DOI:
10.1039/C9RA06465F
(Paper)
RSC Adv., 2019,
9, 30139-30143
KOtBu-promoted oxidative dimerizations of 2-methylquinolines to 2-alkenyl bisquinolines with molecular oxygen†
Received
18th August 2019
, Accepted 18th September 2019
First published on 23rd September 2019
Abstract
KOtBu-promoted oxidative dimerizations of 2-methylquinolines with molecular oxygen as the oxidant have been developed for the first time. The mild reaction conditions allow the homo- and cross-dimerizations of 2-methylquinolines to give functionalized 2-alkenyl bisquinolines in highly trans-selective manners.
Introduction
2-Alkenylquinolines are widely present in many medicinally active compounds and natural products of biological significance, such as VUF 5017 (CysLT1 antagonist),1 Chimanine B (antileishmanial activity),2 and Montelukast (drug for asthma).3 As such, the construction of 2-alkenylquinolines remains a significant research area in organic synthesis.4 In particular, 2-alkenyl bisquinolines exhibit significant activity against the replication of HIV-1, and also against intracellular amastigote forms of L. amazonensis and L. infantum.5 However, in comparison with the well-established synthetic methodologies for 2-alkenylquinolines syntheses, the construction of 2-alkenyl bisquinolines is still less explored. The classical synthetic approach to 2-alkenyl bisquinolines relies on the Perkin reaction of 2-methylquinoline with quinoline-2-carboxaldehyde in the presence of excess amount of Ac2O (Scheme 1a).6 Despite the synthetic utility to obtain 2-alkenyl bisquinolines, this methodology suffers from the harsh conditions for condensation. More problematic is that quinoline-2-carboxaldehydes should be synthesized first, mainly based on the oxidation of 2-methylquinolines by using large amounts of toxic SeO2.7 From an economic and synthetic point of view, the development of new strategies to obtain 2-alkenyl bisquinolines in a green manner is highly desirable.
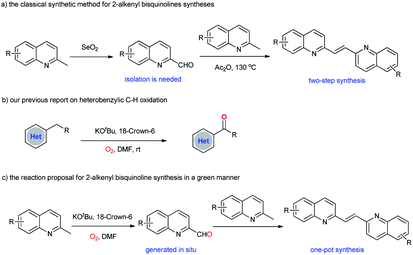 |
| Scheme 1 The classical synthetic approach to 2-alkenyl bisquinolines and the present reaction proposal. | |
Retrosynthetic analysis showed that 2-alkenyl bisquinolines arise via the condensation reactions of 2-methylquinolines with quinoline-2-carboxaldehydes would be a straightforward, and efficient way.8 In this context, the development of an efficient method for the in situ generation of quinoline-2-carboxaldehydes would solve the problems associated with 2-alkenyl bisquinolines syntheses. Even though many methodologies for quinoline-2-carboxaldehydes syntheses from 2-methylquinolines have been developed,9 the compatibility of two distinct reaction conditions between quinoline-2-carboxaldehyde synthesis and the following condensation reaction with 2-methylquinoline remains an unsolved problem. Our group recently reported a KOtBu-promoted (hetero)benzylic C–H oxidation with molecular oxygen as the oxidant (Scheme 1b).10 A variety of (hetero)aryl ketones were obtained in good to excellent yields under basic reaction conditions. We envisioned that 2-methylquinolines could also undergo this KOtBu-promoted oxidation reaction to give quinoline-2-carboxaldehydes in an effective manner.11,12 The subsequent condensation reaction under basic reaction conditions could realize one-pot synthesis of 2-alkenyl bisquinolines (Scheme 1c). Herein, we report that symmetric and unsymmetric 2-alkenyl bisquinolines could be obtained in one-pot via a KOtBu-promoted oxidative dimerization of 2-methylquinolines with molecular oxygen as the oxidant.
Results and discussions
Initially, the oxidative dimerization of 2-methylquinoline (1a) was chosen as the model reaction to optimize the reaction conditions (Table 1). When the reaction was carried out in N,N-dimethylformamide (DMF) with KOtBu as the base and 18-crown-6 (18-C-6) as the additive under oxygen balloon at 50 °C for 20 h, the desired 2-alkenyl bisquinoline (2a) was obtained in 72% yield (entry 1). Replacement of KOtBu with NaOtBu, LiOtBu, or KOH led to lower yields (entries 2, 3 and 5). GC-MS analysis of the reaction mixtures showed that quinoline-2-carboxaldehyde was existed. However, when Cs2CO3 was employed as the base, no desired product was detected, and the conversion of 1a was less than 5% (entry 4). Next, tetramethylethylenediamine (TMEDA), or 1,10-phenanthroline (1,10-phen) as the additive were examined, and lower yields were observed (entries 6 and 7). The solvent optimizations showed that no desired product was obtained when using DMSO, toluene, or CH3CN as the solvent (entries 8–10). Then, a series of chemical oxidants such as TBHP, K2S2O8, and m-CPBA were screened, however, no desired product was observed (entries 11–13). When the reaction was carried out under air atmosphere, only trace amount of desired product was observed (entry 14); while no desired product was observed in the absence of KOtBu (entry 15). These two control experiments demonstrated that KOtBu and oxygen atmosphere are essential for this dimerization reaction. Increasing or decreasing the reaction temperature failed to improve the isolated yield of 2a (entries 16 and 17).
Table 1 Optimization studiesa

|
Entry |
Base |
Oxidant |
Additive |
Solvent |
Yieldb,c (%) |
Reaction conditions: 1a (0.2 mmol), base (0.22 mmol), oxidant, additive (0.22 mol), and solvent (1.5 mL) were stirred at 50 °C for 20 h. Isolated yield. The conversion of 1a determined by GC-MS was shown in the parentheses. 0.2 mmol of oxidant was used. 80 °C. 30 °C. |
1 |
KOtBu |
O2 balloon |
18-C-6 |
DMF |
72 (94) |
2 |
NaOtBu |
O2 balloon |
18-C-6 |
DMF |
61 (88) |
3 |
LiOtBu |
O2 balloon |
18-C-6 |
DMF |
55 (71) |
4 |
Cs2CO3 |
O2 balloon |
18-C-6 |
DMF |
0 (<5) |
5 |
KOH |
O2 balloon |
18-C-6 |
DMF |
41 (70) |
6 |
KOtBu |
O2 balloon |
TMEDA |
DMF |
57 (74) |
7 |
KOtBu |
O2 balloon |
1,10-Phen |
DMF |
55 (79) |
8 |
KOtBu |
O2 balloon |
18-C-6 |
DMSO |
0 (<5) |
9 |
KOtBu |
O2 balloon |
18-C-6 |
Tol |
0 (<5) |
10 |
KOtBu |
O2 balloon |
18-C-6 |
CH3CN |
0 (<5) |
11d |
KOtBu |
TBHP |
18-C-6 |
DMF |
0 (15) |
12d |
KOtBu |
K2S2O8 |
18-C-6 |
DMF |
0 (11) |
13d |
KOtBu |
m-CPBA |
18-C-6 |
DMF |
0 (<5) |
14 |
KOtBu |
Air |
18-C-6 |
DMF |
Trace (16) |
15 |
none |
O2 balloon |
18-C-6 |
DMF |
0 (0) |
16e |
KOtBu |
O2 balloon |
18-C-6 |
DMF |
62 (95) |
17f |
KOtBu |
O2 balloon |
18-C-6 |
DMF |
29 (57) |
With the optimized reaction conditions in hand, the substrate scope of the homo-dimerization reaction was investigated (Scheme 2). It was found that the electronic nature of the substituents on the aromatic ring had a significant effect on the yield. When the substrates bearing electron-donating groups, such as 2,6-dimethylquinoline, 6-methoxyquinaldine, and 8-methoxyquinaldine, were employed as the substrates under the optimized conditions, the dimerization reactions proceeded smoothly to afford the corresponding products 2b–2d in 64–71% yields. However, when 6-nitroquinaldine or 6-chloroquinaldine were examined under the optimized conditions, the reaction mixtures were complex. Some uncharacterized byproducts were obtained, however, no desired 2-alkenyl bisquinolines (2k–2l) were observed by GC-MS. 2-Methylbenzo[h]quinoline was also a suitable substrate to give the desired product 2e in 49% yield. Then, pyridine derivatives were tested under the optimized conditions. However, pyridine derivatives showed low reactivity. By increasing the reaction temperature to 100 °C, the desired products 2f–2h were obtained in good yields. It is noteworthy that 4-methylpyrimidine and 2-methylpyrazine were also well tolerated under this reaction conditions, giving the desired products 2i and 2j in 53% and 52% yields, respectively.
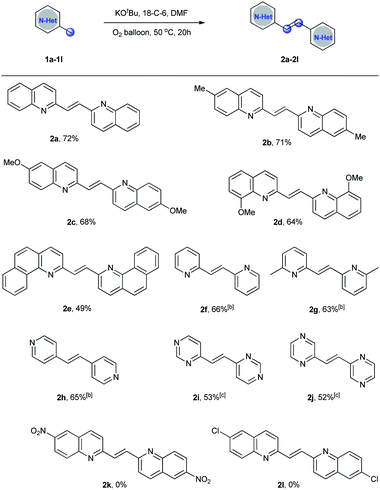 |
| Scheme 2 The substrate scope of homo-dimerization reactionsa. a Reaction conditions: substrates (0.2 mmol), KOtBu (0.22 mmol), and 18-Crown-6 (0.22 mmol) were stirred in 1.5 mL DMF at 50 °C for 20 h under O2 balloon. b 100 °C, 24 h. c 36 h. | |
To make this methodology more appealing, the substrate scope of cross-dimerization reactions was also examined. As shown in Scheme 3, with a minor modification of the optimized reaction conditions, the cross-dimerization reactions were realized. Even though the unexpected self-dimerizations of 2-methylquinoline and substituted 2-methylquinolines make the reaction mixture difficult for purification, the desired unsymmetrical 2-alkenyl bisquinolines 3–7 were obtained in acceptable yields.
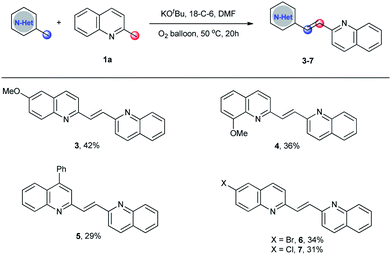 |
| Scheme 3 The substrate scope of cross-dimerization reactionsa. a Reaction conditions: 2-methylquinoline (0.6 mmol), substituted 2-methylquinoline (0.2 mmol), KOtBu (0.4 mmol), and 18-Crown-6 (0.4 mmol) were stirred in 3.0 mL DMF at 50 °C for 20 h under O2 balloon. | |
To get a mechanistic insight into the oxidative dimerization reactions, the condensation reaction of 2-methylquinoline (1a) with 2-quinolinecarboxaldehyde (8) was carried out. As shown in Scheme 4a, 2-alkenyl bisquinoline (2a) was obtained in 79% yield under N2 atmosphere. This result suggests that 2-quinolinecarboxaldehyde (8) is a key intermediate for this reaction. Besides, the successful transformation of this condensation reaction under N2 atmosphere also indicates that O2 atmosphere is essential for the oxidation of 2-methylquionline (1a) into 2-quinolinecarboxaldehyde (8), not for the condensation reaction sequence. Besides, the adding of 2,2,6,6-tetramethylpiperidine-1-oxyl (TEMPO) to the standard conditions led to the decrease of the yield of 2a to 37% (Scheme 4b), which indicates that this oxidative dimerization involved the radical intermediate, however, reaction pathway involving anion species could not be ruled out. The competition reactions suggest that the electronic nature of substituents on the aromatic rings has much influence on the reaction of methylquinolines with 2-quinolinecarboxaldehyde (8) (Scheme 4c and d), and the electron-donating group slowed the reaction rate.
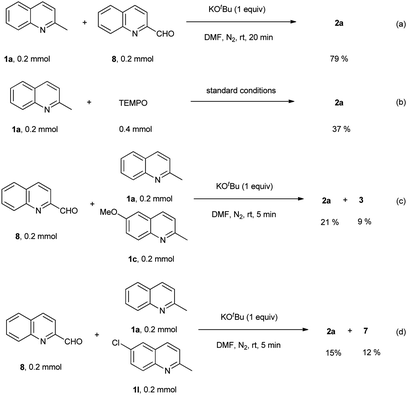 |
| Scheme 4 Control experiments. | |
Based on the previous reports and control experiments, a plausible reaction mechanism for the formation of 2-alkenyl bisquinoline 2a and 7 was shown in Scheme 5. First, t-BuO− abstracts a proton from DMF to give carbamoyl anion 9.13 The intermolecular interaction between DMF and the complex of 18-C-6 with KOtBu was demonstrated in our previous report.10 Subsequently, intermediate 9 has an electron transfer with DMF to give carbamoyl radical 11.14 Intermediate 11 then abstracts a hydrogen from 1a to generate benzylic type radical 12, which is quenched by molecular oxygen to give the hydroperoxide intermediate 14. Finally, intermediate 14 eliminated one molecule of water to generate 2-quinolinecarboxaldehyde 8, which has a Knoevenagel-type condensation reaction with another molecule of methylquionline to give symmetric 2-alkenyl bisquinoline 2a or unsymmetric 2-alkenyl bisquinoline 7.
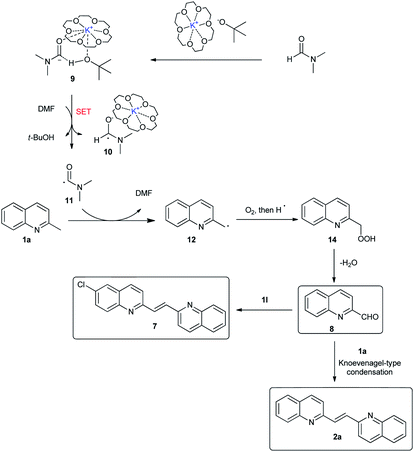 |
| Scheme 5 Plausible mechanism for the formation of 2a and 7. | |
Conclusions
We have developed the first example of oxidative dimerization of 2-methylquinolines for the highly trans-selective synthesis of 2-alkenyl bisquinolines with molecular oxygen as the oxidant. The current method is superior to the previously reported 2-alkenyl bisquinoline synthetic strategies because mild reaction conditions and readily accessible starting materials are used, and toxic reagents are avoided.
Experimental
All reactions were performed in oven-dried reaction tubes under oxygen atmosphere unless otherwise mentioned. Commercial reagents were purchased from Energy chemical, and TCI. Flash column chromatographic purification of products was accomplished using forced-flow chromatography on Silica Gel (100–200 mesh). 1H-NMR, and 13C-NMR spectra were recorded on a Bruker Avance 300 or 400 spectrometer at ambient temperature. The chemical shifts (δ) and coupling constants (J) were expressed in ppm and Hz respectively. The abbreviations used for explaining the multiplicities were as follows: s = singlet, d = doublet, t = triplet, q = quartet, m = multiplet, br = broad. High resolution mass spectra (HRMS) were measured using EI-TOF Mass spectrometer. Melting points were uncorrected and measured with a micro melting point apparatus.
General procedure for the synthesis of symmetric 2-alkenyl bisquinolines 2a–2l
To a reaction tube (10 mL) was added 2-methylquinolines (0.2 mmol), KOtBu (25 mg, 0.22 mmol), and dry 18-Crown-6 (58 mg, 0.22 mmol). The tube was then evacuated and back-filled with oxygen three times. Anhydrous DMF (1.5 mL) was added subsequently. The reaction mixture was stirred at 50 °C for 20 h under oxygen balloon. After the reaction, DMF was removed by vacuum distillation. The resulting crude was directly purified by column chromatography on silica gel (elute: petroleum
:
ethyl acetate = 15
:
1–8
:
1) to give the desired 2-alkenyl bisquinolines as white or yellow solids.
General procedure for the synthesis of unsymmetric 2-alkenyl bisquinolines 3–7
To a reaction tube (10 mL) was added 2-methylquinoline (86 mg, 0.6 mmol), substituted 2-methylquinoline (0.2 mmol), KOtBu (45 mg, 0.4 mmol), and 18-Crown-6 (106 mg, 0.4 mmol). The tube was then evacuated and back-filled with oxygen three times. Anhydrous DMF (3.0 mL) was added subsequently. The reaction mixture was stirred at 50 °C for 20 h under oxygen balloon. After the reaction, DMF was removed by vacuum distillation. The resulting crude was directly purified by column chromatography on silica gel (elute: petroleum
:
ethyl acetate = 15
:
1–8
:
1) to give the desired 2-alkenyl bisquinolines as white or pale yellow solids.
Conflicts of interest
There are no conflicts to declare.
Acknowledgements
We are grateful to the financial support from the Foundation of He'nan Educational Committee (16A150057) and the Science and Technology Bureau of Nanyang (2016KJGG39).
References
- M. E. Zwaagstra, S. H. H. F. Schoenmakers, P. H. J. Nederkoorn, E. Gelens, H. Timmerman and M.-Q. Zhang, J. Med. Chem., 1998, 41, 1439 CrossRef CAS PubMed.
- J. P. Michael, Nat. Prod. Rep., 2005, 22, 627 RSC.
- M. L. Belley, S. Leger, M. Labelle, P. Roy, Y. B. Xiang and D. Guay, US Pat. Appl., US 5565473, 1996.
- For recent examples, see:
(a) J. J. Mousseau, J. A. Bull and A. B. Charette, Angew. Chem., Int. Ed., 2010, 49, 1115 CrossRef CAS PubMed;
(b) B. Qian, P. Xie, Y. Xie and H. Huang, Org. Lett., 2011, 13, 2580 CrossRef CAS PubMed;
(c) Y.-G. Zhang, J.-K. Xu, X.-M. Li and S.-K. Tian, Eur. J. Org. Chem., 2013, 2013, 3648 CrossRef CAS;
(d) P. Wen, Y. Li, K. Zhou, C. Ma, X. Lan, C. Ma and G. Huang, Adv. Synth. Catal., 2012, 354, 2135 CrossRef CAS;
(e) L. Xu, Z. Shao, L. Wang, H. Zhao and J. Xiao, Tetrahedron Lett., 2014, 55, 6856 CrossRef CAS;
(f) L. Bering and A. P. Antonchick, Org. Lett., 2015, 17, 3134 CrossRef CAS PubMed;
(g) H. Xia, Y. H. Liu, P. Zhao, S. H. Gou and J. Wang, Org. Lett., 2016, 18, 1796 CrossRef CAS PubMed;
(h) G. E. M. Crisenza, E. M. Dauncey and J. F. Bower, Org. Biomol. Chem., 2016, 14, 5820 RSC;
(i) Z. Zhang, C. Pi, H. Tong, X. Cui and Y. Wu, Org. Lett., 2017, 19, 440 CrossRef CAS PubMed.
- M. A. Fakhfakh, A. Fournet, E. Prina, J. F. Mouscadet, X. Franck, R. Hocquemiller and B. Figadere, Bioorg. Med. Chem., 2003, 11, 5013 CrossRef CAS PubMed.
-
(a) M. A. Fakhfakh, X. Franck, A. Fournet, R. Hocquemiller and B. Figadère, Synth. Commun., 2002, 32, 2863 CrossRef CAS;
(b) L. He, J.-Q. Wang, Y. Gong, Y.-M. Liu, Y. Cao, H.-Y. He and K.-N. Fan, Angew. Chem., Int. Ed., 2011, 50, 10216 CrossRef CAS PubMed.
- For selected examples on the oxidation of 2-methylquionline to 2-quinolinecarboxaldehyde using SeO2, see:
(a) D. Ding, L. P. Dwoskin and P. A. Crooks, Tetrahedron Lett., 2013, 54, 5211 CrossRef CAS PubMed;
(b) H. Bao, B. Zhou, H. Jin and Y. Liu, Org. Biomol. Chem., 2019, 17, 5021 RSC.
- A pioneering work concerning the oxidative dimerization of toluene with benzaldehyde giving stilbene has been described, see: S. Takemoto, E. Shibata, M. Nakajima, Y. Yumoto, M. Shimamoto and H. Matsuzaka, J. Am. Chem. Soc., 2016, 138, 14836 CrossRef CAS PubMed.
- For selected examples on the oxidation of 2-methylquionline into 2-quinolinecarboxaldehyde using other oxidants, see:
(a) Y. Nagasawa, Y. Tachikawa, E. Yamaguchi, N. Tada, T. Miura and A. Itoh, Adv. Synth. Catal., 2016, 358, 178 CrossRef CAS;
(b) M. Liu, X. Chen, T. Chen, Q. Xu and S.-F. Yin, Org. Biomol. Chem., 2017, 15, 9845 RSC;
(c) A. Rahim, S. P. Shaik, M. F. Baig, A. Alarifi and A. Kamal, Org. Biomol. Chem., 2018, 16, 635 RSC;
(d) Z. C. Yan, C. F. Wan, Y. Yang, Z. G. Zha and Z. Y. Wang, RSC Adv., 2018, 8, 23058 RSC;
(e) R. Ye, Y. Cao, X. Xi, L. Liu and T. Chen, Org. Biomol. Chem., 2019, 17, 4220 RSC.
- H. Q. Wang, Z. Wang, H. C. Huang, J. J. Tan and K. Xu, Org. Lett., 2016, 18, 5680 CrossRef CAS PubMed.
- Recent examples on the t-BuOK-mediated oxidative reactions, see:
(a) H. Yang, D.-Z. Chu and L. Jiao, Chem. Sci., 2018, 9, 1534 RSC;
(b) L. Wu, V. T. Annibale, H. Jiao, A. Brookfield, D. Collision and I. Manners, Nat. Commun., 2019, 10, 2786 CrossRef PubMed.
- For selected recent examples on metal-free activated C(sp3)–H functionalization, see:
(a) C. J. Evoniuk, G. P. Gomes, S. P. Hill, S. Fujita, K. Hanson and I. V. Alabugin, J. Am. Chem. Soc., 2017, 139, 16210 CrossRef CAS PubMed;
(b) J. Tan, T. Zheng, Y. Yu and K. Xu, RSC Adv., 2017, 7, 15176 RSC;
(c) A. Gini, T. Brandhofer and O. G. Mancheño, Org. Biomol. Chem., 2017, 15, 1294 RSC;
(d) S. Zhang, F. Lian, M. Xue, T. Qin, L. Li, X. Zhang and K. Xu, Org. Lett., 2017, 19, 6622 CrossRef CAS PubMed;
(e) L. A. Combee, B. Raya, D. Wang and M. K. Hilinski, Chem. Sci., 2018, 9, 935 RSC;
(f) H. Wang, J. Zhang, J. Tan, L. Xin, Y. Li, S. Zhang and K. Xu, Org. Lett., 2018, 20, 2505 CrossRef CAS PubMed;
(g) T. Zheng, J. Tan, R. Fan, S. Su, B. Liu, C. Tan and K. Xu, Chem. Commun., 2018, 54, 1303 RSC;
(h) X. Tian, X. Cheng, X. Yang, Y.-L. Ren, K. Yao, H. Wang and J. Wang, Org. Chem. Front., 2019, 6, 952 RSC;
(i) S. Haldar and C. K. Jana, Org. Biomol. Chem., 2019, 17, 1800 RSC.
- M. P. Drapeau, I. Fabre, L. Grimaud, I. Ciofini, T. Ollevier and M. Taillefer, Angew. Chem., Int. Ed., 2015, 54, 10587 CrossRef PubMed.
-
(a) W.-T. Wei, X.-J. Dong, S.-Z. Nie, Y.-Y. Chen, X.-J. Zhang and M. Yan, Org. Lett., 2013, 15, 6018 CrossRef CAS PubMed;
(b) W.-T. Wei, Y.-J. Cheng, Y. Hu, Y.-Y. Chen, X.-J. Zhang, Y. Zou and M. Yan, Adv. Synth. Catal., 2015, 357, 3474 CrossRef CAS;
(c) J. P. Barham, G. Coulthard, K. J. Emery, E. Doni, F. Cumine, G. Nocera, M. P. John, L. E. A. Berlouis, T. McGuire, T. Tuttle and J. A. Murphy, J. Am. Chem. Soc., 2016, 138, 7402 CrossRef CAS PubMed.
Footnotes |
† Electronic supplementary information (ESI) available. See DOI: 10.1039/c9ra06465f |
‡ These two authors contributed equally to this work. |
|
This journal is © The Royal Society of Chemistry 2019 |
Click here to see how this site uses Cookies. View our privacy policy here.