An examination of students' perceptions of the Kekulé resonance representation using a perceptual learning theory lens
Received
11th January 2019
, Accepted 18th March 2019
First published on 23rd March 2019
Abstract
Students in chemistry often demonstrate difficulty with the principle of resonance. Despite many attempts to mitigate this difficulty, there have been few attempts to examine the root cause of these issues. In this study, students were assessed for their perception of Kekulé structures based on perceptual learning theory, which is grounded in cognitive mechanisms of visual perception. The data from this assessment shows that students are perceiving inappropriate clues from this representation, which infers that the image itself might be an impediment to learning about resonance. Employment of a metarepresentational competence approach was used to address these misperceptions.
Introduction
The concept of resonance, which is commonly introduced to undergraduate students during general chemistry instruction, helps explain certain molecular arrangements when simple Lewis structure rules are insufficient to produce a singular correct structure. Resonance is a critical concept for general chemistry students to master as it helps them understand the electronic structure and reaction properties of molecules and ions (Richardson, 1986). Undergraduate students are then reintroduced to resonance during organic chemistry as a way to help explain additional properties of molecules and intermediates (Richardson, 1986), making resonance a fundamental concept in organic chemistry as well (Mullins, 2008). Despite repeated exposure and numerous attempts to clarify this concept for students (Delvigne, 1989; Abel and Hemmerlin, 1991; Starkey, 1995; Liu and Asato, 1997; Silverstein, 1999; Lin, 2007), the concept of resonance remains a difficult one for learners. Some of this difficulty appears to arise from students' interpretation of resonance to be a function of alternation rather than hybridization (Taber, 2001, 2002).
The nature of representations in chemistry may also contribute to students’ poor conceptual understanding of resonance. Like many canonical representations in chemistry, the Kekulé representation of resonance was derived from the communication of ideas and phenomena among experts, not developed intentionally as instructional materials for novice learners (Kozma et al., 2000). While experts can easily translate between representations at the various levels of Johnstone's triangle (macroscopic, sub-microscopic, and symbolic), students cannot and must develop the ability to operate along the edges of this triangle (Johnstone, 2010). This ability to translate and navigate between different representations is termed representational competence (Kozma and Russell, 2005), a skill that is underdeveloped in many undergraduate students. Poor representational competence corresponds with poor prior knowledge (Hilton and Nichols, 2011), and students with poor representational competence tend to focus on superficial features of representations and to view the representations as being exclusively static (Luxford and Bretz, 2014; Olimpo et al., 2015). These students may be unaware of the requirement to move back and forth between representations, and thus, are unable to judge the representation's affordances and limitations (Coppola et al., 1997).
Meaningful learning requires that students intentionally connect new information to prior knowledge and/or experiences in a productive way (Ausubel et al., 1978; Taber, 2001). Meaningful learning in chemistry also relies on visualization and interpretation of representations (Gilbert, 2005), but students cannot correctly interpret representations if their prior knowledge is deficient in the corresponding subject matter (Harle and Towns, 2012). This lack of representational reasoning is compounded by instructional practices that focus on fixed or static figures rather than actively translating between representations (Olimpo et al., 2015). Misconceptions held by chemistry students can be attributed, in part, to prior instruction where symbolic representations are used without explicit connections to their underlying contexts (Hilton and Nichols, 2011).
This study aims to understand how students use a canonical resonance representation to generate mental models and to determine if that representation is sufficient for students to develop a meaningful understanding of resonance. By analyzing the perceptual learning mechanisms that students employ, we aim to identify affordances and pitfalls associated with the representation and to suggest an alternate approach to developing representational competence and conceptual understanding of resonance.
Theoretical framework
This study was informed by the application of perceptual learning theory to frame students' use of a canonical resonance representation (Goldstone, 1998). Unlike tasks that require translation between multiple representations of an image, e.g., symbolic to macroscopic (Johnstone, 1993), the Kekulé representation provides only a single perspective to the student. The singular way in which the resonance image is presented requires that the viewer discriminate features within only that symbolic image to construct a mental model of the resonance phenomenon. Thus, it becomes necessary to examine a different framework for the sense-making mechanisms that a viewer uses to glean direct meaning from the resonance image (Keehner et al., 2008).
Perceptual learning theory focuses on the sensory stimulus that an individual perceives when interacting with the natural world. In terms of visual perception, a visual landscape creates a field of view that is sensed or perceived through neurological stimulus and is differentiated as a function of an individual's interaction with that stimulus (Gibson, 2000). Current understanding of perceptual learning further examines how learning can influence perception, i.e., the interaction between learned experience and perception (Goldstone et al., 2010; Kellman and Massey, 2013; Schwartz and Goldstone, 2015). In particular, these current interpretations of perceptual learning theory address issues pertaining to perceptions tied to abstract or symbolic concepts in the context of high-level cognitive domains, such as mathematics and science (Goldstone et al., 2010). Based on Goldstone's model of perceptual learning theory (Goldstone, 1998), perceptual learning can be analyzed through the lens of a discrete set of mechanisms, including differentiation and unitization. Differentiation corresponds to the perception of distinguishing or discriminating characteristics within an area of interest. Unitization, by contrast, corresponds to the construction of integrated units from disparate features within an area of interest. The concept of unitization bears similarity to the concept of “chunking” within the context of cognitive load theory (Goldstone, 2000). These perceptual mechanisms provide a direct means for examining student interpretations of a single representation, which lacks the translation tasks that are commensurate with the use of multiple representations. Furthermore, the alignment of measures to perceptual learning theory makes it possible to measure changes in students' perceptions as a function of learning interventions employed in this study.
Research question
What features/affordances do student perceive when viewing a canonical representation of resonance and how does this perception affect their conceptualization of resonance?
Methods
Study 1: preliminary assessment of students' perceptions of resonance
This study and its resulting data were reviewed and approved by the Institutional Review Board as an exempted study. In Study 1, an initial cohort of students (Cohort A, N = 33) was asked to respond the open response item shown in Fig. 1. These students were enrolled in a one-semester, general chemistry course targeted towards Biochemistry and Chemistry majors at a large, private northeastern university. The general chemistry course was conducted using Process Oriented Guided Inquiry Learning (POGIL), a widely disseminated active-learning pedagogy built on constructivist principles (Moog and Spencer, 2008). POGIL was originally developed as an inquiry-based approach to instruction in Chemistry, but has expanded to numerous other fields, including mathematics, engineering, and secondary-level science (Eberlein et al., 2008). The students in Study 1 were instructed using General Chemistry POGIL instructional materials directly as published (Moog and Farrell, 2011). Following their engagement with the resonance activity within the published POGIL material, the students in Study 1 were presented with a Reflection on Learning question (Fig. 1), a formative assessment that is a standard practice within the POGIL framework. In this particular assessment, the students were presented with the resonance structures of benzene, and they were asked to explain what the image means to them in an open response question format. The student responses were transcribed into Microsoft Excel and coded using an emergent coding scheme based on grounded theory (Strauss and Corbin, 1990). Transcribed student responses were delineated by assignment of random ID codes and were not identified further. Only data marked with these random codes were subjected to analysis throughout the rest of this study. Upon further clustering of response codes, the resulting categories strongly inferred differential perceptions of the representation, either commensurate or contrary to the concept of resonance.
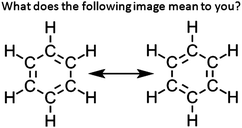 |
| Fig. 1 Open response inquiry item to assess students’ perceptions of a canonical resonance representation. | |
Study 2: targeted development of a perceptual learning theory-based coding scheme
To better resolve the possible perceptual issues in the student responses, a new coding framework based on perceptual learning theory was developed (Goldstone, 1998). After the initial data collection, two additional cohorts of students (Cohort B, N = 29; Cohort C, N = 38) were surveyed using the same assessment item. Collectively, these two cohorts constituted the population for Study 2. Within Study 2, the students provided responses prior to instruction on resonance using a modification of the POGIL activity presented in Study 1. Students provided responses to the same prompts after instruction. The responses were transcribed, as in Study 1, and analyzed to identify the potential perceptual learning methods employed by students. Perceptual learning theory was used to construct an axial coding scheme focused on elucidating the affordances that students draw from the resonance representation. The coding elements are outlined in Table 1.
Table 1 Perceptual learning theory coding scheme
Perceptual mechanism |
Description |
Example quote |
Dimensionalization |
Witnessing variation along a perceptual dimension, e.g., rotation, reflection |
“The same molecule just flipped. Two different ways of drawing the same molecule.” |
Segmentation |
Breaking objects into parts that are relevant or important, e.g., bonds, bond order, isomers |
“They are the same, the double bonds are just in different places – resonance structures” |
Unitization |
Creation of a single unit from multiple parts that occur together, e.g., averaging, mixing, multiple representations |
“These two molecules are resonance structures with the real structure being a blending of the two.” |
Idealization |
Simplification of objects to capture the basic essence of the underlying concept |
Re-drawing of figure as a hexagon with circle embedded inside |
Details regarding the perceptual learning codes are described below. When students wrote that the molecule was being rotated or viewed from a different perspective (e.g., mirror image), dimensionalization was coded. More generally, dimensionalization corresponds to perception of changes that apply to the structure as a whole, e.g., reflection. Unitization was coded when students discussed the true structure of benzene being an average of both represented structures or in both forms simultaneously (i.e. multiple representations of the same phenomenon). These responses align with the ideas of hybridization or delocalization. Segmentation was coded when students considered changes that occurred within specific parts and components of the benzene ring. Examples of segmentation are noted when students discussed the figure's bonds switching or changing bond orders. Idealization was coded when students simplified the figure and drew a hexagon with a circle inscribed as part of their responses.
To determine the inter-rater reliability of the perceptual learning coding scheme, two of the authors (LKW and TDK) coded data from Study 2 students (Cohorts B&C; N = 66). An inter-rater reliability analysis using the kappa statistic was performed to determine consistency among raters (Landis and Koch, 1977). This initial round of coding yielded 71.8% agreement with a Cohen's kappa of 0.30. The coding rubric was revisited by both coders and disagreements were discussed. After this reconsideration of the initial round of coding, the data was coded a second time yielding 90.6% agreement with a Cohen's kappa of 0.75, which is consistent with a substantial level of agreement (Landis and Koch, 1977). All remaining samples were then coded by a single author (TDK).
Study 2: instructional intervention and assessment
In an attempt to ameliorate students’ perceptions of the resonance figure in question, an instructional intervention was designed to enhance development of metarepresentational competence (diSessa, 2004) for Study 2 students (Cohorts B & C). Metarepresentational competence seeks to have students move beyond the competent usage of established representations to more fully understand the strengths and limitation of representational models. One way in which metarepresentational competence might be attained is by having students create or invent representations that move beyond “sanctioned” representations (diSessa, 2004). This approach is similar to “inventing with contrasting cases”, where students use data to create model frameworks prior to “telling” or more formalized instruction (Schwartz et al., 2011). In either case, students are exposed to canon only after they have had the opportunity to further develop their existing prior knowledge.
The same initial assessment (Fig. 1) was performed prior to instruction and the students engaged in the POGIL resonance activity, as described above. However, in this case, the activity was modified by the removal of the resonance figure from the activity in question (Fig. 2). In its place, students, working in groups of 4 as per standard POGIL practice, were tasked with developing a single (visual) representational model that captured the contradictions inherent in the information contained within the bonding data provided for the structure of benzene (Fig. 3). After these representations were created, all groups then presented their representations on whiteboards at the front of the classroom. As a collective, the class then considered each representation and discussed its relative strengths and limitations. After all representations had been discussed, the student groups continued with the POGIL activity without any further changes to the instructional materials. The effect of this instruction was measured by inclusion of the same open response item (Fig. 1) on a subsequent hour-long midterm examination. The responses from the exam item were coded according to the perceptual learning theory coding scheme, described above.
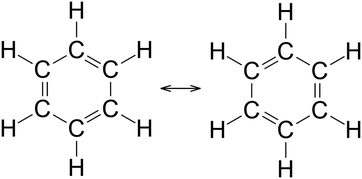 |
| Fig. 2 Resonance representation of benzene removed from student activity. | |
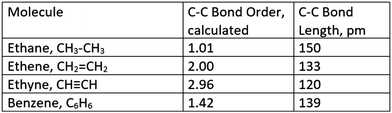 |
| Fig. 3 Table of resonance-related bond orders and bond lengths used to develop a single visual representation of benzene. | |
Results and discussion
Study 1: preliminary assessment of students' comprehension of resonance
The emergent coding from Study 1 students identified several common responses related to resonance descriptions (Table 2). There were 13 themes found in the responses describing students’ perceptions of the resonance representation:
Table 2 Emergent coding of Study 1 (Cohort A) responses
Student response/emergent code |
No. of occurrences (% frequency) |
Generalization |
Bonds “switch” positions |
9 (39%) |
“Two species” 18 students (78%) |
Rotation |
6 (26%) |
Changing bond orders |
3 (13%) |
Isomers |
2 (9%) |
Reflection |
2 (9%) |
Symmetry |
1 (4%) |
|
Multiple representations |
3 (13%) |
“One species” 5 students (22%) |
Mixing/averaging |
2 (9%) |
• rotation
• bonds switch positions
• different properties
• symmetry
• resonance
• different bond orders
• more than one way to draw structure
• both forms at the same time
• mixture or average of structures
• equivalent structures
• isomers/different structures
• reflection
• shared electron cloud or delocalized electrons.
These themes were further clustered by way of a phenomenographic lens (Orgill and Bodner, 2004) and collapsed into 8 codes (Table 2) which generally aggregated into two basic categories: (1) the existence of two discrete species, and (2) multiple representations of a single species. The response frequencies are summarized with their respective codes in Table 2. Some student responses contained statements that yielded multiple codes which explains why the number of occurrences exceeds the number of students in the sample. From the response frequencies, it becomes clear that an overwhelming majority of students (78%) perceive that each of the two hexagonal structures within the image is considered a distinct entity, rather than complementary representations of a single phenomenon. The observation of this misperception after instruction with an established active learning pedagogy (POGIL) provides evidence for the robust nature of this misperception. An important question arises as to why students persist in perceiving two discrete species in spite of focused instruction and focused deliberations on the nature of a single benzene species in the POGIL activity.
Retrospective analysis of Study 1 student responses, using the lens of perceptual learning theory demonstrates a strong tendency for student to employ mechanisms of Dimensionalization and Segmentation (Fig. 4). These mechanisms align with students’ tendencies to describe two discrete species within the resonance figure. Dimensionalization identifies variations along a particular dimension or orientation, e.g., mirror planes, rotational axes. Students who describe the differences between the structures in terms of wholesale changes, e.g., rotation of one structure to yield the other, are likely using dimensionalization to draw distinctions. Segmentation identifies component parts with a larger structure. Within the resonance image, those students who employ segmentation are identifying changes that occur within a subset of the greater image, e.g., bonds moving. Unitization is the perceptual mechanisms that aligns best with the overarching idea of resonance. It identifies ways in which distinct components can be coalesced or chunked into a single unit. The coding frequency of unitization within these responses is consistent with the emergent coding results in revealing that a majority of students do not perceive a single phenomenon, but rather perceive two distinct species based on visual perception.
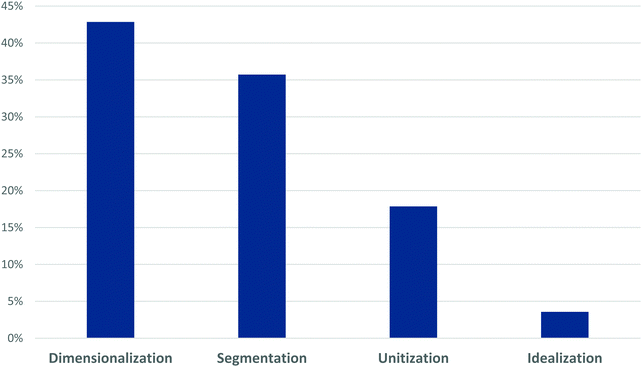 |
| Fig. 4 Perceptual Learning Theory code frequency for initial responses of students in Study 1 (Cohort A) after instruction using original POGIL activity (N = 33). | |
Study 2: instructional intervention outcomes and analysis
Based on the influence prior knowledge exerts in the development of representational competence (Cook, 2006), we investigated the impact of providing additional scaffolding prior to the introduction of the representation. Metarepresentational competence provides a generalizable approach for improving students' use of representations (diSessa, 2004). Metarepresentational competence demands that students look beyond sanctioned or canonical representations and focus on the underlying nature of representations by articulating their limitations and affordances and creating new representations. By using metarepresentational competence as an approach to augment students’ knowledge and comprehension of the resonance phenomenon, we postulated that the misperceptions surrounding the resonance representation might be reduced as students developed a fuller appreciation for what the representation is and is not capable of conveying. By modifying the POGIL resonance activity, as described in Methods, above, students developed self-generated representations that attempted to resolve the contradictory nature of the bonding data provided in the POGIL exercise (Fig. 3).
The most common representations produced by students during the metarepresentational exercise can be seen in Table 3. Each representation is accompanied its strengths and weaknesses, summarized from student discussions. Similar to contrasting cases (Schwartz et al., 2011), the presentation of all representations to the class along with a collective examination of strengths and weaknesses allows students to view each representation in relation to a broader landscape of representational forms. From the declared strengths and weaknesses, it becomes apparent that students are provided with an opportunity to appreciate the limitations that are inherent in any single representation. These outcomes align with previous observations that students' perceptual sense-making is an important element in the development of their representational competence (Rau, 2015).
Table 3 Prevalent student self-generated representations from Study 2 (Cohorts B&C)
Representation |
Strengths |
Weaknesses |
|
Conforms to octet rule |
Doesn’t resolve bond order data |
|
Demonstrates equivalence of bond order |
Doesn’t allow electron counting for octet rule |
|
Provides appropriate bond orders and electron counts |
Doesn’t conform to standard practice for Lewis structures |
To measure the effect of this metarepresentational exercise, the students were assessed with the same item (Fig. 1) prior to instruction and post-instruction (after a lag time of several days) on a mid-term exam as described in Methods. The responses to the open-response item were coded according to the perceptual coding rubric established within Methods, above. A comparison between pre- and post-intervention response code frequencies can be seen in Fig. 5. From the changes observed between pre- and post-intervention measurements, there is an observable change in the frequency for all of the perceptual mechanisms coded. The use of unitization and idealization both increase while dimensionalization and segmentation both decrease. Since a pre-/post-measurement relies on measures of the same student, the responses cannot be considered independent, thus a chi square test of significance cannot be employed here. More appropriately, McNemar's test of independence was used to test the significance of the changes observed in perceptual mechanism use. Using a probability (alpha) of 0.05, the changes between pre- and post-observations for dimensionalization (p = 0.00596) and unitization (p = 2.31 × 10−6) were both found to be statistically significant. In contrast, the changes observed for segmentation (p = 0.248) and elaboration (p = 0.239) are not statistically significant.
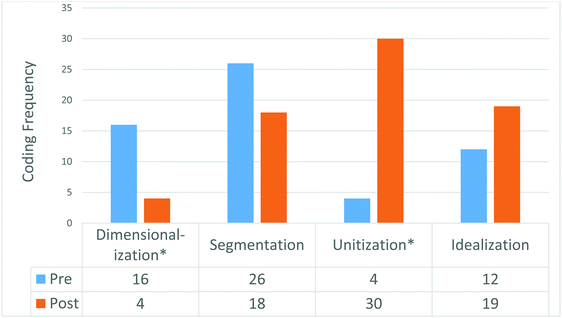 |
| Fig. 5 Pre-/post-comparison of perceptual learning coding responses for Study 2 (N = 66, *statistically significant at p < 0.01). | |
Based on the measured reduction in dimensionalization use and the commensurate increase in unitization, the introduction of metarepresentation into this learning opportunity corresponds to a substantial realignment of how students perceive the figure in question. The lack of a significant reduction in segmentation would appear to run counter to the development of a more authentic mental model. However, it is worth noting that segmentation requires that a student focus within a particular structure (e.g., the double bonds within a benzene representation) whereas dimensionalization requires that a student interpret each hexagonal ring as an independent entity (e.g., one benzene ring is transformed to become an independently distinct benzene ring). By virtue of these distinctions, it should not be surprising that the dimensionalization mechanism is more likely to oppose students' use of a unitization lens when engaging the resonance representation.
Conclusions
This study examined students' usage of a canonical representation of chemical resonance. As has been identified in previous studies, students armed with insufficient prior knowledge are prone to face difficulties when confronting external representations (Cook, 2006; Chittleborough and Treagust, 2008; Corradi et al., 2014; Olimpo et al., 2015). The evidence collected in the course of this study demonstrates that students' usage of this representation can cause them to anchor onto inappropriate features of the resonance representation in the formation of mental models. Specifically, the students in both studies drew upon their existing prior knowledge to interpret the dualistic nature of the Kekulé resonance representation as two distinct species rather than complementary forms of a single species. This initial misperception of the representation can then influence the students' development of a mental model for resonance. Once formed, these inappropriate mental models are robust and somewhat impervious to instructional interventions.
To unpack the process by which students perceive the figure in question, perceptual learning theory provides a useful lens for discriminating how students interpret different visual features of the representation (Goldstone, 1998). Many of the mechanisms articulated within perceptual learning theory appear to align with responses conveyed by students in their examination of the resonance figure. In particular, the mechanisms of segmentation and dimensionalization line up with the observation that students are incorrectly assigning distinctness to visual features (structures) that are meant to be considered in concert, whereas, the mechanism of unitization aligns with the accepted interpretation that the two structures are multiple representations of a single phenomenon. This observed alignment of perceptual mechanisms with ways of interpreting the resonance representation provide a means for examining potential changes in student perceptions of that representation. This apparent alignment between perceptual learning theory and potential (mis)interpretations of the resonance representation can be further exploited to evaluate the efficacy of treatments intended to ameliorate students' misperceptions.
For the purpose of this study, a metarepresentational approach was employed to mitigate students' misperceptions regarding the resonance representation (diSessa, 2004). This approach also provided a testbed to examine the analytical power of perceptual learning theory to measure changes resulting from the instructional approach. The use of a metarepresentational framework prior to the introduction of the resonance figure allows students to view the figure through a more critical lens and avoid the instruction-resistant mental model that emerged in students that were not exposed to the metarepresentational framework. Measurement of this perceptual shift via the perceptual learning theory lens yielded a statistically significant result that demonstrates a clear change in how students viewed and interpreted the representation.
Limitations
Due to the peculiarities of the resonance representation, the scope of this study is limited. Unlike translation of representations across different dimensions, e.g., symbolic to particulate, the resonance representation requires the amalgamation of representations within a single dimension for the development of an appropriate mental model and these mental models must be inferred rather than measured directly. While perceptual learning theory aligns well with the affordances within this particular representation, the utilization of the perceptual learning theory framework does not necessarily translate to other issues related to representational competence. The study population is also limited in scope and scale. Correspondingly, these studies lack the statistical power to be broadly generalizable.
Implications for teaching
The results presented in this study reinforce the claim that students must leverage a certain level of prior knowledge in order to develop appropriate representational competence. Furthermore, the results of this study infer that a lack of prior knowledge can cause students to develop robust but misleading mental models when working with canonical representations (Hilton and Nichols, 2011). To develop more appropriate mental models, great care and consideration should be taken before presenting students with representations. One approach is for students to engage directly in the self-development of representational models prior to being presented with canonical representations. By providing students with the ability to distinguish both the affordances and limitations of representations as they relate to the respective referent, their ability to discriminate those same affordances and limitations within the canonical representation are enhanced. This metarepresentational approach allows for the healthy development of perceptual sense-making regarding these images and circumvents the likelihood that students consider only the superficial features of the representation (Chi et al., 1981; Rau, 2015). Although the specifics of the resonance representation limit the scalability of this study, the development of metarepresentational competence for students engaged in the generation of mental models from external representations is a practice with numerous opportunities for implementation outside the confines of this study.
Implications for research
The results of this study demonstrate that students are likely to make inappropriate use of external representations in the construction of mental models. The alignment of these results with the mechanisms of perceptual learning theory demonstrates that visual perception and visual affordances can play a significant role in how students might use and misuse visual representations. While resonance is somewhat unique in its representational affordances, the fact that students misconstrue its meaning from initial exposure to an external representation infers that an examination of a representation's affordances can shed light on how robust misconceptions can arise from inappropriate interpretation of those external representations. The changes that were observed when metarepresentational competence was used to mitigate the construction of incorrect mental models show that student perception of this representation can be malleable, as long as students are trained to turn a critical eye towards this representation. Perceptual learning theory may or may not provide an appropriate lens for examining other representations. However, the development of metarepresentational competence provides a generalizable approach for improving students' ability to unpack a broad array of representations.
Conflicts of interest
There are no conflicts to declare.
Acknowledgements
This research is based upon work supported by the National Science Foundation under Grant No. DUE 1359262. Additionally, this material is partially based on work while Thomas Kim was serving at the National Science Foundation. Any opinion, findings, and conclusions or recommendations expressed in this material are those of the authors and do not necessarily reflect the views of the National Science Foundation.
References
- Abel K. B. and Hemmerlin W. M., (1991), Explaining resonance – a colorful approach, J. Chem. Educ., 68(10), 834, DOI:10.1021/ed068p834.
- Ausubel D. P., Novak J. D. and Hanesian H., (1978), Educational psychology: a cognitive view, New York: Holt, Rinehart and Winston.
- Chi M. T. H., Feltovich P. J. and Glaser R., (1981), Categorization and Representation of Physics Problems by Experts and Novices, Cognit. Sci., 5(2), 121–152, DOI:10.1207/s15516709cog0502_2.
- Chittleborough G. and Treagust D., (2008), Correct Interpretation of Chemical Diagrams Requires Transforming from One Level of Representation to Another, Res. Sci. Educ., 38(4), 463–482, DOI:10.1007/s11165-007-9059-4.
- Cook M. P., (2006), Visual representations in science education: the influence of prior knowledge and cognitive load theory on instructional design principles, Sci. Educ., 90(6), 1073–1091, DOI:10.1002/sce.20164.
- Coppola B. P., Ege S. N. and Lawton R. G., (1997), The University of Michigan Undergraduate Chemistry Curriculum 2. Instructional Strategies and Assessment. J. Chem. Educ., 74(1), 84, DOI:10.1021/ed074p84.
- Corradi D. M. J., Elen J., Schraepen B. and Clarebout G., (2014), Understanding Possibilities and Limitations of Abstract Chemical Representations for Achieving Conceptual Understanding, Int. J. Sci. Educ., 36(5), 715–734, DOI:10.1080/09500693.2013.824630.
- Delvigne F., (1989), A visual aid for teaching the resonance concept, J. Chem. Educ., 66(6), 461, DOI:10.1021/ed066p461.
- diSessa A. A., (2004), Metarepresentation: Native Competence and Targets for Instruction, Cognit. Instruct., 22(3), 293–331.
- Eberlein T., Kampmeier J., Minderhout V., Moog R. S., Platt T., Varma-Nelson P. and White H. B., (2008), Pedagogies of engagement in science, Biochem. Mol. Biol. Educ., 36(4), 262–273, DOI:10.1002/bmb.20204.
- Gibson E. J., (2000), Perceptual Learning in Development: Some Basic Concepts, Ecol. Psychol., 12(4), 295–302, DOI:10.1207/S15326969ECO1204_04.
- Gilbert J., (2005), Visualization in science education, Dordrecht: Springer, retrieved from http://public.eblib.com/choice/publicfullrecord.aspx?p=303335.
- Goldstone R. L., (1998), Perceptual Learning, Annu. Rev. Psychol., 49(1), 585–612, DOI:10.1146/annurev.psych.49.1.585.
- Goldstone R. L., (2000), Unitization during category learning, J. Exp. Psychol.: Hum. Percept. Perform., 26(1), 86–112, DOI:10.1037//0096-1523.26.1.86.
- Goldstone R. L., Landy D. H. and Son J. Y., (2010), The Education of Perception, Top. Cognit. Sci., 2(2), 265–284, DOI:10.1111/j.1756-8765.2009.01055.x.
- Harle M. and Towns M. H., (2012), Students’ Understanding of External Representations of the Potassium Ion Channel Protein, Part I: Affordances and Limitations of Ribbon Diagrams, Vines, and Hydrophobic/Polar Representations, Biochem. Mol. Biol. Educ., 40(6), 349–356, DOI:10.1002/bmb.20641.
- Hilton A. and Nichols K., (2011), Representational Classroom Practices that Contribute to Students’ Conceptual and Representational Understanding of Chemical Bonding, Int. J. Sci. Educ., 33(16), 2215–2246.
- Johnstone A. H., (1993), The development of chemistry teaching: a changing response to changing demand, J. Chem. Educ., 70(9), 701, DOI:10.1021/ed070p701.
- Johnstone A. H., (2010), You Can’t Get There from Here, J. Chem. Educ., 87(1), 22–29, DOI:10.1021/ed800026d.
- Keehner M., Hegarty M., Cohen C., Khooshabeh P. and Montello D. R., (2008), Spatial Reasoning With External Visualizations: What Matters Is What You See, Not Whether You Interact, Cognit. Sci., 32(7), 1099–1132, DOI:10.1080/03640210801898177.
- Kellman P. J. and Massey C. M., (2013), Chapter Four – Perceptual Learning, Cognition, and Expertisee, in Ross B. H. (ed.), Psychology of Learning and Motivation, Academic Press, vol. 58, pp. 117–165, DOI:10.1016/B978-0-12-407237-4.00004-9.
- Kozma R. and Russell J., (2005), Students Becoming Chemists: Developing Representationl Competence, in Gilbert J. K. (ed.), Visualization in Science Education, Dordrecht: Springer Netherlands, pp. 121–145, retrieved from DOI:10.1007/1-4020-3613-2_8.
- Kozma R., Chin E., Russell J. and Marx N., (2000), The Roles of Representations and Tools in the Chemistry Laboratory and Their Implications for Chemistry Learning. J. Learn. Sci., 9(2), 105.
- Landis J. R. and Koch G. G., (1977), The Measurement of Observer Agreement for Categorical Data, Biometrics, 33(1), 159, DOI:10.2307/2529310.
- Lin S., (2007), Aromatic Bagels: An Edible Resonance Analogy, J. Chem. Educ., 84(5), 779, DOI:10.1021/ed084p779.
- Liu R. S. H. and Asato A. E., (1997), Making Organic Concepts Visible, J. Chem. Educ., 74(7), 783, DOI:10.1021/ed074p783.
- Luxford C. J. and Bretz S. L., (2014), Development of the Bonding Representations Inventory To Identify Student Misconceptions about Covalent and Ionic Bonding Representations, J. Chem. Educ., 91(3), 312–320, DOI:10.1021/ed400700q.
- Moog R. S. and Farrell J. J., (2011), Chemistry: a guided inquiry, Hoboken, NJ: John Wiley & Sons.
- Moog R. S. and Spencer J. N., (2008), POGIL: An Overview, In Process Oriented Guided Inquiry Learning (POGIL), American Chemical Society, vol. 994, pp. 1–13, DOI:10.1021/bk-2008-0994.ch001.
- Mullins J. J., (2008), Six Pillars of Organic Chemistry, J. Chem. Educ., 85(1), 83, DOI:10.1021/ed085p83.
- Olimpo J. T., Kumi B. C., Wroblewski R. and Dixon B. L., (2015), Examining the relationship between 2D diagrammatic conventions and students’ success on representational translation tasks in organic chemistry, Chem. Educ. Res. Pract., 16(1), 143–153, 10.1039/C4RP00169A.
- Orgill M. and Bodner G., (2004), What research tells us about using analogies to teach chemistry, Chem. Educ. Res. Pract., 5(1), 15–32, 10.1039/B3RP90028B.
- Rau M. A., (2015), Enhancing undergraduate chemistry learning by helping students make connections among multiple graphical representations, Chem. Educ. Res. Pract., 16(3), 654–669, 10.1039/C5RP00065C.
- Richardson W. S., (1986), Teaching the concept of resonance with transparent overlays, J. Chem. Educ., 63(6), 518, DOI:10.1021/ed063p518.
- Schwartz D. L. and Goldstone R., (2015), Learning as coordination: cognitive psychology and education, in Corno L. and Anderman E. M. (ed.), Handbook of educational psychology, New York; London: Routledge is an imprint of the Taylor & Francis Group, an Informa business, 3rd edn, pp. 61–75.
- Schwartz
D. L., Chase C. C., Oppezzo M. A. and Chin D. B., (2011), Practicing versus inventing with contrasting cases: the effects of telling first on learning and transfer, J. Educ. Psychol., 103(4), 759.
- Silverstein T. P., (1999), The “Big Dog-Puppy Dog” Analogy for Resonance, J. Chem. Educ., 76(2), 206, DOI:10.1021/ed076p206.
- Starkey R., (1995), Resonance Analogy Using Cartoon Characters, J. Chem. Educ., 72(6), 542, DOI:10.1021/ed072p542.
- Strauss A. L. and Corbin J. M., (1990), Basics of qualitative research: grounded theory procedures and techniques, Newbury Park, Calif.: Sage Publications.
- Taber K. S., (2001), Building the structural concepts of chemistry: some considerations from educational research, 2(2), 123–158, 10.1039/B1RP90014E.
- Taber K. S., (2002), Compounding quanta: probing the frontiers of student understanding of molecular orbitals, Chem. Educ. Res. Pract., 3(2), 159–173, 10.1039/B2RP90013K.
|
This journal is © The Royal Society of Chemistry 2019 |
Click here to see how this site uses Cookies. View our privacy policy here.