Attraction vs. repulsion – learning about forces and energy in chemical bonding with the ELI-Chem simulation
Received
10th January 2019
, Accepted 28th March 2019
First published on 17th April 2019
Abstract
This work seeks to solve one of the basic problems in chemistry learning: understanding the chemical bond as a dynamic equilibrium between attractive and repulsive forces. This force-based model is difficult to grasp, as there are no analogues from everyday life for both attractions and repulsions happening simultaneously. In addition, current teaching approaches often mislead by using mainly the ‘octet rule’ heuristic. As a result, students construct naïve models of the chemical bond, usually viewing atoms as solid balls that are attached to each other in order to “achieve an octet.” To represent the force-based dynamics of the bond, we designed the ELI-Chem learning environment. This environment enables interaction as an atom with another atom while observing the underlying forces and the potential energy curve. Our theoretical framework is based on Embodied Learning theory by relating conceptual learning to bodily experiences. The study uses qualitative and quantitative methods with 21 high school chemistry students in a pretest–intervention–posttest design. During a 40 minute activity with the ELI-Chem simulation, students were prompted to discover the underlying forces of bonding and relate them to energy changes. Findings show that learning with the ELI-Chem simulation supports students in gaining the knowledge elements that are required to build the dynamic force-based mental model of chemical bonding, and to conceptualize chemical energy as due to forces. Finally, the design principles of the ELI-Chem environment are discussed. Aligned with science standards, attending to students’ difficulties, and using the advantages of a computer simulation, the ELI-Chem environment provides an appropriate representation of chemical bonding, which is more valid scientifically yet makes the abstract concept accessible.
Introduction
Teaching and learning the topic of chemical bonding is most challenging. The chemical bond presents an unusual scheme of attractive and repulsive forces acting simultaneously between atoms. These forces cause the atoms to come closer and farther apart continuously, setting the atoms in motion around the point of equilibrium. Such a scheme is not intuitive; in fact, it is outside of the range of physical sensations experienced in the world. There is no familiar physical system in our world where opposing forces act simultaneously. Moreover, the most stable state (i.e., minimum energy) of chemical bonding is a dynamic state. It is a dynamic equilibrium between the forces, meaning that the magnitudes of the attractive and repulsive forces are equal and the net force is zero. Yet, the forces act, they do not cancel each other out. Grasping this conflict, in which a stable state is dynamic, is even more paradoxical and counter-intuitive.
Understanding this invisible, non-intuitive and dynamic phenomenon of chemical bonding requires an appropriate representation. With computer simulations it is possible to create dynamic metaphors, which might constitute a more suitable representation. According to Embodied Cognition theories, learners rely on representations, which are based on abstraction from direct experience of the world (Lakoff and Johnson, 1980; Barsalou, 1999). Without such experiences, students resort to imagining a chain of events set in an unfamiliar and non-intuitive world.
The question is, which representation is most appropriate for high school chemistry? On the one hand, the scientific model of the chemical bond is based on quantum mechanical theories which are beyond high school students’ understanding. On the other hand, the oversimplified ‘octet rule’ approach (i.e. atoms form bonds “in order to complete an octet, as this is their most stable state”) leads to learning impediments and misunderstanding by many students (Taber and Coll, 2002; de Jong and Taber, 2014; Tsaparlis et al., 2018). It would seem that a more appropriate simplification for high school students is a force-based representation of the chemical bond (Taber and Coll, 2002; Levy-Nahum et al., 2007; Stevens et al., 2010). Moreover, the force-based approach has recently been introduced into the NGSS standards for science education (NGSS, 2013). According to this approach, the attractive and repulsive forces between atoms arise from their subatomic structure and govern the behaviour of atoms and molecules. Thus, a bond is formed due to electrical forces; it is most stable when the attractive forces balance the repulsive forces.
To represent the force-based explanation, we developed the ELI-Chem (Embodied Learning Interactive Chemistry; Zohar and Levy, 2015) learning environment which includes a computer simulation and an activity guide. Based on the Lenard-Jones mathematical model of chemical bonding (Jones, 1924), the simulation enables an active manipulation of atoms while observing the resultant forces and the potential energy curve. The theoretical framework of the ELI-Chem learning environment is based on embodied learning theories that view bodily experiences as central to foster understanding (Abrahamson and Lindgren, 2014).
Our main goals were to reveal students’ conceptions of chemical bonding and to study the effect of the bodily experience on their understanding of the force-based explanation. However, already in the pretest interview, we noticed an important finding which we have named the lacuna of repulsion: most students did not acknowledge the repulsive forces between the atoms, perceiving the chemical bond as two attached atoms standing still. We then studied the effect of this lacuna on students’ mental models (internal representation that captures people's understanding of phenomena in a single framework; Norman, 1983) and described them in our previous paper (Zohar and Levy, 2019). Based on this previous research, we designed the activity guide and adapted the chemical bonding simulation. For example, we had found that the bottle-neck for understanding bonding was perceiving the repulsion force between atoms. Therefore, in the design of the activity, perceiving repulsion was the first challenge, from which other learning could proceed. In addition, we included in the simulation an option to run the process of bonding without repulsion so that students could create and test “what if” questions.
In the present paper, we demonstrate and discuss the changes in students’ mental models after working with the ELI-Chem learning environment, compared with the pretest mental models described previously. We then discuss the design principles of the ELI-Chem learning environment – simulation and activity guide – and wrap up with implications for teaching.
Literature review
In the following, we first describe several representations of chemical bonding while considering their appropriateness to high school chemistry; among them, we choose to base our design on the force-based representation. Next, we describe the design principles of the learning environment, based upon the learning goals and difficulties in understanding.
Representations of chemical bonding: quantum, forces or octet?
Chemical bonding is a key and basic concept in high school chemistry, yet it is difficult both to understand and to demonstrate. It is an abstract, non-intuitive phenomenon which has no analogues in our everyday life. According to some chemists, it cannot be clearly defined, because of its intangible nature (Croft and de Berg, 2014). In order to define a chemical bond, several conceptual models can be used, each emphasizing a different aspect of the bond and explaining different chemical phenomena. These conceptual models span the range from being too advanced for high-school chemistry students to over-simplifications that might impede learning. On the one hand, scientists describe chemical bonding by using quantum-mechanical theories, which are beyond high school chemistry. Educational research argues that several aspects of the quantum mechanical model are too difficult for most beginning students to grasp, as they are purely mathematical constructs that cannot be determined by experiments (Gillespie, 1997; Shusterman and Shusterman, 1997; Taber and Coll, 2002; Kozma and Russell, 2005; Stevens, Delgado and Krajcik, 2010). Furthermore, the quantum theory gives students the incorrect impression that chemistry is a difficult, abstract, mathematical subject that is not and cannot be satisfactorily explained at the introductory level (Gillespie, 1997). On the other hand, chemistry textbooks and teachers oversimplify the topic of chemical bonding by presenting it as based on the octet rule (Taber and Coll, 2002; Pabuçcu and Geban, 2006; Levy-Nahum et al., 2007; Talanquer, 2007; Bergqvist et al., 2013). These oversimplified representations are easy to demonstrate and easy to understand; however, they do not provide students with enough scientific tools that may promote their causal understanding. Studies show that many students do not appreciate the electrical interactions that drive chemical bonding; instead, they rely on rote learning and heuristics (Taber and Coll, 2002; Levy-Nahum et al., 2007; Venkataraman, 2017; Joki and Aksela, 2018). Thus, students believe that atoms form bonds “in order to complete an octet,” that stable species have octets whereas species without octets are unstable, or that atoms will spontaneously lose electrons in order to obtain octets (de Jong and Taber, 2014). Consequently, they do not comprehend the energetics associated with chemical bonding. Rather than using forces to explain the energy changes, they base their explanations on intuitive interpretations. Some students think of atoms as physical entities that require energy to bring them together (Boo, 1998), or as coiled springs that release energy when relaxed (Hapkiewicz, 1991). Others think that potential energy represents an ability to form a bond, to interact, or to move (Becker and Cooper, 2014; Lindsey, 2014). These naïve ideas are carried over to related concepts such as polarity, ionization energy and the molecular-level interactions that govern phase transitions (Teichert and Stacy, 2002; Taber, 2003).
Aware of the difficulties in both representing chemical bonding and understanding this topic, different approaches have been presented.
Teaching sequence
Dhindsa and Treagust (2014) proposed a new sequence for teaching chemical bonding: covalent, polar covalent and ionic bonding. They argue that in this sequence, the concepts are developed with a minimum reorganization of previously learned information, leading to more effective and sustainable learning. By contrast, Bergqvist et al. (2013) suggest the opposite sequence of metallic, ionic, and covalent bonding, to avoid students applying the ‘molecule presence’ in all structures. As far as we know, these teaching sequences were not researched with students.
Force-based approach
Based on science education documents (NGSS, 2013), bonding is a result of forces, proximity, and energy. Aligned with this description, educational research proposed representing the topic of chemical bonding as due to electrical forces (Taber and Coll, 2002; Levy-Nahum et al., 2007; Stevens et al., 2010).
According to the force-based approach, the attractive and repulsive forces between atoms arise from their subatomic structure and govern the behaviour of atoms and molecules. Therefore, a bond is formed due to electrical forces. The attractive and repulsive forces act simultaneously so that the atoms move closer and farther apart continuously. The bond is most stable when the attractive forces balance the repulsive forces. At this equilibrium point, the net force is zero; hence, the potential energy of the system is minimal. Thus, bond formation happens spontaneously – the energy decreases and is released; whereas, breaking a bond requires energy to overcome the balance between the forces.
To our knowledge, only two studies investigated the force-based explanation of chemical bonding with students; both reported a successful shift from the octet-based reasoning to an appreciation of electrical forces (Joki et al., 2015; Venkataraman, 2017). Regarding energy and forces that are involved in chemical bonding, Dreyfus et al. (2014) and Nagel and Lindsey (2015) showed how appropriate scaffolding supported students in relating bond energy to forces. Learning technologies that support students in perceiving the chemical bond as forces include interactive simulations such as Molecular Workbench (Xie and Pallant, 2011) and PhET (Wieman et al., 2008). Despite these research's recommendations and findings, updated chemical education literature shows that most instructional materials (e.g., teachers and textbooks) did not shift to the force-based approach (Bergqvist and Chang Rundgren, 2017; Erman, 2017; Joki and Aksela, 2018; Tsaparlis et al., 2018).
We choose to represent the force-based approach because we consider this approach to be the “sweet spot” between difficulty and over-simplification. It is an appropriate simplification of the quantum-mechanical theory, as it accounts for and discusses the stability of molecules in terms of the classical concept of a balance between the electrostatic forces of attraction and repulsion (Bader, n.d.). In addition, it can be represented with visible and familiar objects – balls and arrows – rather than quantum-mechanical equations (restructuration; Wilensky and Papert, 2010). Yet, the force-based approach is not an oversimplification, as it is based on scientific principles. Although the electrical forces do not explain all aspects of chemical bonding (e.g., the influence of the ‘spin’ of the electron), they do provide a proper scientific interpretation (Taber and Coll, 2002).
Learning-goals-driven design of the force-based approach
Having decided to implement the force-based approach, we designed a learning environment aligned with the learning-goals-driven design model (Krajcik et al., 2008). The design integrates two aspects: (1) the content is aligned with chemistry education research and with science standards, and (2) the learning process is based on discovery models. In specifying the learning goals, we identified two levels of difficulties: general in learning chemistry and specific to chemical bonding.
Learning chemistry
The science of chemistry describes an abstract dynamic molecular world in which atoms and sub-atomic particles interact in a non-intuitive way. Students cannot access this world or experience analogues of the forces underlying the particles’ behaviour. They are required to imagine phenomena at an atomic-scale level and explain them by applying scientific explanations. Moreover, the representations used in textbooks or in classrooms – both on the board or the ball-and-stick models – are static. Students are expected to understand that the static representation is just one specific frame of the dynamic molecular world and to visualize the endless motion using their own imagination (Gilbert et al., 2006). Thus, our first goals are to make the molecular world accessible and dynamic.
Chemical bonding
The NGSS (2013) standards in science education state that students should understand that “Bonding is a result of forces, proximity, and energy. This provides a basis for understanding all types of bonding, including ionic, covalent, metallic, and hydrogen bonding”. As such, the main learning goals are to describe the chemical bonding process and its energetics through the relationships between attractive and repulsive forces (Taber and Coll, 2002; Levy-Nahum et al., 2007; Stevens et al., 2010). In particular, students should be aware of the existence of both attractive and repulsive forces, to recognize that a chemical bond is a dynamic equilibrium between opposing forces, and to relate this to the involved potential energy. Students should base their explanations on the idea that chemical bonds are formed because atoms are more stable when bonded together, that energy is released during bond formation as the atoms become more stable, and that energy is required in order to break a bond, to overcome the balance between the forces.
Related learning goals are understanding the scientific meanings of the concepts of ‘force’ and ‘equilibrium,’ as the force-based approach is based on these concepts.
Forces
The NGSS (2013) standards state that students should have an understanding that a force may be exerted on an object without touching it through fields. Novice students do not conceive of force as a process of interaction between two material objects. Rather, the most commonly reported naïve conception is that force is an internal property of a single object or an intentional interference of an external agent (McCloskey, 1983; Driver, 1994; Reiner et al., 2000). Applying this naïve idea to chemical bonding, it is possible that students would see bonding as due to a single atom property, such as “every atom wants to complete the octet.” Based on this naïve understanding, two atoms will bond if each of them gains an octet, rather than the more scientific approach of electrical interaction between them.
Equilibrium
The NGSS (2013) standards in science education state that students should understand that an equilibrium is a dynamic state in which forces of the same magnitude occur in opposite directions. However, they typically think of equilibrium as a static situation and interpret a lack of change in the system to indicate that nothing is happening (Driver, 1994). The same static view of equilibrium states was found in the chemical education research: most chemistry students think of equilibrium as a static state in which no reactions occur (for a review, see Özmen, 2008; Bain and Towns, 2016). Zohar and Levy (2019) reported that students perceive bonded atoms as static and attached to each other. They showed that a dynamic equilibrium between atoms is understood only when students refer to both attractive and repulsive forces that act in opposing directions.
Nevertheless, the concepts of force and equilibrium are not being taught in the context of chemical bonding. Forces are included in Physical Science under the core idea of “Forces and Interactions,” and equilibrium in Crosscutting Concepts under “Stability and Change” (NGSS, 2013). Indeed, Taber (1998) has shown that many chemistry students are not familiar with basic physics, although it is implicitly assumed that they are.
To sum it up, the design of learning materials, which is based on a force-based explanation of chemical bonding, should address the following learning goals: chemical bonding as the combined attractive and repulsive forces; relating the energetics of chemical bonding to forces; and a dynamic rather than static view of the molecule.
Research question
The overarching aim of this study is to explore how force-based representations in chemistry could support students’ understanding of chemical bonding. The ELI-Chem learning environment was designed to enable students’ interactions with a force-based simulation of chemical bonding. The study assesses the effectiveness of this design by comparing students’ mental models before and after working with the learning environment.
This paper addresses the following research question: What characterizes the shift in students’ mental models following their learning experiences with the force-based ELI-Chem learning environment?
Learning environment
In designing the ELI-Chem environment, we considered both the conceptual structure of the force-based approach and the learning process. To address these goals, ELI-Chem environment is composed of a computer simulation and an online activity guide.
ELI-Chem simulation
The ELI-Chem simulation was created with NetLogo (Wilensky, 1999), a platform for creating simulations. The mathematical model of the simulation is based on the Lennard-Jones (L-J) potential for two neutral atoms (Jones, 1924) approximating the electrical forces between them (see Appendix 1). This model consists of two opposing components: a steep repulsive term at short ranges and a more gradual attractive term at slightly longer ranges. To display the magnitude and direction of each force separately and simultaneously, we decomposed the L-J equation into its two components – attraction and repulsion. The student can vary the atoms’ properties – radius and electronegativity – and change the distance between them with the mouse. The model computes the forces and energy according the distance.
The student controls one of the atoms, moves it closer and further away from the other atom, changing the distance between the atoms. For each distance, the simulation calculates the magnitude and direction of the attractive and repulsive forces and the energy of the system. The forces are represented by arrows. Their length changes with the forces’ magnitude. When the student brings the atom closer to another atom, the arrows both become larger, reflecting that the forces are increasing. The dependence upon distance of the two forces is usually different, with repulsion dominating on very close approach. At equilibrium, or bond length, the length of the arrows is the same, reflecting that the forces are equal. At this point, the atoms vibrate – they are attracted and repelled. As a result of the changing forces, their balance changes continuously, moving the atom erratically around equilibrium. At the same time, the potential energy curve is forming out of the student's motion, and it shows the changes in energy: when the atoms are getting closer it decreases until a minimum where the atoms are bonded. If they approach even closer, the energy increase to a much higher values (Fig. 1). Users can also run the simulation without attraction or without repulsion to learn their meaning and the role of each force in making a chemical bond. In addition, users can vary the radius and electronegativity of each atom to explore and compare different bond types. Future design of the ELI-Chem learning materials will use the same simulation to learn about covalent and ionic bonds.
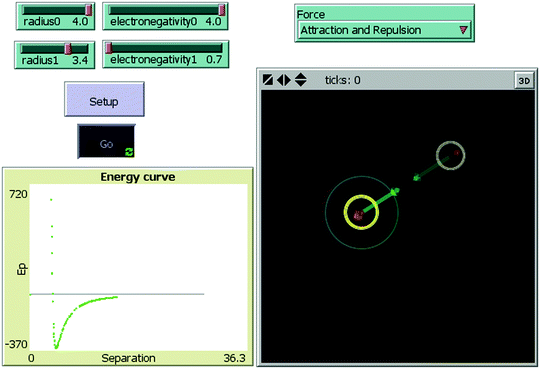 |
| Fig. 1 ELI-Chem screenshot: setup properties of the atoms (top left), setup type of forces (top right), the simulated atoms (on the right), potential–energy curve (bottom left). | |
ELI-Chem activity
The activity was designed to support students’ understanding of the force-based principles underlying chemical bonding and to apply this understanding in recognizing the accompanying energy changes. The sequence of the activity was based on our findings from the previous study (Zohar and Levy, 2019). We noticed that without repulsion forces, students cannot form a dynamic view of the chemical bond, of attraction and repulsion that act simultaneously. Moreover, we found that several components of the scientific model could accrue only in a certain order. Therefore, the activity began with a focus on recognizing the repulsion force, continued with how forces are changed with the distance between the atoms, segued to comparing the repulsion and attraction forces and culminated with the bond as a dynamic equilibrium. Next, students ran the simulation under various “what if” conditions – bonding without attraction or without repulsion. The energy activity began with introducing the energy curve, continued with the relationships to the forces and finished with the imaginary worlds of ‘no attraction’ or ‘no repulsion’. The main tasks and learning goals are introduced in Table 1.
Table 1 ELI-Chem activity main tasks and learning goals
Task |
Learning goals |
Part-I-forces |
Find the smallest distance between the atoms. |
Discovering the forces that are involved in bonding, i.e., attraction and repulsion. |
Explore how each of the forces changes while changing the distance between the atoms. |
Learning the properties of the forces in the context of chemical bonds. |
Compare between the attractive and repulsive forces at various distances along the bonding process. |
Understanding the parallelism between the forces’ relationships and bonding states. In particular, learning that a bond is a dynamic equilibrium between opposing forces. |
Explore the bonding process under imaginary conditions: no attraction or no repulsion. |
Referring to the role and meaning of each force and its impact on bonding. |
|
Part-II-energy |
Explore when energy is released, absorbed, or does not change. |
Acquaintance with the various phases of the energy curve (the L-J curve). |
Compare the relationships of the attractive-repulsive forces and the energy changes along the bonding process. |
Understanding the parallelism between forces’ relationships, bonding states, and the energy of the system; locating these phases on the energy curve. Comprehending that in a stable state, the forces are equal, the net force is zero, and the energy of the system is at minimum. |
Explore the energy in the bonding process under imaginary conditions: no attraction or no repulsion. |
Referring to the role of each force and its impact on the energy; relating these effects to the shape of the energy curve. |
The activity guide included instructions and challenges, questions and explanations. The approach involves learning through exploring models (De Jong and Van Joolingen, 1998), includes “exposing events” (Nussbaum and Novick, 1982), and use the Predict–Observe–Explain structure (White and Gunstone, 1992). The activity had two parts: Part-I-Forces, which introduces the forces as the underlying principles of bonding, and Part-II-Energy, which builds the energy explanations upon the concept of forces.
Methods
Research approach
A combination of qualitative and quantitative methods was used. Clinical interviews were used to collect the data (Ginsburg, 1997). Qualitative analysis of the interviews was conducted, obtaining the main themes (Creswell, 2011). Following this analysis, patterns were explored through visual analysis (Hmelo-Silver et al., 2009).
Participants
The participants included 21 chemistry students from two rural high schools in the northern peripheral region in Israel. The student population in these schools is fairly homogenous, ranging from middle to high socioeconomic status. Participants’ mean age was 15.9 years (SD = 1.1). Their characteristics are detailed in Table 2.
Table 2 Participants’ demographic characteristics
Characteristic |
Number of participants (N = 21) |
Matriculation exam at the highest level after chemistry studies of 3 years as a major subject.
Internal exam at a low level after chemistry studies of 1 year as a compulsory subject.
|
10th grade |
13 |
11th grade |
4 |
12th grade |
4 |
Majoring in chemistry (matriculation exam at 5 credit pointsa) |
19 |
Chemistry as a compulsory subject (2 credit pointsb) |
2 |
Female |
12 |
Male |
9 |
Kibbutz high school |
4 |
Small villages high school |
17 |
Most participants were in the tenth grade, majoring in chemistry. In Israel, all the students study a basic- sciences or chemistry course in tenth grade. Students who choose to major in chemistry study more advanced topics for three years – 10th, 11th and 12th grade. Participants were sampled opportunistically; they all showed willingness and interest in participating in the research. Internal Review Board at the Faculty of Education at the University of Haifa was obtained for the study, as was the approval of the Ministry of Education's chief scientist. Full consent was given by all participants and their parents. At the time of the interviews, all students had already learned the topic of chemical bonding; the topic was taught using the octet rule approach.
Research procedure
The study was framed as a pretest–intervention–posttest design. The students were active during a 1 hour meeting: 10 minutes were devoted to a semi-structured pretest interview; 40 minutes of an online guided activity; and 10 minutes, in the end, were allotted to a semi-structured posttest interview. A total of 21 students were interviewed; 15 students were interviewed individually, and six students were interviewed in dyads. At the time of the interviews, all participants had already learned the topic of chemical bonding, but only some of them (15/21) had learned also about energy changes in chemical bonding. Therefore, all the students conducted the Part-I-Forces activity, and 15 students continued to Part-II-Energy. For the qualitative analysis, we selected two of the 15 students who had learned about energy.
The meetings took place either during recess at school or outside of school hours at the interviewer's house or at the student's school. The first author conducted all the interviews. During the hands-on activities, she answered clarifying questions about how to work with the software. She explained to the students in advance that she would not be allowed to answer questions about chemistry.
Data collection instruments
Data collection tools included a semi-structured interview protocol, students’ filled activity guides, a video camera, and screen-capture software.
The interview protocol was designed to explore students’ understanding of the topic of chemical bonding, especially whether and how they relate bonding to electrical forces. The pre- and posttest interviews were composed of the same questions; the posttest interview included additional questions as to students’ attitudes towards the activity with the simulation (see Appendix 2). The questions were created in-house and refined through several pilot studies. Some of the items have been used in other studies (Griffiths and Preston, 1992; Nicoll, 2001; Teichert and Stacy, 2002; Pabuçcu and Geban, 2006). The activity with the simulation was described above in the “ELI-Chem activity” section.
During the interviews, students were asked to use gestures. According to Goldin-Meadow and Wagner (2005), gestures that accompany speech convey the entire idea, whereas speech conveys several separate ideas, consisting of collected words and fragmented expressions.
Data analysis
The analysis of the interviews was a two-step process. First, we watched the screen-capture movies and read all the transcripts and students’ filled activity guides to conceptualize and extract the knowledge elements. By knowledge elements, we mean basic concepts or ideas upon which students build their answers (Sherin, 2013), such as “atoms are attracted” or “completion to eight.” We also captured students’ gestures while describing the process of bond formation and breaking, looking for common gestures shared by different students.
In the second step, we used visual cluster analysis to depict common patterns in students’ perception of the chemical bond. For each student (a row) the knowledge elements (columns) that were mentioned were highlighted (Fig. 4). The columns and rows were then arranged by descending use. The use of visualizations in educational research supports understanding of how mental models develop and change in context and how knowledge and strategies are constructed (Hmelo-Silver et al., 2009).
Reliability
The first author and a research assistant independently coded the transcripts of five students (24%). The coding results were compared and discussed, and the differences in were resolved. This process resulted in a refined coding scheme and more detailed coding guidelines. Next, the first author and the research assistant independently coded the additional 16 students and examined the inter-rater reliability. Cohen's Kappa coefficient of agreement for the quotes was κ = 0.892. According to Landis and Koch (1977), this is an almost perfect strength of agreement.
Findings
We present students’ conceptual learning by comparing the use of the knowledge elements (Sherin, 2013) before and after working with the ELI-Chem simulation, followed by the students’ resultant pre- and posttest mental models. This two-step analysis is presented separately, first for the forces knowledge elements, then for the energy knowledge elements. Finally, we use qualitative analysis to describe how two students worked with the ELI-Chem simulation, as two contrasting cases.
Conceptual learning: forces in chemical bonding
Change in forces knowledge elements.
Table 3 lists and describes the knowledge elements that students used before and after working with the ELI-Chem force activity, in order of decreasing use in the pretest interview. The change in the use of these knowledge elements is displayed in Fig. 3.
Table 3 Forces knowledge elements, abbreviation
Knowledge element |
Abbreviation |
Description |
Example |
Knowledge elements that showed up only in the posttest interviews.
|
Attraction |
Attraction |
There is an attraction between atoms. |
“A chemical bond is when two atoms are attracted to each other.” |
Electronic level completion |
Completion |
Completion or a full energy level is the reason for bond formation; a bond is most stable when each of the bonded atoms has a full energy level. |
“He [the atom] will search for another Hydrogen and join him in order to complete to eight electrons. This is their most stable state, like the noble gases.” |
Electrons sharing/transfer |
Sharing |
Bond formation is a process of sharing or transferring electrons between atoms “in order to achieve an octet.” |
“The O is looking for two bonds and the H can offer one. If he [the Hydrogen] needs one bond and he [the Oxygen] needs two more bonds then the two H's will be connected to him so that they are happy… everybody has what he needs.” |
Repulsion |
Repulsion |
There is repulsion between atoms. |
“They [the atoms] will approach until both nuclei will repel each other because both are positive.” |
Distance between atoms (implicitly) |
Dist-impl |
The spatial perception of a bond without explicitly mentioning the distance between atoms. This knowledge element was used in the negative form. |
“I think that they will not touch each other.” Or, “I don’t think they are attached.” |
Distance between atoms (explicitly) |
Dist-expl |
The spatial perception of a bond while explicitly mentioning the distance between atoms. |
“They approach and stay [shows a distance between his hands], they don’t continue to be attracted.” |
Attraction–repulsion relationshipsa |
Attr-Repl |
The relationships between the magnitude and direction of repulsive and attractive forces. |
“It happens simultaneously and the forces [attractive and repulsive] should be the same.” |
Dynamics |
Dynamics |
The perception of the dynamics within a bond. Gestures show a continually changing distance between atoms (Fig. 2a), in contrast to a static view in which gestures show atoms still and attached (Fig. 2b). |
“At a certain distance, their nuclei repel each other and then they stop [approaching] and keep small oscillations.” |
Stability is equal forcesa |
Stability-forces |
A stable state of a bond is when attractive forces balance repulsion forces. |
“A stable state between two atoms is when they attract each other with a force that equals the force that they repel each other.” |
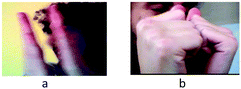 |
| Fig. 2 Gestures showing the dynamic perception of a bond. (a) Dynamic view and (b) static view. | |
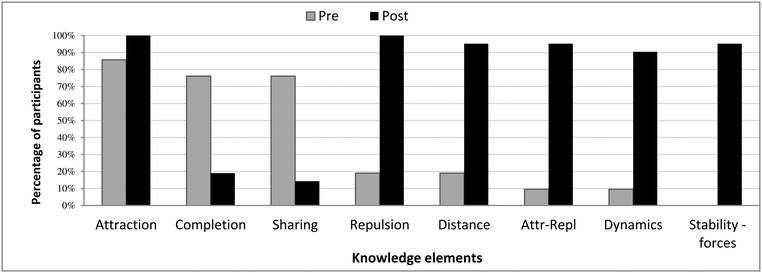 |
| Fig. 3 The change in the use of forces knowledge elements in pre- and posttest interviews (N = 21). | |
Fig. 3 shows a shift in students’ use of knowledge elements after working with the ELI-Chem simulation. Whereas in the pretest most (16/21) students used the octet-based knowledge elements, in the posttest, all students appreciated both attractive and repulsive forces expressed as spontaneously oscillating atoms.
Change in mental models.
We have operationalized mental models as the distribution of knowledge elements for each student. To describe and compare students’ mental models of chemical bonding, visual clustering of the knowledge elements was conducted for the pre- and posttest interviews. For the pretest interview clustering graph, the columns and rows were arranged by descending use of the knowledge elements (Fig. 4a). The posttest interview results are presented in the same order of rows as that for the pretest interviews, to enable comparison (Fig. 4b).
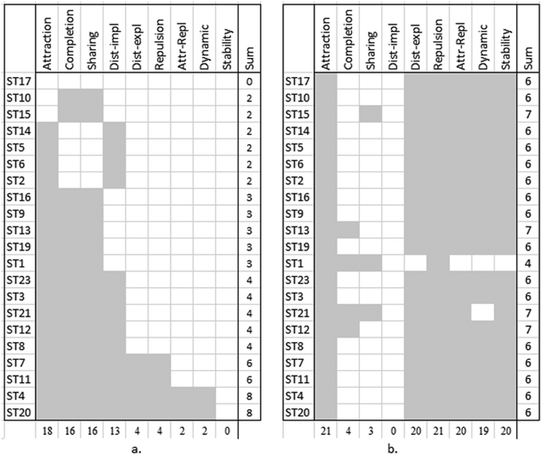 |
| Fig. 4 Visual clustering graphs of the distribution of forces knowledge elements. (a) Pretest knowledge elements and (b) posttest knowledge elements. | |
The visual clustering indicates a strong shift in the group pattern. From the descending trend-line in the pretest interview, which is mostly composed of the octet rule knowledge elements, into a “block” of forced-based knowledge elements. The “descending stairs” trend-line in the pretest (Fig. 4a) signifies a nested relationship – the knowledge elements are built upon each other, rather than being exclusive ways of thinking about chemical bonding (Zohar and Levy, 2019). We identified six types of nesting that compose the six mental models used by students to describe chemical bonding (Fig. 5a). The sketches on the right side of the figure depict the mental models (Fig. 5b). Following the sketches from top to bottom shows how each of the added knowledge elements enriches the next mental model in the hierarchy and leads to a more complete and scientific view of the chemical bond. Thus, attraction or the completion/sharing octet rule knowledge elements are basic for most students, leading to a mental model of attached still atoms. Repulsive forces are added by only those students who also explicitly mentioned the distance between atoms, resulting with a mental model of atoms that are apart, or that there is some distance between them. Finally, dynamic descriptions can take place only after a full force-based model is understood, that is, including both attractive and repulsive forces and understanding that they act simultaneously.
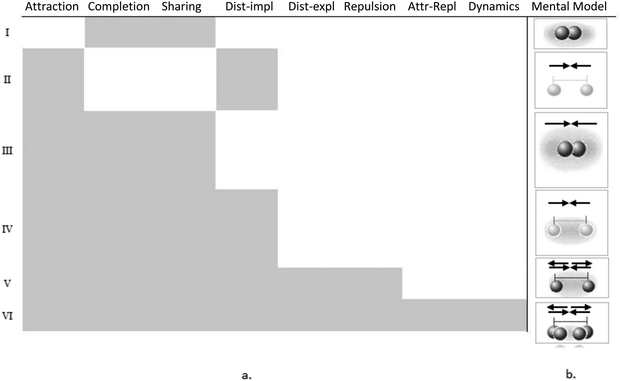 |
| Fig. 5 Pretest mental models of chemical bonding in terms of knowledge elements. (a) Visual clustering of knowledge elements and (b) sketches of students’ mental models of chemical bonding. Reprinted from “Students' reasoning about chemical bonding: The lacuna of repulsion”, by A. R. Zohar and S. T. Levy, J. Res. Sci. Teach., 2019, 1–24. Copyright 2019 by Wiley|JRST. | |
With respect to the pretest, in the posttest, there is a greater similarity between the students’ mental models. The “block” of the force-based knowledge elements (Fig. 4b) is distinct from the pretest results that showed a distribution of models. It reflects that most students (19/21) learned the entire set of knowledge elements and were able to build a force-based runnable model of the chemical bond, the sixth mental model.
Conceptual learning: energy in chemical bonding
Change in energy knowledge elements.
Table 4 describes the energy knowledge elements that students used before and after working with the ELI-Chem energy activity (Part-II). We would like to clarify two things: (1) the ‘stability’ knowledge element appears in both the force and the energy tables (Tables 3 and 4), as it was mentioned in the pre- and posttest interviews for both activities; (2) the energy table (Table 4) includes also the incorrect knowledge elements, such as “energy is required to form a bond.” We decided to include them, as most students used both correct and incorrect knowledge elements, reflecting confusion and contradictions. The change in the frequency of these knowledge elements in students’ explanations is displayed in Fig. 6. As with the forces knowledge elements, also with the energy knowledge elements there is a shift towards the force-based explanation after working with the ELI-Chem simulation (Fig. 6). In the pretest interview, most students (12/15) used the octet rule to explain stability. When asked about energy changes (i.e., when energy is released or required), their answers were based on memorized statements that were unexplainable axioms, such as “When a bond is formed, energy is released.” Most of them (13/15) included both correct and incorrect knowledge elements in their responses, e.g., “energy is required to create a bond (incorrect) and also to break a bond (correct).” Such statements indicate that students were confused, reflecting that they had no reasonable explanation that they could refer to. Another example of lacking a reasonable explanation is ST9, who tried to explain the energetics of bond-breaking by logical elimination: “In order to break a bond you need to invest energy, [so] in order to form a bond, energy is released, right?” Following learning, most students (14/15) used forces to describe bond stability, and the confusion mostly disappeared.
Table 4 Energy knowledge elements, abbreviations, descriptions and examples
Knowledge element |
Abbreviation |
Description |
Example |
For this knowledge element, the term used more frequently by the students is first; after the slash, the scientific term is named.
Incorrect knowledge elements.
|
Stability is equal forces |
Stability-forces |
A stable state of a bond is when attractive forces balance repulsive forces. |
“A stable state between two atoms or two molecules is when they attract each other with a force that equals the force that they repel each other.” |
Bond formation – energy released |
Formation-released |
Chemical bonds release energy when they form. |
“The energy is released while new bonds are being formed.” |
Bond breaking – energy required/absorbeda |
Breaking-required |
Energy is required to break chemical bonds. |
“It is worthwhile energetically for atoms to be in a bond. That is, if we want to break a bond, to destabilize the bond, we need to invest energy so that… so that the atom will be destabilized and the atoms themselves can separate from each other.” |
Bond formation – energy required (I)b |
Formation-required |
Energy is required to form chemical bonds. |
“You need to invest more energy to form it [a bond]. Because you need to push the elements [atoms] together. Like pushing them, bringing them closer to each other.” |
Bond breaking – energy released (I)b |
Breaking-released |
Chemical bonds release energy as they break. |
“We’ve learned in biology that there is some kind of molecule whose internal energy is very very high. So when it breaks down, it releases lots and lots of energy.” |
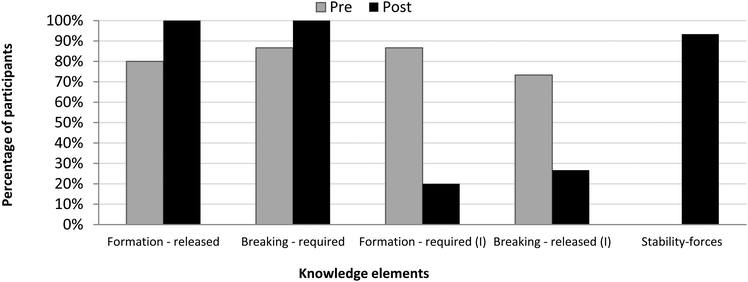 |
| Fig. 6 The change in the use of energy knowledge elements in pre- and posttest interviews (N = 21). | |
Change in mental models.
The same process of visual clustering was done for the energy pre- and posttest knowledge elements (Fig. 7). From the pre- to the posttest interviews there are two main changes that go together: a shift to the force-based explanation and the disappearance of the incorrect knowledge elements. In the pretest interview, we see that all columns are mostly filled except the forces column, which is completely empty. This indicates that without referring to forces, students do not have a clear idea or a logical explanation for the energy changes. They were confused and contradicted themselves, including both correct and incorrect knowledge elements in their descriptions. Following the activity, however, most students (14/15) used forces to explain the energy changes. For example, “When a bond is formed it is spontaneous; the attractive forces are attracted in a way that you don’t need to invest energy” (ST16).
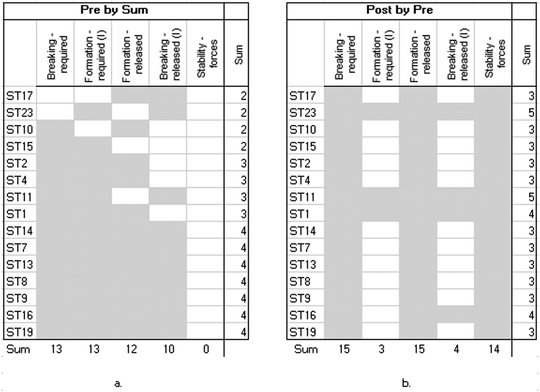 |
| Fig. 7 Visual clustering graphs of the distribution of energy knowledge elements. (a) Pretest knowledge elements and (b) posttest knowledge elements. | |
The process of learning with the ELI-Chem simulation
Two contrasting cases make up the analysis of the learning processes. Miki (ST10) represents the majority of students who shifted from perceiving the bond as static attached atoms to a dynamic equilibrium between forces. Ella (ST1) began the activity in the same position as Miki; however, unlike him, she ended up unable to integrate her previous and new understandings. As such, she represents the minority of students who benefited less from learning with the ELI-Chem environment.
To analyze their processes of learning, we compared their screen-capture videos and their activity guide responses at three points in time. Critical events were selected from the two activity guides: discovering repulsion, identifying the forces, and relating to energy changes (Table 5).
Table 5 Times for activity events
Activity event |
Times in the video of the activity |
Miki |
Ella |
Discovering repulsion |
4:30 |
2:00 |
Identifying the forces |
5:00–6:54 |
4:00–5:30 |
Relating to energy changes |
27:30 |
23:43 |
Discovering repulsion.
The activity begins by displaying on the screen two non-bonded atoms. Forces are hidden. The students are asked to form a chemical bond. Both Ella and Miki reacted similarly when they noticed that they could not attach the two atoms together. They were surprised; they laughed and found it very strange, both saying “this is so weird.” After just a few trials, a rather short duration, Ella said: “I think that there is repulsion or something similar between them [the atoms].” By comparison, the odd experience with the atoms did not create immediate recognition for Miki. He arrived at the idea of repulsion only later when he was asked to explain the atoms’ behavior in writing:
This is probably repulsion that results from the electrical forces [while writing the text, he says “maybe”]; the nuclei of the two atoms repel each other or the electrons… [The student wrote the three dots.]
For both of them this interaction between atoms was unusual and disjointed. They were surprised and even embarrassed as they could not understand what was happening. However, both Ella and Miki benefited from learning with ELI-Chem to discover repulsion – whether by the “exposing event” or later upon reflection by responding to the activity questions. As we have shown previously in the findings (Fig. 5) and elaborated in a previous paper (Zohar and Levy, 2019), understanding repulsion is critical to understanding chemical bonding.
Identifying the forces.
The activity continues with displaying the forces of attraction (green arrows) and repulsion (red arrows; Fig. 1). Students were asked to identify the forces and explain their responses. When Ella was asked “what are the green arrows?” she could not figure out what they are:
“I don’t know… in the beginning, it looks like attraction and then like repulsion. [While writing, she says] “Although I know it is not the correct answer, but it can’t be both [attraction and repulsion], I just know it; it is either attraction or repulsion.” [Finally, she wrote “attraction.”]
Ella could not separate the phenomena into a combination of attraction and repulsion. She could not accept the idea that attraction and repulsion act simultaneously. Consequently, she did not compare attraction and repulsion. This might be related to her idea about the source of repulsion, the octet rule:
“If this [atom] needs two [electrons] to complete [the octet] and this [the second atom] needs one [electron] they will not be attracted, there will be repulsion.”
She considered repulsion as “not completing the octet” rather than a force between charges; therefore, she could not perceive the chemical bond as an equilibrium between two forces. As we will show, this will prevent her from relating the relationships between forces to the distance between atoms and, later on, to changes in energy.
In contrast, Miki understood the dynamic relationships between the atoms. Although he first wrote incorrectly, “The green [arrows] are the repulsion. Because it grows as we approach,” after he compared the size of the forces, he said:
“Wait… maybe it indicates attraction? No, it is probably… The question is which is bigger, I can’t see… The red arrows are bigger, ah, yes! This atom acts on that atom with a force in that direction and that atom activates a force to here, this is the force that pushes them apart, [so] these are the red arrows” [fixes his answer from repulsion to attraction].
We can see how the visualization of the forces – size, direction, and the relationships between them – supported Miki (and most students) in understanding the forces as the principles underlying chemical bonding. In contrast, Ella's ascribing repulsion to octet considerations prevented her from separating between repulsive and attractive forces; in this case, the forces’ representations do not map onto her own explanation and she does not reach a resolution between the two.
Relating to energy changes.
Before running the simulation, both Ella and Miki were confused about the energetics of chemical bonding. Ella thought that energy is required for both breaking and forming a bond. Miki answered correctly that energy is released when a bond is formed and that energy is required to break a bond, however, he could not explain it.
During the activity, Ella could not use forces to explain the energy changes, or interpret the minimum of the energy curve as an equilibrium of equal forces. This results from her lack of understanding of repulsion as a force. Thus, she could not compare attraction and repulsion to make sense of the well in the energy curve.
Ella did not know how to respond to question 10 (Fig. 8). In explaining the phenomena she was experiencing, she talked only about attractive forces. She could not abstract the concept of repulsion from her experiences with the simulation. As a result, she could not explain why energy is required to break the bond.
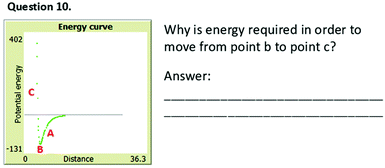 |
| Fig. 8 Question 10 in the activity guide. | |
By contrast, when Miki ran the simulation and observed the resulting energy curve, he immediately made the connection to forces with no additional prompt. While moving the atoms, he said:
“Ahha! So the thing that happens here [energy curve going down] is that attraction is bigger than repulsion until reaching a state of bonding; and when we approach too much then the repulsion is larger and the atoms escape from each other.”
Continuing with the activity guide, Miki used the forces and the energy curve to explain his answers:
“Point b (inFig. 8) is the most energetically stable state, in which the energy is the lowest, meaning that in order to move the atoms apart and in order to make “energy reach zero” [the student wrote the quotation marks], investing energy is required.”
Describing the impact of the simulation.
Finally, we include Ella and Miki's responses in the posttest interview relating to the impact of the simulation.
Ella reflected on her experiences:
Interviewer: Has working with the simulation changed your understanding of chemical bonding?
Ella: Yes, it confused me a little bit.
Interviewer: Why did it confuse you?
Ella: Because I did not think about attractive and repulsive forces. I did not think about it at all and now it got into my head and I don’t know where I should put it. When bringing [the atoms] together there is an attraction and when moving apart then… maybe then there is repulsion, I don’t know. I assume that there is a reasonable explanation for this and then I will understand; but I don’t know.
Ella's gestures reflect that she could not apply the new knowledge element of repulsion. In representing the atoms with her hands, she brings them together until they touch – in both the pretest and the posttest interviews, showing attraction without repulsion (Fig. 9).
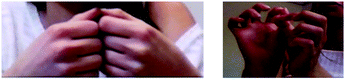 |
| Fig. 9 Ella's gesture description of a chemical bond: pretest interview (left), posttest interview (right). | |
Miki reflected on his experiences in the following way.
Interviewer: Has working with the simulation changed your understanding of chemical bonding?
Miki: It completely changed my understanding. In the previous [pretest] interview I tried to search for the words and the concepts in order to explain and also… it was disconnected from reality because I did not refer to the forces between the atoms at all. I referred only to the stability which I defined as the fact that the atom has a certain number of electrons in the energy levels. Which is correct, it exists, but what really holds the bond is the attractive and repulsive forces between the atoms. So yes, it changed my understanding.
Interviewer: Do you remember what in the simulation changed your understanding? What helped you?
Miki: I think that when I saw the arrows there was a moment that I saw the arrows and I understood that… at a certain stage, the attraction and the repulsion are equal and this is the most stable state. Because in the beginning, I thought that when I [the atom in the simulation] come closer and closer and closer and it is simply repelled, a bond is not being formed, this is what I thought at the beginning. Later on, I understood that the bond is created in the most stable sta… in the nearest state so there is a bond. If I approach more so there won’t be a bond, the bond is breaking.
Miki's gestures reflect his conceptual learning. In the pretest, he brings his hands together until they touch, showing only attraction. In the posttest, he signifies the two forces as arrows with his fingers that work in the opposite direction, showing both repulsion and attraction (Fig. 10).
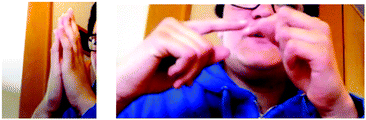 |
| Fig. 10 Miki's gesture description of a chemical bond: pretest interview (left), posttest interview (right). | |
To conclude, while these two students grew to appreciate the existence of repulsion in the chemical bond during the first task of the ELI-Chem simulation, only Miki – as an example to most students – succeeded in building the force-based model. He used the activity prompts and the simulation's objects – visualization of the forces and formation of the energy curve in real time – to replace the naïve knowledge elements with a force-based description.
In comparison, Ella also took advantage of the simulation to learn about the repulsive force. However, she related repulsion to the octet rule explanation without the ability to build upon it or to replace it with the force-based mental model.
Discussion
In this paper, we introduced a learning environment that is based on a force-based approach to learning chemistry and studied its effect on students’ mental models. We used visual clustering and qualitative analyses to explore the data. The visual clustering was used to portray and compare the pre- and posttest mental models, whereas the qualitative analysis was used to examine more thoroughly the learning process that occurred while working with the learning environment.
Our findings show that learning with ELI-Chem overcomes two persistent hurdles that have been highlighted by several leading chemistry education researchers: (1) chemical bonding is based on attractive and repulsive forces, and (2) the relationships between these forces explain the energetics of chemical bonding. Thus, interacting with the dynamic force-based representation is suitable for developing mental models that are more consistent and causal, and include a more scientific and comprehensive set of ideas.
In the following, we discuss the change in students’ mental models followed by the design principles of the learning environment that contributed to this change.
Mental models
The shift in students’ mental models.
Analysis of the pretest interviews shows that most students viewed the chemical bond as two attached static atoms while disregarding the repulsive force, the distance between atoms, and the dynamic character of the system. Holding such a mental model, whether students based their explanations on the attractive force (only) or on the octet rule, cannot lead to a mental model of a dynamic equilibrium between attractive and repulsive forces. By following the knowledge elements that composed each of the mental models (visual clustering; Fig. 4a), we could specify those knowledge elements that are required to build the force-based mental model: attraction, repulsion, the distance between atoms, and dynamics. Only when one refers to the entire set of these knowledge elements is a full force-based model understood.
The nesting of the knowledge elements shows that repulsion and the distance between the atoms were the “bottleneck” in shifting to the force-based approach. Understanding that there is a repulsion between atoms was odd for the students, reflecting that this interaction is outside of their bodily experiences in the world. Once students learned about repulsion, they could explore and understand the effect of the relationships between the forces on the distance between atoms. Through their investigation, they discovered the state of dynamic equilibrium in which the opposing forces are equal. At this stage, the students had a runnable mechanistic mental model (de Kleer and Brown, 1983) of chemical bonding – they were aware of the components and the relationships between them that could emerge into the single dynamic equilibrium of bonding.
Having this mental model in place enabled the integration of the energetic aspect expressed in term of forces. Understanding why energy is released during bond formation or why it is required to break a bond was feasible. The change in their understanding was reflected in two main points: (1) whereas in the pretest interviews they either could not explain their answers or used memorized rules of thumb, in the posttest interviews they were capable of constructing a causal explanation based on forces; and (2) whereas in the pretest interviews they used both correct and incorrect energy knowledge elements, reflecting a state of confusion, in the posttest interviews they used mostly the correct knowledge elements, indicating that their confusion had almost disappeared. These changes indicate that after working with the ELI-Chem environment, students gained new knowledge elements upon which they could build causal explanations. As ST16 said in the posttest interview,
“For example, in thermodynamics, the teacher explained that when you break a bond then you have to invest energy; I did not really understand why. Now I understand that you have to invest energy to overcome the forces of attraction that are spontaneous between the atoms. And this [the simulation] allows me to understand this.”
Mental models in terms of general physical dimensions.
To study the principles by which the students built their mental models, we categorized the knowledge elements by their related theoretical underpinnings. Attraction and repulsion result from forces; therefore, we grouped them as a Force-dimension. Electronic level completion and electron sharing/transfer both relate to the concept of bonding as due to electrons; these were grouped as the Electrons-dimension. Distance between atoms, both implicit and explicit, describes spatial aspects of the bond; these are grouped as the Space-dimension. Dynamic descriptors add the temporal aspect, and these are defined as the Time-dimension. Thus, students use four dimensions when reasoning about chemical bonding: Electrons, Force, Space, and Time. In the pretest, we can see that forces and electrons were most basic whereas the time dimension was referred to by only a few students, showing that it is dependent on perceiving all the other dimensions. In the posttest, however, students used the entire set of four dimensions, reflecting that learning with the ELI-Chem environment enriched their scientific understanding by enabling a step-by-step reasoning through the dynamics in the system.
Design principles of ELI-Chem learning environment
Given the success we have seen in using the design for force-based learning about bonding, we turn to a more systematic presentation of the learning environment's unique set of design principles and demonstrate them with short examples.
Restructuration.
Reframing content in light of learnability and productivity considerations (Wilensky and Papert, 2010) forms the most basic rationale for this study. Learnability involves choosing representations that enable causal reasoning with coherent and simple causes. Productivity relates to the range of phenomena to which the representation can be applied. In creating the dynamic schema of equilibrium for chemical bonding, understanding the forces that generate this dynamic equilibrium becomes accessible to learners.
Mechanistic reasoning.
Mechanistic explanation focuses on the processes that underlie cause-effect relationships (Russ et al., 2008). Mechanistic reasoning is (1) characterized by causal reasoning, as it explains the process by which a cause brings about an effect; (2) nonteleological, in contrast to goal-driven explanations of change in the system; and (3) built from experience – p-prims are used to assign causal explanations for what happens under certain circumstances (diSessa, 1993). Reasoning about mechanisms is an important aspect of students’ inquiry processes, as they seek an explanation of how things happen. It is a potentially taxing cognitive activity, and external models provide the “vehicle for keeping in mind all the complex interactions among the operations” (Russ et al., 2008). Observing the changes in the forces along the bonding process grounds students’ explanations in the underlying principles of chemical bonding.
Content is aligned with standards and attends to students’ difficulties.
Standards and curriculum frameworks set the expectations for what ideas are important to learn and what students should know and are able to do at each grade level (NGSS, 2013). The standards ensure that students will develop an in-depth understanding of the appropriate content and key skills. According to the NGSS (2013), the topic of chemical bonding should be taught under the framework of the force-based explanation. Based on the literature, students have difficulties relating chemical bonding to forces; therefore, they rely on heuristics or rote memorization (Taber and Coll, 2002; Özmen, 2004; Ünal et al., 2006; Levy-Nahum et al., 2010). Instead of using rules of thumb to predict whether energy will be released or absorbed, students can use the simulation to visualize and understand the force-based reasons for the energy changes.
Participation in the simulation.
Rather than observing a simulation, a participatory simulation allows the learner to be an individual entity in the simulation, to embody a virtual component in the model (Langbeheim and Levy, 2018). By dragging the atom, the user embodies the atom's movement toward and apart from the other atom while “feeling” the pushes and pulls, or the acting attractive/repulsive forces.
Learning through exploring models.
Being visual, dynamic, and manipulable, the computer simulation environment is very suited for discovery learning. It allows inferring, through experimentation, the characteristics of the model underlying the simulation (De Jong and Van Joolingen, 1998). Furthermore, the extensibility and adjustability of the models enable students to engage in real inquiry by asking what-if questions of the models and adjusting the rules to answer their questions (Wilensky and Papert, 2010). By controlling the acting forces in the ELI-Chem simulation (e.g., only attraction, only repulsion) learners can explore a scenario of chemical bonding in a world without repulsion or without attraction, highlighting the dynamic equilibrium concept.
Multiple linked representations.
A linkage between different representations of the same concepts allows students to map features of one representation onto those of another. Thus, the actions that a student takes with one representation can correspond to certain outcomes in another representation. This affords certain ways of thinking and inferring about the underlying entities and processes (Kozma, 2003). In ELI-Chem, the force-based dynamic molecular representations are connected to the energy graph representation, showing the linkage between forces, energy changes, and inter-atomic distance.
Instructional strategy of conceptual conflicts.
Understanding necessitates, first of all, a recognition by the learner of a problem and his or her inability to solve it with existing conceptions (Dewey 1910; in Nussbaum and Novick, 1982). To achieve this purpose, Nussbaum and Novick (1982) suggest initiating instruction with an “exposing event” – a phenomenon that makes students aware of the differences between their predictions and the observed phenomenon, inviting them to explain the conflict in their own words. In our case, asking the students to bring the atoms together as close as they can supported a change in their mental model of chemical bonding. Most students experienced conceptual conflict: in contrast to their expectations, the atoms could not attach. Searching for an explanation, they became aware of the repulsion between atoms.
Limitations and future directions
The first limitation concerns the limited scope of learning topics in the current ELI-Chem activity. It is important to know that we have also merged the Lennard-Jones framework with electronegativity to support learning of topics that are known as difficult to understand (see Appendix 1): (1) the continuum of ionic-covalent bonding; (2) the energy of the system in comparison to energy of the environment; and (3) the relationships between the concepts of energy and force. Future research will test to what degree these additional changes and activities will support learning.
The second limitation is the sample size. In balancing the study's depth and breadth, we interviewed 21 high school chemistry students who described and explained their understanding of chemical bonding. These explanations were then analyzed through a knowledge elements perspective, aggregating in six mental models of chemical bonding. The interviews were extensive and the analyses were in-depth using both top-down and bottom-up methods. Thus, in the balance between a larger sample to increase validity and the advantages of qualitative analyses in producing a deeper understanding of the phenomenon, the study was conducted with this number of students. It may be that not all possible knowledge elements or mental models of chemical bonding have been revealed, but we do think that an in-depth analysis that reflects the changes in students’ mental models has been constructed. A related limitation concerns the analysis method used. With a large number of participants, one could do a factor analysis or clustering process with statistical software. However, this was not feasible in the present study because of the smaller sample.
The third limitation is in the single group research design, in which the pretest's impact on students’ thinking is not controlled for.
Conclusions and implications
This paper introduces a study into students’ learning of the force-based explanation of chemical bonding through an interactive, visualized, and dynamic representation. The ELI-Chem learning environment – simulation and activity guide, makes the abstract molecular world accessible by representing the force-based mathematical model of chemical bonding (Jones, 1924) through visualized objects. Its design principles integrate conceptual structures of knowledge and support for the learning process. By setting the force-based model in motion the ability to imagine a changeable system is supported. By separating the Lennard-Jones equation into its attraction and repulsion terms, students’ could control variables to explore the process of bonding. They could control each of the forces, gradually forming an understanding of the role and meaning of each force. The linked representations of the forces and energy foster causal mechanistic reasoning. The activity guide takes the advantages of an interactive simulation, encouraging students to learn through discovering. Moreover, the activity sequence addresses the student's lack of particular knowledge elements, guiding them to build the integrated force-based mental model gradually. Our findings show that learning with the ELI-Chem environment provided students the vocabulary, concepts, principles, and analogical sensorimotor schemes that are required to shift to a more force-based explanation. From a simple mental model based on one or two basic knowledge elements (i.e., attraction and/or electrons), they shifted to the more complex mental model that is composed of and relates the concepts of forces, distance, and dynamic knowledge elements to each other.
The uniqueness of this work stems mainly from the fact that the design of the learning environment, both the simulation and the activity, was based on a combination of three aspects: the scientific model of the chemical context, the principles of the pedagogical design and the results of a previous study into the process of learning.
Based on our findings, we make two main recommendations. One concerns both teaching chemical bonding, but more generally when teaching about dynamic equilibrium phenomena. Our recommendation is emphasizing the existence of the two opposing forces acting simultaneously in a chemical bond – attraction and repulsion. The technology of the computer simulation allows visualizing the forces and their relative magnitudes as they shift and combine with even minute movements of one atom with respect to another. Teachers can demonstrate that the most stable state is a dynamic state in which the atoms are continuously attracted and repelled, moving around the point of equilibrium at bond-length.
The second recommendation is for a design that is based on how students’ ideas evolve. The accompanying activities should relate to students’ mental models. According to Özmen's review (2004), teachers are not aware of students’ difficulties and alternative conceptions in learning science concepts. Resulting from this, students do not fully grasp the studied concepts. Based on a Knowledge in Pieces view of learning (diSessa, 1993), research may help teachers in noticing what fragments of knowledge are not integrated into the students’ explanations. Following this, the process of learning can be supported by presenting the missing parts and helping students construct a more consistent mental model. Envisioning or running a mental model takes place only when the system's entities are placed in space and their interactions are fully defined (de Kleer and Brown, 1983).
Conflicts of interest
There are no conflicts to declare.
Appendix 1: the mathematical equations of the ELI-Chem simulation
The ELI-Chem model is based on Lennard-Jones eqn (1) that approximates the interaction between a pair of neutral atoms or molecules. It is worth mentioning that the Lennard-Jones equation is not the most accurate representation of the potential energy, but rather a simplified form that is used for simulation software.
As students move an atom on the screen, they change the distance between the atoms. For each distance, the attractive and repulsive forces, and the potential energy are calculated and displayed.
The common expression of Lennard-Jones equation is:
| 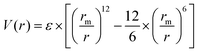 | (1) |
where:
• V(r) – is the potential energy between the two atoms.
• ε – is the well depth and a measure of how strongly the two atoms attract each other.
• rm – is the distance at which the potential energy between the two atoms is zero. It gives a measurement of how close the two atoms can approach and is equals to half the distance between atoms’ center. Thus, in the ELI-Chem model:
• r – is the distance between the atoms as set by the student (measured from the center of one atom to the center of the other atom).
The
term describes the repulsion at short ranges and the
term describes the attraction at long ranges. To display the attractive force and repulsive force separately, we created variables for each force. The energy is the difference between these terms. Thus,
• 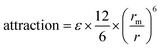
• 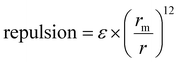
• potential energy = repulsion − attraction
Ionic-covalent bond character
Since one of our goals was simulating the whole scale of ionic-covalent bond type, we changed the attractive term to include a changeable parameter, b, as follows:
where:
• b – is a linear function of the ionic character of a bond which we call “pic” (percent ionic covalent). Thus,
• pic – is the percent ionic character of a bond; it is a function of the difference between the electronegativity of the elements in the bond (student's setup). Thus, we define pic as
(Fig. 11).
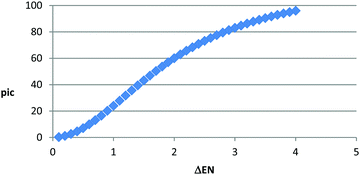 |
| Fig. 11 Percent ionic-covalent vs. difference between atoms’ electronegativity. | |
Appendix 2: pre- and posttest interview protocols
Pretest interview |
Posttest interview |
Part-I-Forces |
1. What is a chemical bond? |
1. What is a chemical bond? |
2. Why do atoms bond? |
2. Why do atoms bond? |
3. How close can atoms approach one another? |
3. How close can atoms approach one another? |
4. What holds atom together? |
4. What holds atom together? |
5. What is bond stability? |
5. What is bond stability? |
6. How would you explain to your friend (that didn’t yet learn about bonding) what a chemical bond is? Please use gestures. |
6. How would you explain to your friend (that didn’t yet learn about bonding) what a chemical bond is? Please use gestures. |
7. Which example from everyday life would you use in your explanation of bonding? A chemical bond is like….? |
7. Most students used a magnet as an example for chemical bond. Do you think that this example is an appropriate representation of chemical bond? Why or why not? Would you use the same example you used before working with the simulation? |
|
8. Did the simulation change your understanding of chemical bonding? How did you understand it [pending student's response] from the simulation? Please provide details. |
|
9. Currently, this simulation is under development. What would you change or how would you improve the simulation? |
|
Part-II-Energy |
1. Why do atoms form bonds? |
1. Why do atoms form bonds? |
2. What is bond stability? |
2. What is bond stability? |
3. During which phase of chemical bonding is energy released, bond formation or bond breaking? Why? |
3. During which phase of chemical bonding is energy released, bond formation or bond breaking? Why? |
4. For which phase of the bonding process, energy is required: to form a bond or to break a bond? Why? |
4. For which phase of chemical bonding, energy is required: to form a bond or to break a bond? Why? |
5. What does it mean ‘high-energy’ bond? |
5. What does it mean ‘strong bond’? and ‘weak bond’? |
6. What does it mean ‘strong bond’? and ‘weak bond’? |
6. Did the simulation change your understanding of chemical bonding? How did you understand it [pending student's response] from the simulation? Please provide details. |
7. Compare the formation of Hydrogen molecule from two Hydrogen atoms to the formation of Nitrogen molecule from two Nitrogen atoms. In which process more energy is released? |
7. Currently, this simulation is under development. What would you change or how would you improve the simulation? |
Acknowledgements
Special thanks to Mrs Elisheva Geva, the chemistry teacher who welcomed the first author into her chemistry classes. Observing Elisheva and her students helped me become aware of students' questions and confusions. We would like to thank Dr Ido Gilary for the thoughtful scientific advice on developing the mathematical model, to Dr Malka Yinon for the kind support and enabling in using her bank of chemistry items, and to Danielle Menuhin for her help in explaining the logic behind the visual clustering analysis. This research was supported through a scholarship by the Ministry of Science, Technology and Space, Israel (Grant No. 3-13260).
References
- Abrahamson D. and Lindgren R., (2014), Embodiment and embodied design, in Sawyer R. K. (ed.), The Cambridge handbook of the learning sciences, 2nd edn, Cambridge, UK: Cambridge University Press, pp. 358–376.
- Bader F. W., (n.d.), An Introduction to the Electronic Structure of Atoms and Molecules, retrieved April 03, 2018, from http://www.chemistry.mcmaster.ca/esam/Chapter_6/section_1.html.
- Bain K. and Towns M., (2016), A review of research on the teaching and learning of chemical kinetics, Chem. Educ. Res. Pract., 17(2), 246–262.
- Barsalou L. W., (1999), Perceptions of perceptual symbols, Behav. Brain Sci., 22(4), 637–660.
- Becker N. M. and Cooper M. M., (2014), College Chemistry Students’ Understanding of Potential Energy in the Context of Atomic–Molecular Interactions, J. Res. Sci. Teach.51, 789–808.
- Bergqvist A. and Chang Rundgren S.-N., (2017), The influence of textbooks on teachers’ knowledge of chemical bonding representations relative to students’ difficulties understanding, Res. Sci. Technol. Educ., 35(2), 215–237.
- Bergqvist A. C., Drechsler M., de Jong O. and Chang Rundgren S.-N., (2013), Representations of Chemical Bonding models in School Textbooks – Help or Hindrance for Understanding? Chem. Educ. Res. Pract., 14, 589–606.
- Boo H. K., (1998), Students' understandings of chemical bonds and the energetics of chemical reactions, J. Res. Sci. Teach., 35(5), 569–581.
- Creswell J., (2011), Educational Research: Planning, Conducting, and Evaluating Quantitative and Qualitative Research, 4th edn, Boston: Pearson.
- Croft M. and de Berg K., (2014), From Common Sense Concepts to Scientifically Conditioned Concepts of Chemical Bonding: An Historical and Textbook Approach Designed to Address Learning and Teaching Issues at the Secondary School Level, Sci. Educ., 23(9), 1733–1761.
- de Jong O. and Taber K. S., (2014), The many faces of high school chemistry, Handbook Res. Sci. Educ., 2, 457–480.
- De Jong T. and Van Joolingen W. R., (1998), Scientific discovery learning with computer simulations of conceptual domains, Rev. Educ. Res., 68(2), 179–201.
- de Kleer J. and Brown J. S., (1983), Assumptions and ambiguities in mechanistic mental models, in Gentner D. and Stevens A. (ed.), Mental models, New York: Psychology Press, pp. 155–190.
- Dewey J., (1910), How we think, Boston: Heath.
- Dhindsa H. S. and Treagust D. F., (2014), Prospective pedagogy for teaching chemical bonding for smart and sustainable learning, Chem. Educ. Res. Pract., 15, 435–446.
- diSessa A. A., (1993), Toward an epistemology of physics, Cognit. Instruct., 10(2–3), 105–225.
- Dreyfus B. W., Gouvea J., Geller B. D., Sawtelle, V., Turpen, C. and Redish E. F., (2014), Chemical energy in an introductory physics course for the life sciences, Am. J. Phys., 82(5), 403–411.
- Driver R., (1994), Making sense of secondary science: Support materials for teachers, Psychology Press.
- Erman E., (2017), Factors contributing to students’ misconceptions in learning covalent bonds, J. Res. Sci. Teach., 54(4), 520–537.
- Gilbert J. K., de Jong O., Justi R., Treagust D. F. and van Driel J. H, (ed.), (2006), Chemical education: towards research-based practice, Springer Science and Business Media, vol. 17.
- Gillespie R. J., (1997), The great ideas of chemistry, J. Chem. Educ., 74(7), 862.
- Ginsburg H., (1997), Entering the child's mind: the clinical interview in psychological research and practice, Cambridge University Press.
- Goldin-Meadow S., and Wagner S., (2005), How our hands help us learn, Trends Cognit. Sci., 9(5), 234–241.
- Griffiths A. K. and Preston K. R., (1992), Grade-12 students’ misconceptions relating to fundamental characteristics of atoms and molecules, J. Res. Sci. Teach., 29(6), 611–628.
- Hapkiewicz A., (1991), Clarifying chemical bonding: overcoming our misconceptions, Sci. Teach., 58(3), 24.
- Hmelo-Silver C. E., Liu L., and Jordan R., (2009), Visual representation of a multidimensional coding scheme for understanding technology-mediated learning about complex natural systems, Res. Pract. Technol. Enhanced Learn., 4(03), 253–280.
- Joki J. and Aksela M., (2018), The challenges of learning and teaching chemical bonding at different school levels using electrostatic interactions instead of the octet rule as a teaching model, Chem. Educ. Res. Pract., 19(3), 932–953.
- Joki J., Lavonen J., Juuti K. and Aksela M., (2015), Coulombic interaction in Finnish middle school chemistry: a systemic perspective on students’ conceptual structure of chemical bonding, Chem. Educ. Res. Pract., 16(4), 901–917.
- Jones J. E., (1924), On the determination of molecular fields II: from the equation of state of a gas, Proc. R. Soc. London A: Math., Phys. Eng. Sci., 106(738), 463–477.
- Krajcik J., McNeill K. L., and Reiser B. J., (2008), Learning-goals-driven design model: developing curriculum materials that align with national standards and incorporate project-based pedagogy, Sci. Educ., 92(1), 1–32.
- Kozma R., (2003), The material features of multiple representations and their cognitive and social affordances for science understanding, Learn. Instruct., 13(2), 205–226.
- Kozma R. and Russell J., (2005), Students becoming chemists: Developing representational competence, in Visualization in science education, Dordrecht: Springer, pp. 121–145.
- Lakoff G. and Johnson M., (1980), The metaphorical structure of the human conceptual system, Cognit. Sci., 4(2), 195–208.
- Langbeheim E. and Levy S. T., (2018), Feeling the forces within materials: bringing inter-molecular bonding to the fore using embodied modelling, Int. J. Sci. Educ., 40(13), 1567–1586.
- Landis J. R. and Koch G. G., (1977), The measurement of observer agreement for categorical data, Biometrics, 33(1), 159–174.
- Levy-Nahum T., Mamlok-Naaman R., Hofstein A. and Krajcik J., (2007), Developing a new teaching approach for the chemical bonding concept aligned with current scientific and pedagogical knowledge, Sci. Educ., 91(4), 579–603.
- Levy-Nahum T., Mamlok-Naaman R., Hofstein A. and Taber K. S., (2010), Teaching and learning the concept of chemical bonding, Studies in Science Education, 46(2), 179–207.
- Lindsey B. A., (2014), Student reasoning about electrostatic and gravitational potential energy: an exploratory study with interdisciplinary consequences, Phys. Rev. ST – Phys. Educ. Res., 10(1).
- McCloskey M, (1983), Naive theories of motion, in Mental models, D. Gentner and A. L. Stevens, (ed.), Hillsdale, NJ: Lawrence Erlbaum Associates Inc., pp. 299–324.
- Nagel M. L. and Lindsey B. A., (2015), Student use
of energy concepts from physics in chemistry courses, Chem. Educ. Res. Pract., 16(1), 67–81.
- NGSS Lead States, (2013), Next Generation Science Standards: For States, By States, Washington, DC: The National Academies Press, retrieved November 23, 2017 from https://www.nextgenscience.org/.
- Nicoll G., (2001), A report of undergraduates’ bonding misconceptions, Int. J. Sci. Educ., 23(7), 707–730.
- Norman D. A., (1983), Some observations on mental models, in D. Gentner and A. L. Stevens (ed.), Mental models, Hillsdale, NJ: Erlbaum, pp. 7–14.
- Nussbaum J. and Novick S., (1982), Alternative frameworks, conceptual conflict and accommodation: toward a principled teaching strategy, Instruct. Sci., 11(3), 183–200.
- Özmen H., (2004), Some student misconceptions in chemistry: a literature review of chemical bonding, J. Sci. Educ. Technol., 13(2), 147–159.
- Özmen H., (2008), Determination of students’ alternative conceptions about chemical equilibrium: a review of research and the case of Turkey, Chem. Educ. Res. Pract., 9(3), 225–233.
- Pabuçcu A. and Geban Ö., (2006), Remediating misconceptions concerning chemical bonding through conceptual change text, Hacettepe Üniversitesi Eğitim Fakültesi Dergisi, 30(30).
- Reiner M., Slotta J. D., Chi M. T. and Resnick L. B., (2000), Naive physics reasoning: a commitment to substance-based conceptions, Cognit. Instruct., 18(1), 1–34.
- Russ R. S., Scherr R. E., Hammer D. and Mikeska J., (2008), Recognizing mechanistic reasoning in student scientific inquiry: a framework for discourse analysis developed from philosophy of science, Sci. Educ., 92(3), 499–525.
- Sherin B., (2013), A Computational Study of Commonsense Science: An Exploration in the Automated Analysis of Clinical Interview Data, J. Learn. Sci., 22(4), 600–638.
- Shusterman A. J. and Shusterman G. P., (1997), Teaching Chemistry with Electron Density Models, J. Chem. Educ., 74(7), 771.
- Stevens S. Y., Delgado C. and Krajcik J. S., (2010), Developing a hypothetical multi-dimensional learning progression for the nature of matter, J. Res. Sci. Teach., 47(6), 687–715.
- Taber K. S., (1998), The sharing-out of nuclear attraction: or ‘I can't think about physics in chemistry’, Int. J. Sci. Educ., 20(8), 1001–1014.
- Taber K. S., (2003), Mediating mental models of metals: acknowledging the priority of the learner's prior learning, Sci. Educ., 87(5), 732–758.
- Taber K. S. and Coll R. K., (2002), Bonding, in Gilbert J. K., de Jong O., Justi R., Treagust D. F. and Van Driel J. H. (ed.), Chemical Education: Towards Research-based Practice, Kluwer Academic Publishers, pp. 213–234.
- Talanquer V., (2007), Explanations and Teleology in Chemistry Education, Int. J. Sci. Educ., 29(7), 853–870.
- Teichert M. A. and Stacy A. M., (2002), Promoting understanding of chemical bonding and spontaneity through student explanation and integration of ideas, J. Res. Sci. Teach., 39(6), 464–496.
- Tsaparlis G., Pappa E. T. and Byers B., (2018), Teaching and learning chemical bonding: research-based evidence for misconceptions and conceptual difficulties experienced by students in upper secondary schools and the effect of an enriched text, Chem. Educ. Res. Pract.
- Ünal S., Çalık M., Ayas A. and Coll R. K., (2006), A review of chemical bonding studies: needs, aims, methods of exploring students’ conceptions, general knowledge claims and students’ alternative conceptions, Res. Sci. Technol. Educ., 24(2), 141–172.
- Venkataraman B., (2017), Emphasizing the Significance of Electrostatic Interactions in Chemical Bonding, J. Chem. Educ., 94(3), 296–303.
- White R. and Gunstone R., (1992), Probing understanding, London: The Falmer Press.
- Wieman C. E, Adams W. K and Perkins K. K., (2008), PhET: simulations that enhance learning, Science, 322(5902), 682–683.
- Wilensky U., (1999), NetLogo, http://ccl.northwestern.edu/netlogo/, Center for Connected Learning and Computer-Based Modeling, Evanston, IL: Northwestern University.
- Wilensky U. and Papert S., (2010), Restructurations: Reformulations of knowledge disciplines through new representational forms, Constructionism.
- Xie Q. and Pallant A., (2011), The molecular workbench software: an innovative dynamic modeling tool for nanoscience education, in Khine M. S. and Salch I. M. (ed.), Models and modeling: cognitive tools for scientific enquiry, New York: Springer, pp. 121–132.
- Zohar A. R. and Levy S. T., (2015), ELI-Chem (Embodied Learning Interactive-Chemistry): learning through interacting with atoms, Developed in the Systems Learning & Development Lab (SLDL) at the University of Haifa.
- Zohar A. R. and Levy S. T., (2019), Students' reasoning about chemical bonding: the lacuna of repulsion, J. Res. Sci. Teach., 1–24.
|
This journal is © The Royal Society of Chemistry 2019 |