DOI:
10.1039/C9RA06243B
(Paper)
RSC Adv., 2019,
9, 29652-29658
Synthesis of novel functional ionic liquids and their application in biomass
Received
10th August 2019
, Accepted 30th August 2019
First published on 19th September 2019
Abstract
A series of dicationic ionic liquids (ILs) including [PF6][(PYR)C4(MIM)][Cl], [PF6][(PYR)C4(PYR)][Cl], [PF6][(PYR)C5(MIM)][Cl], and [PF6][(PYR)C5(PYR)][Cl], and monocationic ILs including [(PYR)C4Cl][PF6], [(PYR)C5Cl][PF6], [(MIM)C2COOH][PF6] and [(PYR)C2COOH][PF6] were synthesized. Their thermal stability and melting points were determined. Their solubility with organic solvents and the miscibility with water were investigated. These functional ILs are hydrophilic at high temperatures and they are hydrophobic at low temperatures, which enable the effective isolation of the resulting reducing sugar. High yields of reducing sugar were obtained for corn stalk after 8 h (20.73%) and potato starch after 6 h (72.50%) by the treatment with the mixture of [PF6][(PYR)C4(PYR)][Cl] and [(PYR)C2COOH][PF6]. The reuse of dicationic and monocationic ILs was successfully performed and no significant reduction in yields of reducing sugar was observed. These functional ILs have important implications in the design of homogeneous and heterogeneous systems with water and organic solvents, which could be used to satisfy some specific applications.
Introduction
Ionic liquids (ILs) generally consist of organic cations such as imidazolium, pyridinium, ammonium and phosphonium cations with inorganic or organic anions such as halogen, tetrafluoroborate, and hexafluorophosphate anions.1,2 ILs have several superior properties including extremely low vapor pressure, excellent thermal and chemical stability, and a large liquid temperature range.3,4 Therefore, ILs have been reported in a variety of applications, such as separation processes, organic synthesis, and biomass pretreatment.5–8
Recently, dicationic ionic liquids (DILs) that are composed of two mono-cations have been reported.9–12 DILs have been classified as symmetrical DILs (two mono-cations are identical) and unsymmetrical DILs (two mono-cations are different). In particular, imidazolium and pyridinium based DILs have shown greater promising physicochemical properties.13,14 DILs can be adjusted by changing their cations, anions, and the length of linker chains between the two cations to achieve significant properties to satisfy some specific chemical task.15–17
Over the years, ILs-pretreatment of biomass has received dominant attention. ILs are described to be capable to decrease the crystallinity of cellulose and to reduce the portions of hemicellulose and lignin.18–20 In recent years, ILs as pretreated solvents followed by the enzymatic hydrolysis, are widely utilized in academic and industrial applications.21–25 The pretreatment of cotton stalk was performed at 150 °C for 30 min in 1-ethyl-3-imidazolium acetate ([EMIM]CH3COO) to release 65% of reducing sugars after 72 h of enzymatic hydrolysis.26 The saccharication degree of [EMIM]CH3COO-pretreated corn stalk by the crude enzyme of Penicillium oxalicum HC6 and the cellulase from Trichoderma reesei ATCC26921 were determined as 24.58% and 21.40%, respectively.27
Currently, functional acidic ILs as catalysts and solvents become potentially a sustainable approach, which avoid the addition of mineral acid in the hydrolysis process of lignocelluloses. As reported, hydrolysis of fiber sludge using hydroxyalkylimidazolium hydrogen sulphate produced 29% of reducing sugars at 100 °C for 30 min.28 Hydrolysis of switchgrass in 1-(sulfobutyl)-3-methylimidazolium chloride resulted in 58.1% reducing sugars under thermal and microwave conditions for the pretreatment for 1 h at 70 °C followed by treatment with the addition of water for another 1 h at 70 °C.29
Although ILs-pretreatments of lignocelluloses are successfully utilized, the low rate and high cost of cellulase limit their industrial utilization. The addition of a large amount of corrosive inorganic acid may cause environmental problems. Actually, all the ILs for lignocellulose treatment are hydrophilic in current studies, thus it is much difficult to separate reducing sugar from the common ILs and also functional acidic ILs. The recovery and reuse of hydrophilic ILs become a problem.30,31 The alternative techniques are still necessary to achieve the sugars in the conversion of biomass to biofuels.
In this study, a series of novel functional ionic liquids were synthesized. These ILs are hydrophilic at high temperatures and they are hydrophobic at low temperatures, which provide an opportunity to improve the isolation of sugars and the reuse of ILs. Both dicationic ILs and monocationic ILs were investigated for their physicochemical properties including thermal stability, the melting points, the solubility in water and organic solvents. The ILs were investigated for the first time in the conversion of potato starch and corn stalk to prepare reducing sugar.
Results and discussion
Synthesis of ionic liquids
Ionic liquids were synthesized using quaternization reaction followed by anion metathesis. The synthetic route for dicationic ionic liquids (DILs) was shown in Scheme 1. Initially, [(PYR)CnCl][PF6] was prepared by heating one equivalent of pyridine with one equivalent of dichloroalkane in solvent-free conditions, followed by the anion exchange reaction with KPF6. After that, DILs were obtained by the alkylation of [(PYR)CnCl][PF6] with one equivalent of 1-methylimidazole or pyridine to afford symmetrical and unsymmetrical dicationic compounds such as [PF6][(PYR)Cn(PYR)][Cl] and [PF6][(PYR)Cn(MIM)][Cl]. Monocationic acidic ILs were obtained by the alkylation of 1-methylimidazole or pyridine with 3-chloropropionic acid, followed by the anion metathesis with KPF6 to give [(PYR)C2COOH][PF6] and [(MIM)C2COOH][PF6] (Scheme 2).
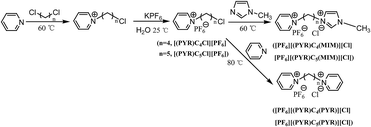 |
| Scheme 1 Synthetic routes of dicationic ILs. | |
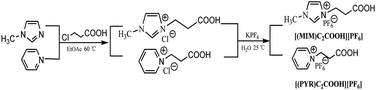 |
| Scheme 2 Synthetic routes of monocationic ILs. | |
In the synthetic process of DILs, pyridine as the weak nucleophile was used in the first step, because it replaced only one chloride of dichloroalkane, whereas stronger nucleophiles such as 1-methylimidazole would attach both sides of the dichloroalkane. However, a yield of product, for example, [Cl][(PYR)Cn(PYR)][Cl] was still observed, which was isolated by the column chromatography (CH2Cl2/MeOH = 3/1).
Thermal stability
Thermal stability and melting points of ILs were determined and the results were presented in Table 1 and Fig. 1. Generally, unsymmetrical dicationic ILs containing 1-methylimidazolium group, e.g. [PF6][(PYR)C4(MIM)][Cl] (237 °C) were more stable than those symmetrical dicationic ILs containing the pyridinium ring, e.g. [PF6][(PYR)C4(PYR)][Cl] (235 °C), possibly due to the better nucleophilic capability of 1-methylimidazolium than pyridinium.32–36 A similar study showed that the thermal stability of [PF6][(PYR)C3(MIM)][Br] was 252 °C, which was higher than that of [C3(PYR)2][Br]2 (240 °C).37 Less thermal stability was also obtained for [(PYR)C2COOH][PF6] compared with [(MIM)C2COOH][PF6] in this work. The low thermal stability was caused for monocationic acidic ILs by introducing of the carboxyl group.
Table 1 Thermal decomposition temperature and melting points of ILsa
ILs |
Td (°C) |
Mp (°C) |
Td decomposition temperature; Mp melting points. |
[(PYR)C4Cl][PF6] |
238 |
49.6 |
[(PYR)C5Cl][PF6] |
248 |
40.7 |
[PF6][(PYR)C4(MIM)][Cl] |
237 |
98.3 |
[PF6][(PYR)C4(PYR)][Cl] |
235 |
160.9 |
[PF6][(PYR)C5(MIM)][Cl] |
232 |
81.5 |
[PF6][(PYR)C5(PYR)][Cl] |
230 |
97.3 |
[(MIM)C2COOH][PF6] |
215 |
91.7 |
[(PYR)C2COOH][PF6] |
190 |
101.3 |
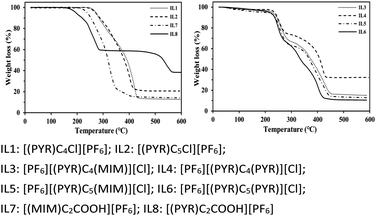 |
| Fig. 1 Thermogravimetry curves of ILs. | |
In addition, an impact of the symmetry of the linkage chain of the dication on the thermal stability was observed.38 A slight decrease of thermal stability was obtained for [PF6][(PYR)C5(MIM)][Cl] and [PF6][(PYR)C5(PYR)][Cl] than that of [PF6][(PYR)C4(MIM)][Cl] and [PF6][(PYR)C4(PYR)][Cl].
Melting points
As shown in Table 1, symmetrical dicationic ILs containing pyridinium group ([PF6][(PYR)C4(PYR)][Cl]) (160.9 °C) have higher melting points than unsymmetrical dicationic ILs with imidazolium ring ([PF6][(PYR)C4(MIM)][Cl]) (98.3 °C). As suggested by Welton and Wasserscheid, this may be attributed to the relative degree of charge dispersion around the cation. Imidazolium has good charge dispersion compared with pyridine.35,39 A low symmetry of dication of [PF6][(PYR)C4(MIM)][Cl] also assisted the low melting point.
Previous reports indicated that the melting points of dicationic ILs decreased with the increase of alkyl linkage chain.40 It was described that the melting point of [C3(MIM)2][PF6]2 was 131 °C, which was higher that of [C4(MIM)2][PF6]2 (120 °C).27,37 In this study, [(PYR)C5Cl][PF6] that generated longer linkage chain displayed a lower melting point than that of [(PYR)C4Cl][PF6]. In addition, the symmetry of the linkage chain of the dication has a significant effect on the melting points. A reduced symmetry of linkage caused decreased melting points. As a result, [PF6][(PYR)C5(MIM)][Cl] and [PF6][(PYR)C5(PYR)][Cl] exhibited lower melting points than that of [PF6][(PYR)C4(MIM)][Cl] and [PF6][(PYR)C4(PYR)][Cl].
Solubility of ionic liquids with organic solvents
The synthesized ILs were determined in several representative solvents at room temperature. As summarized in Table 2, both dicationic and monocationic ILs exhibited good solubility in high polar solvents,41 e.g. dimethyl sulfoxide (DMSO), but they were not soluble in non-polar solvents, e.g. diethyl ether (Et2O).
Table 2 Solubility of ILs in various organic solventsa
|
DMSO |
MeOH |
EtOH |
CH3CN |
DMK |
EtOAc |
CH2Cl2 |
Et2O |
IL1: [(PYR)C4Cl][PF6]; IL2: [(PYR)C5Cl][PF6]; IL3: [PF6][(PYR)C4(MIM)][Cl]; IL4: [PF6][(PYR)C4(PYR)][Cl]; IL5: [PF6][(PYR)C5(MIM)][Cl]; IL6: [PF6][(PYR)C5(PYR)][Cl]; IL7: [(MIM)C2COOH][PF6]; IL8: [(PYR)C2COOH][PF6]. “+++”: 0.1 g ionic liquid was easily dissolved in the solvent (<0.1 mL). “++”: 0.1 g ionic liquid was easily dissolved in the solvent (0.1–1 mL). “+”: 0.1 g ionic liquids was dissolved in the solvent (1–10 mL). “−”: 0.1 g ionic liquid was not dissolved in the solvent (>10 mL). |
IL1 |
+ |
+ |
+ |
+++ |
+++ |
+++ |
++ |
− |
IL2 |
+ |
+ |
+ |
+++ |
+++ |
+++ |
++ |
− |
IL3 |
+ |
− |
− |
+ |
+ |
− |
− |
− |
IL4 |
+ |
− |
− |
− |
− |
− |
− |
− |
IL5 |
+ |
− |
− |
+ |
+ |
− |
− |
− |
IL6 |
+ |
− |
− |
− |
− |
− |
− |
− |
IL7 |
+ |
+ |
+ |
++ |
++ |
− |
− |
− |
IL8 |
+ |
+ |
+ |
− |
− |
− |
− |
− |
In this work, monocationic ILs generated better solubility than dicationic ILs. Monocationic ILs were soluble in most organic solvents. Dicationic ILs were not dissolved in methanol (MeOH), ethanol (EtOH), and dichloromethane (CH2Cl2). However, monocationic acidic ILs with carboxyl group were soluble in MeOH, EtOH, but they were not soluble in CH2Cl2.
Particularly, imidazolium-based ILs displayed good solubility compared with pyridinium-based ILs. Dicationic ILs with imidazolium group were dissolved in acetonitrile (CH3CN) and acetone (DMK). In contrast, dicationic ILs with pyridinium ring were not soluble in CH3CN and DMK. Moreover, [(MIM)C2COOH][PF6] was soluble in CH3CN and DMK, whereas [(PYR)C2COOH][PF6] was not soluble in these two organic solvents.
In current studies, the reported ILs were insoluble in ethyl acetate (EtOAc), because of their polar properties. Interestingly, [(PYR)C4Cl][PF6] and [(PYR)C5Cl][PF6] with haloalkyl chain were readily dissolved in EtOAc. Therefore, these ILs with different solubility could be selected and employed in various areas to satisfy different requirements.
Miscibility of ionic liquids with water
Generally, ILs with the anions of PF6− and NTf2− are water-immiscible, whereas others are water-miscible. Interestingly, these dicationic and monocationic ILs are miscible with water at high temperatures and they are immiscible with water at low temperatures. It was found that dicationic ILs could form a homogeneous or heterogeneous system with water, depending on the dealing temperature.
As illustrated in Fig. 2, when the temperature was higher, the miscibility of dicationic ILs with water was better with a reduced amount of water. For example, 1.0 g of [PF6][(PYR)C4(MIM)][Cl] was miscible with 4 mL of water at 100 °C. However, 105 mL of water was required at room temperature. Similarly, 6 mL of water was used to dissolve 1.0 g of [PF6][(PYR)C4(PYR)][Cl] at 100 °C, whereas 232 mL of water was employed at room temperature.
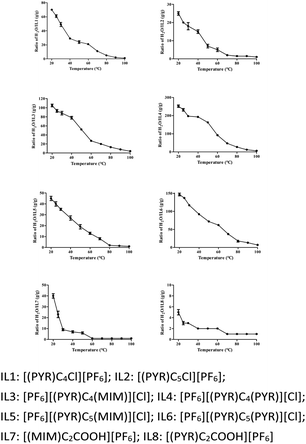 |
| Fig. 2 Miscibility of ILs with water at different temperature. | |
Monocationic ILs were more soluble with water than dicationic ILs. For instance, 1.0 g of [(PYR)C4Cl][PF6] was miscible with 61 mL of water at room temperature, whereas 105 mL and 232 mL of water for [PF6][(PYR)C4(MIM)][Cl] and [PF6][(PYR)C4(PYR)][Cl] were required, respectively. Meanwhile, good miscibility was observed with imidazolium-based dicationic ILs than pyridinium-based dicationic ILs. Moreover, monocationic ILs containing carbonyl group (–COOH) displayed better solubility than others, for example, [(PYR)C2COOH][PF6] was completely dissolved only with 5 mL of water under the assay condition.42
With the enhance of cationic unsymmetry, the miscibility of dicationic ILs with water was increased (Fig. 2). Significantly, 105 mL of water was used to form a homogeneous system with 1.0 g of [PF6][(PYR)C4(MIM)][Cl] at room temperature. And yet, 45 mL was required for 1.0 g of [PF6][(PYR)C5(MIM)][Cl], because an increase of the of the linkage chain of dication improved the hydrophilic properties of dicationic ILs.
Hydrolysis of corn stalk and potato starch in ILs
Currently, ILs with the addition of mineral acids or functional acidic ILs for the treatment of biomass were reported. Hernoux-Villière et al.43 found that the treatment of potato starch in aqueous 1-(4-sulfobutyl)-3-methylimidazolium chloride at 80 °C for 2 h produced 43% of the total reducing sugar. Seddon et al.44 reported that the total yield of reducing sugar for potato starch was 54% with the Brønsted-acidic ionic liquids and was 6% with the 1-allyl-3-methylimidazolium chloride at 80 °C for 1 h. In this study, a high yield of reducing sugar (72.50%) for potato starch was achieved with the treatment of the mixture of [PF6][(PYR)C4(PYR)][Cl] and [PF6][(PYR)C2COOH] at 100 °C for 6 h (Fig. 3). However, when potato starch was treated with [(PYR)C2COOH][PF6] or [(MIM)C2COOH][PF6] as a sole solvent at 100 °C for 6 h, the yields of reducing sugar were 43.28% and 15.25%, respectively.
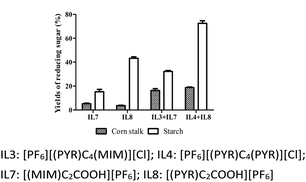 |
| Fig. 3 Yields of reducing sugar after ILs-treatment. | |
In addition, Li et al.45 reported that corn stalk was hydrolyzed in 1-buty-3-methylimidazolium chloride with sulfuric acid as a catalyst at 100 °C for 6 h to give 37% of total reducing sugar. Ma et al.46 demonstrated that corn stalk was treated by N-methyl-2-pyrrolidonium methanesulfonate, N-methyl-2-pyrrolidonium hydrogen sulfate and N-methyl-2-pyrrolidonium chloride at 90 °C for 30 min, and the resulting yield of reducing sugar was 23.51%, 22.26% and 20.19%, respectively. In this study, a high yield of reducing sugar for corn stalk was achieved at 18.80% for 6 h (Fig. 3) and 20.73% for 8 h (Fig. 4) with the mixture of [PF6][(PYR)C4(PYR)][Cl] and [(PYR)C2COOH][PF6] at 100 °C. Nevertheless, when corn stalk was treated with [(PYR)C2COOH][PF6] or [(MIM)C2COOH][PF6] as the sole solvent at 100 °C for 6 h, the yields of reducing sugar were 6.94% and 6.50%, respectively.
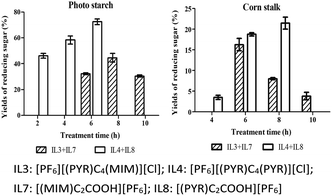 |
| Fig. 4 Yields of reducing sugar for different treatment time. | |
Initially, as the treatment time was increased, the total yield of reducing sugar was increased (Fig. 4). When the treatment time for potato starch was increased from 2 h to 6 h, the yield of reducing sugar was improved from 47.60% to 72.50% with the mixture of [PF6][(PYR)C4(PYR)][Cl] and [(PYR)C2COOH][PF6]. With the same ILs, as the treatment time for corn stalk was increased from 4 h to 8 h, the yield of reducing sugar was improved from 5.87% to 20.73%. However, the yields of reducing sugar were reduced as the treatment time was increased with the mixture of [PF6][(PYR)C4(MIM)][Cl] and [(MIM)C2COOH][PF6] from 8 h to 10 h for both potato starch and corn stalk.
Obviously, high yields of reducing sugar were obtained from potato starch and corn stalk with the mixture of dicationic ILs and monocationic acidic ILs. Monicationic ILs provided the acidic nature to assist the hydrolysis of potato starch and corn stalk. The basicity of one of the anion (Cl−) of dicationic ILs made it more efficient at disrupting inter- and intra-molecular hydrogen bonding in polymers. The other anion (PF6−) of dicationic ILs provided the hydrophobic and hydrophilic properties at different temperature. In current studies, all the ILs used for polymers-hydrolysis and biomass-treatment are hydrophilic, which increase the difficulty of the isolation of sugar and reuse of ILs. The variable miscibility of these functional ILs with water in this work provided the opportunity for hydrolysis of various carbohydrate polymers.
Reuse of ionic liquids
The feasibility and sustainable development of any catalytic process depends on the reusability of catalysts and solvents. Generally, after ILs-treatment of biomass, reducing sugar was readily extracted by the addition of water at a low temperature. However, a yield of monocationic acidic ILs would be lost in the aqueous layer. Further work will focus on the design of functional acidic ILs to increase their hydrophobic property. Alternatively, both of [PF6][(PYR)C4(MIM)][Cl] and [(MIM)C2COOH][PF6] could be simply extracted with acetonitrile, which were readily reused after the removal of acetonitrile. Otherwise, monocationic acidic [(PYR)C2COOH][PF6] or [(MIM)C2COOH][PF6] could be extracted with methanol. After the isolation of reducing sugar with water at room temperature, an amount of deionized water was added into the mixture with heating and stirring to provide [PF6][(PYR)C4(PYR)][Cl]. As shown in Fig. 5, high re-covery of ILs including [PF6][(PYR)C4(PYR)][Cl] and [(PYR)C2COOH][PF6] were obtained in the range of 80.20% to 88.37% for potato starch and 80.73% to 85.27% for corn stalk. Good recovery of ILs including [PF6][(PYR)C4(MIM)][Cl] and [(MIM)C2COOH][PF6] were achieved in the range of 81.45% to 94.49% for potato starch and 86.76% to 94.91% for corn stalk.
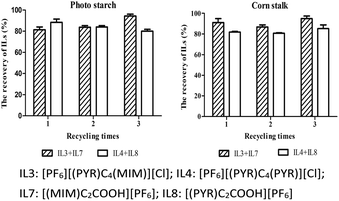 |
| Fig. 5 The recovery of ILs. | |
In this work, no significant reduced yields of reducing sugar was observed. The rates of reducing sugar for potato starch were 100% (1st run), 82.41% (2nd run) and 73.69% (3rd run) with the treatment of [PF6][(PYR)C4(PYR)][Cl] and [(PYR)C2COOH][PF6] and were 100% (1st run), 83.13% (2nd run) and 90.00% (3rd run) with the treatment of [PF6][(PYR)C4(MIM)][Cl] and [(MIM)C2COOH][PF6] (Fig. 6). Similarly, the rates of reducing sugar for corn stalk were 100% (1st run), 75.10% (2nd run) and 79.05% (3rd run) with the treatment of [PF6][(PYR)C4(PYR)][Cl] and [(PYR)C2COOH][PF6] and were 100% (1st run), 71.91% (2nd run) and 70.02% (3rd run) with the treatment of [PF6][(PYR)C4(MIM)][Cl] and [(MIM)C2COOH][PF6].
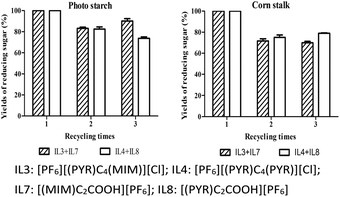 |
| Fig. 6 The rates of reducing sugar after three cycles. | |
Experimental
Materials
1-Methylimidazole (99%), pyridine (99%), 1,4-dichlorobutane (99%), 1,5-dichloropentane (99%), 3-chloropropionic acid (99%), potassium hexafluorophosphate (99%) were purchased from Aldrich Reagent Co., Ltd (Shanghai, China). All the reagents were used without further purification. Corn stalk was supplied by local farm in Harbin City (China), milled to pass through 40 mesh sieves and dried at 60 °C for 24 h. Potato starch was purchased from Tianjin Kaitong Chemical Reagent Co., Ltd (Tianjin, China).
Synthesis of ionic liquids
[1-(4-Chlorobutyl) pyridinium] hexafluorophosphate [(PYR)C4Cl][PF6]. Pyridine (1.0 mmol) and 1, 4-dichlorobutane (1.2 mmol) were stirred at 60 °C for 4 days. The resulting precipitate was filtered and washed with ethyl acetate, which was further purified by silica column chromatography (CH2Cl2/MeOH = 3/1) and was dried at 60 °C under vacuum for 4 h. After that, the precipitate (1.0 mmol) was reacted with KPF6 (1.5 mmol) in deionized water at room temperature for 3 h. The resulting product was washed with deionized water and was dried in a vacuum for 12 h. [1-(5-Chloropentyl) pyridinium] hexafluorophosphate [(PYR)C5Cl][PF6] was prepared using a similar method as [(PYR)C4Cl] [PF6].[(PYR)C4Cl][PF6]: 1H-NMR (400 MHz, DMSO-d6) (ppm): 9.34 (m, 2H), 8.63 (t, 1H), 8.19 (m, 2H), 4.77 (t, 2H), 3.66 (t, 2H), 2.03 (m, 2H), 1.71 (m, 2H).
[(PYR)C5Cl][PF6]: 1H-NMR (400 MHz, DMSO-d6) (ppm): 9.09 (d, 2H), 8.60 (t, 1H), 8.17 (t, 2H), 4.61 (t, 2H), 3.65 (t, 2H), 1.95 (quint, 2H), 1.76 (m, 2H), 1.39 (m, 2H).
Hexafluorophosphate [1-(1-pyridinium-yl-butyl)-4-methylimidazolium] chloride [PF6][([PYR)C4(MIM)][Cl]. [(PYR)C4Cl][PF6] (3.16 g) and 1-methylimidazolium (0.1 mmol) were added into 2 mL of ethyl acetate. The reaction proceeded at 60 °C for 24 h. The desired product was purified by recrystallize of acetonitrile/ethyl acetate (1/10) and then was dried in a vacuum at 60 °C for 4 h. Hexafluorophosphate [1-(1-pyridinium-yl-pentyl)-5-methylimidazolium] chloride [PF6][(PYR)C5(MIM)][Cl] was synthesized using a similar method as [PF6][(PYR)C4(MIM)][Cl].[PF6][(PYR)C4(MIM)][Cl]: 1H-NMR (400 MHz, DMSO-d6) (ppm): 9.42 (s, 1H), 9.26 (d, 2H), 8.60 (t, 1H), 8.16 (t, 2H), 7.84 (s, 1H), 7.74 (s, 1H), 4.74 (t, 2H), 4.27 (t, 2H), 3.85 (s, 3H), 1.92 (quint, 2H), 1.83 (quint, 2H).
[PF6][(PYR)C5(MIM)][Cl]: 1H-NMR (400 MHz, DMSO-d6) (ppm): 9.43 (s, 1H), 9.27 (d, 2H), 8.59 (m, 1H), 8.14 (m, 2H), 7.84 (s, 1H), 7.72 (s, 1H), 4.67(m, 2H), 4.19 (m, 2H), 3.85 (s, 3H), 1.96 (m, 2H), 1.83(m, 2H), 1.23(m, 2H).
Hexafluorophosphate [1-(1-pyridinium-yl-butyl)-4-pyridinium] chloride [PF6][(PYR)C4(PYR)][Cl]. [(PYR)C4Cl] [PF6] (0.1 mmol) was reacted with pyridinium (0.12 mmol) at 80 °C for 24 h. Following that, the product was recrystallized with acetonitrile/ethyl acetate (1/10) and dried in a vacuum at 60 °C for 4 h. Hexafluorophosphate [1-(1-pyridinium-yl-pentyl)-5-pyridinium] chloride ([PF6][(PYR)C5(PYR)][Cl]) was prepared using a similar method as [PF6][(PYR)C4(PYR)][Cl].[PF6][(PYR)C4(PYR)][Cl]: 1H-NMR (400 MHz, DMSO-d6) (ppm): 9.19 (m, 4H), 8.62 (t, 2H), 8.17 (t, 4H), 4.69 (t, 4H), 1.97 (quint, 4H).
[PF6][(PYR)C5(PYR)][Cl]: 1H-NMR (400 MHz, DMSO-d6) (ppm): 9.25 (d, 4H), 8.60 (t, 2H), 8.17 (t, 4H), 4.67 (t, 4H), 1.99 (quint, 4H), 1.31 (t, 2H).
[1-(2-Carboxylpropyl)-1-methylimidazolium] hexafluorophosphate [(MIM)C2COOH][PF6]. Methylimidazolium (0.4 mol) was dropped slowly into 3-chloropropionic acid (0.4 mol) in 200 mL of ethyl acetate. The mixture was stirred constantly at 70 °C for 24 h.47 The resulting product was washed with ethyl acetate (20 mL × 3) to give [(MIM)C2COOH][Cl]. [(MIM)C2COOH][Cl] (0.1 mmol) was reacted with hexafluorophosphate (0.1 mmol) in 30 mL of deionized water at room temperature for 3 h. The product was filtered and dried at 50 °C for 12 h to obtain [(MIM)C2COOH][PF6]. [1-(2-Carboxylpropyl)-pyridinium] hexafluorophosphate [(PYR)C2COOH][PF6] was synthesized using a similar method as [(MIM)C2COOH][PF6].[(MIM)C2COOH][PF6]: 1H-NMR (400 MHz, DMSO-d6) (ppm): 9.11 (s, 1H), 7.76 (s, 1H), 7.68 (s, 1H), 4.35 (t, 2H), 3.85 (s, 3H), 2.89 (t, 2H).
[(PYR)C2COOH][PF6]: 1H-NMR (400 MHz, DMSO-d6) (ppm): 9.14(d, 2H), 8.60(t, 1H), 8.15(t, 2H), 4.80(t, 2H), 3.10(t, 2H).
Thermal stability analysis
The thermal behavior of ionic liquids was investigated using a TG 209 F3 thermogravimetric analyzer (NETZSCH, Germany) by heating 10 mg of sample over temperature range from ambient to about 600 °C in nitrogen at a scanning rate of 10 °C min−1. The melting points were determined using a XPL-2 polarizing microscope melting point apparatus (NOVEL, China).48–51
Solubility of ionic liquids with organic solvents
The ionic liquid (0.1 g) was mixed with one of organic solvents (10 mL). The mixture was shaking strongly using magnetic stirrer for 30 min at room temperature. If the ionic liquid was insoluble in the organic solvent, which was added further until the ionic liquid was completely dissolved. The volumes of the organic solvent were recorded.
Miscibility of ionic liquids with water
The ionic liquid (1.0 g) was mixed with deionized water (1 mL). The mixture was stirred with 200 rpm for 30 min at different temperature. If the ionic liquid was immiscible with deionized water, the deionized water was added into the mixture further, which was repeated until it become a clear solution. The volumes of water were recorded.27
Treatment of corn stalk and potato starch
Corn stalk or potato starch (200 mg) was added into the mixture containing the dicationic ILs (3 g), monocationic acidic ILs (1 g) and deionized water (1.5 mL). The mixture was stirred at 100 °C for 6 h in a reaction kettle in an oil bath. After that, 15 mL of deionized water was added to the mixture when the reaction was finished. The supernatant by centrifugation was used for the determination of reducing sugar. The yield of reducing sugar was calculated as eqn (1): |
 | (1) |
Reuse of ionic liquids
Corn stalk or potato starch (200 mg) was treated with the mixture containing the dicationic ILs (3 g), monocationic acidic ILs (1 g) and deionized water (1.5 mL) at 100 °C for 6 h in a reaction kettle in an oil bath. After that, the mixture in a kettle was dried at 60 °C for 6 h. 20 mL of acetonitrile was added into the mixture. The filtrate was collected and acetonitrile was evaporated to give [(MIM)C2COOH][PF6] and [PF6][(PYR)C4(MIM)][Cl]. The reducing sugar was then obtained by the addition of deionized water (20 mL). Otherwise, 20 mL of methanol was added into the mixture. The filtrate was collected and methanol was evaporated to give [(PYR)C2COOH][PF6]. The reducing sugar was then obtained by the addition of deionized water (20 mL). Following that, 60 mL of deionized water was added into the filtered solid with stirring and heating at 100 °C. In practical application, when a small amount of water was evaporated, [PF6][(PYR)C4(PYR)][Cl] will precipitate out of the solution at a low temperature, which can be obtained through filtration. In order to obtain the accurate yield for reuse, all water was evaporated instead of the precipitation. All products were further dried in a vacuum at 60 °C for 6 h. The rate of reducing sugar was calculated as eqn (2): |
 | (2) |
Conclusions
Dicaionic and monocationic ILs with variable miscibility with water at different temperatures were synthesized. These functional ILs have important implications in the design of homogeneous and heterogeneous system with water and organic solvents, which will greatly benefit some specific applications. Particularly, the mixture of [PF6][(PYR)C4(PYR)][Cl] and [(PYR)C2COOH][PF6] played a crucial effect on the hydrolysis of carbohydrate polymers. Total reducing sugar was up to 72.50% for potato starch after 6 h and 20.73% for corn stalk after 8 h. respectively. The recovery of ILs were higher than 80% and the rates of reducing sugar were more than 70% after three cycles. The variable miscibility of these functional ILs with water provides the possibility for polymers-hydrolysis, biomass-treatment and other industrial specific utilizations.
Conflicts of interest
There are no conflicts to declare.
Acknowledgements
This work was financially supported by University Nursing Program for Young Scholars with Creative Talents in Heilongjiang Province (UNPYSCT-2017021).
Notes and references
- K. K. Laali and K. Kenneth, Synthesis, 2003, 11, 1752 CrossRef.
- P. L. Verma and S. P. Gejji, J. Mol. Graphics Modell., 2018, 85, 304–315 CrossRef CAS PubMed.
- K. J. Stevenson, J. Am. Chem. Soc., 2005, 127, 11878 CrossRef CAS.
- S. Letaief and C. Detellier, J. Mater. Chem., 2007, 17, 1476–1484 RSC.
- F. Guo, S. Zhang, J. Wang, B. Teng, T. Zhang and M. Fan, Curr. Org. Chem., 2015, 19, 455–468 CrossRef CAS.
- Z. S. Qureshi, K. M. Deshmukh and B. M. Bhanage, Clean Technol. Environ. Policy, 2013, 16(8), 1487–1513 CrossRef.
- H. T. Xu, C. H. Zhang, G. Chen, R. P. Shen and A. G. Ying, Chin. J. Chem., 2016, 36, 2353 CrossRef CAS.
- C. Yue, D. Fang, L. Liu and T. F. Yi, J. Mol. Liq., 2011, 163, 99–121 CrossRef CAS.
- A. S. Khan, Z. Man, A. Arvina, M. A. Bustam, A. Nasrullah and Z. Ullah, J. Mol. Liq., 2017, 227, 98–105 CrossRef CAS.
- M. G. Montalbán, G. Víllora and P. Licence, Data in Brief, 2018, 19, 769–788 CrossRef PubMed.
- T. Payagala, J. Huang, Z. S. Breitbach, P. S. Sharma and D. W. Armstrong, Chem. Mater., 2007, 19, 5848–5850 CrossRef CAS.
- Z. Zeng, B. S. Phillips, J. C. Xiao and J. M. Shreeve, Chem. Mater., 2008, 20, 2719–2726 CrossRef CAS.
- S. B. Aher and P. R. Bhagat, Res. Chem. Intermed., 2016, 42, 5587–5596 CrossRef CAS.
- R. Kore and R. Srivastava, J. Mol. Catal. A: Chem., 2003, 345, 117–126 CrossRef.
- U. Domańska, E. V. Lukoshko and M. Królikowski, Chem. Eng. J., 2012, 183, 261–270 CrossRef.
- V. Misuk, D. Breuch and H. Löwe, Chem. Eng. J., 2011, 173, 536–540 CrossRef CAS.
- S. A. Shamsi and N. D. Danielson, J. Sep. Sci., 2007, 30, 1729–1750 CrossRef CAS PubMed.
- A. M. Da Costa Lopes, K. G. João, A. R. C. Morais, E. Bogel-Łukasik and R. Bogel-Łukasik, Sustainable Chem. Processes, 2013, 1, 3 CrossRef.
- A. P. Dadi, C. A. Schall and S. Varanasi, Appl. Biochem. Biotechnol., 2007, 137–140, 407–421 CrossRef CAS PubMed.
- F. L. Wang, S. Li, Y. X. Sun, H. Y. Han, B. X. Zhang and B. Z. Hu, RSC Adv., 2017, 7, 47990–47998 RSC.
- J. P. Hallett and T. Welton, Chem. Rev., 2011, 111, 3508–3576 CrossRef CAS PubMed.
- S. H. Lee, T. V. Doherty, R. J. Linhardt and J. S. Dordick, Biotechnol. Bioeng., 2009, 102, 1368–1376 CrossRef CAS PubMed.
- H. T. Tan and K. T. Lee, Chem. Eng. J., 2012, 183, 448–458 CrossRef CAS.
- Z. C. Zhang, Adv. Catal., 2006, 49, 153–237 CAS.
- H. Zhao, Chem. Eng. Commun., 2006, 193, 1660–1677 CrossRef CAS.
- N. I. Haykir, E. Bahcegul, N. Bicak and U. Bakir, Ind. Crops Prod., 2013, 41, 430–436 CrossRef CAS.
- Y. X. Sun, Y. Y. Wang, B. B. Shen, B. X. Zhang and X. M. Hu, R. Soc. Open Sci., 2018, 5, 181230 CrossRef CAS PubMed.
- Y. Dong, J. Holm, J. Karkkainen, J. Nowicki and U. Lassi, Biomass Bioenergy, 2014, 70, 461–467 CrossRef CAS.
- A. S. Amarasekara and P. Shanbhag, BioEnergy Res., 2012, 6, 719–724 CrossRef.
- Y. X. An, M. H. Zong, H. Wu and N. Li, Bioresour. Technol., 2015, 192, 165–171 CrossRef CAS PubMed.
- L. Bai, X. L. Wang, Y. Nie, H. F. Dong and X. P. Zhang, Sci. China Chem., 2013, 56, 1811–1816 CrossRef CAS.
- A. H. Jadhav and H. Kim, Chem. Eng. J., 2012, 200–202, 264–274 CrossRef CAS.
- M. Talebi, R. A. Patil and D. W. Armstrong, J. Mol. Liq., 2018, 256, 247–255 CrossRef CAS.
- J. F. Vélez, L. V. Álvarez, C. del Río, B. Herradón, E. Mann and E. Morales, Electrochim. Acta, 2017, 241, 517–525 CrossRef.
- H. Zhang, M. Li and B. Yang, J. Phys. Chem. C, 2018, 122, 2467–2474 CrossRef CAS.
- B. S. Wang, L. Qin, T. C. Mu, Z. M. Xue and G. H. Gao, Chem. Rev., 2017, 10, 7113–7313 CrossRef PubMed.
- J. C. Chang, W. Y. Ho, I. W. Sun, Y. L. Tung, M. C. Tsui, T. Y. Wu and S. S. Liang, Tetrahedron, 2010, 66, 6150–6155 CrossRef CAS.
- Z. M. Xue, L. Qin, J. Y. Jiang, T. C. Mu and G. H. Gao, Phys. Chem. Chem. Phys., 2018, 20, 8382–8402 RSC.
- A. Chinnappan and H. Kim, Chem. Eng. J., 2012, 187, 283–288 CrossRef CAS.
- Y. S. Ding, M. Zha, J. Zhang and S. S. Wang, Colloids Surf., A, 2007, 298, 201–205 CrossRef CAS.
- Y. Chen, Y. Y. Cao, X. F. Sun and T. C. Mu, J. Mol. Liq., 2014, 190, 151–158 CrossRef CAS.
- Y. Y. Cao, Y. Chen, X. F. Sun, Z. M. Zhang and T. C. Mu, Phys. Chem. Chem. Phys., 2012, 14, 12252–12262 RSC.
- A. Hernoux-Villière, J. M. Lévêque, J. Kärkkäinen, N. Papaiconomou, M. Lajunen and U. Lassi, Catal. Today, 2014, 223, 11–17 CrossRef.
- K. R. Seddon, A. Stark, M. J. Torres and T. María-José, Pure Appl. Chem., 2000, 72, 2275–2287 CAS.
- C. Z. Li, Q. Wang and Z. B. K. Zhao, Green Chem., 2008, 2, 177–182 RSC.
- H. H. Ma, B. X. Zhang, P. Zhang, S. Li, Y. F. Gao and X. M. Hu, Fuel Process. Technol., 2016, 148, 138–145 CrossRef CAS.
- P. Zhang, S. J. Dong, H. H. Ma, B. X. Zhang, Y. F. Wang and X. M. Hu, Ind. Crops Prod., 2015, 76, 688–696 CrossRef CAS.
- J. L. Anderson, R. Ding, A. Ellern and D. W. Armstrong, J. Am. Chem. Soc., 2005, 127, 593–604 CrossRef CAS PubMed.
- M. Moniruzzaman and T. Ono, Bioresour. Technol., 2013, 127, 132–137 CrossRef CAS PubMed.
- J. Salgado, M. Villanueva, J. J. Parajó and J. Fernández, J. Chem. Thermodyn., 2013, 65, 184–190 CrossRef CAS.
- G. Yu, S. Yan, F. Zhou, X. Liu, W. Liu and Y. Liang, Tribol. Lett., 2006, 25, 197–205 CrossRef.
|
This journal is © The Royal Society of Chemistry 2019 |
Click here to see how this site uses Cookies. View our privacy policy here.