DOI:
10.1039/C9RA06778G
(Paper)
RSC Adv., 2019,
9, 30659-30665
Transition metal-free one-pot synthesis of substituted pyrroles by employing aza-Wittig reaction†
Received
20th July 2019
, Accepted 12th September 2019
First published on 27th September 2019
Abstract
A mild and metal-free one-pot synthetic strategy has been developed for the construction of substituted pyrroles by employing aza-Wittig reaction from a unique and unexplored combination of chromones and phenacyl azides. This method does not compromise the diverse substitutions on both the phenacyl azides and chromones. The merits of this method are wide substrate scope, easy functionalization, short reaction time, operationally simple, and higher yields. Moreover, this method is amenable for the generation of a library of key pyrrole building blocks.
Introduction
Pyrroles are ubiquitous and important privileged scaffolds amongst the family of five membered N-heterocyclic pharmacophores which are widely distributed in natural products,1 medicinal agents2 and agrochemical research.3 Pyrroles are also having broad applications in electronics,4a molecular optics4b and widely used as versatile building blocks in organic synthesis.5 The biological activities of different substituted pyrroles such as antitumour,6 antibacterial,7 antifungal8 and immunosuppressant9 are immensely explored. A large number of pyrrole derivatives have been found to act as tubulin polymerization inhibitors,10 pesticides, as a natural antibiotic pyoluteorin11a and Cdc7 kinase inhibitor11b (I–III, Fig. 1). Therefore, the development of novel synthetic methods that provide substituted pyrrole derivatives is important. The traditional methods available for the synthesis of pyrroles are Paal Knorr,12 Hantzsch13 and Barton–Zard synthesis.14 These classical methods not only provide insights about the advancement in developing various methods in synthesis of pyrroles but also throw light regarding the vibrant activity in this field. However these methods employ multistep operations and harsh conditions. While a plethora of outstanding synthetic methods have been developed, however, it is still challenging to achieve direct access to substituted pyrroles.
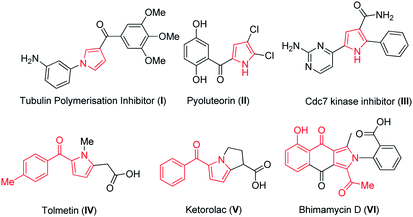 |
| Fig. 1 Representative examples of pyrrole based pharmaceutical derivatives. | |
Chromone-3-carbaldehyde is a versatile compound and widely used in the synthesis of different heterocyclic compounds. The synthesis and reactivity of the compounds have been reviewed.15
More precisely, formyl chromones are known to demonstrate their ability to react via electron deficient centers C-2, C-4 and the formyl group.16 Beyond its reactivity, they can provide access to compounds where the chromone ring is intact or converted to 2-hydroxy benzoyl derivatives resulting from the opening of pyran-4-one ring.17,18
On the other hand, organic azides are also extensively used as amine precursors, a source of highly reactive nitrene intermediates, a valuable dipole in 1,3-dipolar cycloaddition reactions19 and as a starting material of phosphoranes employed in aza-Wittig reactions to construct a variety of heterocycles.20–26
In recent years, many reports provide inputs regarding the use of chromones in the construction of substituted pyrroles. Kamijo et al. (Scheme 1a) reported the synthesis of pyrroles with metal salts i.e., silver or copper salts by using isocyanides and alkynes as starting materials.27 Liao et al.28 gave insights for the synthesis of pyrrole using formyl chromones, anilines and isocyanoacetates, a three component reaction in presence of silver catalyst (Ag2O) and PPh3. The reaction proceeds via an unexpected aza-Michael addition rather than conventional imine condensation to give polysubstituted pyrroles. Later, Qi et al. (Scheme 1b) illustrated the formation of pyrrole using chromones with isocyanides in presence of silver metal salt (Ag2CO3).29 The main drawbacks were usage of metal salt, harsh reaction conditions and multiple product formation. Hence, the efficient preparation of this class of molecules is still highly enviable. In continuation of our earlier efforts in the development of novel methods,30 herein we have reported a facile approach for synthesis of substituted pyrroles through a sequential one-pot reaction of formyl chromone and phenacyl azides (Scheme 1c). Moreover, the related highly functionalized pyrroles such as tolmetin, ketorolac (NSAIDS) and bhimamycin D (IV–VI, Fig. 1) which are the important targets in medicinal chemistry,31 this protocol provides a rapid access to the core structure of those compounds.
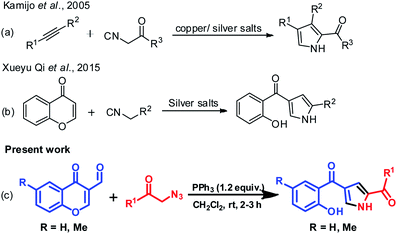 |
| Scheme 1 Synthesis of substituted functionalized pyrroles. (a) Isocyanide and alkyne cycloaddition, (b) chromones and isocyanides and (c) the method described in the manuscript. | |
Results and discussion
To develop simple and efficient route for the synthesis of pyrroles with readily available building blocks 1a and 2a, the synthetic strategy began with 1a and 2a by employing dppf (entry 1, Table 1) in toluene at 110 °C for 8 h, the reaction afforded the desired product 4a (entry 1, Table 1) in very mild 20% yield. When we used dppe, similar pattern was observed, where desired product was obtained in low yield (14%) (entry 2, Table 1). Notably, the use of triphenylphosphine (PPh3) (0.5 and 1 equiv.) in toluene, at 110 °C for 8 h, led to the formation of the product 4a albeit in lower yields i.e., 30% and 45% respectively (entry 3 and 4 Table 1). Nevertheless, to achieve higher yields, several other reaction parameters, such as reactant, solvents, reaction time and temperature were investigated (Table 1). By screening the equivalents of the reactant used in the reaction i.e., PPh3 suggested that 1.2 equiv. was considered to be optimum and the desired product 4a was obtained in good yield (entry 5, Table 1). Further, to increase the yields, we have performed the reaction with different solvents such as CH3OH, CH3CN, DMF, CH2Cl2, DMSO, THF and 1,4-dioxane. When the reaction was carried out in methanol at room temperature for 6 h, only 24% yield of the product was obtained, even though we have continued the reaction for longer time i.e., for 10 h (entry 6, Table 1).
Table 1 Optimization of the reaction conditions for 4aa
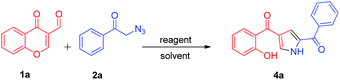
|
Entry |
Reactant |
Equiv. |
Solvent |
Temp (°C) |
Time (h) |
Yieldb (%) |
Reactions were performed with 1a (0.5 mmol), 2a (0.5 mmol).
Isolated yields.
n.d = not detected.
|
1 |
dppf |
0.5 |
Toluene |
110 |
8 |
20 |
2 |
dppe |
0.5 |
Toluene |
110 |
8 |
14 |
3 |
PPh3 |
0.5 |
Toluene |
110 |
8 |
30 |
4 |
PPh3 |
1 |
Toluene |
110 |
8 |
45 |
5 |
PPh3 |
1.2 |
Toluene |
110 |
8 |
65 |
6 |
PPh3 |
1.2 |
CH3OH |
rt |
6 |
24 |
7 |
PPh3 |
1.2 |
CH3CN |
rt |
4 |
28 |
8
|
PPh
3
|
1.2
|
CH
2
Cl
2
|
rt
|
2
|
85
|
9 |
PPh3 |
1.2 |
DMSO |
rt |
12 |
n.dc |
10 |
PPh3 |
1.2 |
THF |
rt |
4 |
28 |
11 |
PPh3 |
1.2 |
Dioxane |
rt |
12 |
15 |
12 |
PPh3 |
1.2 |
DMF |
rt |
8 |
n.dc |
In addition, even CH3CN was inferior with regard to the yield of 4a (28%, entry 7, Table 1). It was interesting to observe that when the reaction was carried out in CH2Cl2 at room temperature gave the desired product 4a in higher yield (85%, entry 8, Table 1). The other solvents such as THF and 1,4-dioxane gave less than 30% yield of the product (entry 10 and 11, Table 1). When DMSO and DMF were used as solvents, there was no formation of product 4a (entry 9 and 12, Table 1). Thus, use of 1.2 equiv. of PPh3 in CH2Cl2 (entry 8, Table 1) was found to be the optimized reaction condition for this transformation. During the course of reaction, we could identify that the reaction was proceeding via aza-Wittig mechanism by the formation of a key intermediate iminophosphorane which is responsible for the formation of substituted pyrrole. For further clarification of the product formed, we have carried out the D2O exchange (spectra can be observed in ESI†) of the compound 4b where we could observe the trap of OH and NH proton and could confirm the formation of 4b.
With the optimized reaction conditions in hand, substrate scope was examined by using broad range of phenacyl azides (Table 2). Taking into consideration of electronic effect by various electron rich and electron deficient substituents, we performed the reaction with different phenacyl azides having both electron donating and electron with-drawing groups. Surprisingly, both the groups were well tolerated, however electron with-drawing groups were little inferior as compared to donating groups as in case of 4a and 4e gave 85 and 60% yields respectively. The yields were slightly decreased and required longer reaction time when phenacyl azide was substituted with 4-bromo and 4-cyano (4g and 4h) which gave 55 and 45% respectively. Notably, the methodology also exhibited compatibility of electron with-drawing substitution on ortho, para and meta of the aromatic ring and does not adversely influence the reaction outcome (4k, 4l and 4m).
Table 2 Synthesis of different substituted pyrroles 4a–m from phenacyl azides and formyl chromonea
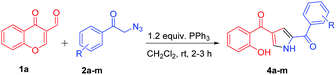
|
Reactions were performed with 1a (0.5 mmol), 2a (0.5 mmol) and 0.6 mmol of PPh3 in 5 mL CH2Cl2.
|
|
Further, the feasibility of this method could also be demonstrated concerning formyl chromones which were well tolerated with wide variety of phenacyl azides (Table 3). Methyl substitution on chromene ring was suitable for this reaction and was well tolerated with all electron donating and electron with-drawing groups. Moderate yields were reported with electron with-drawing substitutions such as F (5e, 69%), Cl (5f, 65%), Br (5g, 68%) and CN (5h, 62%). Having verified the viability of the developed protocol to obtain substituted pyrroles, we further explored the synthetic utility of this protocol by employing aliphatic azide as a substrate. To our delight, the reaction proceeded smoothly and the desired product 5n was obtained, albeit in low yield (23%).
Table 3 Scope of phenacyl azides with 6-methyl formyl chromonea
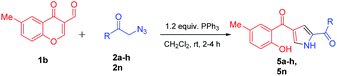
|
Reactions were performed with 1a (0.5 mmol), 2a (0.5 mmol) and 0.6 mmol of PPh3 in 5 mL CH2Cl2.
|
|
In order to investigate the efficiency and utility of this protocol, gram-scale reaction was carried out by employing substrates 1a (2 g, 11.4 mmol) and 2b (2.4 g, 11.4 mmol) using TPP under optimal reaction conditions. To our delight, reaction proceeded smoothly to give the corresponding product 4b in 77% isolated yield (Scheme 2). This facile metal-free approach under mild conditions makes it as a useful process for the synthesis of medicinally important molecules.
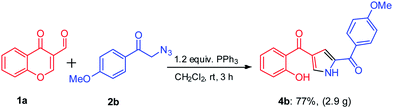 |
| Scheme 2 Gram scale synthesis of 4b. | |
To shed some light on the transformation of the reaction, a model reaction was performed with 2b and PPh3 which was monitored for the formation of (i) with the help of NMR. From NMR we could speculate that the appearance of protons in the region 4.0–4.5 ppm are of ylide protons in spectra (B) after 1.5 h of reaction time indicates the formation of (i). Later 1a was added and scanned for NMR we could detect an aldehydic proton at around 10.5 ppm, after 1.5 h of reaction time the aldehydic proton was diminishing indicating the formation of pyrrole ring by the observation of NH proton peak at 12 ppm (spectra (C)) and similarly OH proton peak at 11 ppm was observed with concomitant decrease in the intensity of active methylene proton of chromone (comparative NMR spectra can be viewed from ESI†).
Based on the above observation and prior available literature reports32 a plausible mechanistic pathway for the formation of pyrroles is outlined in Scheme 3. The reaction proceeds via formation of a key intermediate N-(triphenylphosphoranylidene) benzamide (i), the iminophosphorane through nucleophilic addition of the aryl phosphine at the terminal nitrogen atom of the phenacyl azide with expulsion of nitrogen. The nucleophilic nitrogen of iminophosphorane i further attacks the electrophilic carbon of aldehydic group of chromone 1a forming the carbon–nitrogen double bond of an imine ii along with the formation of byproduct phosphine oxide (iii), later an enolate (iv) is generated from ii through keto–enol tautomerisation which then readily cyclises resulting in the formation of dihydrochromeno pyrrole (v). Later, the keto intermediate v may readily aromatize and then followed by subsequent pyran ring opening and formation of pyrrole ring vi was observed.
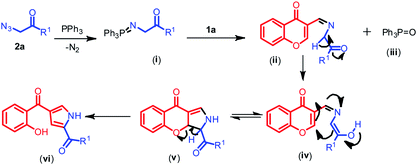 |
| Scheme 3 Plausible reaction pathway. | |
Conclusion
In conclusion, we have developed an operationally simple one-pot strategy for the synthesis of substituted pyrroles in metal-free conditions. Both electron with-drawing and electron donating groups on phenacyl azides were well tolerated including with chromones. Metal-free, mild reaction conditions and short reaction time is the exclusive feature of this approach. Moreover, the gram scale synthesis was accomplished and this protocol may possibly find applications in the natural product synthesis and drug discovery. The reactions were performed under an ambient atmosphere with no need for the exclusion of moisture or air. In addition, it is an environmentally friendly procedure as triphenyl phosphine oxide is the only byproduct generated during the reaction. Further applications of this approach in synthesis of various natural and pharmaceutically important heterocyclic compounds are ongoing in our laboratory.
Experimental section
General information
Unless otherwise specified, all solvents and other reagents are commercially available and used without further purification. All reagents were weighed and handled in air at room temperature. Column chromatography was performed on silica gel (60–120 and 100–200 mesh). NMR spectra were recorded on Bruker 500 NMR spectrometer. Chemical shifts were mentioned in parts per million (ppm, δ). Proton coupling patterns are described as singlet (s), doublet (d), triplet (t), quartet (q), doublet of doublet (dd), doublet triplet (dt) and multipet (m). HRMS were determined in negative mode with Agilent QTOF mass spectrometer 6540 series instrument. Melting points are determined on an electro thermal melting point apparatus and are uncorrected. The names of all compounds given in the Experimental section were taken from Chem ultra, version 12.0. The reactions wherever anhydrous conditions required are carried under nitrogen positive pressure using freshly distilled solvents. All evaporation of solvents was carried out under reduced pressure on Heidolph rotary evaporator below 40–45 °C.
General procedure for synthesis of substituted pyrroles: (4a–m), (5a–5h, 5n)
A mixture of phenacyl azide and CH2Cl2 was taken in a 10 mL round bottomed flask and stirred for 5 min; later PPh3 was added and further stirred for 30 min. Then the chromone was added and left at rt until the completion of reaction. After confirmed by TLC, the reaction mixture was quenched with water and extracted with CH2Cl2 (25 mL × 2), washed with brine (10 mL) and dried over anhydrous Na2SO4, filtered and concentrated in vacuo to give the crude product, which was further purified by silica gel chromatography using an ethyl acetate/petroleum ether gradient mixture to afford the desired product (4a–4m), (5a–5h, 5n).
(5-Benzoyl-1H-pyrrol-3-yl)(2-hydroxyphenyl) methanone (4a).
As a off white solid, yield 85%, mp 138–140 °C; 1H NMR (500 MHz, CDCl3): δ 11.97 (s, 1H), 10.29 (s, 1H), 7.98–7.93 (m, 2H), 7.87 (dd, J = 8.0, 1.6 Hz, 1H), 7.75 (dd, J = 3.2, 1.3 Hz, 1H), 7.66–7.60 (m, 1H), 7.56–7.47 (m, 3H), 7.38 (dd, J = 2.4, 1.4 Hz, 1H), 7.06 (dd, J = 8.4, 0.9 Hz, 1H), 6.96–6.91 (m, 1H); 13C NMR (125 MHz, CDCl3): δ 193.6, 185.3, 162.6, 137.3, 135.7, 132.7, 131.7, 131.5, 129.4, 129.1, 128.7, 125.5, 120.1, 119.8, 118.9, 118.5; HRMS (ESI): m/z cald for C18H13NO3 [M − H]−˙ 290.0823 found 290.0824.
(4-(2-Hydroxybenzoyl)-1H-pyrrol-2-yl)(4-methoxyphenyl) methanone (4b).
As a yellow solid, yield, 80%, mp 125–127 °C; 1H NMR (500 MHz, CDCl3): δ 11.99 (s, 1H), 10.32 (s, 1H), 8.02–7.96 (m, 2H), 7.88 (dd, J = 8.0, 1.5 Hz, 1H), 7.72 (dd, J = 3.2, 1.3 Hz, 1H), 7.52–7.47 (m, 1H), 7.38 (dd, J = 2.3, 1.5 Hz, 1H), 7.06 (d, J = 7.9 Hz, 1H), 7.01 (dd, J = 9.2, 2.3 Hz, 2H), 6.94 (dd, J = 11.1, 4.0 Hz, 1H), 3.91 (s, 3H); 13C NMR (125 MHz, CDCl3): δ 193.7, 184.0, 163.5, 162.5, 135.6, 131.8, 131.6, 131.5, 129.8, 129.2, 125.3, 120.2, 119.1, 118.9, 118.4, 114.0, 55.5; HRMS (ESI): m/z cald for C19H15NO4 [M − H]−˙ 320.0928 found 320.0931.
(4-(2-Hydroxybenzoyl)-1H-pyrrol-2-yl)(p-tolyl)methanone (4c).
As a light yellow solid, yield 70%, mp 154–156 °C; 1H NMR (500 MHz, CDCl3): δ 11.98 (s, 1H), 10.36 (d, J = 40.7 Hz, 1H), 7.90–7.86 (m, 3H), 7.74 (dd, J = 3.2, 1.4 Hz, 1H), 7.50 (ddd, J = 8.6, 7.3, 1.6 Hz, 1H), 7.38 (dd, J = 2.4, 1.4 Hz, 1H), 7.33 (d, J = 7.9 Hz, 2H), 7.08–7.04 (m, 1H), 6.95–6.91 (m, 1H), 2.46 (s, 3H); 13C NMR (125 MHz, CDCl3): δ 193.6, 185.1, 162.6, 143.6, 135.6, 134.6, 131.9, 131.6, 129.4, 129.4, 129.3, 125.4, 120.2, 119.6, 118.9, 118.4, 21.7; HRMS (ESI): m/z cald for C19H15NO3 [M − H]−˙ 304.0979 found 304.0982.
(5-([1,1′-Biphenyl]-4-carbonyl)-1H-pyrrol-3-yl)(2-hydroxyphenyl)methanone (4d).
As a cream solid 75%, mp 188–190 °C; 1H NMR (500 MHz, CDCl3): δ 11.97 (s, 1H), 10.15 (s, 1H), 8.07–8.03 (m, 2H), 7.89 (dd, J = 8.0, 1.6 Hz, 1H), 7.77–7.74 (m, 3H), 7.67–7.64 (m, 2H), 7.52–7.47 (m, 3H), 7.45–7.40 (m, 2H), 7.07 (dd, J = 8.4, 1.0 Hz, 1H), 6.94 (ddd, J = 8.2, 7.3, 1.1 Hz, 1H) ppm; 13C NMR (125 MHz, CDCl3): δ 193.6, 184.6, 162.6, 145.7, 139.8, 135.8, 135.7, 131.8, 131.6, 129.7, 129.2, 129.0, 128.3, 127.4, 127.3, 125.6, 120.2, 119.4, 118.9, 118.5; HRMS (ESI): m/z cald for C24H17NO3 [M − H]−˙ 366.1136 found 366.1140.
(5-(4-Fluorobenzoyl)-1H-pyrrol-3-yl)(2-hydroxyphenyl)methanone (4e).
As a pale yellow solid, yield 60%, mp 162–165 °C; 1H NMR (500 MHz, DMSO-d6): δ 12.83 (s, 1H), 10.92 (s, 1H), 7.97 (dd, J = 10.2, 3.8 Hz, 2H), 7.73 (s, 1H), 7.63 (d, J = 7.7 Hz, 1H), 7.49–7.37 (m, 3H), 7.15 (s, 1H), 7.02–6.92 (m, 2H); 13C NMR (125 MHz, DMSO-d6) δ 191.9, 183.6, 165.8, 163.9, 158.2, 134.4, 134.1, 131.8, 131.1, 131.6, 131.9, 130.8, 125.6, 124.7, 119.1, 119.7, 117.1, 116.1, 116.3; HRMS (ESI): m/z cald for C18H12FNO3 [M − H]−˙ 308.0728 found 308.0735.
(5-(4-Chlorobenzoyl)-1H-pyrrol-3-yl)(2-hydroxyphenyl) methanone (4f).
As a light yellow solid, yield 65%, mp 185–187 °C; 1H NMR (500 MHz, CDCl3): δ 11.94 (s, 1H), 10.32 (s, 1H), 7.91 (d, J = 8.4 Hz, 2H), 7.85 (dd, J = 7.9, 1.2 Hz, 1H), 7.76 (d, J = 1.9 Hz, 1H), 7.50 (t, J = 7.0 Hz, 2H), 7.36 (s, 1H), 7.07 (d, J = 8.2 Hz, 1H), 6.93 (t, J = 7.5 Hz, 1H); 13C NMR (125 MHz, CDCl3): δ 193.5, 183.8, 162.6, 139.3, 135.8, 135.5, 131.5, 131.4, 130.6, 130.4, 129.2, 129.2, 129.0, 125.7, 120.1, 119.6, 118.9, 118.5; HRMS (ESI): m/z cald for C18H12ClNO3 [M − H]−˙ 324.0433 found 324.0409.
(5-(4-Bromobenzoyl)-1H-pyrrol-3-yl)(2-hydroxyphenyl)methanone (4g).
As a light brown solid, yield 55%, mp 178–180 °C; 1H NMR (500 MHz, DMSO-d6): δ 12.84 (s, 1H), 10.90 (s, 1H), 7.86–7.75 (m, 4H), 7.71 (dd, J = 12.5, 5.3 Hz, 1H), 7.62 (d, J = 7.2 Hz, 1H), 7.44 (t, J = 7.2 Hz, 1H), 7.15 (s, 1H), 7.01–6.91 (m, 2H); 13C NMR (125 MHz, DMSO-d6): δ 191.8, 183.8, 158.5, 137.0, 134.1, 132.3, 132.1, 132.0, 131.7, 131.5, 131.1, 130.8, 126.6, 126.2, 125.8, 124.3, 119.9, 119.6, 117.5; HRMS (ESI): m/z cald for C18H12BrNO3 [M − 2H]−˙ 367.9928 found 367.9929.
4-(4-(2-Hydroxybenzoyl)-1H-pyrrole-2-carbonyl)benzonitrile (4h).
As a pale yellow solid, yield 45%, mp 208–210 °C; 1H NMR (500 MHz, DMSO-d6): δ 12.94 (s, 1H), 10.89 (s, 1H), 8.03 (dt, J = 16.3, 5.5 Hz, 4H), 7.77 (d, J = 2.0 Hz, 1H), 7.61 (d, J = 7.6 Hz, 1H), 7.45 (t, J = 7.7 Hz, 1H), 7.14 (s, 1H), 7.00–6.92 (m, 2H); 13C NMR (125 MHz, DMSO-d6): δ 191.7, 185.2, 183.6, 168.8, 158.6, 141.8, 134.4, 134.1, 133.5, 133.1, 132.4, 131.4, 131.0, 130.2, 129.7, 126.2, 126.0, 124.5, 124.3, 120.6, 119.6, 118.6, 117.6, 114.8, 104.0; HRMS (ESI): m/z cald for C19H12N2O3 [M − H]−˙ 315.0775 found 315.0779.
(4-(2-Hydroxybenzoyl)-1H-pyrrol-2-yl)(3,4,5 trimethoxyphenyl)methanone (4i).
As a light yellow solid, yield 70%, mp 203–205 °C; 1H NMR (500 MHz, CDCl3): δ 11.96 (s, 1H), 10.08 (s, 1H), 7.88 (dd, J = 7.9, 1.2 Hz, 1H), 7.74 (d, J = 1.7 Hz, 1H), 7.53–7.48 (m, 1H), 7.38 (s, 1H), 7.26 (s, 1H), 7.07 (d, J = 8.1 Hz, 1H), 6.92 (t, J = 7.3 Hz, 1H), 3.92 (dd, J = 32.3, 12.9 Hz, 9H); 13C NMR (125 MHz, CDCl3): δ 193.6, 184.0, 162.6, 153.2, 155.0, 142.5, 135.8, 134.3, 132.2, 131.6, 131.5, 129.0, 126.4, 125.7, 125.5, 120.1, 119.0, 118.8, 118.5, 108.3, 106.7, 61.0, 56.4, 56.3; HRMS (ESI): m/z cald for C21H19NO6 [M − H]−˙ 380.1140 found 380.1142.
(5-(2-Naphthoyl)-1H-pyrrol-3-yl)(2-hydroxyphenyl)methanone (4j).
As a cream solid, yield 75%, mp 194–196 °C; 1H NMR (500 MHz, DMSO-d6): δ 12.89 (s, 1H), 10.96 (s, 1H), 8.56 (s, 1H), 8.24–7.91 (m, 4H), 7.72 (d, J = 46.2 Hz, 4H), 7.45 (s, 1H), 7.26 (s, 1H), 7.03–6.93 (m, 2H); 13C NMR (125 MHz, DMSO-d6): δ 191.9, 184.8, 158.6, 135.3, 135.1, 134.1, 132.5, 131.9, 131.7, 131.0, 130.3, 129.9, 128.8, 128.8, 128.2, 127.5, 125.7, 125.4, 124.4, 119.9, 119.6, 117.6; HRMS (ESI): m/z cald for C22H15NO3 [M − H]−˙ 340.0979 found 340.0983.
(1H-Pyrrole-2,4-diyl)bis((2-hydroxyphenyl)methanone) (4k).
As a brown solid, yield 60%, mp 175–178 °C; 1H NMR (500 MHz, DMSO-d6): δ 12.76 (s, 1H), 10.89 (s, 1H), 10.43 (s, 1H), 7.68 (s, 1H), 7.58 (dd, J = 18.0, 7.6 Hz, 2H), 7.43 (s, 2H), 7.03 (s, 1H), 6.96 (dt, J = 12.6, 7.4 Hz, 4H) ppm; 13C NMR (125 MHz, DMSO-d6): δ 191.8, 186.1, 158.5, 157.4, 134.0, 133.5, 132.5, 131.5, 130.8, 130.3, 125.6, 124.6, 124.5, 120.1, 119.6, 119.5, 117.5, 117.4; HRMS (ESI): m/z cald for C14H13NO4 [M − H]−˙ 306.0772 found 306.0775.
(4-(2-Hydroxybenzoyl)-1H-pyrrol-2-yl)(4-nitrophenyl) methanone (4l).
As a yellow solid, yield 50%, mp 210–212 °C; 1H NMR (500 MHz, DMSO-d6): δ 12.93 (s, 1H), 10.89 (s, 1H), 8.37 (d, J = 8.1 Hz, 2H), 8.07 (d, J = 8.1 Hz, 2H), 7.77 (s, 1H), 7.59 (d, J = 7.4 Hz, 1H), 7.43 (t, J = 7.6 Hz, 1H), 7.15 (s, 1H), 6.95 (dd, J = 19.5, 7.8 Hz, 2H); 13C NMR (125 MHz, DMSO-d6): δ 191.7, 183.4, 158.3, 149.8, 143.3, 134.1, 132.6, 131.4, 130.8, 130.4, 126.0, 124.4, 124.3, 120.7, 119.6, 117.5; HRMS (ESI): m/z cald for C18H12N2O5 [M − H]−˙ 335.0673 found 335.0676.
(4-(2-Hydroxybenzoyl)-1H-pyrrol-2-yl)(3-nitrophenyl)methanone (4m).
As a light yellow solid, yield 52%, mp 205–207 °C; 1H NMR (500 MHz, DMSO-d6): δ 12.98 (s, 1H), 10.91 (s, 1H), 8.54 (d, J = 36.2 Hz, 2H), 8.32 (s, 1H), 7.83 (d, J = 49.5 Hz, 2H), 7.64 (s, 1H), 7.46 (s, 1H), 7.27 (s, 1H), 6.98 (s, 1H); 13C NMR (125 MHz, DMSO-d6): δ 191.7, 158.5 148.3, 135.3, 134.2, 132.5, 131.3, 131.0, 127.1, 126.0, 123.8, 120.5, 119.6, 117.6; HRMS (ESI): m/z cald for C18H12N2O5 [M − H]−˙ 335.0673 found 335.0676.
(5-Benzoyl-1H-pyrrol-3-yl)(2-hydroxy-5-methylphenyl)methanone (5a).
As a pale yellow solid, yield 84%, mp 146–148 °C; 1H NMR (500 MHz, CDCl3): δ 11.73 (s, 1H), 10.02 (s, 1H), 7.98–7.94 (m, 2H), 7.72 (dd, J = 3.1, 1.3 Hz, 1H), 7.67–7.61 (m, 2H), 7.56–7.51 (m, 2H), 7.38 (dd, J = 2.3, 1.3 Hz, 1H), 7.31 (dd, J = 8.4, 1.9 Hz, 1H), 6.97 (d, J = 8.4 Hz, 1H), 2.32 (s, 3H); 13C NMR (125 MHz, CDCl3): δ 193.5, 185.0, 160.4, 137.2, 136.7, 132.2, 131.6, 131.3, 129.0, 128.9, 128.6, 127.9, 125.6, 119.8, 119.5, 118.2, 20.6; HRMS (ESI): m/z cald for C19H15NO3 [M − H]−˙ 304.0979 found 304.0984.
(4-(2-Hydroxy-5-methylbenzoyl)-1H-pyrrol-2-yl)(4-methoxyphenyl) methanone (5b).
As a light yellow solid, yield 81%, mp 132–135 °C; 1H NMR (500 MHz, CDCl3): δ 11.75 (s, 1H), 10.25 (s, 1H), 8.04–7.96 (m, 2H), 7.70 (dd, J = 3.1, 1.3 Hz, 1H), 7.66 (d, J = 1.6 Hz, 1H), 7.37 (dd, J = 2.3, 1.3 Hz, 1H), 7.31 (dd, J = 8.4, 2.0 Hz, 1H), 7.03–7.00 (m, 2H), 6.97 (d, J = 8.4 Hz, 1H), 3.91 (d, J = 4.7 Hz, 3H), 2.32 (s, 3H); 13C NMR (125 MHz, CDCl3): δ 193.6, 183.7, 163.5, 160.4, 136.6, 131.7, 131.4, 131.3, 129.8, 128.6, 127.9, 125.5, 119.9, 118.7, 118.2, 113.9, 55.5, 20.6; HRMS (ESI): m/z cald for C20H17NO4 [M − H]−˙ 334.1085 found 334.1087.
(4-(2-Hydroxy-5-methylbenzoyl)-1H-pyrrol-2-yl)(p-tolyl) methanone (5c).
As a pale brown solid, yield 75%, mp 198–200 °C; 1H NMR (500 MHz, CDCl3): δ 11.75 (s, 1H), 10.26 (s, 1H), 7.88 (d, J = 8.1 Hz, 2H), 7.71 (dd, J = 3.2, 1.3 Hz, 1H), 7.65 (d, J = 1.6 Hz, 1H), 7.37 (dd, J = 2.4, 1.4 Hz, 1H), 7.31 (dd, J = 12.1, 5.2 Hz, 3H), 6.97 (d, J = 8.4 Hz, 1H), 2.46 (s, 3H), 2.32 (s, 3H); 13C NMR (125 MHz, CDCl3): δ 193.6, 185.0, 160.4, 143.6, 136.6, 134.6, 131.7, 131.4, 129.4, 129.3, 129.2, 129.0, 128.0, 125.5, 120.0, 119.3, 118.2, 21.6, 20.6; HRMS (ESI): m/z cald for C20H17NO3 [M − H]−˙ 318.1136 found 318.1140.
(5-([1,1′-Biphenyl]-4-carbonyl)-1H-pyrrol-3-yl)(2-hydroxy-5-methylphenyl)methanone (5d).
As a pale yellow solid, yield 74%, mp 143–145 °C; 1H NMR (500 MHz, CDCl3): δ 11.74 (s, 1H), 10.00 (s, 1H), 8.07–8.04 (m, 2H), 7.79–7.74 (m, 2H), 7.73 (dd, J = 3.1, 1.3 Hz, 1H), 7.67 (dt, J = 8.4, 4.2 Hz, 3H), 7.52–7.47 (m, 2H), 7.44–7.40 (m, 2H), 7.32 (dd, J = 8.5, 2.0 Hz, 1H), 6.97 (d, J = 8.4 Hz, 1H), 2.33 (s, 3H); 13C NMR (125 MHz, DMSO-d6): δ 191.9, 184.4, 156.0, 144.4, 139.4, 136.8, 134.7, 131.0, 131.7, 130.6, 129.9, 129.6, 129.6, 128.8, 128.3, 127.4, 127.4, 127.3, 125.8, 124.3, 119.6, 117.4, 40.3, 40.3, 40.2, 40.1, 40.0, 39.9, 39.7, 39.6, 39.4, 39.2, 20.4; HRMS (ESI): m/z cald for C25H19NO3 [M − H]−˙ 380.1292 found 380.1294.
(5-(4-Fluorobenzoyl)-1H-pyrrol-3-yl)(2-hydroxy-5-methylphenyl)methanone (5e).
As a pale brown solid, yield 69%, mp 110–112 °C; 1H NMR (500 MHz, DMSO-d6): δ 12.79 (s, 1H), 10.66 (s, 1H), 7.97 (dd, J = 8.5, 5.6 Hz, 2H), 7.73 (d, J = 1.7 Hz, 1H), 7.40 (dd, J = 9.8, 7.7 Hz, 3H), 7.26 (d, J = 8.4 Hz, 1H), 7.14 (s, 1H), 6.88 (d, J = 8.3 Hz, 1H), 2.27 (s, 3H); 13C NMR (125 MHz, DMSO-d6): δ 191.8, 183.5, 156.4, 134.8, 132.0, 131.9, 131.7, 131.6, 130.8, 128.2, 125.8, 124.2, 119.7, 117.4, 116.3, 116.1, 20.5; HRMS (ESI): m/z cald for C19H14FNO3 [M − H]−˙ 322.0885 found 323.0888.
(5-(4-Chlorobenzoyl)-1H-pyrrol-3-yl)(2-hydroxy-5-methylphenyl) methanone (5f).
As a pale brown solid, yield 65%, mp 201–204 °C; 1H NMR (500 MHz, CDCl3): δ 11.71 (s, 1H), 10.12 (s, 1H), 7.93–7.90 (m, 2H), 7.73 (dd, J = 3.1, 1.3 Hz, 1H), 7.63 (d, J = 1.6 Hz, 1H), 7.53–7.49 (m, 2H), 7.35 (dd, J = 2.3, 1.3 Hz, 1H), 7.32 (dd, J = 8.5, 1.9 Hz, 1H), 6.97 (d, J = 8.4 Hz, 1H), 2.30 (d, J = 19.1 Hz, 3H); 13C NMR (125 MHz, CDCl3): δ 193.4, 183.8, 160.4, 139.1, 136.7, 135.7, 135.4, 131.4, 131.3, 130.6, 130.4, 129.5, 129.1, 128.9, 127.9, 125.6, 125.5, 119.8, 119.6, 118.2, 117.9, 102.6, 40.0, 29.6, 20.8; HRMS (ESI): m/z cald. for C19H14ClNO3 [M − H]−˙ 338.0589 found 338.0594.
(5-(4-Bromobenzoyl)-1H-pyrrol-3-yl)(2-hydroxy-5-methylphenyl) methanone (5g).
As a pale yellow solid, yield 68%, mp 184–187 °C; 1H NMR (500 MHz, CDCl3): δ 11.70 (s, 1H), 9.99 (s, 1H), 7.85–7.82 (m, 2H), 7.73–7.70 (m, 1H), 7.69–7.66 (m, 2H), 7.63 (d, J = 1.6 Hz, 1H), 7.34 (dt, J = 3.5, 1.8 Hz, 1H), 7.32 (dd, J = 8.5, 2.0 Hz, 1H), 6.97 (d, J = 8.4 Hz, 1H), 2.32 (s, 3H); 13C NMR (125 MHz, CDCl3): δ 160.5, 136.8, 132.0, 131.9, 131.2, 130.5, 129.1, 127.9, 125.8, 119.4, 118.2, 20.3; HRMS (ESI): m/z cald for C19H14BrNO3 [M − H]−˙ 382.0084 found 382.0084.
4-(4-(2-Hydroxy-5-methylbenzoyl)-1H-pyrrole-2-carbonyl) benzonitrile (5h).
As a yellow solid, yield 62%, mp 198–203 °C; 1H NMR (400 MHz, CDCl3): δ 11.68 (s, 1H), 10.31 (s, 1H), 8.03 (d, J = 8.4 Hz, 2H), 7.84 (d, J = 8.4 Hz, 2H), 7.77 (dd, J = 3.2, 1.2 Hz, 1H), 7.61 (d, J = 1.4 Hz, 1H), 7.33 (dd, J = 8.5, 1.6 Hz, 2H), 6.97 (d, J = 8.5 Hz, 1H), 2.32 (s, 3H); 13C NMR (125 MHz, CDCl3): δ 193.3, 183.4, 160.5, 140.8, 137.0, 132.5, 131.2, 131.0, 130.0, 129.5, 128.0, 126.0, 120.3, 119.7, 118.4, 117.9, 116.1, 20.6; HRMS (ESI): m/z cald. for C20H14N2O3 [M − H]−˙ 329.0932 found 329.0935.
Ethyl 4-(2-hydroxy-5-methylbenzoyl)-1H-pyrrole-2-carboxylate (5n).
As a light yellow solid, yield 23%, mp 146–148 °C; 1H NMR (500 MHz, DMSO-d6): δ 12.64 (s, 1H), 10.67 (d, J = 19.6 Hz, 1H), 7.57 (dd, J = 3.3, 1.6 Hz, 1H), 7.37 (t, J = 10.0 Hz, 1H), 7.25 (dd, J = 8.4, 2.0 Hz, 1H), 7.15–7.08 (m, 1H), 6.89 (t, J = 14.1 Hz, 1H), 4.28 (q, J = 7.1 Hz, 2H), 2.27 (s, 3H), 1.30 (t, J = 7.1 Hz, 3H); 13C NMR (125 MHz, DMSO-d6): δ 191.9, 160.5, 156.4, 134.6, 130.6, 129.8, 128.1, 125.3, 125.0, 124.2, 124.1, 119.2, 117.4, 116.2, 60.7, 20.5, 14.8; HRMS (ESI): m/z cald for C25H19NO3 [M − H]−˙ 380.1292 found 380.1294.
Conflicts of interest
There are no conflicts to declare.
Acknowledgements
We are thankful to the Department of Pharmaceuticals (DoP), the Ministry of Chemicals and Fertilizers Govt of India, New Delhi for the award of NIPER Fellowship.
References
-
(a) V. Estevez, M. Villacampa and J. C. Menendez, Chem. Soc. Rev., 2010, 39, 4402 RSC;
(b) V. Estevez, M. Villacampa and J. C. Menendez, Chem. Soc. Rev., 2014, 43, 4633 RSC;
(c) H. Fan, J. Peng, M. T. Hamann and J. F. Hu, Chem. Rev., 2008, 108, 264 CrossRef CAS;
(d) A. Hall, S. Atkinson, S. H. Brown, I. P. Chessell, A. Chowdhury, G. M. P. Giblin, P. Goldsmith, M. P. Healy, K. S. Jandu, M. R. Johnson, A. D. Michel, A. Naylor and J. A. Sweeting, Bioorg. Med. Chem. Lett., 2007, 17, 1200 CrossRef CAS;
(e) C. V. Galliford and K. A. Scheidt, Angew. Chem., Int. Ed., 2007, 46, 8748 CrossRef CAS PubMed.
-
(a) J. Lehuede, B. Fauconneau, L. Barrier, M. Ourakow, A. Piriou and J. M. Vierfond, Eur. J. Med. Chem., 1999, 34, 991 CrossRef CAS;
(b) A. Furstner, Angew. Chem., 2003, 115, 3706 (
Angew. Chem., Int. Ed.
, 2003
, 42
, 3582
) CrossRef.
-
(a) L. M. D. Coen, T. S. A. Heugebaert, D. Garcia and C. V. Stevens, Chem. Rev., 2016, 116, 80 CrossRef PubMed;
(b) J. W. Blunt, B. R. Copp, R. A. Keyzers, M. H. G. Munroa and M. R. Prinsep, Nat. Prod. Rep., 2014, 31, 160 RSC;
(c) M. S. Mohamed and S. S. Fathallah, Mini-Rev. Org. Chem., 2014, 11, 477 CrossRef CAS;
(d) S. S. Gholap, Eur. J. Med. Chem., 2016, 110, 13 CrossRef CAS;
(e) M. Takase, T. Narita, W. Fujita, M. S. Asano, T. Nishinaga, H. Benten, K. Yoza and K. Mullen, J. Am. Chem. Soc., 2013, 135, 8031 CrossRef CAS;
(f) D. Imbri, J. Tauber and T. Opatz, Mar. Drugs, 2014, 12, 6142 CrossRef CAS.
-
(a) R. E. Nizurski-Mann and M. P. Cava, Heterocycles, 1992, 34, 2003 CrossRef;
(b) C. D'Silva and D. A. Walker, J. Org. Chem., 1998, 63, 6715 CrossRef.
-
(a)
T. P. Toube, B. A. Trofimov, G. Cirrincione, A. M. Almerico, E. Aiello, G. Dattolo, H. McNab and L. C. Monahan, in Chemistry of Heterocyclic Compounds: Pyrroles, Part II, The Synthesis, Reactivity and Physical Properties of Substituted Pyrroles, ed. R. A. Jones, John Wiley & Sons Inc., New York, 2008, vol. 48, p. 1 Search PubMed;
(b)
E. T. Pelkey, in Progress in Heterocyclic Chemistry, ed. G. W. Gribble and J. A. Joule, Elsevier, S. A., 2005, vol. 17, p. 109 Search PubMed.
-
(a) J. T. Gupton, Top. Heterocycl. Chem., 2006, 2, 53 CrossRef CAS;
(b) N. Amishiro, S. Nagamura, E. Kobayashi, A. Okamoto, K. Gomi and H. Saito, Chem. Pharm. Bull., 1999, 47, 1393 CrossRef CAS;
(c) N. Amishiro, S. Nagamura, E. Kobayashi, A. Okamoto, K. Gomi, M. Okabe and H. Saito, Bioorg. Med. Chem., 2000, 8, 1637 CrossRef CAS.
- R. W. Burli, D. McMinn, J. A. Kaizerman, W. Hu, Y. Ge, Q. Pack, V. Jiang, M. Gross, M. Garcia, R. Tanaka and H. E. Moser, Bioorg. Med. Chem. Lett., 2004, 14, 1253 CrossRef CAS.
-
(a) H. M. Meshram, B. R. V. Prasad and D. A. Kumar, Tetrahedron Lett., 2010, 51, 3477 CrossRef CAS;
(b) M. Del Poeta, W. A. Schell, C. C. Dykstra, S. Jones, R. R. Tidwell, A. Czarny, M. Bajic, A. Kumar, D. Boykin and J. R. Perfect, Antimicrob. Agents Chemother., 1998, 42, 2495 CrossRef CAS;
(c) M. Z. Wang, H. Xu, T. W. Liu, Q. Feng, S. J. Yu, S.-H. Wang and Z. M. Li, Eur. J. Med. Chem., 2011, 46, 1463 CrossRef CAS.
- F. A. Davis, K. Bowen, H. Xu and V. Velvadapu, Tetrahedron, 2008, 64, 4174 CrossRef CAS.
- G. La Regina, R. Bai, A. Coluccia, V. Famiglini, S. Pelliccia, S. Passacantilli, C. Mazzoccoli, V. Ruggieri, L. Sisinni, A. Bolognesi, W. M. Rensen, A. Miele, M. Nalli, R. Alfonsi, L. Di Marcotullio, A. Gulino, A. Brancale, E. Novellino, G. Dondio, S. Vultaggio, M. Varasi, C. Mercurio, E. Hamel, P. Lavia and R. Silvestri, J. Med. Chem., 2014, 57, 6531 CrossRef CAS.
-
(a) M. Menichincheri, C. Albanese, C. Alli, D. Ballinari, A. Bargiotti, M. Caldarelli, A. Ciavolella, A. Cirla, M. Colombo, F. Colotta, V. Croci, R. D'Alessio, M. D'Anello, A. Ermoli, F. Fiorentini, B. Forte, A. Galvani, P. Giordano, A. Isacchi, K. Martina, A. Molinari, J. K. Moll, A. Montagnoli, P. Orsini, F. Orzi, E. Pesenti, A. Pillan, F. Roletto, A. Scolaro, M. Tato, M. Tibolla, B. Valsasina, M. Varasi, P. Vianello, D. Volpi, C. Santocanale and E. Vanotti, J. Med. Chem., 2010, 53, 7296 CrossRef CAS;
(b) D. M. Bailey, R. E. Johnson and U. J. Salvador, J. Med. Chem., 1973, 16, 1298 CrossRef CAS.
-
(a) L. Knorr, Ber. Dtsch. Chem. Ges., 1884, 17, 1635 CrossRef;
(b) C. Paal, Ber. Dtsch. Chem. Ges., 1885, 18, 367 CrossRef;
(c) H. S. P. Rao, S. Jothilingam and H. W. Scheeren, Tetrahedron, 2004, 60, 1625 CrossRef CAS;
(d) G. Minetto, L. F. Raveglia, A. Sega and M. Taddei, Eur. J. Org. Chem., 2005, 2005, 5277 CrossRef.
- A. Hantzsch, Ber. Dtsch. Chem. Ges., 1890, 23, 1474 CrossRef.
-
(a) N. C. Misra, K. Panda, H. Ila and H. Junjappa, J. Org. Chem., 2007, 72, 1246 CrossRef CAS;
(b) J. L. Bullington, R. R. Wolff and P. F. Jackson, J. Org. Chem., 2002, 67, 9439 CrossRef CAS;
(c) D. H. R. Barton, J. Kervagoret and S. Z. Zard, Tetrahedron, 1990, 46, 7587 CrossRef CAS.
- C. K. Ghosh, J. Heterocycl. Chem., 1983, 20, 1437 CrossRef CAS.
- G. Sabitha, Aldrichimica Acta, 1996, 29, 15 Search PubMed.
- C. K. Ghosh, Indian J. Chem., Sect. B: Org. Chem.
Incl. Med. Chem., 1997, 36, 968 Search PubMed.
-
(a) C. K. Ghosh, Heterocycles, 2004, 63, 2875 CrossRef CAS;
(b) J. H. Boyer and F. C. Canter, Chem. Rev., 1954, 54, 1 CrossRef CAS;
(c) A. S. Plaskon, S. V. Ryabukhin, D. M. Volochnyuk, K. S. Gavrilenko, A. N. Shivanyuk and A. A. Tolmachev, J. Org. Chem., 2008, 73, 6010 CrossRef CAS PubMed;
(d) M. Lacova, A. Puchala, E. Solcanyova, J. Lac, P. Kois, J. Chovancova and D. Rasala, Molecules, 2005, 10, 809 CrossRef CAS PubMed;
(e) A. G. P. R. Figueiredo, A. C. Tome, A. M. S. Silva and J. A. S. Cavaleiro, Tetrahedron, 2007, 63, 910 CrossRef CAS;
(f) L. M. Sanchez, A. G. Sathicq, J. L. Jios, G. T. Baronetti, H. J. Thomas and G. P. Romanelli, Tetrahedron Lett., 2011, 52, 4412 CrossRef CAS.
-
(a) G. L'Abbe, Chem. Rev., 1969, 69, 345 CrossRef;
(b) S. Nekkanti, V. Pooladanda, M. Veldandi, R. Tokala, C. Godugu and N. Shankaraiah, ChemistrySelect, 2017, 2, 7210 CrossRef CAS.
- T. Patonay, K. Konya and E. Juhasz-Toth, Chem. Soc. Rev., 2011, 40, 2797 RSC.
-
The Chemistry of the Azido Group, ed. S. Patai, Wiley-Interscience, London-New York-Sydney-Toronto, 1971 Search PubMed.
- G. L'Abbe and A. Hassner, Angew. Chem., Int. Ed. Engl., 1971, 10, 98 CrossRef.
- G. L'Abbe, Angew. Chem., 1975, 87, 831 CrossRef.
-
Azides and Nitrenes; Reactivity and Utility, ed. E. V. F. Scriven, Academic Press, Orlando, 1984 Search PubMed.
- E. V. F. Scriven and K. Turnbull, Chem. Rev., 1988, 88, 297 CrossRef CAS.
- S. Brase, C. Gil, K. Knepper and V. Zimmermann, Angew. Chem., Int. Ed., 2005, 44, 5188 CrossRef CAS; K. Banert, Liebigs Ann./Recl., 1997, 2005 CrossRef.
- S. Kamijo, C. Kanazawa and Y. Yamamoto, J. Am. Chem. Soc., 2005, 127, 9260 CrossRef CAS.
- J. Y. Liao, W. J. Yap, J. E. Wu, M. W. Wong and Y. Zhao, Chem. Commun., 2017, 53, 9067 RSC.
- X. Qi, H. Xiang, Y. Yang and C. Yang, RSC Adv., 2015, 5, 98549 RSC.
- M. Sathish, J. Chetna, N. H. Krishna, N. Shankaraiah, A. Alarifi and A. Kamal, J. Org. Chem., 2016, 81, 2159 CrossRef CAS.
-
(a) Y. Arikawa, H. Nishida, O. Kurasawa, A. Hasuoka, K. Hirase, N. Inatomi, Y. Hori, J. Matsukawa, A. Imanishi, M. Kondo, N. Tarui, T. Hamada, T. Takagi, T. Takeuchi and M. Kajino, J. Med. Chem., 2012, 55, 4446 CrossRef CAS;
(b) B. D. Roth, Prog. Med. Chem., 2002, 40, 1 CrossRef CAS.
-
(a) Z. Lao and P. H. Toy, Beilstein J. Org. Chem., 2016, 12, 2577 CrossRef CAS;
(b) H. B. Tukhtaev, K. L. Ivanov, S. I. Bezzubov, D. A. Cheshkov, M. Y. Melnikov and E. M. Budynina, Org. Lett., 2019, 21, 1087 CrossRef CAS.
Footnote |
† Electronic supplementary information (ESI) available. See DOI: 10.1039/c9ra06778g |
|
This journal is © The Royal Society of Chemistry 2019 |
Click here to see how this site uses Cookies. View our privacy policy here.