChemistrySelect, 2017, 2, 1117–1121 CrossRef CAS.
- O. S. Tee and N. R. Yengar, Can. J. Chem., 1987, 65, 1714–1718 CrossRef CAS.
-
(a) M. Yamaguchi, A. Hayashi and M. Hirama, J. Am. Chem. Soc., 1995, 117, 1151–1152 CrossRef CAS;
(b) K. Kobayashi, M. Arisawa and M. Yamaguchi, J. Am. Chem. Soc., 2002, 124, 8528–8529 CrossRef CAS PubMed.
- D.-H. Lee, K.-H. Kwon and C. S. Yi, J. Am. Chem. Soc., 2012, 134, 7325–7328 CrossRef CAS PubMed.
- For selected recent general reviews, see:
(a)
A. S. K. Hashmi and F. D. Toste, Modern Gold Catalyzed Synthesis, Wiley-VCH, Weinheim, 2012 CrossRef;
(b) D. J. Gorin and F. D. Toste, Nature, 2007, 446, 395–403 CrossRef CAS PubMed;
(c) A. S. K. Hashmi, Chem. Rev., 2007, 107, 3180–3211 CrossRef CAS PubMed;
(d) Z. Li, C. Brouwer and C. He, Chem. Rev., 2008, 108, 3239–3265 CrossRef CAS PubMed;
(e) A. Arcadi, Chem. Rev., 2008, 108, 3266–3325 CrossRef CAS PubMed;
(f) E. Jiménez-Núñez and A. M. Echavarren, Chem. Rev., 2008, 108, 3326–3350 CrossRef PubMed;
(g) D. Garayalde and C. Nevado, ACS Catal., 2012, 2, 1462–1479 CrossRef CAS;
(h) N. Krause and C. Winter, Chem. Rev., 2011, 111, 1994–2009 CrossRef CAS PubMed;
(i) M. Bandini, Chem. Soc. Rev., 2011, 40, 1358–1367 RSC;
(j) L. Zhang, Acc. Chem. Res., 2014, 47, 877–888 CrossRef CAS PubMed;
(k) A. Fürstner, Acc. Chem. Res., 2014, 47, 925–938 CrossRef PubMed;
(l) L. Fensterbank and M. Malacria, Acc. Chem. Res., 2014, 47, 953–965 CrossRef CAS PubMed;
(m) R. Dorel and A. M. Echavarren, Chem. Rev., 2015, 115, 9028–9072 CrossRef CAS PubMed.
- For reviews and selected examples of gold-catalyzed transformations of diazoacetates, see:
(a) L. Liu and J. Zhang, Chem. Soc. Rev., 2016, 45, 506–516 RSC;
(b) F. Wei, C. Song, Y. Ma, L. Zhou, C.-H. Tung and Z. Xu, Sci. Bull., 2015, 60, 1479–1492 CrossRef CAS;
(c) M. R. Fructos, M. M. Díaz-Requejo and P. J. Pérez, Chem. Commun., 2016, 52, 7326–7335 RSC;
(d) M. R. Fructos, T. R. Belderrain, P. de Frémont, N. M. Scott, S. P. Nolan, M. M. Díaz-Requejo and P. J. Pérez, Angew. Chem., Int. Ed., 2005, 44, 5284–5288 CrossRef CAS PubMed;
(e) J. F. Briones and H. M. L. Davies, J. Am. Chem. Soc., 2012, 134, 11916–11919 CrossRef CAS PubMed;
(f) J. F. Briones and H. M. L. Davies, J. Am. Chem. Soc., 2013, 135, 13314–13317 CrossRef CAS PubMed;
(g) D. Zhang, G. Xu, D. Ding, C. Zhu, J. Li and J. Sun, Angew. Chem., Int. Ed., 2014, 53, 11070–11074 CrossRef CAS PubMed;
(h) G. Lonzi and L. A. López, Adv. Synth. Catal., 2013, 355, 1948–1954 CrossRef CAS;
(i) J. Wang, X. Yao, T. Wang, J. Han, J. Zhang, X. Zhang, P. Wang and Z. Zhang, Org. Lett., 2015, 17, 5124–5127 CrossRef CAS PubMed;
(j) Z. Cao, X. Wang, C. Tan, X. Zhao, J. Zhou and K. Ding, J. Am. Chem. Soc., 2013, 135, 8197–8200 CrossRef CAS PubMed;
(k) V. V. Pagar and R.-S. Liu, Angew. Chem., Int. Ed., 2015, 54, 4923–4926 CrossRef CAS PubMed;
(l) F. Zhao, N. Li, T. Zhang, Z.-Y. Han, S.-W. Luo and L.-Z. Gong, Angew. Chem., Int. Ed., 2017, 56, 3247–3251 CrossRef CAS PubMed;
(m) F.-M. Liao, Z.-Y. Cao, J.-S. Yu and J. Zhou, Angew. Chem., Int. Ed., 2017, 56, 2459–2463 CrossRef CAS PubMed.
- For a review, see: R. E. M. Brooner and R. A. Widenhoefer, Angew. Chem., Int. Ed., 2013, 52, 11714–11724 CrossRef CAS PubMed.
- A. Homs, I. Escofet and A. M. Echavarren, Org. Lett., 2013, 15, 5782–5785 CrossRef CAS PubMed.
- N. Huguet, D. Leboeuf and A. M. Echavarren, Chem.–Eur. J., 2013, 19, 6581–6585 CrossRef CAS PubMed.
-
(a) D. Bykowski, K.-H. Wu and M. P. Doyle, J. Am. Chem. Soc., 2006, 128, 16038–16039 CrossRef CAS PubMed;
(b) S. Chuprakov, S. W. Kwok, L. Zhang, L. Lercher and V. V. Fokin, J. Am. Chem. Soc., 2009, 131, 18034–18035 CrossRef CAS PubMed;
(c) V. N. G. Lindsay, D. Fiset, P. J. Gristch, S. Azzi and A. B. Charette, J. Am. Chem. Soc., 2013, 135, 1463–1470 CrossRef CAS PubMed;
(d) X. Xu, H.-J. Lu, J. V. Ruppel, X. Cui, S. L. Mesa, L. Wojtas and X. P. Zhang, J. Am. Chem. Soc., 2011, 133, 15292–15295 CrossRef CAS PubMed;
(e) Y. Wang, X. Wen, X. Cui, L. Wojtas and X. P. Zhang, J. Am. Chem. Soc., 2017, 139, 1049–1052 CrossRef CAS PubMed.
- CCDC 1822536 (3ah), 1884847 (4al), 1822537 (7i), 1822538 (9).†.
- The references on tri-coordinated Au(I) complexes:
(a) M. C. Gimeno and A. Laguna, Chem. Rev., 1997, 97, 511–522 CrossRef CAS PubMed;
(b) C. Khin, A. S. K. Hashmi and F. Rominger, Eur. J. Inorg. Chem., 2010, 1063–1069 CrossRef CAS;
(c) Y. Luo, K. Ji, Y. Li and L. Zhang, J. Am. Chem. Soc., 2012, 134, 17412–17415 CrossRef CAS PubMed;
(d) P. Gualco, S. Mallet-Ladeira, H. Kameo, H. Nakazawa, M. Mercy, L. Maron, A. Amgoune and D. Bourissou, Organometallics, 2015, 34, 1449–1453 CrossRef CAS;
(e) H. Yang and F. P. Gabbaï, J. Am. Chem. Soc., 2015, 137, 13425–13432 CrossRef CAS PubMed.
- A. Padwa, D. J. Austin, A. T. Price, M. A. Semones, M. P. Doyle, M. N. Protopopova, W. R. Winchester and A. Tran, J. Am. Chem. Soc., 1993, 115, 8669–8680 CrossRef CAS.
- D. Wang, R. Cai, S. Sharma, J. Jirak, S. K. Thummanapelli, N. G. Akhmedov, H. Zhang, X. Liu, J. L. Petersen and X. Shi, J. Am. Chem. Soc., 2012, 134, 9012–9019 CrossRef CAS PubMed.
Footnote |
† Electronic supplementary information (ESI) available: Data for new compounds, experimental procedures and theoretical studies on mechanisms. CCDC 1822536–1822538, 1884847. For ESI and crystallographic data in CIF or other electronic format see DOI: 10.1039/c9sc01657k |
|
This journal is © The Royal Society of Chemistry 2019 |
Click here to see how this site uses Cookies. View our privacy policy here.