DOI:
10.1039/C9RA06108H
(Paper)
RSC Adv., 2019,
9, 32081-32084
Palladium-catalyzed oxidative cross-coupling for the synthesis of α-amino ketones†
Received
6th August 2019
, Accepted 18th September 2019
First published on 9th October 2019
Abstract
A novel oxidative cross-coupling reaction for the synthesis of α-aryl α-amino ketones in the presence of palladium catalysts using T+BF4− as an oxidant has been developed. This transformation was achieved by direct C–H oxidation of α-aminocarbonyl compounds and arylation. The mild reaction has a broad reaction scope and gives desired α-aryl α-amino ketones in moderate to excellent yields.
Transition metal-catalyzed oxidative coupling reactions involving the formation of C–C bonds from C–H bonds have attracted considerable attention, indicating excellent atom economy and environmental friendliness.1 α-Amino carbonyl compounds have important roles in natural products and are the key structural units of natural products.2 These compounds have also been used in organic chemistry to synthesize biological activites, therapeutic agents, quinazolines, imidazoles, pyrazines, indoles, and pyrroles.3 Therefore, the direct oxidative C–H functionalization has gained significant attention for the synthesis of a series of α-amino carbonyl compounds.2i,2j,4 For example, Li's group employed an oxidative coupling reaction to synthesize α-amino carbonyl compounds from N-glycine derivatives by direct C–C bond formation under the catalysis of copper salts.5 Subsequently, stoichiometric amounts of chemical oxidants, such as DTBP, DDQ, TBHP, and 2,2,6,6-tetramethylpiperidine-1-oxoammonium tetra-fluoroborate (T+BF4−), have been applied to these reactions.4a,4d,4p,4t,6 In 2013, Yang's group described a novel protocol for a copper-catalyzed oxidative phosphonation reaction by using α-aminocarbonyls and diphenylphosphine ((1), Scheme 1).7 Huang's group disclosed a general and efficient method for C–N oxidative cross-coupling through direct Csp3–H bond functionalization of α-aminocarbonyl compounds with amines under the catalysis of copper salts ((2), Scheme 1).6h In 2015, Yang's group developed a highly efficient route to synthetize chiral arylglycine derivatives via a palladium-catalyzed enantioselective direct C–H oxidation arylation reaction ((3), Scheme 1).4p Furthermore, transition metal-catalyzed direct C–H functionalization by an oxidative cross-coupling reaction has been reported in the past few years.8 Although significant advances have been made along these lines, the development of efficient synthetic methodologies for the synthesis of α-aminocarbonyl compounds via palladium-catalyzed oxidative cross-coupling still remains a challenging topic. Based on these considerable progresses, in this paper, we describe a highly efficient C–H oxidative cross-coupling reaction strategy for the synthesis of α-amino ketone compounds by palladium-catalyzed direct C–H oxidation and arylation reactions ((4), Scheme 1).
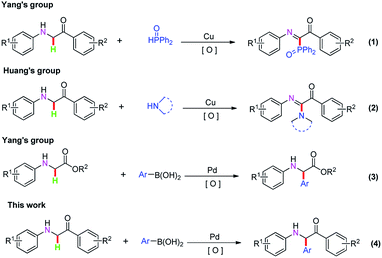 |
| Scheme 1 Transition metal-catalyzed reaction for the synthesis of α-aminocarbonyl compounds. | |
In an initial study, we chose 2-((4-chlorophenyl)amino)-1-phenylethanone 1a and para-methylphenyl boric acid as the model substrate to evaluate different oxidants in the presence of 10 mol% Pd(OAc)2 with 2,2-bipyridine as a ligand in TFE at 60 °C (Table 1, entries 1–8). To our delight, the desired product 2a was obtained in 14% yield by using 2,2,6,6-tetramethylpiperidine-1-oxoammonium tetra-fluoroborate (T+BF4−)4p as an oxidant (Table 1, entry 8). Based on these results, various ligands were used to carry out the reaction in the presence of 10 mol% Pd(OAc)2. As expected, the best result of 29% yield was obtained by employing L3 as a ligand (Table 1, entries 9–15). Then, different solvents were screened; using CH3OH as the solvent with the set reaction conditions gave comparable results (entry 25), but others gave lower yields (Table 1, entries 16–25). When the temperature was increased to 80 °C, the yield of 2a reached 71% (Table 1, entries 26 and 27). To our delight, the reaction could occur in the presence of 10 mol% of catalysts such as Pd(NO3)2, Pd(TFA)2, PdCl2, Pd(PPh3)2Cl2, Pd(PPh3)4, Pd(CH3CN)2Cl2, and Pd(acac)2, while the reactivity of Pd(PCy3)2Cl2 was better than others, affording the desired product 2a in 86% yield (Table 1, entries 28–37). Furthermore, control experiments showed that no or trace amounts of the desired product was obtained in the absence of Pd(PCy3)2Cl2 or T+BF4− (Table 1, entries 38 and 39).
Table 1 Optimization of the reaction conditionsa,b
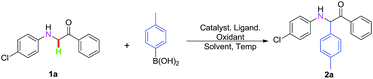
|
Entry |
Catalyst |
Ligand |
Oxidant |
Solvent |
Yieldb |
Reaction conditions: 1a (0.1 mmol), para-methyphenyl boric acid (1.2 equiv.), catalyst (10 mol%), ligand (10 mol%) and oxidant (1.2 equiv.) was stirred in solvent (1 mL) at 60 °C under Ar for 20 h.
Yield of the isolated product.
100 °C.
80 °C.
|
1 |
Pd(OAc)2 |
bpy |
BQ |
TFE |
10% |
2 |
Pd(OAc)2 |
bpy |
Ag2CO3 |
TFE |
13% |
3 |
Pd(OAc)2 |
bpy |
K2S2O8 |
TFE |
Trace |
4 |
Pd(OAc)2 |
bpy |
Air |
TFE |
8% |
5 |
Pd(OAc)2 |
bpy |
PhI(OAc)2 |
TFE |
Trace |
6 |
Pd(OAc)2 |
bpy |
Ph3CBF4 |
TFE |
NR |
7 |
Pd(OAc)2 |
bpy |
C7H7BF4 |
TFE |
Trace |
8 |
Pd(OAc)2 |
bpy |
T+BF4− |
TFE |
14% |
9 |
Pd(OAc)2 |
L1
|
T+BF4− |
TFE |
24% |
10 |
Pd(OAc)2 |
L2
|
T+BF4− |
TFE |
13% |
11 |
Pd(OAc)2 |
L3
|
T+BF4− |
TFE |
29% |
12 |
Pd(OAc)2 |
L4
|
T+BF4− |
TFE |
15% |
13 |
Pd(OAc)2 |
L5
|
T+BF4− |
TFE |
20% |
14 |
Pd(OAc)2 |
L6
|
T+BF4− |
TFE |
12% |
15 |
Pd(OAc)2 |
PPh3 |
T+BF4− |
TFE |
16% |
16 |
Pd(OAc)2 |
L3
|
T+BF4− |
DCE |
21% |
17 |
Pd(OAc)2 |
L3
|
T+BF4− |
THF |
31% |
18 |
Pd(OAc)2 |
L3
|
T+BF4− |
TOL |
Trace |
19 |
Pd(OAc)2 |
L3
|
T+BF4− |
CH3NO2 |
16 |
20 |
Pd(OAc)2 |
L3
|
T+BF4− |
DCM |
18% |
21 |
Pd(OAc)2 |
L3
|
T+BF4− |
C2H5OH |
37% |
22 |
Pd(OAc)2 |
L3
|
T+BF4− |
DME |
40% |
23 |
Pd(OAc)2 |
L3
|
T+BF4− |
DMF |
8% |
24 |
Pd(OAc)2 |
L3
|
T+BF4− |
Dioxane |
32% |
25 |
Pd(OAc)2 |
L3
|
T+BF4− |
CH3OH |
47% |
26c |
Pd(OAc)2 |
L3
|
T+BF4− |
CH3OH |
23% |
27d |
Pd(OAc)2 |
L3
|
T+BF4− |
CH3OH |
71% |
28d |
Pd(NO3)2 |
L3
|
T+BF4− |
CH3OH |
56% |
29d |
Pd(TFA)2 |
L3
|
T+BF4− |
CH3OH |
43% |
30d |
PdCl2 |
L3
|
T+BF4− |
CH3OH |
67% |
31d |
Pd(PPh3)2Cl2 |
|
T+BF4− |
CH3OH |
60% |
32d |
Pd(PPh3)4 |
|
T+BF4− |
CH3OH |
38% |
33d |
Pd(CH3CN)2Cl2 |
|
T+BF4− |
CH3OH |
74% |
34d |
Pd(acac)2 |
|
T+BF4− |
CH3OH |
58% |
35d |
Pd(PhCN)2Cl2 |
|
T+BF4− |
CH3OH |
63% |
36d |
Pd(cod)Cl2 |
|
T+BF4− |
CH3OH |
66% |
37
d
|
Pd(PCy
3
)
2
Cl
2
|
|
T
+
BF
4
−
|
CH
3
OH
|
86%
|
38d |
Pd(PCy3)2Cl2 |
|
|
CH3OH |
Trace |
39d |
|
|
T+BF4− |
CH3OH |
No |
|
With the optimal reaction conditions in hand (Table 1, entry 37), we explored the C–H oxidative cross-coupling reaction of 2-((4-chlorophenyl)amino)-1-phenylethanone 1a with arylboric acids, as shown in Table 2. We first surveyed different substituents of arylboric acids with electron-donating groups, such as methyl, ethyl, isopropyl and methoxy, and found that they gave the desired product in 80–86% yields (Table 2, entries 2a–2d). Meanwhile, the steric effect was examined using the meta- and ortho-methyl phenylboric acids under identical conditions (Table 2, entries 2e and 2f). However, the steric effect in this transformation was very significant; only trace amounts of the product was obtained when ortho-methyl phenylboric acids were introduced for the optimization of reaction conditions (Table 2, entry 2f). When arylboric acids with different electron-donating or electron-withdrawing groups afforded the desired products in excellent to moderate yields (Table 2, entries 2g–2m). Moreover, in order to further expand the substrate scope, we selected potassium phenyltrifluoroborate as the arylated reagent under the optimized reaction conditions; the corresponding α-alkylation product 2i was obtained in 45% yield (Table 2, entry 2i).
Table 2 Reaction conditions screeninga,b
Reaction conditions: 1a (0.1 mmol), para-methyphenyl boric acid (1.2 equiv.), Pd(PCy3)2Cl2 (10 mol%), and 2,2,6,6-tetramethylpiperidine-1-oxoammonium tetra-fluoroborate (T+BF4−) (1.2 equiv.) was stirred in CH3OH (1 mL) at 80 °C under Ar for 20 h.
Yield of the isolated product.
Potassium phenyltrifluoroborate as arylated reagents.
|
|
Furthermore, the naphthalen-1-ylboronic acid and benzo[1,3]dioxol-5-ylboronic acid could also afford α-aminocarbonyl compounds 2n and 2o in 73–77% yields (Table 2, entries 2n and 2o). Of particular note is the heterocyclic boronic acid, which was also compatible for the reaction (Table 2, entries 2p and 2q). Moreover, the introduction of various electron-withdrawing or electron-donating substituents on the aniline moeity gave the corresponding α-aminocarbonyl compounds in 30–88% yields (Table 2, entries 2r–2x); the electronic effect and the steric effect in this transformation was very notable (Table 2, entries 2t–2v). Next, different substituent groups of α-carbonyl compounds bearing different functional groups were additionally examined and the corresponding products were generated in moderate yields (Table 2, entries 2y and 2z).
To investigate the mechanism of this transformation, experiments were carried out. The desired product was obtained in the range of 86% to 65% and 86% to 45% yield when 2.0 equivalents of radical-trapping reagents 2,2,6,6-tetramethyl-1-piperidinyloxy (TEMPO) and 2,6-di-tert-butyl-4-methylphenol (BHT) were used, respectively, under standardized reaction conditions (Scheme 2). To our delight, the key α-imino intermediate A was detected by GC-MS (see ESI†). Based on the observed experimental results and pioneering reports,4p,9 we have described a plausible mechanistic pathway in Scheme 3. Initially, the arylpalladium intermediate B was produced via a transmetallation reaction of Pd(PCy3)2Cl2 with aryl boric acid, which attacks the α-imino intermediate A obtained by the in situ oxidation of 1a by T+BF4− to form the complex C. Then, an aryl group was added to the imine to generate intermediate D. Finally, the product 2a was obtained upon dissociation in the presence of H+. At the same time, the palladium catalyst was regenerated and synchronized into the next catalytic cycle (Scheme 3).
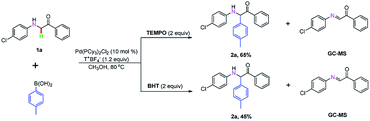 |
| Scheme 2 Radical-trapping experiment. | |
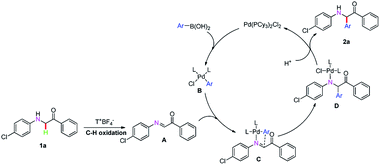 |
| Scheme 3 Proposed mechanism. | |
In summary, we have achieved a novel pattern for the synthesis of α-aryl α-amino ketone compounds via Pd(II)-catalyzed oxidative coupling of α-aminocarbonyl compounds with arylboric acids. This reaction occurs via direct C–H oxidation and arylation reactions. The coupling of α-aminocarbonyl compounds gave functionalized α-aryl α-amino ketone compounds in moderate to excellent yields.
Conflicts of interest
There are no conflicts to declare.
Acknowledgements
This work was financially supported by the National Natural Science Foundation of China (No. 21762038 and 21962016), the Fundamental Research Funds for the Central Universities (31920190077, 31920180040 and 31920190015), the Scientific Research Foundation of Northwest University for Nationalities (xbmuyjrc201603).
Notes and references
-
(a) S. H. Cho, J. Y. Kim, J. Kwak and S. Chang, Chem. Soc. Rev., 2011, 40, 5068–5083 RSC;
(b) S. A. Girard, T. Knauber and C.-J. Li, Angew. Chem., Int. Ed., 2014, 53, 74–100 CrossRef CAS;
(c) C.-J. Li, Acc. Chem. Res., 2009, 42, 335–344 CrossRef CAS;
(d) C.-J. Li and Z. Li, Pure Appl. Chem., 2006, 78, 935 CAS;
(e) C. J. Scheuermann, Chem.–Asian J., 2010, 5, 436–451 CrossRef CAS PubMed;
(f) C. S. Yeung and V. M. Dong, Chem. Rev., 2011, 111, 1215–1292 CrossRef CAS;
(g) A. H. Cherney, N. T. Kadunce and S. E. Reisman, Chem. Rev., 2015, 115, 9587–9652 CrossRef CAS;
(h) C. G. Newton, S.-G. Wang, C. C. Oliveira and N. Cramer, Chem. Rev., 2017, 117, 8908–8976 CrossRef CAS.
-
(a) G. Choudhary and R. K. Peddinti, Green Chem., 2011, 13, 276 RSC;
(b) M. B. Gawande, V. D. Bonifácio, R. Luque, P. S. Branco and R. S. Varma, Chem. Soc. Rev., 2013, 42, 5522 RSC;
(c) S. L. Jain, S. Singhal and B. Sain, Green Chem., 2007, 9, 740 RSC;
(d) G. L. Khatik, R. Kumar and A. K. Chakraborti, Org. Lett., 2006, 8, 2433 CrossRef CAS;
(e) M. V. Reddy, B. S. Kumar, K. T. Lim, B. G. Cho and Y. T. Jeong, Tetrahedron Lett., 2016, 57, 476 CrossRef;
(f) R. S. Varma, Green Chem., 1999, 1, 43 RSC;
(g) G. Zhang, Y. Zhang and R. Wang, Angew. Chem., Int. Ed., 2011, 50, 10429–10432 CrossRef CAS;
(h) J. Zhang, Z. Cui, F. Wang, Y. Wang, Z. Miao and R. Chen, Green Chem., 2007, 9, 1341 RSC;
(i) L. Zhao and C.-J. Li, Angew. Chem., Int. Ed., 2008, 47, 7075–7078 CrossRef CAS;
(j) Z.-Q. Zhu, P. Bai and Z.-Z. Huang, Org. Lett., 2014, 16, 4881–4883 CrossRef CAS.
-
(a) A. Bodtke and P. Langer, Tetrahedron Lett., 2004, 45, 8741 CrossRef CAS;
(b) L. Bryan, A. Richard, K. F. Buzon, T. Chiu, M. L. Colgan and N. Kasthurikrishnan, Tetrahedron Lett., 2004, 45, 6887 CrossRef;
(c) V. Chandrashaker, M. Taniguchi, M. Ptaszek and J. S. Lindsey, Tetrahedron, 2012, 68, 6957 CrossRef CAS;
(d) B. P. Medaer and G. J. Hoornaert, Tetrahedron, 1999, 55, 3987 CrossRef CAS;
(e) A. D. Murkute, J. E. Jackson and D. J. Miller, J. Catal., 2011, 278, 189–199 CrossRef CAS;
(f) S. H. Gellman, Acc. Chem. Res., 1998, 31, 173–180 CrossRef CAS;
(g) M. C. Jose and R.-S. Humberto, Curr. Org. Chem., 2008, 12, 524–543 CrossRef;
(h) F. D. Klingler, Acc. Chem. Res., 2007, 40, 1367–1376 CrossRef CAS;
(i) Y. Ohfune, Acc. Chem. Res., 1992, 25, 360–366 CrossRef CAS;
(j) M. Farahi, F. Tamaddon, B. Karami and S. Pasdar, Tetrahedron Lett., 2015, 56, 1887–1890 CrossRef CAS;
(k) F. Tamaddon and A. Dehghani Tafti, Synlett, 2016, 27, 2217–2220 CrossRef CAS.
-
(a) J. Xie and Z.-Z. Huang, Angew. Chem., Int. Ed., 2010, 49, 10181–10185 CrossRef CAS;
(b) J. S. Bandar and T. H. Lambert, J. Am. Chem. Soc., 2013, 135, 11799–11802 CrossRef CAS;
(c) J. T. Binder, C. J. Cordier and G. C. Fu, J. Am. Chem. Soc., 2012, 134, 17003–17006 CrossRef CAS;
(d) C. Huo, C. Wang, M. Wu, X. Jia, H. Xie and Y. Yuan, Adv. Synth. Catal., 2014, 356, 411–415 CrossRef CAS;
(e) C. Huo, M. Wu, F. Chen, X. Jia, Y. Yuan and H. Xie, Chem. Commun., 2015, 51, 4708–4711 RSC;
(f) C. Huo, H. Xie, F. Chen, J. Tang and Y. Wang, Adv. Synth. Catal., 2016, 358, 724–730 CrossRef CAS;
(g) S. Kotha, Acc. Chem. Res., 2003, 36, 342–351 CrossRef CAS;
(h) C. Nájera and J. M. Sansano, Chem. Rev., 2007, 107, 4584–4671 CrossRef;
(i) S. Saito, T. Tsubogo and S. Kobayashi, J. Am. Chem. Soc., 2007, 129, 5364–5365 CrossRef CAS;
(j) M. Salman, Z.-Q. Zhu and Z.-Z. Huang, Org. Lett., 2016, 18, 1526–1529 CrossRef CAS;
(k) B. M. Trost and F. Miege, J. Am. Chem. Soc., 2014, 136, 3016–3019 CrossRef CAS;
(l) M. Wan, H. Lou and L. Liu, Chem. Commun., 2015, 51, 13953–13956 RSC;
(m) E. W. Werner, T.-S. Mei, A. J. Burckle and M. S. Sigman, Science, 2012, 338, 1455–1458 CrossRef CAS;
(n) Z.-Y. Xue, Q.-H. Li, H.-Y. Tao and C.-J. Wang, J. Am. Chem. Soc., 2011, 133, 11757–11765 CrossRef CAS;
(o) Y. Hui, X. Yilan, D. Rui and F. Yan, Adv. Synth. Catal., 2017, 359, 39–43 CrossRef;
(p) X.-H. Wei, G.-W. Wang and S.-D. Yang, Chem. Commun., 2015, 51, 832–835 RSC;
(q) X.-H. Wei, L.-B. Zhao and H.-C. Zhou, RSC Adv., 2017, 7, 16561–16564 RSC;
(r) Y. Wei, J. Wang, Y. Wang, X. Yao, C. Yang and C. Huo, Org. Biomol. Chem., 2018, 16, 4985–4989 RSC;
(s) Y. Zhang, X. Yang, H. Zhou, S. Li, Y. Zhu and Y. Li, Org. Chem. Front., 2018, 5, 2120–2125 RSC;
(t) R. V. Daggupati and C. Malapaka, Org. Chem. Front., 2018, 5, 788–792 RSC;
(u) L. Revathi, L. Ravindar, W.-Y. Fang, K. P. Rakesh and H.-L. Qin, Adv. Synth. Catal., 2018, 360, 4652–4698 CrossRef CAS;
(v) X.-H. Wei, L.-B. Zhao and H.-C. Zhou, RSC Adv., 2017, 7, 16561–16564 RSC;
(w) G.-F. Zha, G. A. L. Bare, J. Leng, Z.-P. Shang, Z. Luo and H.-L. Qin, Adv. Synth. Catal., 2017, 359, 3237–3242 CrossRef CAS;
(x) X. Zhang, K. P. Rakesh, L. Ravindar and H.-L. Qin, Green Chem., 2018, 20, 4790–4833 RSC;
(y) C. Zhao, G.-F. Zha, W.-Y. Fang, K. P. Rakesh and H.-L. Qin, Eur. J. Org. Chem., 2019, 2019, 1801–1807 CrossRef CAS.
-
(a) J.-C. Wu, R.-J. Song, Z.-Q. Wang, X.-C. Huang, Y.-X. Xie and J.-H. Li, Angew. Chem., Int. Ed., 2012, 51, 3453–3457 CrossRef CAS;
(b) W.-T. Wei, R.-J. Song and J.-H. Li, Adv. Synth. Catal., 2014, 356, 1703–1707 CrossRef CAS.
-
(a) P. Liu, Y. Li, H. Wang, Z. Wang and X. Hu, Tetrahedron Lett., 2012, 53, 6654–6656 CrossRef CAS;
(b) P. Liu, Z. Wang, J. Lin and X. Hu, Eur. J. Org. Chem., 2012, 2012, 1583–1589 CrossRef CAS;
(c) H. Richter and O. García Mancheño, Org. Lett., 2011, 13, 6066–6069 CrossRef CAS;
(d) R. Rohlmann, T. Stopka, H. Richter and O. García Mancheño, J. Org. Chem., 2013, 78, 6050–6064 CrossRef CAS;
(e) Z.-Q. Wang, M. Hu, X.-C. Huang, L.-B. Gong, Y.-X. Xie and J.-H. Li, J. Org. Chem., 2012, 77, 8705–8711 CrossRef CAS;
(f) W.-T. Wei, R.-J. Song and J.-H. Li, Adv. Synth. Catal., 2014, 356, 1703–1707 CrossRef CAS;
(g) L. Zhao, O. Baslé and C.-J. Li, Proc. Natl. Acad. Sci. U. S. A., 2009, 106, 4106–4111 CrossRef CAS;
(h) X.-X. Liu, Z.-Y. Wu, Y.-Q. He, X.-Q. Zhou, T. Hu, C.-W. Ma and G.-S. Huang, Adv. Synth. Catal., 2016, 358, 2385–2391 CrossRef CAS;
(i) Z. Xie, J. Jia, X. Liu and L. Liu, Adv. Synth. Catal., 2016, 358, 919–925 CrossRef CAS.
- B. Yang, T.-T. Yang, X.-A. Li, J.-J. Wang and S.-D. Yang, Org. Lett., 2013, 15, 5024–5027 CrossRef CAS.
-
(a) M.-X. Cheng, R.-S. Ma, Q. Yang and S.-D. Yang, Org. Lett., 2016, 18, 3262–3265 CrossRef CAS;
(b) Y.-Y. Huang, C. Cai, X. Yang, Z.-C. Lv and U. Schneider, ACS Catal., 2016, 6, 5747–5763 CrossRef CAS;
(c) Z. Meng, S. Sun, H. Yuan, H. Lou and L. Liu, Angew. Chem., Int. Ed., 2014, 53, 543–547 CrossRef CAS;
(d) S. Stockerl and O. G. Mancheno, Org. Chem. Front., 2016, 3, 277–280 RSC;
(e) S. Sun, C. Li, P. E. Floreancig, H. Lou and L. Liu, Org. Lett., 2015, 17, 1684–1687 CrossRef CAS;
(f) S. Sun, Y. Mao, H. Lou and L. Liu, Chem. Commun., 2015, 51, 10691–10694 RSC;
(g) Z. Xie, X. Zan, S. Sun, X. Pan and L. Liu, Org. Lett., 2016, 18, 3944–3947 CrossRef CAS;
(h) J. Chen, X. Lu, W. Lou, Y. Ye, H. Jiang and W. Zeng, J. Org. Chem., 2012, 77, 8541–8548 CrossRef CAS;
(i) A. Córdova, Acc. Chem. Res., 2004, 37, 102–112 CrossRef;
(j) N. Gommermann, C. Koradin, K. Polborn and P. Knochel, Angew. Chem., Int. Ed., 2003, 42, 5763–5766 CrossRef CAS;
(k) M. Hatano, T. Horibe and K. Ishihara, J. Am. Chem. Soc., 2009, 132, 56–57 CrossRef;
(l) K. R. Knudsen, T. Risgaard, N. Nishiwaki, K. V. Gothelf and K. A. Jørgensen, J. Am. Chem. Soc., 2001, 123, 5843–5844 CrossRef CAS PubMed;
(m) S. Kobayashi, T. Hamada and K. Manabe, J. Am. Chem. Soc., 2002, 124, 5640–5641 CrossRef CAS PubMed;
(n) S. Kobayashi, Y. Mori, J. S. Fossey and M. M. Salter, Chem. Rev., 2011, 111, 2626–2704 CrossRef CAS;
(o) C. Koradin, K. Polborn and P. Knochel, Angew. Chem., Int. Ed., 2002, 41, 2535–2538 CrossRef CAS;
(p) C. Qian, J. Chen, M. Fu, S. Zhu, W.-H. Chen, H. Jiang and W. Zeng, Org. Biomol. Chem., 2013, 11, 6013–6022 RSC;
(q) A. E. Taggi, A. M. Hafez and T. Lectka, Acc. Chem. Res., 2002, 36, 10–19 CrossRef;
(r) B. M. Trost and L. R. Terrell, J. Am. Chem. Soc., 2002, 125, 338–339 CrossRef;
(s) C. Wei and C.-J. Li, J. Am. Chem. Soc., 2002, 124, 5638–5639 CrossRef CAS;
(t) C. Wei, J. T. Mague and C.-J. Li, Proc. Natl. Acad. Sci. U. S. A., 2004, 101, 5749–5754 CrossRef CAS;
(u) C.-Y. Zhou, S.-F. Zhu, L.-X. Wang and Q.-L. Zhou, J. Am. Chem. Soc., 2010, 132, 10955–10957 CrossRef CAS.
-
(a) R. Bloch, Chem. Rev., 1998, 98, 1407–1438 CrossRef CAS;
(b) H. Dai and X. Lu, Org. Lett., 2007, 9, 3077–3080 CrossRef CAS;
(c) S. Kobayashi and H. Ishitani, Chem. Rev., 1999, 99, 1069–1094 CrossRef CAS.
Footnote |
† Electronic supplementary information (ESI) available. See DOI: 10.1039/c9ra06108h |
|
This journal is © The Royal Society of Chemistry 2019 |