DOI:
10.1039/C9RA06876G
(Paper)
RSC Adv., 2019,
9, 41287-41297
Preparation and in vitro bioactivity evaluation of N-heterocyclic-linked dihomooxacalix[4]arene derivatives†
Received
29th August 2019
, Accepted 22nd November 2019
First published on 13th December 2019
Abstract
Based on the superior prospects of calixarenes-based agents and N-heterocyclic pharmacophores in biomedical applications, 14 new dihomooxacalix[4]arene N-heterocyclic (pyridine, quinoline, and thiazole) derivatives 4a–4n were efficiently synthesized from the parent compound, namely, p-tert-butyldihomooxacalix[4]arene 1; they were further investigated by using their IR, 1H NMR, 13C NMR, and HRMS spectra. Among these derivatives, the crystal and molecular structures of 2-aminomethyl-pyridine-substituted dihomooxacalix[4]arene 4f (obtained from methanol) have been determined by X-ray diffraction. In the case of the inhibition assay of cell growth, we evaluated the effects on four select tumor cell lines (MCF-7, HepG2, SKOV3, and HeLa), as well as the normal cell lines of HUVEC, using paclitaxel as the positive control drug. It was found that the derivatives 4d–4f, 4i, 4k, and 4l could inhibit tumoral activity up to varying degrees. Mechanistically, the cell cycle analysis demonstrated that dihomooxacalix[4]arene N-heterocyclic derivatives could induce apoptosis of MCF cells. In addition, the results of the western blot and immunofluorescence studies revealed the upregulation of the protein expression levels of Bax and cleaved caspase-3, as well as the downregulation of Bcl-2, which are in good agreement with the corresponding inhibitory potencies. Therefore, these findings suggest that N-heterocyclic derivatives based on the dihomooxacalix[4]arene scaffold are promising candidates for use against cancer.
Introduction
From the past several decades, cancer has been predominantly considered to be one of the most critical health problems worldwide. Recently, the International Agency for Research on Cancer (IARC) reported that in 2018, the number of new cancer cases is expected to reach 18.1 million and cancer deaths reaching 9.6 million.1 Research on designing anticancer agents is also growing fairly rapidly and broadly, leading to drug diversity.2 It is well known that conventional chemotherapy based on synthetic drugs has occupied a critical position in the treatment of cancer.3 However, it suffers from severe nonselectivity, drug resistance, and other side-effects.4 In this way, cancer research is still a formidable challenge.
With regard to conventional chemotherapy, supramolecular chemotherapy can prove to be a highly promising candidate because it can resolve the drawbacks of traditional chemotherapy by using host–guest interactions such as π–π stacking effects, electrostatic interactions, and hydrogen bonding interactions with small drug molecules.5 Calixarenes—the third generation of supramolecules—are an important class of cyclic oligomers formed by the condensation of multiple phenol units and formaldehyde under alkaline conditions.6 In the last few decades, owing to their flexible conformations, variable cavity dimensions, easy modifications, and limited toxicity and immune responses, research on the therapeutic applications of calixarenes and their derivatives has formed an emerging area of interest.7 A large number of calixarene derivatives have been reported as drug building blocks.8
It has been proven that N-heterocyclic compounds, such as quinoline, pyridine, pyrimidine, anthraquinone, benzimidazole, and so on, have high efficiency and enhanced structural diversity, because of which they have become a research hotspot in the field of pesticides and medicines.9 Many natural products (e.g., camptothecin, reserpine, and analgesic morphine) or chemically synthesized drugs have exhibited biological activity mainly due to the presence of N-heterocyclic groups.10 The introduction of N-heterocyclic units in well-defined calixarene scaffolds has been proven to be feasible and useful. For example, quinoline–pyrimidine-linked calix[4]arene scaffolds exhibited worthwhile antimalarial activity against the P. falciparum strain.11 Further, 8-oxyquinolinepropoxycalix[4]arene and 5-Cl-8-oxyquinolinepropoxycalix[4]arene exerted antifungal activity against C. albicans.12 In addition, pyrrolidine-appended calix[4]arene exhibited excellent antifungal action toward A. niger.13 Homooxacalixarenes are an analog of calixarenes,14 where the methylene groups (–CH2–) are partially or totally replaced by the oxypropylene group (–CH2OCH2–). They have improved conformational mobility and structural flexibility characteristics; moreover, they are much more suitable for use in an interesting framework for the new drug design of a molecular platform. In the present work, we are interested to formulate the pyridine, quinoline, or thiazole groups via the amido acetoethoxy spacer to the lower rim of dihomooxacalix[4]arene to afford novel N-heterocyclic-amido-attached dihomooxacalix[4]arene derivatives. Further, the antitumor effects of these compounds on breast (MCF-7), liver (HepG2), ovarian (SKOV3), and cervical (HeLa) cancer cells were evaluated, as well as the possible mechanisms were discussed.
Results and discussion
Synthesis and characterization
The synthesis strategy for dihomooxacalix[4]arene N-heterocyclic derivatives 4a–4n are shown in Scheme 1.
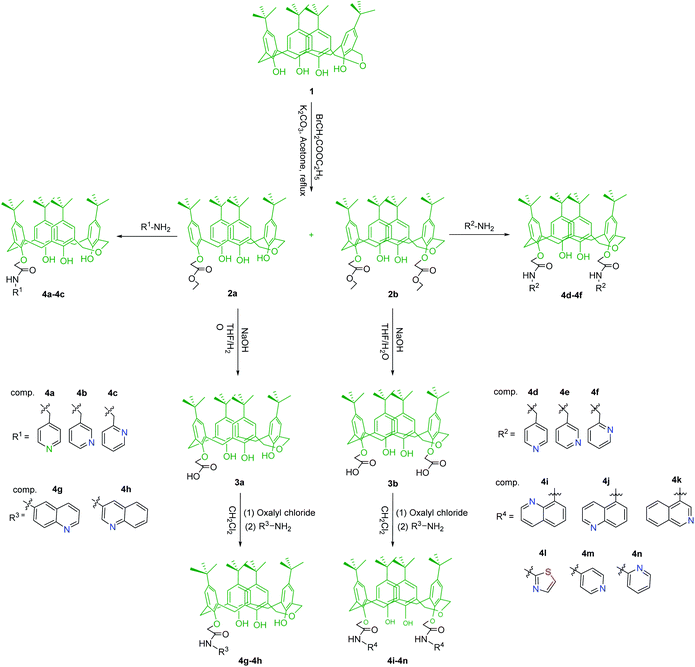 |
| Scheme 1 Synthesis of dihomooxacalix[4]arene N-heterocyclic derivatives 4a–4n. | |
Evidently, compounds 4a–4n were synthesized starting from tert-butyl dihomooxacalix[4]arene 1.
For attaching the N-heterocyclic units to the lower rim of the dihomooxacalix[4]arene scaffold, we initially introduced ester groups to form an acetoethoxy spacer using a moderate condition similar to the procedures reported by Marcos and us.8d,15,16 We found that compound 1 easily reacted with ethyl 2-bromoacetate and potassium carbonate at a molar ratio of 1
:
2
:
12 in refluxing acetone, yielding a mixture of nearly 1
:
1 mono and 1,3-disubstituted ester 2a and 2b, which were separated and purified by silica gel column chromatography (4
:
1 v/v, petroleum ether/ethyl acetate). The further reaction of ester 2a or 2b with excess 4-aminomethylpyridine was carried out at the ambient temperature. The consequent reaction was rapid and the corresponding products of 4a and 4d were readily obtained in the form of white solids in good yields of 90.1% and 93.2%, respectively. In addition, the analogs of the above compounds bearing 3- or 2-aminomethyl pyridine moieties (4b–4c; 4e–4f) were yielded varying from 82.9% to 94.5%. However, the same reaction, when conducted with 4-aminopyridine, hardly proceeded and failed to yield the expected compound with more hindrance and less activity, regardless of improving the reaction conditions. Hydrolysis was subsequently conducted by the treatment of intermediates 2a and 2b with sodium hydroxide in aqueous THF to yield carboxylic acids 3a and 3b, respectively. Thereafter, carboxylic acid formed into acid chloride, followed by amidation with amino-N-heterocyclic compounds in dry THF in an ice bath for 24 h, affording 4g–4n in a quantitative yield.
Such synthetic dihomooxacalix[4]arene N-heterocyclic derivatives 4a–4n have not been reported before; their structures were confirmed from their 1H NMR, 13C NMR, and HRMS spectra. As expected, the 1H NMR spectra of 4a exhibited three single signals at 8.86, 8.49, and 7.80 ppm for protons of the unreacted free phenolic hydroxyl groups. A triple singlet for the protons of the –NH– group appeared at 9.31 ppm. Aromatic protons appeared at 7.28, 7.16, 7.12–7.14, 7.09, 6.93, and 6.91 ppm for the complicated characteristic resonances. In addition, the chemical shift at 8.53 (d) and 7.39 (d) ppm, arose from the aminomethyl pyridine ring, further revealing the successful preparation of 4a.
X-ray crystal structure of 4f
The X-ray crystal structure of derivative 4f was determined, as shown in Fig. 1.
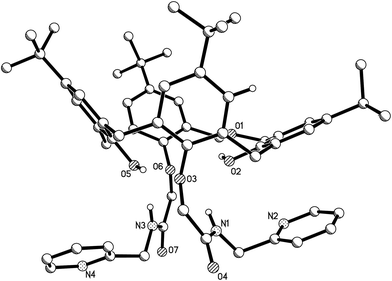 |
| Fig. 1 Molecular structure of 4f (hydrogen atoms are omitted for clarity; displacement ellipsoids are drawn at the 30% probability level). | |
As shown in Fig. 1, dihomooxacalix[4]arene derivative 4f possesses a cone conformation, where the two 2-aminomethyl pyridyl-carbonyl methoxyl substitutes are in the 1,3-alternate position at the lower rim of the macrocyclic ring. This belongs to the monoclinic space group of C2/c. The X-ray structure of 4f also reveals that one of the aromatic rings constructed by C2, C3, C4, C5, C6, and C7 atoms is nearly parallel to the mean plane defined by O2, O3, O5, and O6 phenolic oxygen atoms. The corresponding dihedral angle between the mean plane and this ring is 20.630° (53). The other three aromatic rings, bearing O3, O5, and O6 atoms, have the dihedral angles of 77.448° (66), 54.353° (57), and 53.698° (53), respectively. In addition, in the molecule of 4f, the two pyridine rings exist on the same side of the dihomooxacalix[4]arene and are constrained to be roughly parallel to each other. The N2 and N4 atoms in the pyridine rings are, therefore, located at slightly longer distances of 8.2457 Å (48), which provides further stabilization.
Cytotoxicity assay
To determine the effect of dihomooxacalix[4]arene N-heterocyclic derivatives 4a–4n on cell cytotoxicity, the cell viabilities of these compounds were assessed by the MTT reduction assay. Initially, we investigated the single-concentration inhibition rate after 72 treatments (concentration: 15 μM) against 4 different cell types: MCF-7, HepG2, SKOV3, and HeLa cells. The results are listed in Table 1.
Table 1 Single-concentration inhibition of compounds 4a–4n (concentration: 15 μM)
Compd |
Single concentration inhibition (%) |
MCF-7 |
HepG2 |
SKOV3 |
HeLa |
HUVEC |
4a |
26 |
37 |
35 |
27 |
12 |
4b |
25 |
29 |
52 |
39 |
15 |
4c |
14 |
26 |
35 |
40 |
18 |
4d |
96 |
66 |
69 |
64 |
25 |
4e |
95 |
94 |
83 |
64 |
33 |
4f |
67 |
94 |
54 |
50 |
16 |
4g |
<0 |
30 |
44 |
38 |
34 |
4h |
<0 |
24 |
73 |
38 |
<0 |
4i |
24 |
26 |
39 |
71 |
23 |
4j |
23 |
25 |
50 |
18 |
<0 |
4k |
94 |
91 |
75 |
82 |
27 |
4l |
89 |
16 |
40 |
54 |
43 |
4m |
26 |
22 |
36 |
39 |
24 |
4n |
23 |
26 |
48 |
28 |
31 |
N-Heterocyclic rings, e.g., a quinoline scaffold, have always attracted attention and played important roles in the development of drugs because of their anticancer, antimicrobial, and antimalarial activities.9e,17 Abouzid and coworkers prepared 6-alkoxy-4-substituted-aminoquinazolines and exploited the potent antitumor activity toward MCF-7 cells with IC50 values in the nanomolar range.18 In our design, pyridine, quinoline, and thiazole were introduced in the dihomooxacalix[4]arene platform by using a linker in the form of an acetoethoxy spacer at the lower rim. The cell cytotoxicity results of the synthetic derivatives of 4a–4n (Table 1) showed that nearly all the mono-aminomethyl-pyridine- and quinoline-substituted dihomooxacalix[4]arene derivatives exhibited weak effects on the four tested cancer cells, except 4h toward SKOV3 cells (73%). In contrast, a majority of 1,3-di-N-heterocyclic-substituted dihomooxacalix[4]arene derivatives exhibited effective improvements in cell inhibition. For example, 1,3-di-4-aminomethyl pyridine derivatives 4d showed a significant effect on MCF-7 cells with single-concentration inhibition of 96%. Derivative 4e had worthwhile inhibition rates of 95%, 94%, 83%, and 64% on MCF-7, HepG2, SKOV3, and HeLa cells. Interestingly, the obtained results are similar to those of 1,3-di-3-aminoquinoline-substituted derivative 4k, where the inhibition cancer cells ranged from 75% to 94%. As expected, 4k also exhibited low toxicity toward HUVEC cells (inhibition: 27%). The differences in the inhibition activities of 4a–4n reveal that the numbers, kinds, and positions of the N-heterocyclic groups may have a definite influence on the activity. Further, it was noted that our design of N-heterocyclic compounds was not based on a clear target. Therefore, we could only speculate that such an influence may be attributable to the conjugate effect and steric hindrance of N-heterocyclic rings with an acylamino spacer.
According to the preliminary screening results, the cytotoxic activities (IC50 values) of select compounds with single-concentration inhibition rates in excess of 50% are listed in Table 2.
Table 2 IC50 values (μM) of dihomooxacalix[4]arene-based compounds on cell viabilitya
Compd |
IC50 (μM) |
MCF-7 |
HepG2 |
SKOV3 |
Hela |
“NT” means not detected. |
4d |
1.2 ± 0.2 |
17.6 ± 1.2 |
3.8 ± 0.9 |
3.9 ± 0.6 |
4e |
4.1 ± 0.8 |
8.8 ± 0.8 |
5.8 ± 0.9 |
7.9 ± 1.0 |
4f |
5.4 ± 0.3 |
22.7 ± 3.0 |
NT |
10.9 ± 1.5 |
4h |
NT |
NT |
7.6 ± 0.4 |
NT |
4i |
NT |
NT |
NT |
7.3 ± 0.8 |
4k |
3.7 ± 1.3 |
2.3 ± 0.4 |
3.5 ± 0.8 |
6.0 ± 1.7 |
4l |
2.7 ± 0.3 |
NT |
NT |
NT |
Paclitaxel |
(1.7 ± 0.8) nM |
(1.5 ± 0.2) nM |
(3.8 ± 1.2) nM |
(3.0 ± 1.0) nM |
Overall, they exhibited strong cytotoxic effects (IC50 values ranging from 1.2 ± 0.2 μM to 22.7 ± 3.0 μM) against all the four types of cancer cells. These preliminary results tend to confirm that 1,3-di-N-heterocyclic dihomooxacalix[4] derivatives have efficient antitumor activity, which encouraged us to mechanically perform the molecular studies.
Morphological observation
MCF-7 cells were treated with different concentrations (0, 10, and 20 μM) of dihomooxacalix[4]arene N-heterocyclic derivative 4k for 24 h and then photographed by an inverted optical microscope. The morphological changes are shown in Fig. 2.
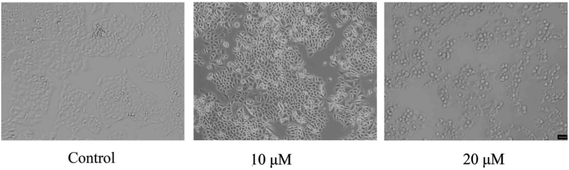 |
| Fig. 2 Cell morphological changes observed under an inverted optical microscope. Scale: 10 μm. | |
Evidently, the cell morphology significantly changed as the drug concentration increased as compared to that of the control group. When the concentration was 20 μM, a large number of cells became spherical and got separated from the surface of the culture dish, indicating that cell proliferation was inhibited.
Western blotting assay
As a pro-apoptotic protein in the Bcl-2 family, Bax overexpression in cells can antagonize the expression of Bcl-2, leading to apoptosis. Therefore, the relative expression levels of Bax and Bcl-2 can be used as the apoptotic signals of cells. In addition, caspase-3 is a vital executioner molecule during the apoptotic process.19 In this work, in order to qualitatively determine whether 4k had an apoptotic effect on MCF-7 cells, the expression levels of various apoptosis-related proteins in MCF-7 cells induced by different concentrations of 4k (0, 10, and 20 μM) were detected by immunoblotting. The results are shown in Fig. 3.
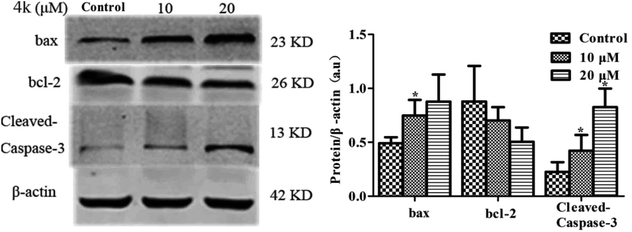 |
| Fig. 3 Effects of 4k on the apoptotic protein expressions in MCF-7 cells. The protein bands of Bax, Bcl-2, cleaved caspase-3, and β-actin in MCF-7 cells were determined by western blotting; β-actin was used as the internal standard for each sample. Relative levels of Bax, Bcl-2, and cleaved caspase-3 in MCF-7 cells performed by densitometric analysis. *P < 0.05 vs. the control group. | |
As shown in Fig. 3, with an increase in the concentration of administration, the expression levels of Bcl-2 and Bax were negatively and positively correlated with the dose, respectively; Bax concentration gradually increased. It should be noted that cleaved caspase-3 usually acted as the key protein for pro-apoptosis and its expression level increased, indicating that 4k could promote the apoptosis of MCF-7 cells.
Immunofluorescence assay
To further elucidate the apoptotic effect of 4k on MCF-7 cells, the expression levels of the related apoptotic proteins were evaluated by immunofluorescence staining. After the treatment of MCF-7 cells with different concentrations of 4k (0, 10, and 20 μM) for 24 h, the Bcl-2 and cleaved caspase-3 antibodies were added at 4 °C overnight, the fluorescent secondary antibody was incubated, the nucleus was stained with DAPI, and the cells were mounted and observed using an inverted fluorescence microscope. The results are shown in Fig. 4.
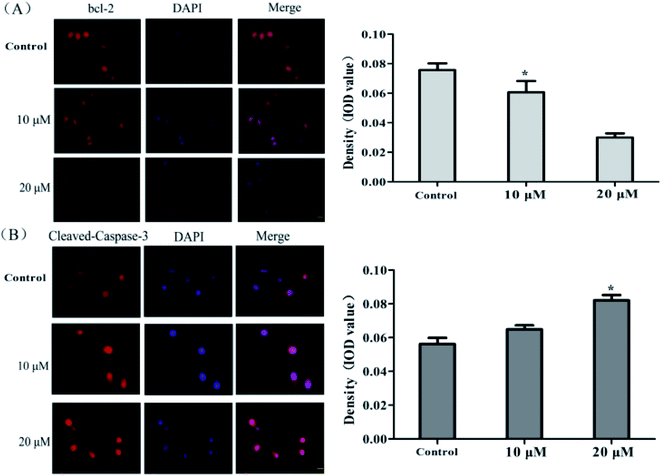 |
| Fig. 4 Effects of 4k on the apoptotic protein expressions in MCF-7 cells. (A) Bcl-2 and (B) cleaved caspase-3 protein antibodies and fluorescein-conjugated second antibody were used to visualize the corresponding protein; DAPI was used to stain the cell nuclei (blue). Scale: 10 μm. *P < 0.05 vs. the control group. | |
From Fig. 4, it is evident that as the drug concentration increases, the fluorescence intensity of Bcl-2 gradually decreases, and the fluorescence intensity of cleaved caspase-3 gradually increases. This result is consistent with the immunoblotting data, further indicating that 4k could promote the apoptosis of MCF-7 cells.
Flow cytometry assay
In order to quantitatively determine the apoptotic rate of MCF-7 cells induced by 4k, flow cytometry was used for the detection; the results are shown in Fig. 5.
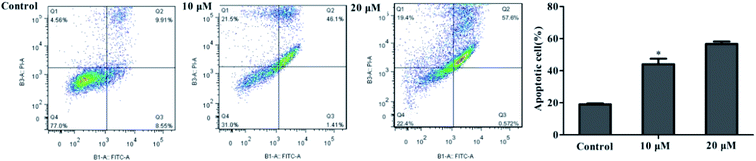 |
| Fig. 5 Effect of 4k on apoptosis in MCF-7 cells. Flow cytometry analyses of MCF-7 cells after Annexin V-FITC/PI staining revealed that 4k exposure resulted in apoptosis in MCF-7 cells in a concentration-dependent manner. *P < 0.05 vs. the control group. | |
It is well known that cells are divided into four subgroups, namely, Q1, Q2, Q3, and Q4, where the proportions of cells in Q2 and Q3 are used to reveal the overall apoptosis of cells. As shown in Fig. 5, the apoptosis rate of the control group was (19.05 ± 0.83)%; however, the treatment of MCF-7 cells for 24 h in the low-concentration group (10 μM) of 4k led to a reduction in cell viability of (46.63 ± 1.25)%. Moreover, a higher value of (57.69 ± 0.68)% was observed at a higher concentration (20 μM) of 4k. Therefore, it is suggested that at these concentrations, 4k could induce cell death in MCF-7 cells.
Conclusions
In this work, we synthesized 14 novel dihomooxacalix[4]arene N-heterocyclic derivatives, namely, 4a–4n. In the subsequent cytotoxicity test, 1,3-disubstituted N-heterocyclic derivatives were found to be more effective than mono-N-heterocyclic-linked derivatives. Moreover, 4d–4f and 4k show significant effects at inducing cytotoxicity on all the four parental types of cancer cells, but exhibit low toxicity toward normal cells. The results of the molecular studies presented in this study revealed that 4k could induce apoptosis, upregulate protein expression levels of Bax and cleaved caspase-3, and downregulate Bcl-2 in human breast cancer cells. Therefore, the dihomooxacalix[4]arene N-heterocyclic derivative is expected to garner a new generation of supramolecular antitumor drugs.
Experimental section
Materials and apparatus
All the reagents and solvents were commercial reagents with analytical grade. Further, p-tert-butyl dihomooxacalix[4]arene 1 was prepared according to the procedures in the literature.20 Furthermore, dihomooxacalix[4]arene ester 2a–2b and carboxylic acid 3a–3b were similarly synthesized as described earlier.15,16,21 The melting points were determined with capillaries with a YRT-3 microscope apparatus and were uncorrected. The 1H NMR and 13C NMR spectra were recorded at 400 MHz on a Bruker AV-400 spectrometer. The HRMS data were obtained using a maXis 4G (UHR-TOF) instrument. The reactions were monitored by thin-layer chromatography (TLC) using 2.5 mm Merck silica gel F254 strips. Distilled water was used in the experiments. Experimental cells were obtained from the Cell Bank, Chinese Academy of Sciences. All the reagents and kits used in the cell experiments are commercially available.
Generic procedure for the synthesis of compounds 4a–4f
Here, 2a (0.23 g, 0.3 mmol) or 2b (0.26 g, 0.3 mmol) was dissolved in aminomethylpyridine (2 mL) and stirred at the ambient temperature. Upon the completion of the reaction (TLC monitoring; typically, 4 h), water was added to the reaction mixture and then filtered, leaving a pale-yellow solid that was later purified by flash chromatography (silica gel/dichloromethane/methanol) to yield the product in a quantitative yield.
7,13,19,25-Tetra-tert-butyl-28,29,30-tri-hydroxy-27-(N-(2-pyridin-4-ylmethyl)-aminocarbonylmethoxyl)-2,3-dihomo-3-oxacalix[4]arene (4a). White solid, yield 90.1%, mp 155.9–157.2 °C; IR (KBr, cm−1) ν 3333, 2961, 2868, 1690, 1603, 1487, 1362, 1209, 1074, 876; 1H NMR (CDCl3, 400 MHz) δ 9.31 (t, 1H, J = 5.6 Hz), 8.86 (s, 1H), 8.53 (dd, 2H, J = 4.4, 1.6 Hz), 8.49 (s, 1H), 7.80 (s, 1H), 7.39 (d, 2H, J = 5.6 Hz), 7.28 (t, 2H, J = 2.4 Hz), 7.16 (d, 1H, J = 2.4 Hz), 7.14–7.12 (m, 2H), 7.09 (d, 1H, J = 2.4 Hz), 6.93 (d, 1H, J = 2.4 Hz), 6.91 (d, 1H, J = 2.4 Hz), 4.97 (d, 1H, J = 15.2 Hz), 4.79 (d, 1H, J = 6.0 Hz), 4.76–4.72 (m, 1H), 4.63–4.50 (m, 3H), 4.36 (d, 1H, J = 10.2 Hz), 4.21 (d, 1H, J = 9.6 Hz), 4.17–4.03 (m, 3H), 3.60 (d, 1H, J = 13.6 Hz), 3.46 (dd, 2H, J = 18.0, 14.0 Hz), 1.26 (s, 9H), 1.23 (d, 18H, J = 1.2 Hz), 1.16 (s, 9H); 13C NMR (CDCl3, 100 MHz) δ 168.2, 151.7, 150.8, 150.1, 149.0, 148.5, 147.2, 146.8, 144.2, 143.0, 142.9, 132.1, 131.6, 128.2, 127.7, 127.3, 127.2, 127.1, 126.8, 126.4, 126.1, 125.8, 125.5, 124.5, 122.9, 122.2, 74.4, 71.7, 71.5, 42.4, 34.3, 34.0, 33.9, 33.8, 32.3, 31.5, 31.4, 31.1, 31.0; MS (m/z): HRMS (ESI) calcd for C53H67N2O6 ([M + H]+): 827.4999, found: 827.5013.
7,13,19,25-Tetra-tert-butyl-28,29,30-tri-hydroxy-27-(N-(2-pyridin-3-ylmethyl)-aminocarbonylmethoxyl)-2,3-dihomo-3-oxacalix[4]arene (4b). White solid, yield 82.9%, mp 154.1–156.9 °C; IR (KBr, cm−1) ν 3331, 2959, 2868, 1686, 1605, 1535, 1487, 1364, 1202, 1076, 712; 1H NMR (CDCl3, 400 MHz) δ 9.24 (t, 1H, J = 5.6 Hz), 8.81 (s, 1H), 8.71 (d, 1H, J = 2.0 Hz), 8.46 (dd, 1H, J = 4.8, 1.6 Hz), 8.33 (s, 1H), 7.86–7.81 (m, 1H), 7.76 (s, 1H), 7.27–7.26 (m, 2H), 7.21 (dd, 1H, J = 8.0, 4.8 Hz), 7.14 (d, 1H, J = 2.4 Hz), 7.11 (s, 2H), 7.08 (d, 1H, J = 2.4 Hz), 6.93 (d, 1H, J = 2.4 Hz), 6.90 (d, 1H, J = 2.4 Hz), 4.96 (d, 1H, J = 14.8 Hz), 4.82 (d, 1H, J = 5.2 Hz), 4.78 (d, 1H, J = 6.2 Hz), 4.62–4.59 (m, 1H), 4.61–4.51 (m, 2H), 4.37 (d, 1H, J = 10.2 Hz), 4.21 (d, 1H, J = 9.6 Hz), 4.13 (d, 1H, J = 9.0 Hz), 4.09 (d, 1H, J = 9.6 Hz), 4.02 (d, 1H, J = 13.6 Hz), 3.59 (d, 1H, J = 13.6 Hz), 3.44 (d, 1H, J = 14.0 Hz), 3.38 (d, 1H, J = 13.2 Hz), 1.26 (s, 9H), 1.22 (d, 18H, J = 2.0 Hz), 1.15 (s, 9H); 13C NMR (CDCl3, 100 MHz) δ 168.0, 151.7, 150.9, 149.8, 149.0, 148.9, 148.5, 147.2, 144.2, 142.9, 142.7, 136.0, 133.5, 132.1, 131.6, 128.2, 127.7, 127.4, 127.2, 127.1, 126.8, 126.4, 126.1, 125.7, 125.6, 125.5, 124.4, 123.6, 122.9, 122.2, 74.3, 71.7, 71.5, 41.0, 34.3, 34.0, 33.9, 32.3, 31.6, 31.5, 31.4, 31.1, 30.9; MS (m/z): HRMS (ESI) calcd for C53H67N2O6 ([M + H]+): 827.4999, found: 827.5012.
7,13,19,25-Tetra-tert-butyl-28,29,30-tri-hydroxy-27-(N-(2-pyridin-2-ylmethyl)-aminocarbonylmethoxyl)-2,3-dihomo-3-oxacalix[4]arene (4c). White solid, yield 89.2%, mp 163.7–165.4 °C; IR (KBr, cm−1) ν 3346, 2961, 2868, 1682, 1593, 1487, 1362, 1209, 1076, 874; 1H NMR (CDCl3, 400 MHz) δ 9.30 (t, 1H, J = 5.6 Hz), 8.84 (s, 1H), 8.55 (d, 1H, J = 4.0 Hz), 8.46 (s, 1H), 7.70 (s, 1H), 7.65–7.61 (m, 1H), 7.47 (d, 1H, J = 7.8 Hz), 7.30–7.24 (m, 2H), 7.16–7.10 (m, 4H), 7.08 (d, 1H, J = 2.4 Hz), 6.93 (d, 1H, J = 2.4 Hz), 6.89 (d, 1H, J = 2.4 Hz), 4.96 (dd, 2H, J = 15.2, 5.6 Hz), 4.82–4.69 (m, 2H), 4.77–4.71 (m, 2H), 4.34 (d, 1H, J = 10.0 Hz), 4.25 (s, 1H), 4.21 (d, 1H, J = 9.0 Hz), 4.17–4.06 (m, 2H), 3.59 (d, 1H, J = 13.8 Hz), 3.43 (t, 2H, J = 13.8 Hz), 1.25 (s, 9H), 1.23 (brs, 18H), 1.16 (s, 9H); 13C NMR (CDCl3, 100 MHz) δ 168.2, 157.5, 151.9, 151.0, 149.4, 148.8, 148.7, 147.4, 144.0, 142.7, 142.6, 136.7, 132.0, 131.8, 128.1, 127.7, 127.5, 127.2, 127.1, 126.7, 126.6, 126.0, 125.8, 125.5, 125.4, 124.4, 122.7, 122.3, 122.1, 121.5, 74.6, 71.8, 71.4, 45.0, 34.3, 34.0, 33.9, 33.8, 32.3, 31.6, 31.5, 31.4, 31.3, 31.1, 31.0; MS (m/z): HRMS (ESI) calcd for C53H67N2O6 ([M + H]+): 827.4999, found: 827.5009.
7,13,19,25-Tetra-tert-butyl-28,30-di-hydroxy-27,29-di-(N-(2-pyridin-4-ylmethyl)-aminocarbonylmethoxyl)-2,3-dihomo-3-oxacalix[4]arene (4d). White solid, yield 93.2%, mp 129.7–130.1 °C; IR (KBr, cm−1) ν 3369, 2969, 2868, 1678, 1537, 1483, 1362, 1192, 1070, 876, 714; 1H NMR (CDCl3, 400 MHz) δ 9.55 (dd, 1H, J = 6.4, 5.6 Hz), 9.18 (t, 1H, J = 5.2 Hz), 8.50 (d, 2H, J = 5.6 Hz), 8.33 (d, 2H, J = 5.2 Hz), 8.00 (s, 1H), 7.44 (d, 1H, J = 1.6 Hz), 7.34–7.32 (m, 3H), 7.23 (d, 3H, J = 3.2 Hz), 7.17 (d, 2H, J = 8.4 Hz), 7.13 (s, 1H), 7.01 (d, 2H, J = 8.4 Hz), 6.85 (d, 1H, J = 1.2 Hz), 4.92–4.85 (m, 2H), 4.81 (d, 1H, J = 10.0 Hz), 4.75 (d, 1H, J = 10.0 Hz), 4.69 (d, 1H, J = 15.2 Hz), 4.56 (d, 1H, J = 15.2 Hz), 4.46–4.36 (m, 3H), 4.26 (s, 2H), 4.18 (d, 1H, J = 10.4 Hz), 4.12 (t, 2H, J = 14.4 Hz), 3.94 (d, 1H, J = 13.6 Hz), 3.53 (d, 1H, J = 13.6 Hz), 3.41 (dd, 2H, J = 13.6, 6.4 Hz), 1.28 (d, 18H, J = 3.2 Hz), 1.24 (s, 9H), 1.16 (s, 9H); 13C NMR (CDCl3, 100 MHz) δ 31.0, 31.1, 31.2, 31.5, 32.3, 33.9, 34.2, 34.3, 42.3, 42.6, 71.2, 72.1, 73.5, 74.3, 122.2, 123.0, 124.5, 125.7, 126.0, 126.1, 126.8, 127.1, 127.2, 127.6, 128.6, 129.5, 131.4, 132.2, 133.6, 143.2, 143.8, 146.1, 146.8, 148.3, 148.8, 149.2, 149.9, 150.0, 150.9, 152.8, 168.4, 168.9; MS (m/z): HRMS (ESI) calcd for C61H74N4NaO7 ([M + Na]+): 997.5458, found: 997.5475.
7,13,19,25-Tetra-tert-butyl-28,30-di-hydroxy-27,29-di-(N-(2-pyridin-3-ylmethyl)-aminocarbonylmethoxyl)-2,3-dihomo-3-oxacalix[4]arene (4e). White solid, yield 94.5%, mp 130.5–131.7 °C; IR (KBr, cm−1) ν 3377, 2961, 2868, 1678, 1603, 1541, 1485, 1417, 1362, 1202, 1070, 876; 1H NMR (CDCl3, 400 MHz) δ 9.43 (t, 1H, J = 5.6 Hz), 9.14 (dd, 1H, J = 5.6, 4.8 Hz), 8.70 (d, 1H, J = 2.0 Hz), 8.63 (d, 1H, J = 2.0 Hz), 8.49 (dd, 1H, J = 4.8, 1.6 Hz), 8.37 (dd, 1H, J = 4.8, 1.6 Hz), 7.88 (s, 1H), 7.75 (dt, 1H, J = 8.0, 2.0 Hz), 7.60 (dt, 1H, J = 8.0, 2.0 Hz), 7.42 (d, 1H, J = 2.4 Hz), 7.31 (s, 1H), 7.17 (dd, 2H, J = 7.6, 5.2 Hz), 7.14 (dd, 2H, J = 4.4, 2.4 Hz), 7.11 (d, 1H, J = 2.4 Hz), 7.03 (d, 1H, J = 2.8 Hz), 6.95 (d, 1H, J = 2.8 Hz), 6.94 (d, 1H, J = 4.8 Hz), 6.84 (d, 1H, J = 2.4 Hz), 4.89–4.86 (m, 1H), 4.82 (d, 1H, J = 5.2 Hz), 4.79 (d, 1H, J = 5.2 Hz), 4.77 (d, 1H, J = 4.4 Hz), 4.72 (d, 1H, J = 15.2 Hz), 4.56 (d, 1H, J = 15.2 Hz), 4.49–4.40 (m, 4H), 4.28 (d, 1H, J = 10.0 Hz), 4.20 (d, 1H, J = 10.4 Hz), 4.10 (d, 1H, J = 13.2 Hz), 4.03 (d, 1H, J = 13.2 Hz), 3.97 (d, 1H, J = 13.2 Hz), 3.49 (d, 1H, J = 13.6 Hz), 3.38 (dd, 2H, J = 13.2, 3.6 Hz), 1.27 (d, 18H, J = 2.8 Hz), 1.24 (s, 9H), 1.15 (s, 9H); 13C NMR (CDCl3, 100 MHz) δ 31.1, 31.2, 31.4, 31.5, 32.2, 33.8, 33.9, 34.2, 34.3, 40.9, 41.0, 71.1, 72.2, 73.7, 122.2, 123.3, 123.4, 124.5, 125.8, 126.2, 126.7, 126.8, 127.0, 127.1, 127.2, 127.6, 128.6, 129.4, 131.4, 132.2, 133.2, 133.6, 133.7, 135.8, 136.0, 143.0, 143.6, 148.2, 148.3, 148.6, 148.7, 148.9, 149.5, 149.6, 149.7, 151.0, 152.8, 168.2, 168.7; MS (m/z): HRMS (ESI) calcd for C61H74N4NaO7 ([M + Na]+): 997.5558, found: 997.5474.
7,13,19,25-Tetra-tert-butyl-28,30-di-hydroxy-27,29-di-(N-(2-pyridin-2-ylmethyl)-aminocarbonylmethoxyl)-2,3-dihomo-3-oxacalix[4]arene (4f). White solid, yield 91.8%, mp 131.3–132.2 °C; IR (KBr, cm−1) ν 3381, 2961, 2868, 1678, 1535, 1483, 1362, 1192, 1070, 874, 754; 1H NMR (CDCl3, 400 MHz) δ 9.22 (t, 1H, J = 5.6 Hz), 8.97 (t, 1H, J = 5.6 Hz), 8.38 (d, 1H, J = 4.4 Hz), 8.21 (d, 1H, J = 4.4 Hz), 7.80 (s, 1H), 7.55–7.47 (m, 2H), 7.40 (d, 1H, J = 2.4 Hz), 7.38 (d, 1H, J = 8.0 Hz), 7.31 (d, 1H, J = 8.0 Hz), 7.17 (s, 2H), 7.13 (d, 1H, J = 2.0 Hz), 7.06 (t, 2H, J = 2.0 Hz), 7.04–7.01 (m, 1H), 7.00 (d, 1H, J = 2.4 Hz), 6.96 (dd, 1H, J = 6.8, 5.2 Hz), 6.89 (d, 1H, J = 2.0 Hz), 6.81 (d, 1H, J = 2.4 Hz), 4.89–4.81 (m, 3H), 4.69–4.62 (m, 4H), 4.59–4.50 (m, 4H), 4.28 (dd, 2H, J = 14.8, 10.4 Hz), 4.20 (d, 1H, J = 5.6 Hz), 4.17 (d, 1H, J = 9.2 Hz), 4.10 (d, 1H, J = 13.2 Hz), 3.44 (d, 1H, J = 13.6 Hz), 3.35 (t, 2H, J = 14.4 Hz), 1.25 (d, 18H, J = 2.0 Hz), 1.22 (s, 9H), 1.09 (s, 9H); 13C NMR (CDCl3, 100 MHz) δ 31.0, 31.2, 31.3, 31.4, 31.5, 31.6, 32.2, 33.8, 33.9, 34.2, 44.8, 45.0, 71.1, 72.4, 73.7, 74.5, 121.9, 122.0, 122.1, 122.3, 124.2, 125.5, 125.6, 125.9, 126.5, 126.8, 126.9, 127.2, 127.6, 127.7, 128.8, 129.4, 131.9, 132.0, 133.9, 136.3, 136.4, 142.3, 143.0, 147.8, 148.1, 149.0, 149.1, 149.2, 150.0, 151.7, 153.1, 157.0, 157.6, 168.4, 168.9; MS (m/z): HRMS (ESI) calcd for C61H74N4NaO7 ([M + Na]+): 997.5558, found: 997.5470.
Generic procedure for the synthesis of compound 4g–4n
A mixture of carboxylic acid 3a or 3b (0.22 g or 0.25 g, 0.3 mmol) and oxalyl chloride (0.75 g, 5.9 mmol) was dissolved in dry dichloromethane (2 mL) and stirred at room temperature. After 5 h, the mixture was evaporated and washed with dichloromethane 3 times to afford the solid. To a solution of the above solid in dry dichloromethane (4 mL), 1.2 mmol of amino-substituted pyridine (or quinoline or thiazole) was slowly added dropwise in 2 mL of THF at 0 °C. The reaction was completed after 6–12 h. The evaporation of the solvent yielded the crude products, which were subsequently subjected to flash chromatography to afford 4g–4n.
7,13,19,25-Tetra-tert-butyl-28,29,30-tri-hydroxy-27-(N-(6-quinolyl)-aminocarbonylmethoxyl)-2,3-dihomo-3-oxacalix[4]arene (4g). White solid, yield 92.4%, mp 179.2–180.6 °C; IR (KBr) ν 3367, 2961, 2868, 1701, 1545, 1487, 1383, 1364, 1209, 875; 1H NMR (CDCl3, 400 MHz) δ 10.65 (s, 1H), 8.99 (s, 1H), 8.86–8.81 (m, 2H), 8.61 (d, 1H, J = 2.2 Hz), 8.29 (s, 1H), 8.25 (dd, 1H, J = 2.4 Hz), 8.20–8.15 (m, 1H), 8.10 (d, 1H, J = 9.2 Hz), 7.38 (dd, 2H, J = 8.4, 4.2 Hz), 7.31 (d, 1H, J = 2.4 Hz), 7.29 (d, 1H, J = 2.4 Hz), 7.19 (d, 1H, J = 2.4 Hz), 7.16 (s, 2H), 7.12 (d, 1H, J = 2.4 Hz), 7.01 (d, 1H, J = 2.4 Hz), 6.93 (d, 1H, J = 2.4 Hz), 5.08 (d, 1H, J = 5.4 Hz), 5.05 (s, 1H), 4.77 (d, 1H, J = 10.2 Hz), 4.68 (d, 1H, J = 15.2 Hz), 4.51 (d, 1H, J = 10.2 Hz), 4.37 (d, 1H, J = 9.6 Hz), 4.25 (d, 1H, J = 1.5 Hz), 4.21 (d, 1H, J = 2.8 Hz), 4.11 (d, 1H, J = 13.8 Hz), 3.66 (d, 1H, J = 13.8 Hz), 3.50 (d, 1H, J = 7.2 Hz), 3.46 (d, 1H, J = 6.4 Hz), 1.24 (d, 27H, J = 1.0 Hz), 1.17 (s, 9H); 13C NMR (CDCl3, 100 MHz) δ 166.5, 151.9, 151.1, 151.0, 149.5, 149.2, 148.6, 147.3, 145.8, 144.5, 144.4, 143.1, 143.0, 136.2, 135.9, 135.8, 132.1, 131.6, 130.0, 128.8, 128.5, 127.7, 127.5, 127.4, 127.3, 126.9, 126.5, 126.3, 125.9, 125.8, 125.6, 124.4, 124.2, 122.8, 122.3, 121.6, 117.2, 74.6, 72.1, 71.9, 34.4, 34.1, 34.0, 32.5, 31.6, 31.5, 31.2; MS (m/z): HRMS (ESI) calcd for C56H67N2O6 ([M + H]+): 863.4999, found: 863.4991.
7,13,19,25-Tetra-tert-butyl-28,29,30-tri-hydroxy-27-(N-(3-quinolyl)-aminocarbonylmethoxyl)-2,3-dihomo-3-oxacalix[4]arene (4h). White solid, yield 93.9%, mp 188.7–189.2 °C; IR (KBr, cm−1) ν 3331, 2961, 2905, 2868, 1697, 1545, 1487, 1382, 1209, 876; 1H NMR (CDCl3, 400 MHz) δ 10.77 (s, 1H), 9.37 (d, 1H, J = 2.4 Hz), 8.98 (d, 2H, J = 2.4 Hz), 8.77 (s, 1H), 8.28 (s, 1H), 8.08 (dd, 1H, J = 8.4, 0.8 Hz), 7.85 (dd, 1H, J = 8.2, 1.2 Hz), 7.66–7.61 (m, 1H), 7.56–7.51 (m, 1H), 7.29 (s, 1H), 7.28 (s, 1H), 7.19 (d, 1H, J = 2.4 Hz), 7.15 (d, 2H, J = 2.4 Hz), 7.12 (d, 1H, J = 2.4 Hz), 6.99 (d, 1H, J = 2.4 Hz), 6.93 (d, 1H, J = 2.4 Hz), 5.11 (d, 1H, J = 3.0 Hz), 5.08 (d, 1H, J = 8.8 Hz), 4.85 (d, 1H, J = 10.2 Hz), 4.71 (d, 1H, J = 15.2 Hz), 4.49 (d, 1H, J = 10.4 Hz), 4.34 (d, 1H, J = 9.6 Hz), 4.24 (d, 1H, J = 7.2 Hz), 4.21 (d, 1H, J = 8.2 Hz), 4.11 (d, 1H, J = 13.6 Hz), 3.65 (d, 1H, J = 14.0 Hz), 3.49 (d, 1H, J = 4.0 Hz), 3.46 (d, 1H, J = 4.8 Hz), 1.24, 1.17 (2s, 36H); 13C NMR (CDCl3, 100 MHz) δ 167.0, 151.8, 151.0, 149.3, 148.6, 147.3, 145.6, 145.0, 144.5, 143.3, 143.0, 132.2, 131.7, 131.6, 129.2, 128.5, 128.4, 128.3, 128.0, 127.7, 127.5, 127.4, 127.2, 127.0, 126.5, 126.3, 126.0, 125.8, 125.6, 124.7, 124.6, 123.1, 122.4, 74.6, 72.1, 71.9, 34.4, 34.1, 34.0, 32.5, 31.7, 31.6, 31.5, 31.4, 31.2; MS (m/z): HRMS (ESI) calcd for C56H67N2O6 ([M + H]+): 863.4999, found: 863.5004.
7,13,19,25-Tetra-tert-butyl-28,30-di-hydroxy-27,29-di-(N-(8-quinolyl)-aminocarbonylmethoxyl)-2,3-dihomo-3-oxacalix[4]arene (4i). White solid, yield 85.4%, mp 157.3–158.5 °C; IR (KBr, cm−1) ν 3435, 2960, 2906, 1686, 1535, 1485, 1194, 874; 1H NMR (CDCl3, 400 MHz) δ 10.74 (s, 2H), 8.54 (d, 1H, J = 3.0 Hz), 8.49–8.41 (m, 3H), 7.32–7.26 (m, 6H), 7.17 (s, 2H), 7.09 (s, 2H), 7.00 (d, 2H, J = 2.4 Hz), 6.90 (d, 1H, J = 1.2 Hz), 6.85 (d, 1H, J = 2.4 Hz), 6.75 (d, 1H, J = 1.2 Hz), 5.02 (d, 1H, J = 10.2 Hz), 4.80 (s, 2H), 4.69 (s, 2H), 4.60–4.33 (m, 5H), 4.29 (d, 1H, J = 10.6 Hz), 3.55 (d, 2H, J = 15.0 Hz), 3.46 (d, 1H, J = 15.0 Hz), 1.30, 1.25, 1.09, 0.95 (4s, 36H); 13C NMR (CDCl3, 100 MHz) δ 166.5, 152.7, 152.5, 150.4, 150.3, 148.6, 147.6, 147.3, 142.3, 141.4, 134.9, 133.6, 133.4, 133.1, 130.9, 128.7, 127.9, 127.6, 127.4, 127.3, 126.5, 126.4, 126.3, 126.0, 125.9, 125.3, 123.9, 122.8, 122.1, 121.5, 121.3, 116.8, 74.3, 73.8, 34.2, 34.1, 34.0, 33.9, 31.8, 31.7, 31.3, 31.2; MS (m/z): HRMS (ESI) calcd for C67H75N4O7 ([M + H]+): 1047.5636, found: 1047.5656.
7,13,19,25-Tetra-tert-butyl-28,30-di-hydroxy-27,29-di-(N-(5-quinolyl)-aminocarbonylmethoxyl)-2,3-dihomo-3-oxacalix[4]arene (4j). White solid, yield 82.6%, mp 167.9–169.5 °C; IR (KBr, cm−1) ν 3404, 2969, 2868, 1680, 1535, 1485, 1363, 1199, 801; 1H NMR (CDCl3, 400 MHz) δ 10.10 (s, 1H), 9.40 (s, 1H), 8.82 (dd, 1H, J = 4.2, 1.6 Hz), 8.69 (dd, 1H, J = 4.2, 1.6 Hz), 8.22 (d, 1H, J = 8.4 Hz), 8.12 (d, 1H, J = 8.4 Hz), 7.90–7.74 (m, 3H), 7.58–7.51 (m, 2H), 7.45 (dd, 2H, J = 10.0, 5.2 Hz), 7.40 (s, 1H), 7.32 (d, 1H, J = 2.4 Hz), 7.30–7.27 (m, 1H), 7.20 (d, 1H, J = 2.4 Hz), 7.15 (d, 1H, J = 2.4 Hz), 7.06 (dt, 3H, J = 8.6, 4.2 Hz), 6.89 (d, 1H, J = 2.4 Hz), 6.85 (d, 1H, J = 2.4 Hz), 6.82 (dd, 1H, J = 8.4, 4.2 Hz), 5.03 (d, 1H, J = 10.8 Hz), 4.69 (d, 1H, J = 15.4 Hz), 4.58 (d, 1H, J = 15.4 Hz), 4.53–4.42 (m, 4H), 4.35 (d, 1H, J = 15.4 Hz), 4.25 (d, 1H, J = 11.0 Hz), 4.20 (d, 1H, J = 3.6 Hz), 4.17 (d, 1H, J = 3.2 Hz), 3.56 (t, 2H, J = 13.6 Hz), 3.37 (d, 1H, J = 13.8 Hz), 1.31, 1.27, 1.24, 1.06 (4s, 36H); 13C NMR (CDCl3, 100 MHz) δ 167.4, 166.5, 152.5, 151.5, 150.2, 150.1, 149.1, 148.6, 148.3, 148.2, 142.8, 132.4, 131.3, 131.0, 129.8, 129.7, 129.3, 128.8, 128.0, 127.8, 127.6, 127.5, 127.3, 127.1, 126.8, 126.3, 126.0, 125.9, 124.1, 122.1, 120.7, 120.5, 120.3, 74.2, 73.9, 72.0, 71.1, 34.3, 34.2, 34.0, 33.9, 32.8, 31.6, 31.5, 31.2, 31.0, 30.8; MS (m/z): HRMS (ESI) calcd for C67H75N4O7 ([M + H]+): 1047.5636, found: 1047.5640.
7,13,19,25-Tetra-tert-butyl-28,30-di-hydroxy-27,29-di-(N-(3-quinolyl)-aminocarbonylmethoxyl)-2,3-dihomo-3-oxacalix[4]arene (4k). White solid, yield 88.7%, mp 156.4–157.9 °C; IR (KBr, cm−1) ν 3400, 2961, 2868, 1699, 1545, 1485, 1364, 1194, 876; 1H NMR (CDCl3, 400 MHz) δ 10.68 (s, 1H), 10.49 (s, 1H), 9.10 (d, 1H, J = 2.6 Hz), 8.93 (d, 1H, J = 2.6 Hz), 8.58 (s, 1H), 8.19–8.17 (m, 1H), 8.11 (dd, 1H, J = 8.4, 0.8 Hz), 8.09–8.05 (m, 3H), 7.69–7.63 (m, 2H), 7.56 (d, 1H, J = 1.0 Hz), 7.55 (d, 1H, J = 0.8 Hz), 7.52 (dd, 2H, J = 7.6, 1.6 Hz), 7.48 (dd, 1H, J = 8.2, 1.4 Hz), 7.30 (d, 1H, J = 2.4 Hz), 7.21 (t, 2H, J = 2.4 Hz), 7.19 (d, 1H, J = 2.4 Hz), 7.09 (d, 1H, J = 2.4 Hz), 7.06 (d, 1H, J = 2.4 Hz), 6.87 (d, 1H, J = 2.4 Hz), 5.29 (d, 1H, J = 10.8 Hz), 5.03 (d, 1H, J = 15.2 Hz), 4.92 (d, 1H, J = 15.2 Hz), 4.80 (d, 1H, J = 10.6 Hz), 4.60 (d, 1H, J = 10.6 Hz), 4.46 (d, 1H, J = 12.8 Hz), 4.38 (s, 1H), 4.34 (d, 1H, J = 8.4 Hz), 4.29 (d, 1H, J = 1.6 Hz), 4.25 (d, 1H, J = 8.6 Hz), 4.20 (d, 1H, J = 4.8 Hz), 4.17 (d, 1H, J = 2.8 Hz), 3.74 (d, 1H, J = 14.0 Hz), 3.47 (d, 1H, J = 12.8 Hz), 3.42 (d, 1H, J = 13.2 Hz), 1.30, 1.26, 1.22, 1.18 (4s, 36H); 13C NMR (CDCl3, 100 MHz) δ 167.0, 165.8, 153.1, 151.3, 149.2, 149.0, 148.9, 148.1, 145.5, 143.5, 143.4, 143.2, 133.6, 132.2, 131.7, 131.3, 130.0, 128.4, 128.1, 127.9, 127.6, 127.5, 127.1, 126.5, 124.5, 123.8, 123.6, 122.3, 74.2, 73.8, 71.5, 34.5, 31.7, 31.6, 31.4, 31.3; MS (m/z): HRMS (ESI) calcd for C67H75N4O7 ([M + H]+): 1047.5636, found: 1047.5641.
7,13,19,25-Tetra-tert-butyl-28,30-di-hydroxy-27,29-di-(N-(2-thiazolyl)-aminocarbonylmethoxyl)-2,3-dihomo-3-oxacalix[4]arene (4l). White solid, yield 93.2%, mp 163.5–165.1 °C; IR (KBr, cm−1) ν 3367, 3325, 2980, 2871, 1598, 1535, 1481, 1333, 1193, 875; 1H NMR (CDCl3, 400 MHz) δ 12.30 (s, 1H), 12.00 (s, 1H), 8.81 (s, 1H), 8.17 (s, 1H), 7.50 (d, 1H, J = 2.4 Hz), 7.44 (d, 1H, J = 3.5 Hz), 7.42 (d, 1H, J = 3.5 Hz), 7.24–7.21 (m, 2H), 7.20 (d, 1H, J = 2.4 Hz), 7.15 (d, 1H, J = 2.3 Hz), 7.09 (dd, 2H, J = 4.4, 2.4 Hz), 6.86 (q, 3H, J = 3.2 Hz), 5.20 (d, 1H, J = 15.6 Hz), 5.14 (s, 1H), 5.11 (d, 1H, J = 4.4 Hz), 4.72 (d, 1H, J = 10.0 Hz), 4.56 (d, 1H, J = 15.4 Hz), 4.46 (d, 1H, J = 5.4 Hz), 4.43 (d, 1H, J = 10.8 Hz), 4.37 (d, 1H, J = 13.0 Hz), 4.31 (d, 1H, J = 10.4 Hz), 4.26 (d, 1H, J = 13.8 Hz), 4.17 (d, 1H, J = 13.1 Hz), 3.65 (d, 1H, J = 13.8 Hz), 3.45 (d, 1H, J = 8.8 Hz), 3.42 (d, 1H, J = 8.8 Hz), 1.27 (d, 18H, J = 1.6 Hz), 1.23, 1.19 (2s, 18H); 13C NMR (CDCl3, 100 MHz) δ 166.8, 166.3, 157.1, 156.7, 153.1, 151.9, 149.5, 149.3, 148.5, 147.9, 143.1, 142.6, 138.1, 137.9, 133.8, 132.3, 131.6, 129.7, 129.2, 127.4, 127.3, 127.2, 126.5, 126.0, 125.9, 125.5, 124.0, 122.2, 113.4, 113.3, 74.0, 73.3, 72.1, 71.2, 34.5, 34.3, 33.9, 32.8, 31.8, 31.6, 31.2, 31.1; MS (m/z): HRMS (ESI) calcd for C55H66N4NaO7S2 ([M + Na]+): 981.4271, found: 981.4281.
7,13,19,25-Tetra-tert-butyl-28,30-di-hydroxy-27,29-di-(N-(4-pyridyl)-aminocarbonylmethoxyl)-2,3-dihomo-3-oxacalix[4]arene (4m). White solid, yield 89.2%, mp 166.8–168.5 °C; IR (KBr, cm−1) ν 3392, 3378, 2980, 2867, 1699, 1591, 1483, 1207, 1193, 825; 1H NMR (CDCl3, 400 MHz) δ 10.54 (s, 1H), 10.31 (s, 1H), 8.51 (s, 1H), 8.48 (t, 4H, J = 5.6 Hz), 7.92 (s, 1H), 7.50 (t, 3H, J = 2.4 Hz), 7.44 (d, 2H, J = 6.4 Hz), 7.32 (d, 1H, J = 2.4 Hz), 7.24 (d, 1H, J = 2.4 Hz), 7.21 (dd, 2H, J = 4.0, 2.4 Hz), 7.09 (t, 2H, J = 2.4 Hz), 6.88 (d, 1H, J = 2.4 Hz), 5.14 (s, 1H), 4.91 (d, 1H, J = 15.6 Hz), 4.81 (d, 1H, J = 15.2 Hz), 4.64 (d, 1H, J = 10.4 Hz), 4.58 (d, 1H, J = 10.8 Hz), 4.39 (s, 1H), 4.35 (d, 1H, J = 3.6 Hz), 4.29 (d, 1H, J = 11.2 Hz), 4.20 (d, 1H, J = 15.2 Hz), 4.14 (d, 1H, J = 14.0 Hz), 4.09 (d, 1H, J = 13.2 Hz), 3.75 (d, 1H, J = 13.6 Hz), 3.47 (d, 1H, J = 12.8 Hz), 3.42 (d, 1H, J = 13.2 Hz), 1.30, 1.27, 1.24, 1.19 (4s, 36H); 13C NMR (CDCl3, 100 MHz) δ 167.2, 166.1, 153.0, 151.1, 150.6, 149.3, 149.0, 148.8, 148.3, 144.6, 144.4, 144.3, 143.6, 133.5, 132.1, 131.6, 128.6, 127.6, 127.7, 127.6, 127.5, 126.4, 126.0, 125.9, 122.0, 113.8, 113.5, 74.1, 73.7, 72.1, 71.5, 34.5, 34.4, 34.2, 34.1, 31.7, 31.6, 31.4, 31.2; MS (m/z): HRMS (ESI) calcd for C59H71N4O7 ([M + H]+): 947.5323, found: 947.5342.
7,13,19,25-Tetra-tert-butyl-28,30-di-hydroxy-27,29-di-(N-(2-pyridyl)-aminocarbonylmethoxyl)-2,3-dihomo-3-oxacalix[4]arene (4n). White solid, yield 93.6%, mp 160.5–162.2 °C; IR (KBr, cm−1) ν 3367, 3325, 2980, 2871, 1598, 1535, 1481, 1333, 1193, 875; 1H NMR (CDCl3, 400 MHz) δ 11.02 (s, 1H), 10.96 (s, 1H), 8.61 (s, 1H), 8.33 (s, 2H), 8.21 (s, 1H), 7.77 (d, 1H, J = 8.2 Hz), 7.67 (d, 1H, J = 8.2 Hz), 7.48 (q, 2H, J = 9.4 Hz), 7.22 (d, 2H, J = 5.6 Hz), 7.19 (s, 1H), 7.15 (s, 1H), 7.08 (s, 2H), 7.03–6.94 (m, 2H), 6.83 (s, 1H), 5.15 (d, 1H, J = 10.6 Hz), 4.96 (d, 1H, J = 15.4 Hz), 4.85 (d, 1H, J = 15.2 Hz), 4.57 (d, 1H, J = 10.2 Hz), 4.50–4.42 (m, 2H), 4.42–4.31 (m, 2H), 4.31–4.27 (m, 2H), 4.24 (d, 1H, J = 6.4 Hz), 3.63 (d, 1H, J = 13.8 Hz), 3.41 (d, 2H, J = 13.0 Hz), 1.27, 1.26, 1.22, 1.19 (4s, 36H); 13C NMR (CDCl3, 100 MHz) δ 167.5, 166.9, 152.2, 151.2, 149.9, 149.8, 148.1, 147.8, 147.7, 147.5, 137.5, 133.8, 132.3, 131.8, 129.7, 129.0, 127.5, 127.1, 126.7, 125.9, 123.7, 122.1, 119.7, 114.4, 113.7, 74.5, 71.7, 34.3, 34.2, 33.9, 33.8, 32.7, 31.6, 31.5, 31.3, 31.2; MS (m/z): HRMS (ESI) calcd for C59H71N4O7 ([M + H]+): 947.5323, found: 947.5310.
Crystallography
The single crystal of compound 4f was formed in ethanol. The single-crystal structure was determined on a Bruker Smart Apex single-crystal diffractometer. All the nonhydrogen atoms were refined with anisotropic displacement parameters. The structure was solved by direct methods (SHELX-86) followed by Fourier-difference synthesis and refined by full-matrix least squares on F2 with SHELXS-97.22
Cell culture
The MCF-7, HeLa, HepG2, SKOV3, and HUVEC cell lines used in this study were kindly provided by the Cell Bank of the Chinese Academy of Sciences. All the cells were cultured at 37 °C in 5% CO2. The MCF-7 cell lines were cultured in a flask with RPMI 1640 medium containing 2 mM L-glutamine, 1.5 g L−1 sodium bicarbonate, 4.5 g L−1 glucose, 10 mM HEPES, 1.0 mM sodium pyruvate, and 10% fetal bovine serum. Other human cell lines were also cultured using 10% fetal bovine serum and 1% penicillin/streptomycin in RPMI 1640 as described earlier.8a
Cytotoxicity by MTT assay
The cells were cultured in a logarithmic growth phase in a culture medium containing 10% fetal bovine serum and seeded in a 96-well culture plate containing 200 μL per well. The cells were preincubated for 24 h at 37 °C in a 5% CO2 incubator. The cells were treated with different concentrations (0, 1.25, 2.5, 5, 10, and 20 μM) of dihomooxacalix[4]arene N-heterocyclic derivatives dissolved in DMSO for 72 h. The cell viability was analyzed by the MTT reduction assay as described below. Further, 20 μL of the MTT solution (concentration: 5 mg mL−1) was added to each well, and the cells were further cultured for 4 h in a 5% CO2 incubator for the color reaction. Then, the medium was carefully discarded from each well; thereafter, 100 μL DMSO was added to each well, shaken well in the dark, and dissolved in the oven for 30 min such that the crystals were fully dissolved and evenly mixed. The OD value of each well was measured by a microplate reader at a wavelength of 550 nm. The inhibition rate of cell proliferation was determined according to the following formula:
Inhibition rate (%) = (control group OD value − medication group OD value)/control group OD value × 100% |
The compound was then processed using GraphPad Prism 5 software to calculate the IC50 value of the compound.
Western blotting assay
MCF-7 cells were extracted with a cell lysis buffer. The total protein concentration was measured by the BCA method and then adjusted so that the different samples had equal concentrations. Protein was separated on a 12% sodium dodecyl sulfate-polyacrylamide gel and transferred to a polyvinylidene fluoride (PVDF) membrane. The PVDF membrane was incubated with the primary antibody overnight at 4 °C and then incubated with the secondary antibody for 2 h. The target band was scanned with Odyssey, and the results were scanned by a gel imaging system. Gray value analysis was performed using the ImageJ software.
Immunofluorescence assay
Dihomooxacalix[4]arene N-heterocyclic derivative 4k was applied to the cells for drug-induced induction at concentrations of 0, 10, and 20 μM. After 24 h of administration, the cells were fixed with 4% paraformaldehyde (diluted in PBS) for 15 min at room temperature. Subsequently, 0.5% Triton X-100 (diluted with PBS) was added for 30 min. An additional 5% BSA blocking solution was added and blocked at room temperature for 30 min. Then, the designated primary antibody was incubated overnight at 4 °C and then incubated with the second antibody at 37 °C for 1 h. Then, DAPI was stained in the dark for 5 min. The images were observed and collected under a confocal microscope or fluorescence microscope.
Flow cytometry assay
MCF-7 cells were seeded in 6-well plates at a density of 2 × 105 cells per well in a DMEM medium (containing 10% fetal bovine serum) and cultured at 37 °C in a 5% CO2 incubator until the cells were attached. The cells were collected by incubating different concentrations of 4k (0, 10, and 20 μM) with the cells for 24 h. The cells were resuspended in 500 μL binding buffer, followed by the addition of 5 μL Annexin V-FITC and 5 μL of PI. The reaction mixture was allowed to stand at room temperature for 15 min. The stained cells were analyzed by flow cytometry, and the results were analyzed using the FlowJo 7.6.1 software.
Conflicts of interest
The authors declare no conflict of interest.
Acknowledgements
This work was financially supported by the National Natural Science Foundation of China (No. 81202490). We also appreciate for technical supporting and assistance from Dr C. G Yan and Y. J. Song.
References
- F. Bray, J. Ferlay, I. Soerjomataram, R. L. S iegel, L. A. Torre and A. J. C. Jemal, Ca-Cancer J. Clin., 2018, 68, 394–424 CrossRef PubMed.
-
(a) D. Shi, F. Khan and R. Abagyan, J. Chem. Inf. Model., 2019, 59(6), 3006–3017 CrossRef CAS PubMed;
(b) Y. C. Wu, L. Cao, W. J. Mei, H. Q. Wu, S. H. Luo, H. Y. Zhan and Z. Y. Wang, Chem. Biol. Drug Des., 2018, 92, 1232–1240 CrossRef CAS PubMed;
(c) C. Li, D. Yang, X. Cao, F. Wang, H. Jiang, H. Guo, L. Du, Q. Guo and X. Yin, Biochem. Pharmacol., 2016, 113, 57–69 CrossRef CAS PubMed;
(d) N. Widmer, C. Bardin, E. Chatelut, A. Paci, J. Beijnen, D. Levêque, G. Veal and A. Astier, Eur. J. Cancer, 2014, 50, 2020–2036 CrossRef CAS PubMed;
(e) A. Levina, A. Mitra and P. A. Lay, Metallomics, 2009, 1, 458–470 RSC;
(f) H. L. Wong, R. Bendayan, A. M. Rauth, Y. Li and X. Y. Wu, Adv. Drug Delivery Rev., 2007, 59, 491–504 CrossRef CAS PubMed;
(g) C. Isanbor and D. O'Hagan, J. Fluorine Chem., 2006, 127, 303–319 CrossRef CAS;
(h) P. Nygren and R. Larsson, J. Intern. Med., 2003, 253, 46–75 CrossRef CAS PubMed.
-
(a) Z. Livshits, R. B. Rao and S. W. Smith, Emerg. Med. Clin. N. Am., 2014, 32, 167–203 CrossRef PubMed;
(b) M. O. Palumbo, P. Kavan, W. H. Miller Jr, L. Panasci, S. Assouline, N. Johnson, V. Cohen, F. Patenaude, M. Pollak, R. T. Jagoe and G. Batist, Front. Pharmacol., 2013, 4, 57 Search PubMed;
(c) V. T. DeVita and E. Chu, Cancer Res., 2008, 68, 8643–8653 CrossRef CAS PubMed;
(d) K. S. Brown, Semin. Interv. Radiol., 2006, 23, 99–108 CrossRef PubMed;
(e) T. Connors, Oncologist, 1996, 1, 180–181 Search PubMed.
- Y. Ohhara and H. Akita, Jpn. J. Clin. Med., 2015, 73, 133–138 Search PubMed.
-
(a) J. Zhou, G. Yu and F. Huang, Chem. Soc. Rev., 2017, 46, 7021–7053 RSC;
(b) H. Cui and B. Xu, Chem. Soc. Rev., 2017, 46, 6430–6432 RSC;
(c) A. G. Cheetham, P. Zhang, Y. A. Lin, L. L. Lock and H. Cui, J. Am. Chem. Soc., 2013, 135, 2907–2910 CrossRef CAS PubMed;
(d) C. Zhou, L. Gan, Y. Zhang, F. Zhang, G. Wang, L. Jin and R. Geng, Sci. China, Ser. B: Chem., 2009, 52, 415–458 CrossRef CAS.
- C. D. Gutsche, B. Dhawan, K. H. No and R. Muthukrishnan, J. Am. Chem. Soc., 1981, 103, 3782–3792 CrossRef CAS.
- A. Yousaf, S. A. Hamid, N. M. Bunnori and A. A. Ishola, Drug Des., Dev. Ther., 2015, 9, 2831–2838 CAS.
-
(a) L. An, J. W. Wang, J. D. Liu, Z. M. Zhao and Y. J. Song, Front. Chem., 2019, 7, 732 CrossRef PubMed;
(b) A. M. Hussain, M. U. Ashraf, G. Muhammad, M. N. Tahir and S. N. A. Bukhari, Curr. Pharm. Des., 2017, 23, 2377–2388 CrossRef PubMed;
(c) S. Karakurt, T. F. Kellici, T. Mavromoustakos, A. G. Tzakos and M. Yilmaz, Curr. Org. Chem., 2016, 20, 1043–1057 CrossRef CAS;
(d) L. An, L. L. Han, Y. G. Zheng, X. N. Peng, Y. S. Xue, X. K. Gu, J. Sun and C. G. Yan, Eur. J. Med. Chem., 2016, 123, 21–30 CrossRef CAS PubMed;
(e) Y. Zhou, H. Li and Y. W. Yang, Chin. Chem. Lett., 2015, 26, 825–828 CrossRef CAS;
(f) S. B. Nimse and T. Kim, Chem. Soc. Rev., 2013, 42, 366–386 RSC;
(g) F. Sansone, L. Baldini, A. Casnati and R. Ungaro, New J. Chem., 2010, 34, 2715–2728 RSC.
-
(a) K. Chojnackia, P. Wińskaa, M. Wielechowskaa, E. Łukowska-Chojnackaa, C. Tölzerb, K. Niefindb and M. Bretnera, Bioorg. Chem., 2018, 80, 266–275 CrossRef PubMed;
(b) N. C. Desai, D. Pandya and D. Vaja, Med. Chem. Res., 2017, 27, 1–9 Search PubMed;
(c) J. Akhtar, A. A. Khan, Z. Ali, R. Haider and M. S. Yar, Eur. J. Med. Chem., 2016, 125, 143–189 CrossRef PubMed;
(d) E. Łukowska-Chojnacka, M. Wielechowska, M. Poprzeczko and M. Bretner, Bioorg. Med. Chem., 2016, 24, 735–741 CrossRef PubMed;
(e) O. Afzal, S. Kumar, M. R. Haider, M. R. Ali, R. Kumar, M. Jaggi and S. Bawa, Eur. J. Med. Chem., 2015, 97, 871–910 CrossRef CAS PubMed;
(f) P. G. Baraldi, R. Romagnoli, I. Beria, P. Cozzi, C. Geroni, N. Mongelli, N. Bianchi, C. Mischiati and R. Gambari, J. Med. Chem., 2000, 43, 2675–2684 CrossRef CAS PubMed.
-
(a) R. F. George, S. S. Panda, E. S. M. Shalaby, A. M. Srour, I. S. A. Faragc and A. S. Girgis, RSC Adv., 2016, 6, 45434–45451 RSC;
(b) A. Malki, M. Mohsen, H. Aziz, O. Rizk, O. Shaban, M. El-Sayed, Z. A. Sherif and H. Ashour, Molecules, 2016, 21, 230–255 CrossRef PubMed;
(c) K. Li, Y. Li, D. Zhou, Y. B. Fan, H. Y. Guo, T. Y. Ma, J. C. Wen, D. Liu and L. X. Zhao, Bioorg. Med. Chem., 2016, 24, 1889–1897 CrossRef CAS PubMed;
(d) Y. T. Han, G. I. Choi, D. Son, N. J. Kim, H. Yun, S. Lee, D. J. Chang, H. Hong, H. Kim, H. Ha, Y. Kim, H. Park, J. Lee and Y. Suh, J. Med. Chem., 2012, 55, 9120–9135 CrossRef CAS PubMed;
(e) V. R. Solomon and H. Lee, Curr. Med. Chem., 2011, 18, 1488–1508 CrossRef CAS PubMed;
(f) K. Matsuno, Y. Masuda, Y. Uehara, H. Sato, A. Muroya, O. Takahashi, T. Yokotagawa, T. Furuya, T. Okawara, M. Otsuka, N. Ogo, T. Ashizawa, C. Oshita, S. Tai, H. Ishii, Y. Akiyama and A. Asai, ACS Med. Chem. Lett., 2010, 1, 371–375 CrossRef CAS PubMed;
(g) V. R. Solomon, C. Hu and H. Lee, Bioorg. Med. Chem., 2009, 17, 7585–7592 CrossRef CAS PubMed;
(h) A. Kamal, M. N. A. Khan, K. S. Reddy and K. Rohini, Bioorg. Med. Chem., 2007, 15, 1004–1013 CrossRef CAS PubMed.
- R. B. Shah, N. N. Valand, P. G. Sutariya and S. K. Menon, J. Inclusion Phenom. Macrocyclic Chem., 2016, 84, 173–178 CrossRef CAS.
- M. N. Soares, T. M. Gáscon, F. L. A. Fonseca, K. S. Ferreira and I. A. Bagatin, Mater. Sci. Eng., C, 2014, 40, 260–266 CrossRef CAS PubMed.
- S. Muneer, S. Memon, Q. K. Pahnwar, A. A. Bhatti and T. S. Khokhar, J. Anal. Sci. Technol., 2017, 8, 3–8 CrossRef.
-
(a) L. An, J. Wang, C. Wang, S. S. Zhou, J. Sun and C. G. Yan, New J. Chem., 2018, 10, 10689–10696 RSC;
(b) Y. Liu, J. Sun and C. G. Yan, J. Inclusion Phenom. Macrocyclic Chem., 2017, 87, 1–10 CrossRef;
(c) T. A. Alhujran, L. N. Dawe and P. E. Georghiou, Org. Lett., 2012, 14, 3530–3533 CrossRef CAS PubMed;
(d) K. Cottet, P. M. Marcos and P. J. Cragg, Beilstein J. Org. Chem., 2012, 8, 201–226 CrossRef CAS PubMed;
(e) E. A. Shokova and V. V. Kovalev, Russ. J. Org. Chem., 2004, 40, 607–643 CrossRef CAS;
(f) B. Masci, Tetrahedron, 2001, 57, 2841–2845 CrossRef CAS;
(g) G. Brodesser and F. J. Vögtle, J. Inclusion Phenom. Mol. Recognit. Chem., 1994, 19, 111–135 CrossRef CAS.
- P. M. Marcos, J. R. Ascenso, M. A. P. Segurado and J. L. C. Pereira, Tetrahedron, 2001, 57, 6977–6984 CrossRef CAS.
- L. An, H. H. Chen, Z. C. Qin and J. Ding, Chin. J. Chem. Reagents, 2009, 20, 17–19 CAS.
-
(a) X. Nqoro, N. Tobeka and B. A. Aderibigbe, Molecules, 2017, 22, 2268 CrossRef PubMed;
(b) A. A. Al-Amiery, R. I. Al-Bayati, K. Y. Saour and M. F. Radi, Res. Chem. Intermed., 2012, 38, 559–569 CrossRef CAS;
(c) S. Eswaran, A. V. Adhikari, N. K. Pal and I. H. Chowdhury, Bioorg. Med. Chem. Lett., 2010, 20, 1040–1044 CrossRef CAS PubMed;
(d) S. Kumar, S. Bawa and H. Gupta, Mini-Rev. Med. Chem., 2009, 9, 1648–1654 CrossRef CAS PubMed.
- K. Abouzid and S. Shouman, Bioorg. Med. Chem., 2008, 16, 7543–7551 CrossRef CAS PubMed.
- O. Norma, C. John, S. Helen, A. D. K. Hill, M. D. Enda, O. Niall and D. Michael, Clin. Cancer Res., 2003, 9, 738–742 Search PubMed.
- C. Bavoux, F. Vocanson, M. Perrin and R. Lamartine, J. Med. Chem., 1995, 22, 119–130 CAS.
- Z. M. Zhao, Y. Wang, J. Han, H. D. Zhu and L. An, Chem. Pharm. Bull., 2015, 63, 180–186 CrossRef CAS PubMed.
- G. M. Sheldrick, SHELXL-97, Program for crystal structure refinement, University of Göttingen, Gottingen, Germany, 1997 Search PubMed.
Footnote |
† Electronic supplementary information (ESI) available. See DOI: 10.1039/c9ra06876g |
|
This journal is © The Royal Society of Chemistry 2019 |
Click here to see how this site uses Cookies. View our privacy policy here.