DOI:
10.1039/C8SC04996C
(Edge Article)
Chem. Sci., 2019,
10, 2124-2129
Direct N–O bond formation via oxidation of amines with benzoyl peroxide†
Received
9th November 2018
, Accepted 11th December 2018
First published on 12th December 2018
Introduction
Despite the prevalence of nitrogen–oxygen bonds in biologically active molecules and natural products, methods that form these N–O bonds remain rare.1 Recently, both industry and academia have paid significant attention to the N–O bond formation because of its synthetic utility.2 Moreover, compounds containing the N–O bond can be useful synthetic intermediates such as N-oxides, hydroxyl amines, hydroxamic acids etc. Whereas tertiary N–O bond synthesis (N-oxide) from tertiary amines using oxidants are well studied,3 very few contributions have been made for primary and secondary N–O bond construction directly from amines which often suffer from competition from N–O vs. C–N bond formation. Significantly, direct construction of the N–O bond from amines provides an attractive approach to access hydroxamic acids and hydroxyl amines.4
Hydroxamic acid and its derivatives are an important class of biologically active molecules, principally known as strong polyfunctional metal ion chelators as they possess a wide range of biological activities such as antibacterial, antifungal, anti-inflammatory and anti-asthmatic behavior5a and have been used in the design of therapeutic agents for cancer,5b,c Alzheimer's disease,5d haemochromatosis5e and malaria.5f Additionally, hydroxamic acids are vastly utilized as excellent ligands in synthetic organic chemistry.6 Interestingly, N-(benzoyloxy)amines (hydroxyl amine precursor) are known to serve as key intermediates in the synthesis of amines (secondary and tertiary), amides, hydroxamic acids etc.7
Meanwhile, it is well known that hydroxyl amine is the best source of reactive nitroso (N–O bond) intermediates8 and for a decade we have used these intermediates to synthesize various new types of N-selective (C–N–O) as well as O-selective (C–O–N) bond-containing synthetically useful chiral compounds as well as bioactive compounds.9 We are eager to investigate if N–O bond formation could be achieved by choosing suitable reaction conditions using amines and benzoyl peroxide.
Very few reliable methods are known for N–O bond formation resulting in N-(benzoyloxy)amines in moderate yields directly from amines using benzoyl peroxide (BPO).10 However, most of the methods suffer from undesirable C–N bond (amide side product) formation, especially in the case of primary amines, which ultimately reduces the efficiency of the overall process (Scheme 1A).10a–c Bis-hydroxamic acids (BHA) are extremely important molecules as chiral building blocks and chiral ligands in modern asymmetric catalysis.11 Unfortunately, the direct synthesis of BHAs also suffers from serious problems from the above side reactions (Scheme 1B). A nucleophilic amine is able to react with BPO at both the peroxide oxygen and at the carbonyl centre, and both are almost equally reactive. The C–N bond (amide) is, however, more stable than the N–O bond (N–OBz). The carbonyl group in N–OBz is active enough to react with another amine leading to competition between intramolecular C–N bond vs. intermolecular N–O bond formation with another equivalent of BPO. The thermodynamically favourable intramolecular reaction leads to 4 as a major product. However, known mono-amine oxidation methods provide poor results (Scheme 1B). Thus, in order to improve the efficiency, controlling the reaction conditions to minimize side products remains a synthetic challenge.
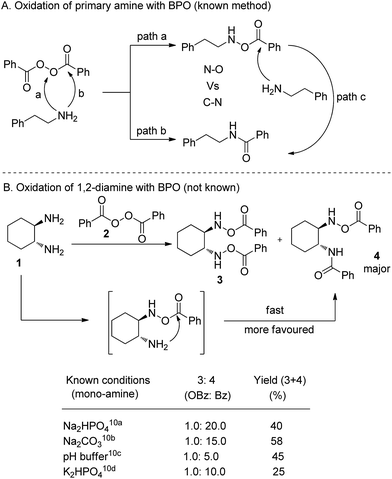 |
| Scheme 1 Inherent problems associated with the oxidation of amines with benzoyl peroxide. | |
Previously, we introduced bis-hydroxamic acids as versatile chiral ligands for several asymmetric epoxidations of olefins to give synthetically challenging chiral epoxy alcohols and asymmetric ring opening of epoxides.12 Although epoxy alcohols are synthesized with an excellent yield (up to 99%) and enantiopurity (ee up to >99%), the ligand synthesis suffers from multistep and time consuming processes (for a detailed scheme see ESI Section 7†).12a,b Thus our current interest is to develop a general and efficient method for the synthesis of bis-hydroxamic acids and bis-hydroxyl amines. Following a retrosynthetic approach bis-hydroxamic acids and bis-hydroxyl amines could be achieved directly from the challenging bis-(benzoyloxy)-1,2-diamines (Fig. 1). Herein we report, for the first time, oxidation of 1,2-diamines with BPO to give bis(benzoyloxy)-1,2-diamines with a very good yield and selectivity. As far as we are aware, this oxidation process is unprecedented in product scope as there are no previous reports on the formation of bis(benzoyloxy)-1,2-diamines from 1,2-diamines.
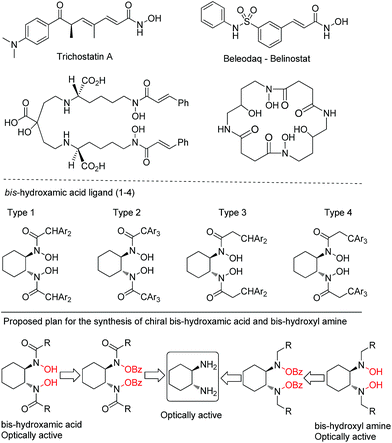 |
| Fig. 1 Biologically and synthetically useful hydroxamic acid derivatives. | |
Results and discussion
We began our investigation using commercially available benzoyl peroxide and trans-1,2-diaminocyclohexane as a model substrate and we decided to alter various parameters to optimize the reaction conditions. Preliminary studies showed that a base that removes the produced benzoic acid in the reaction mixture is necessary to improve the yield of the product. Initially, we chose K2CO3 (4.0 equiv.) as a base and tested it with recrystallized BPO (3.0 equiv.) in various anhydrous solvents which resulted in a moderate yield (<50%) of the product but mostly amide 4 as a major product (OBz
:
Bz = 1.0
:
10.0). Further investigation showed that the presence of a small amount of water is very crucial to improve the yield and selectivity (see the ESI†). From these studies we found that a ratio of BPO
:
water = 3
:
1 is necessary to achieve good results. Interestingly, when we used commercially available 75% BPO (Aldrich, remaining 25% water) in combination with K2CO3, a sharp improvement in yield (88% for CHCl3 and 82% for CH2Cl2) and selectivity (OBz
:
Bz = 1.0
:
1.2) was observed. Further, we tested various metal carbonates (4.0 equiv.) but only Cs2CO3 provided better results with promising selectivity (OBz
:
Bz = 5
:
1) and yield (90%). Then we tested various equivalents of Cs2CO3 to achieve high yield and selectivity. To our delight, when the amine solution (in 2 mL CH2Cl2) was added to a stirred mixture of BPO and Cs2CO3 (Table 1, entry 4), we observed a sharp change in selectivity (OBz
:
Bz = 10
:
1) and yield (95% yield). Significantly, other metal carbonates failed to improve the selectivity and yield (entries 1–3, entries 5–8). Further, we also tested various cesium salts (entries 9–11) and phosphate salts (entries 12–15) but they failed to improve the results (see details in the ESI†).
Table 1 Optimization of oxidation of (±)-trans-1,2-cyclohexanediamine with BPOa

|
Entry |
Base |
3 : 4 (OBz : Bz)b |
Yield (3 + 4)b |
Reaction conditions: 1 (0.5 mmol, 1.0 equiv.), 2 (2.0 mmol, 4.0 equiv.), base (3.0 mmol, 6.0 equiv.), CH2Cl2 (7 mL), r.t., 16 h.
Determined by 1H NMR spectroscopy of the crude reaction mixture using 1,1′,2,2′-tetrachloroethane as an internal standard.
|
1 |
Li2CO3 |
1.0 : 10.0 |
30 |
2 |
Na2CO3 |
1.0 : 5.0 |
78 |
3 |
K2CO3 |
1.0 : 1.2 |
88 |
4
|
Cs
2
CO
3
|
10.0 : 1.0 |
95
|
5 |
Rb2CO3 |
1.0 : 2.0 |
70 |
6 |
CaCO3 |
1.0 : 15.0 |
20 |
7 |
MgCO3 |
1.0 : 6.0 |
30 |
8 |
BaCO3 |
1.0 : 10.0 |
25 |
9 |
CsOAc |
1.0 : 3.0 |
25 |
10 |
CsI |
— |
<5 |
11 |
CsNTf2 |
1.0 : 25.0 |
60 |
12 |
Na2HPO4 |
1.0 : 1.0 |
75 |
13 |
NaH2PO4 |
2.0 : 1.0 |
65 |
14 |
Mg3(PO4)2 |
3.0 : 1.0 |
80 |
15 |
K3PO4 |
1.0 : 2.0 |
60 |
Bis-(benzoyloxy)-1,2-diamine synthesis
After having the optimized conditions in hand, we then explored the substrate scope and the generality of this reaction. We were pleased to find that the high efficiency demonstrated by the model oxidation reaction also applied to a broad range of cyclic as well as acyclic 1,2-diamines. Cyclic and acyclic free diamines such as 1a–1f are good substrates for this reaction, delivering the desired products (3a–3f) in good yields (54 to 83%) and high selectivity (up to OBz
:
Bz = 10
:
1) (Table 2). Interestingly, cyclic as well as acyclic 1,2-disubstituted-1,2-diamine substrates are found to be excellent substrates with excellent selectivity. Substrates 3h, and 3k derived from (1R,2R)-(−)-1,2-diaminocyclohexane and substrates 3i, 3j and 3l derived from (1S,2S)-(+)-1,2-diaminocyclohexane were found to be excellent in terms of selectivity (OBz
:
Bz = 99
:
1) as a single isomer with good yields (41–65%). Furthermore, substrates 3m and 3n prepared from (1R,2R)-(+)-1,2-diphenylethylenediamine also provided excellent selectivity (OBz
:
Bz = 99
:
1) with good yields (76–79%). Interestingly, products 3h–3n could be a good precursor for a novel bis-hydroxyl amine ligand which is not known in the literature. Similarly, N,N′-disubstituted acyclic 1,2-diamines 3o–3v proved to be good substrates under the reaction conditions with good yields (70–80%) and excellent selectivity (OBz
:
Bz = 99
:
1) as a single product. The reaction proceeds with high stereoselectivity with no racemization. Additionally, the reaction can be carried out on more than a gram scale (see the ESI†).
Table 2 Substrate scope of oxidation of 1,2-diamines with benzoyl peroxidea

|
Reaction conditions: 1 (0.5 mmol, 1.0 equiv.), 2 (2.0 mmol, 4.0 equiv.), Cs2CO3 (3.0 mmol, 6.0 equiv.), CH2Cl2 (7 mL), r.t., 16 h.
Yield of the isolated product.
Determined by 1H NMR spectroscopy of the crude reaction mixture using 1,1′,2,2′-tetrachloroethane as an internal standard.
|
|
One pot bis-(benzoyloxy)hydroxamic acid synthesis
After synthesizing a large number of bis-(benzoyloxy)-1,2-diamines, we then focused on the efficient synthesis of bis-(benzoyloxy)hydroxamic acids in one pot. In practice, synthesis of bis-(benzoyloxy)hydroxamic acids from bis-(benzoyloxy)-1,2-diamines is not easy and it requires additional steps using an appropriate base and acyl derivative. According to our plan, after completion of the first step before work-up we added acyl chloride (in CH2Cl2) and stirred for another 6 h at room temperature followed by work-up giving the crude product. The pure bis-(benzoyloxy)hydroxamic acids 5a–5h were achieved with very high yields (70–81%) in one pot without using further base and extra steps (Table 3).
Table 3 One pot synthesis of bis-(benzoyloxy)hydroxamic acida
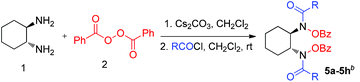
|
Reaction conditions: 1 (0.5 mmol, 1.0 equiv.), 2 (1.0 mmol, 2.0 equiv.), Cs2CO3 (1.5 mmol, 3.0 equiv.), CH2Cl2 (7 mL), r.t., 16 h.
Yield of the isolated product.
|
|
N-Benzoyloxyamine synthesis
After exploring the model reaction, oxidation of 1,2-diamines to bis-(benzoyloxy)hydroxyl amines and bis-(benzoyloxy)hydroxamic acids with a wide substrate scope, we then wanted to test this oxidation method for mono-amine substrates. Interestingly, when we treated simple commercially available 6a (1.0 equiv.) under similar reaction conditions such as treating with BPO (2.0 equiv.) and Cs2CO3 (3.0 equiv.), we observed an excellent yield (95%) of the product 7a with excellent selectivity (N–O
:
C–N = 98
:
2). This inspiring result led us to test the reactivity of other amines (Table 4). Cyclic amines 6a–6d provide very good yields (85–95%) of the products (7a–7d) with good to excellent selectivity (up to N–O
:
C–N = 98
:
02). In order to test the stereochemical integrity, we carried out HPLC analysis of chiral and racemic 7e. As expected no racemization of product 7e was observed (for details see the ESI†). Further acyclic primary amines such as aromatic, aliphatic and allyl substituted amines furnished products 7f–7j in good yields (80–92%) and good selectivity (up to N–O
:
C–N = >25
:
1). The substrate 7g provided almost a single product (N–O
:
C–N = 25
:
1) with 92% yield, a result better than that reported in the literature (60% yield, N–O
:
C–N = 5.4
:
1).10c Further, tert-butylamine 6h as well as secondary amines 6k–6o provide excellent selectivity (N–O
:
C–N = >99
:
1) as a single product with very high yields (85–92%).13 In addition, we have tested some mono-amines with a known method in ref. 10d (the only method for which no amide side product was observed) and compared with the results of our reaction conditions which clearly shows that our method is better than any known method in terms of selectivity and yield (for detailed results see the ESI†).
Table 4 Oxidation of mono-amines with benzoyl peroxide and Cs2CO3a

|
Reaction conditions: 1 (0.5 mmol, 1.0 equiv.), 2 (1.0 mmol, 2.0 equiv.), Cs2CO3 (1.5 mmol, 3.0 equiv.), CH2Cl2 (7 mL), r.t., 16 h.
Yield of the isolated product.
Determined by 1H NMR spectroscopy of the crude reaction mixture using 1,1′,2,2′-tetrachloroethane as an internal standard.
|
|
Applications
So far, we have shown a broad substrate scope for our oxidation method from a variety of 1,2-diamines and monoamines and all these products are good precursors for various synthetically useful intermediates and building blocks. From that aspect we wanted to show some applications of the products because on hydrolysis of N–OBz to N–OH valuable compounds such as hydroxylamines and hydroxamic acids are afforded. We found that LiOH (2.0 equiv.) in MeOH solvent is the best reagent for the hydrolysis of the bis-O-benzoyl group. Thus, 5g on hydrolysis with LiOH/MeOH gave bis-hydroxamic acid 9A in 99% yield (Scheme 2a) whereas 7b′ gave hydroxamic acid 9B in 99% yield (Scheme 2b). Similarly, 3l on hydrolysis gave bis-(hydroxy)-1,2-diamine 9C which is also a new chiral ligand (Scheme 2c) whereas 7b gave hydroxyl amine 9D in 95% yield (Scheme 2d). The application of this method was further extended to the short synthesis of the highly useful chiral BHA ligand 9F in high yield with retention of configuration. The spectroscopic data were in good accordance with the data reported previously by our group.11b
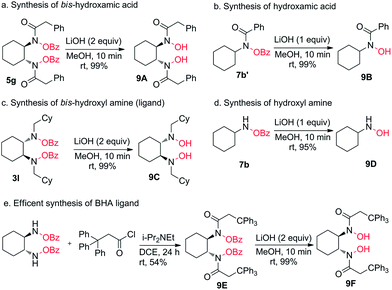 |
| Scheme 2 Application of N-(benzoyloxy)amine derivatives. | |
Conclusions
In summary, we have developed for the first time a general and efficient method for N–O bond synthesis from 1,2-diamines by eliminating undesirable amide (C–N bond) formation in most cases. The oxidation of 1,2-diamines using commercially available 75% BPO and Cs2CO3 furnished the challenging bis-(benzoyloxy)-1,2-diamines with a good yield (up to 85%). The presence of a small amount of water and Cs2CO3 plays a crucial role in achieving high selectivity. The method was successfully explored in the efficient synthesis of bis-(benzoyloxy)hydroxamic acids and bis-hydroxamic acids. Further, this method was extended to the efficient synthesis of bis-(benzoyloxy)-1,2-disubstituted-1,2-diamines and a new type of bis-hydroxyl amine ligand. Excitingly, all of these products can be transformed to a variety of building blocks and chiral ligands, some of them are shown. The reaction proceeds stereoselectively without any racemization. The synthetic utility of this methodology was further demonstrated in the efficient synthesis of a chiral BHA ligand. Currently, a detailed mechanistic study and synthetic application to biologically active molecules is under progress in our laboratory. We believe that the present method will be able to provide the direct synthesis of chiral bis-hydroxyl amines and bis-hydroxamic acids for future use.
Conflicts of interest
There are no conflicts to declare.
Acknowledgements
This work was supported by Grant-in-Aid for Scientific Research (No. 17H06142).
Notes and references
-
(a) D. Enders and E. Schaumann, Sci. Synth., 2009, 40, 1 Search PubMed
;
(b)
M. Katkevics, T. Kukosha and E. Lukevics, in The Chemistry of Hydroxylamines, Oximes and Hydroxamic Acids, ed. Z. Rappoport and J. F. Liebman, Wiley, 2011, vol. 2, pt 1, p. 205 Search PubMed
;
(c) D. Borovika, P. Bertrand and P. Trapencieris, Chem. Heterocycl. Compd., 2014, 49, 1560 CrossRef CAS
;
(d) T. Sakamoto, H. Li and Y. Kikugawa, J. Org. Chem., 1996, 61, 8496 CrossRef CAS
;
(e) T. Kurz, C. Behrendt, U. Kaula, B. Bergmann and R. D. Walter, Aust. J. Chem., 2007, 60, 154 CrossRef CAS
;
(f) T. Bugg, ChemBioChem, 2014, 15, 2467 CrossRef CAS
;
(g) J. H. Weisburger and E. K. Weisburger, Pharmacol. Rev., 1973, 25, 1 CAS
;
(h) A. Banan, H. Valizadeh, A. Heydari and A. Moghimi, Synlett, 2017, 28, 2315 CrossRef CAS
;
(i) N. E. Leadbeater and C. van der Pol, Chem. Commun., 2001, 599 RSC
;
(j) J. D. Fields and P. J. Kropp, J. Org. Chem., 2000, 65, 5937 CrossRef CAS
;
(k) Y.-L. Du, H.-Y. He, M. A. Higgins and K. S. Ryan, Nat. Chem. Biol., 2017, 13, 836 CrossRef CAS PubMed
;
(l) S. D. McCann, J.-P. Lumb, B. A. Arndtsen and S. S. Stahl, ACS Cent. Sci., 2017, 3, 314 CrossRef CAS PubMed
.
-
(a) M. H. Bickel, Xenobiotica, 1971, 1(4–5), 313 CrossRef CAS PubMed
;
(b) R. T. Coutts and A. H. Beckett, Drug Metab. Rev., 1977, 6(1), 51 CrossRef CAS
;
(c) T. A. Wencewicz, B. Yang, J. R. Rudloff, A. G. Oliver and M. J. Miller, J. Med. Chem., 2001, 54, 6843 CrossRef PubMed
;
(d) A. J. Rybarczyk-Pirek, M. Lukomska-Rogala, S. Wojtulewski and M. Palusiak, Cryst. Growth Des., 2015, 15, 5802 CrossRef CAS
;
(e) J. Yuan, X. Long and C. Zhang, J. Phys. Chem., 2016, 120, 9446 CrossRef CAS PubMed
;
(f) K. Murakami, Y. Sasano, M. Tomizawa, M. Shibuya, E. Kwon and Y. Iwabuchi, J. Am. Chem. Soc., 2014, 136, 17591 CrossRef CAS PubMed
;
(g) Y. Sasano, S. Nagasawa, M. Yamazaki, M. Shibuya, J. Park and Y. Iwabuchi, Angew. Chem., Int. Ed., 2014, 53, 3236–3240 CrossRef CAS PubMed
.
- For selected review:
(a) X. Cai, M. Sha, C. Guo and R. M. Pan, Asian J. Chem., 2012, 24, 3781 CAS
;
(b) D. Bernier, U. K. Wefelscheid and S. Woodward, Org. Prep. Proced. Int., 2009, 41, 173 CrossRef CAS
;
(c) S. K. Singh, M. Bajpai and V. K. Tyagi, J. Oleo Sci., 2006, 55, 99 CrossRef CAS
;
(d) A. M. Mfuh and O. V. Larionov, Curr. Med. Chem., 2015, 22, 2819 CrossRef CAS
.
- For selected review on hydroxamic acid:
(a) M. J. Miller, Chem. Rev., 1989, 89, 1563 CrossRef CAS
;
(b) E. Lipczynska-Kochany, Chem. Rev., 1991, 91, 477 CrossRef CAS
;
(c) E. Lipczynska-Kochany, Sci. Total Environ., 1991, 100, 469 CrossRef CAS PubMed
;
(d) M. J. Miller, Acc. Chem. Res., 1986, 19, 49 CrossRef CAS
;
(e) B. Kurzak, H. Kozlowski and E. Farkas, Coord. Chem. Rev., 1992, 114, 169 CrossRef CAS
. For selected review on hydroxyl amine: ;
(f) P. Gross and R. P. Smith, Crit. Rev. Toxicol., 1985, 14, 87 CrossRef CAS PubMed
;
(g) G. R. Tauszik and P. Crocetta, Appl. Catal., 1985, 17, 1 CrossRef CAS
.
-
(a) G. Weber, Cancer Res., 1983, 43, 3466 CAS
;
(b) W. P. Steward and A. L. Thomas, Expert Opin. Invest. Drugs, 2000, 9, 2913 CrossRef CAS PubMed
;
(c) W. P. Steward, Cancer Chemother. Pharmacol., 1999, 43(suppl.), S56 CrossRef CAS PubMed
;
(d) J. L. Domingo, Reprod. Toxicol., 1998, 12, 499 CrossRef CAS PubMed
;
(e) I. Turcot, A. Stintzi, J. Xu and K. N. Raymond, J. Biol. Inorg Chem., 2000, 5, 634 CrossRef CAS PubMed
;
(f) Z. I. Cabantchik, Parasitol. Today, 1995, 11, 74 CrossRef CAS
.
-
(a) A. G. J. Ligtenbarg, R. Hage and B. L. Feringa, Coord. Chem. Rev., 2003, 237, 89 CrossRef CAS
;
(b) C. Bolm, Coord. Chem. Rev., 2003, 237, 245 CrossRef CAS
; selected applications: ;
(c) A. V. Malkov, Z. Bourhani and P. Kočovský, Org. Biomol. Chem., 2005, 3, 3194 RSC
;
(d) Y. Hoshino, N. Murase, M. Oishi and H. Yamamoto, Bull. Chem. Soc. Jpn., 2000, 73, 1653 CrossRef CAS
.
- Selected application of N-benzoyloxy amine:
(a) O. Phanstiel IV, Q. X. Wang, D. H. Powell, M. P. Ospina and B. A. Leeson, J. Org. Chem., 1999, 64, 803 CrossRef
;
(b) A. Nemchik, V. Badescu and O. Phanstiel IV, Tetrahedron, 2003, 59, 4315 CrossRef CAS
;
(c) A. M. Berman and J. S. Johnson, J. Am. Chem. Soc., 2004, 126, 5680 CrossRef CAS PubMed
;
(d) J. S. Arora, N. Kaur and O. Phanstiel IV, J. Org. Chem., 2008, 73, 6182 CrossRef CAS PubMed
;
(e) N. Matsuda, K. Hirano, T. Satoh and M. Miura, Angew. Chem., Int. Ed., 2012, 51, 3642 CrossRef CAS PubMed
;
(f) R. P. Rucker, A. M. Whittaker, H. Dang and G. Lalic, J. Am. Chem. Soc., 2012, 134, 6571 CrossRef CAS PubMed
;
(g) R. P. Rucker, A. M. Whittaker, H. Dang and G. Lalic, Angew. Chem., Int. Ed., 2012, 51, 3953 CrossRef CAS PubMed
;
(h) Y.-H. Chen, S. Graßl and P. Knochel, Angew. Chem., Int. Ed., 2018, 57, 1108 CrossRef CAS PubMed
. Selected application of N-hydroxyl amine: ;
(i) N. Carrillo, E. A. Davalos, A. Russak and J. W. Bode, J. Am. Chem. Soc., 2006, 128, 1452 CrossRef CAS PubMed
;
(j) J. W. Bode, R. M. Fox and K. D. Baucom, Angew. Chem., Int. Ed., 2006, 45, 1248 CrossRef CAS
;
(k) K. Ohmatsu, Y. Ando, T. Nakashima and T. Ooi, Chem, 2016, 1, 802 CrossRef CAS
.
-
(a) H. Yamamoto and N. Momiyama, Chem. Commun., 2005, 3514 RSC
;
(b) W. Adam and O. Krebs, Chem. Rev., 2003, 103, 4131 CrossRef CAS
;
(c) M. Baidya and H. Yamamoto, Synthesis, 2013, 45, 1931 CrossRef CAS
;
(d) S. Dana, I. Ramakrishna and M. Baidya, Synthesis, 2017, 49, 3281 CrossRef CAS
.
- Selected examples:
(a) Y. Yamamoto, N. Momiyama and H. Yamamoto, J. Am. Chem. Soc., 2004, 126, 5962 CrossRef CAS PubMed
;
(b) Y. Yamamoto and H. Yamamoto, J. Am. Chem. Soc., 2004, 126, 4128 CrossRef CAS PubMed
;
(c) Y. Yamamoto, N. Momiyama and H. Yamamoto, J. Am. Chem. Soc., 2004, 126, 5962 CrossRef CAS PubMed
;
(d) Y. Yamamoto and H. Yamamoto, Angew. Chem., Int. Ed., 2005, 44, 7082 CrossRef CAS PubMed
;
(e) N. Momiyama, Y. Yamamoto and H. Yamamoto, J. Am. Chem. Soc., 2007, 129, 1190 CrossRef CAS PubMed
;
(f) M. Baidya and H. Yamamoto, J. Am. Chem. Soc., 2011, 133, 13880 CrossRef CAS
;
(g) M. Baidya, K. A. Griffin and H. Yamamoto, J. Am. Chem. Soc., 2012, 134, 18566 CrossRef CAS
;
(h) B. Maji and H. Yamamoto, Angew. Chem., Int. Ed., 2014, 53, 14472 CrossRef CAS
;
(i) B. Maji and H. Yamamoto, Angew. Chem., Int. Ed., 2014, 53, 8714 CrossRef CAS PubMed
;
(j) B. Maji, M. Baidya and H. Yamamoto, Chem. Sci., 2014, 5, 3941 RSC
;
(k) B. Maji and H. Yamamoto, J. Am. Chem. Soc., 2015, 137, 15957 CrossRef CAS PubMed
.
-
(a) A. J. Biloski and B. Ganem, Synthesis, 1983, 537 CrossRef CAS
;
(b) M. J. Milewska and A. Chimiak, Synthesis, 1990, 233 CrossRef CAS
;
(c) Q. X. Wang, J. King and O. Phanstiel IV, J. Org. Chem., 1997, 62, 8104 CrossRef CAS PubMed
;
(d) A. M. Berman and J. S. Johnson, J. Org. Chem., 2006, 71, 219 CrossRef CAS PubMed
.
-
(a) Z. Li and H. Yamamoto, Acc. Chem. Res., 2013, 46, 506 CrossRef CAS PubMed
;
(b) A. U. Barlan, W. Zhang and H. Yamamoto, Tetrahedron, 2007, 63, 6075 CrossRef CAS PubMed
.
-
(a) W. Zhang and H. Yamamoto, J. Am. Chem. Soc., 2007, 129, 286 CrossRef CAS PubMed
;
(b) Z. Li, W. Zhang and H. Yamamoto, Angew. Chem., Int. Ed., 2008, 47, 7520 CrossRef CAS PubMed
;
(c) Z. Li and H. Yamamoto, J. Am. Chem. Soc., 2010, 132, 7878 CrossRef CAS PubMed
;
(d) J. L. Olivares-Romero, Z. Li and H. Yamamoto, J. Am. Chem. Soc., 2013, 135, 3411 CrossRef CAS
;
(e) C. Wang and H. Yamamoto, J. Am. Chem. Soc., 2014, 136, 1222 CrossRef CAS PubMed
;
(f) A. Banerjee and H. Yamamoto, Org. Lett., 2017, 19, 4363 CrossRef CAS PubMed
;
(g) C. Wang and H. Yamamoto, J. Am. Chem. Soc., 2015, 137, 4308 CrossRef CAS PubMed
;
(h) C. Wang and H. Yamamoto, J. Am. Chem. Soc., 2014, 136, 6888 CrossRef CAS PubMed
.
- According to reviewer's suggestion we tested 2,2,6,6-tetramethylpiperidine under our reaction conditions and the results are summarized below.
.
Footnote |
† Electronic supplementary information (ESI) available. See DOI: 10.1039/c8sc04996c |
|
This journal is © The Royal Society of Chemistry 2019 |
Click here to see how this site uses Cookies. View our privacy policy here.