DOI:
10.1039/C9RA05896F
(Paper)
RSC Adv., 2019,
9, 37344-37354
Co3O4/NiO@GQD@SO3H nanocomposite as a superior catalyst for the synthesis of chromenpyrimidines†
Received
30th July 2019
, Accepted 28th October 2019
First published on 15th November 2019
Abstract
A three-component reaction involving aromatic aldehydes, 6-amino-1,3-dimethyluracil and 4-hydroxycoumarin was achieved in the presence of the Co3O4/NiO@GQD@SO3H nanocomposite as a highly effective heterogeneous catalyst to produce chromenpyrimidines. The catalyst was characterized via FT-IR, SEM, XRD, EDS, TGA, BET and VSM. This new catalyst was demonstrated to be highly effective in the preparation of chromenpyrimidines. Atom economy, low catalyst loading, reusable catalyst, applicability to a wide range of substrates and high product yields are some of the important features of this protocol.
1. Introduction
Chromenpyrimidines are a common scaffold in multiple bioactive compounds and possess several pharmacological properties.1 These compounds are used as analgesic, anti-pyretic,2 anti-microbial,3,4 anti-biofilm,5 anti-inflammatory,6 anticancer,7 antitubercular agents.8 Some other examples of pyrimidines as prominent drug molecules include uramustine, piritrexim isetionate, tegafur, floxuridine, methotrexate, trimethoprim, piromidic acid, tetroxoprim and dipyridamole, which have high bioavailability, slow onset and prolonged effect.9 Chromenpyrimidines have been regarded as significant targets of organic synthesis. Therefore, the development of effective methods for the preparation of chromenpyrimidines is an attractive challenge. Several methods have been reported for the preparation of chromenpyrimidines in the presence of diverse catalysts including L-proline-derived secondary aminothiourea,10 sulfamic acid,11 Zr(HSO4)4,12 L-proline,13 and bifunctional thiourea-based organocatalyst.14 However, some of the reported procedures have disadvantages including long reaction times, use of toxic and non-reusable catalysts and undesirable reaction conditions. Therefore, to avoid these drawbacks, the search for effective methods for the preparation of chromenpyrimidines is still desirable. Nanoparticles exhibit good catalytic activity owing to their large surface area and active sites. Metal oxides are a broad class of materials that have been researched extensively due to their unique attributes and potential applications in various fields. Graphene quantum dots (GQDs) are a new member of the carbon nanostructure family, which have quasi-spherical structures. GQDs have gained intensive attention due to their significant features, biological,15 biomedical,16 and therapeutic applications,17 as a new class of photocatalysts18 and surfactants,19 and electrochemical biosensing,20 electrocatalytic,21 lithium battery,22 optical and photovoltaic,23 photoluminescence,24,25 bioimaging,26 and catalytic applications.27 The potential applications of N-graphene quantum dots were recently reviewed based on experimental and theoretical studies.28–31 The synthesis of highly efficient nanocomposite catalysts for the synthesis of organic compounds is still a big challenge. To obtain a larger surface area and more active sites, nanocatalysts are functionalized with active groups.32–34 It has been demonstrated that the decoration of nanocatalysts with GQDs prevents the aggregation of fine particles, and thus increases the effective surface area and number of reactive sites for efficient catalytic reactions. The chemical groups on GQD can catalyze chemical reactions, and their –COOH and –SO3H groups can serve as acid catalysts for many reactions.27–36 Herein, we report the use of a Co3O4/NiO@GQD@SO3H nanocomposite as a new efficient catalyst for the preparation of chromenpyrimidines through a three-component reaction involving aromatic aldehydes, 6-amino-1,3-dimethyluracil and 4-hydroxycoumarin (Scheme 1).
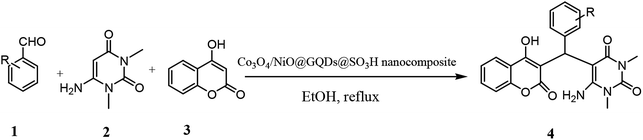 |
| Scheme 1 Synthesis of chromenpyrimidines using the Co3O4/NiO@GQD@SO3H nanocomposite. | |
2. Results and discussion
Initially, we prepared Co3O4/NiO nanoparticles via simple techniques. A facile hydrothermal method was used for the preparation of N-GQDs.37 Sulfonated graphene quantum dots were prepared using chlorosulfonic acid.38 The XRD patterns of Co3O4/NiO, Co3O4/NiO@N-GQDs and Co3O4/NiO@GQD@SO3H nanocomposite are shown in Fig. 1, which confirm the presence of both NiO (JCPDS No.22-1189) and Co3O4 (JCPDS no 65-3103).
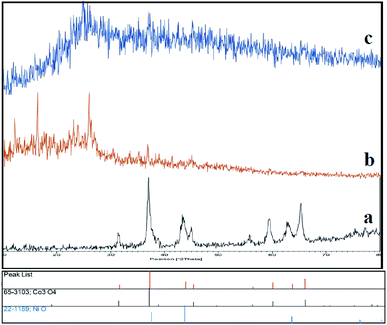 |
| Fig. 1 XRD patterns of (a) Co3O4/NiO, (b) Co3O4/NiO@GQDs and (c) Co3O4/NiO@GQD@SO3H. | |
To investigate the morphology and particle size of the nanoparticles, SEM images of Co3O4/NiO and Co3O4/NiO@ GQD@SO3H nanocomposite were measured, as shown in Fig. 2. The SEM images of the Co3O4/NiO@GQD@SO3H nanocomposite show the formation of uniform particles, and the energy-dispersive X-ray spectrum (EDS) confirms the presence of Co, Ni, O, S and C species in the structure of the nanocomposite (Fig. 3).
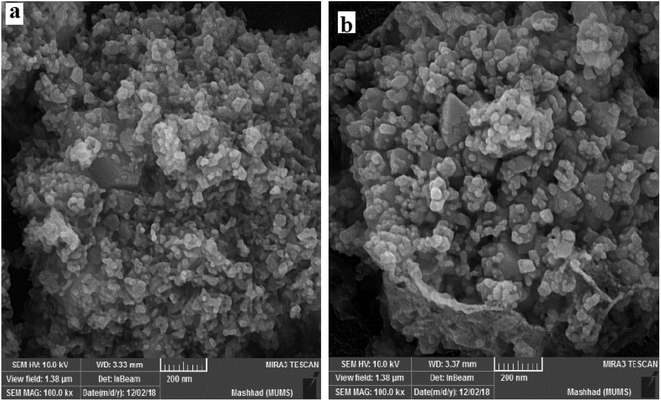 |
| Fig. 2 SEM images of (a) Co3O4/NiO and (b) Co3O4/NiO@GQD@SO3H. | |
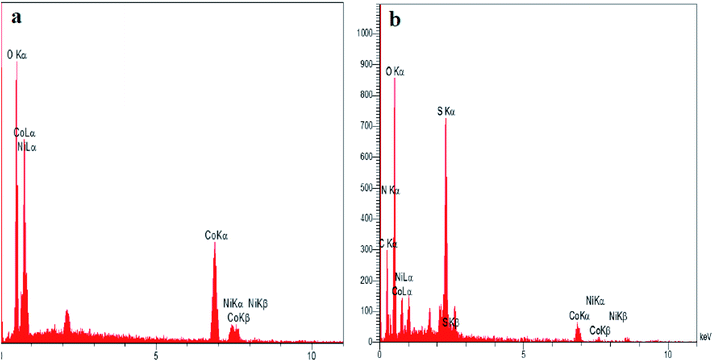 |
| Fig. 3 EDS spectra of (a) Co3O4/NiO and (b) Co3O4/NiO@GQD@SO3H. | |
The magnetic properties of the nanocomposites before and after their decoration with GQDs were tested using a vibrating-sample magnetometer (VSM) (Fig. 4). The lower magnetism of the as-synthesized Co3O4/NiO@GQD@SO3H compared with that of Co3O4/NiO is ascribed to the antiferromagnetic behavior of the GQDs as a dopant. These results demonstrate that the magnetization property decreased by coating and functionalization.39,40
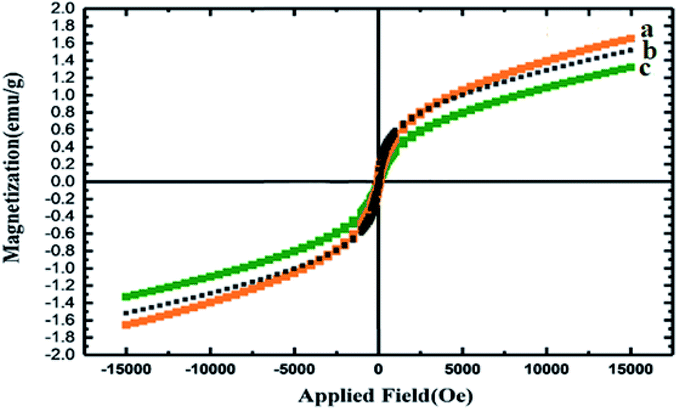 |
| Fig. 4 VSM curves of (a) Co3O4/NiO, (b) Co3O4/NiO@GQDs and (c) Co3O4/NiO@GQD@SO3H. | |
The FT-IR spectra of Co3O4/NiO, Co3O4/NiO@N-GQD and Co3O4/NiO@GQD@SO3H nanocomposite are shown in Fig. 5. The absorption peak at 3335 cm−1 is related to the stretching vibrational absorptions of OH. The peaks at 461.4, 568.4, and 657.1 cm−1 correspond to Ni–O, Co2+–O and Co3+–O respectively. The characteristic peaks at 3440 cm−1 (O–H stretching vibration), 1705 cm−1 (C
O stretching vibration), and 1125 cm−1 (C–O–C stretching vibration) appear in the spectrum in Fig. 5b. The peak at approximately 1475–1580 cm−1 is attributed to the C
C bonds. The presence of the sulfonyl group is also verified by the peaks at 1215 and 1120 cm−1. The broad peak at 3350 cm−1 is related to the stretching vibrational absorptions of OH (SO3H) (Fig. 5c).
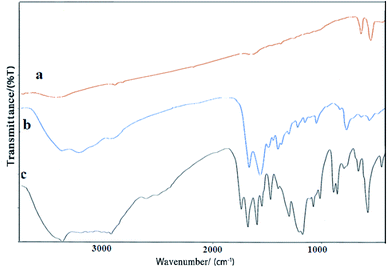 |
| Fig. 5 FT-IR spectra of (a) Co3O4/NiO, (b) Co3O4/NiO@GQDs and (c) Co3O4/NiO@GQD@SO3H. | |
The BET specific surface area of the Co3O4/NiO and Co3O4/NiO@GQD@SO3H nanocomposites was measured by nitrogen gas adsorption–desorption isotherms (Fig. 6). The results indicate that the BET specific surface area of Co3O4/NiO improved from 12.25 to 32.43 m2 g−1 after modification with the GQDs; therefore, more active sites were introduced on the surface of Co3O4/NiO@GQD@SO3H.
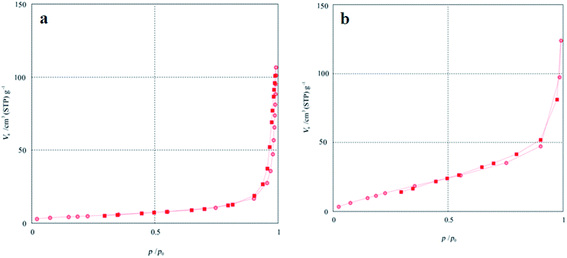 |
| Fig. 6 BET specific surface area of (a) Co3O4/NiO and (b) Co3O4/NiO@GQD@SO3H. | |
TGA (thermogravimetric analysis) was used to evaluate the thermal stability of the Co3O4/NiO@GQD@SO3H nanocomposite. The nanocomposite displayed suitable thermal stability without a significant decrease in weight (Fig. 7). The weight loss at temperatures below 210 °C is owing to the removal of physically adsorbed solvent and surface hydroxyl groups. The curve indicates a weight loss of about 14.06% from 150 °C to 500 °C, which is attributed to the oxidation and degradation of the GQD.
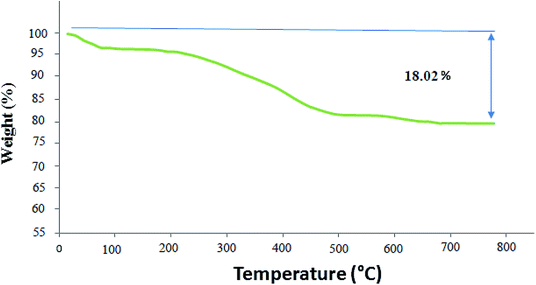 |
| Fig. 7 TGA of Co3O4/NiO@GQD@SO3H nanocomposite. | |
The X-ray photoelectron spectroscopy (XPS) analysis of the Co3O4/NiO@GQD@SO3H nanocomposite is shown in Fig. 8. In the wide-scan spectrum of the nanocatalyst, the predominant components are Ni 2p1/2 (873.4 eV), Ni 2p3/2 (854.4 eV), Co 2p1/2 (792.6 eV), Co 2p3/2 (780.4 eV), O 1s (529.8 eV), N 1s (400 eV), C 1s (284.5 eV) and S 2p (164.3 eV).
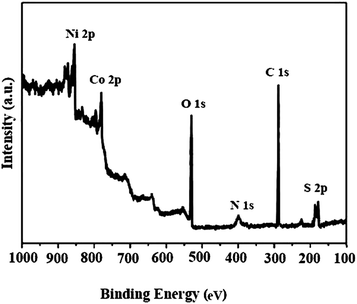 |
| Fig. 8 X-ray photoelectron spectroscopy (XPS) analysis of the Co3O4/NiO@GQD@SO3H nanocomposite. | |
Initially, we carried out a three-component reaction with 4-nitrobenzaldehyde, 6-amino-1,3-dimethyluracil and 4-hydroxycoumarin as a model reaction. The model reactions were performed in the presence of NaHSO4, ZrO2, p-TSA, Co3O4, NiO, Co3O4/NiO, Co3O4/NiO@GQDs and Co3O4/NiO@GQD@SO3H nanocomposite as catalysts. The reactions were tested using diverse solvents including ethanol, acetonitrile, water and dimethylformamide. The best results were obtained in EtOH and we found that the reaction gave convincing results in the presence of the Co3O4/NiO@GQD@SO3H nanocomposite (5 mg) under reflux conditions (Table 1).
Table 1 Optimization of the reaction conditions using different catalystsa
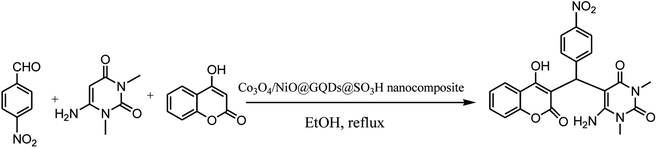
|
Entry |
Catalyst (amount) |
Solvent (reflux) |
Time (min) |
Conversion efficiency |
Yieldb (%) |
4-Nitrobenzaldehyde (1 mmol), 6-amino-1,3-dimethyluracil (1 mmol) and 4-hydroxycoumarin (1 mmol). Isolated yield. |
1 |
None |
EtOH |
300 |
NR |
NR |
2 |
Et3N (5 mol%) |
EtOH |
300 |
28 |
35 |
3 |
NaHSO4 (4 mol%) |
EtOH |
250 |
37 |
42 |
4 |
ZrO2 (4 mol%) |
EtOH |
250 |
43 |
48 |
5 |
pTSA NPs (5 mol%) |
EtOH |
250 |
49 |
54 |
6 |
Nano-Co3O4 |
EtOH |
250 |
40 |
45 |
7 |
Nano-NiO |
EtOH |
250 |
45 |
52 |
8 |
Co3O4/NiO nanocomposite |
EtOH |
250 |
55 |
60 |
9 |
Co3O4/NiO@GQD nanocomposite |
EtOH |
150 |
68 |
72 |
10 |
Co3O4/NiO@GQD@SO3H nanocomposite (3 mg) |
EtOH |
80 |
81 |
85 |
11 |
Co3O4/NiO@GQD@SO3H nanocomposite (5 mg) |
EtOH |
80 |
87 |
93 |
12 |
Co3O4/NiO@GQD@SO3H nanocomposite (7 mg) |
EtOH |
80 |
87 |
93 |
13 |
Co3O4/NiO@GQD@SO3H nanocomposite (5 mg) |
H2O |
100 |
69 |
72 |
14 |
Co3O4/NiO@GQD@SO3H nanocomposite (5 mg) |
DMF |
90 |
71 |
76 |
15 |
Co3O4/NiO@GQD@SO3H nanocomposite (5 mg) |
CH3CN |
80 |
77 |
82 |
A series of aromatic aldehydes were studied under the optimum conditions (Table 2). Good yields were obtained using aromatic aldehydes either bearing electron-withdrawing substituents or electron-donating substituents.
Table 2 Synthesis of chromenpyrimidines using the Co3O4/NiO@GQD@SO3H nanocomposite (5 mg) under reflux conditionsa
Entry |
Aldehyde (1a–1l) |
Product |
Time (min) |
Conversion efficiency |
Yieldb (%) |
mp (°C) (ref.) |
mp (°C) (reported) |
Aromatic aldehydes (1 mmol), 6-amino-1,3-dimethyluracil (1 mmol) and 4-hydroxycoumarin (1 mmol). Isolated yield. |
1 |
 |
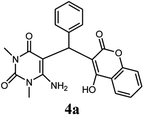 |
80 |
80 |
88 |
218–220 (10) |
220–222 |
2 |
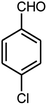 |
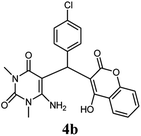 |
80 |
82 |
90 |
219–220 (10) |
218–220 |
3 |
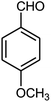 |
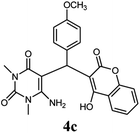 |
90 |
72 |
85 |
180–181 (10) |
178–180 |
4 |
 |
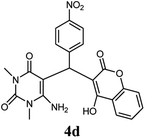 |
80 |
87 |
93 |
240–242 (13) |
240–242 |
5 |
 |
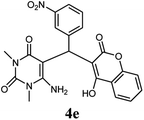 |
80 |
80 |
88 |
230–232 (10) |
230–232 |
6 |
 |
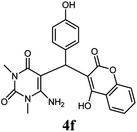 |
110 |
75 |
83 |
— |
230–232 |
7 |
 |
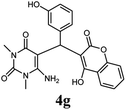 |
100 |
77 |
87 |
— |
232–234 |
8 |
 |
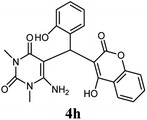 |
110 |
74 |
81 |
— |
240–242 |
9 |
 |
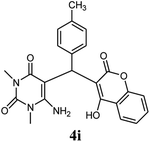 |
90 |
76 |
85 |
202–204 (10) |
202–204 |
10 |
 |
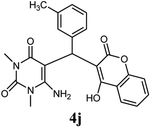 |
85 |
80 |
87 |
— |
200–202 |
11 |
 |
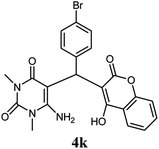 |
80 |
81 |
89 |
236–238 (10) |
236–238 |
12 |
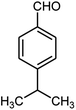 |
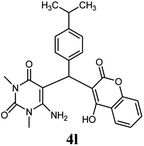 |
100 |
74 |
84 |
257–259 (13) |
257–259 |
13 |
 |
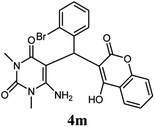 |
80 |
79 |
89 |
210–212 (10) |
210–212 |
14 |
 |
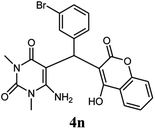 |
80 |
81 |
88 |
245–247 (13) |
245–247 |
We also investigated the recyclability of the Co3O4/NiO@GQD@SO3H nanocomposite as a catalyst for the model reaction under reflux conditions in ethanol. The results showed that nanocomposite can be reused several times without noticeable loss in its catalytic activity (yield in the range of 93% to 91%) (Fig. 9).
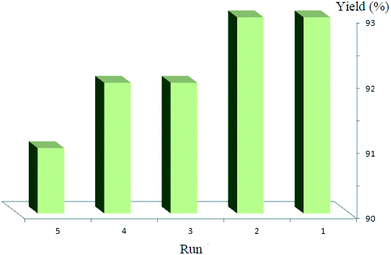 |
| Fig. 9 Recycling of the Co3O4/NiO@GQD@SO3H nanocomposite as a catalyst for the model reaction. | |
The plausible mechanism for the preparation of chromenpyrimidines using the Co3O4/NiO@GQD@SO3H nanocomposite is shown in Scheme 2. Firstly, we assumed that the reaction occurs via condensation between 6-amino-1,3-dimethyluracil and aldehyde to form intermediate I on the active sites of the Co3O4/NiO@GQD@SO3H nanocatalyst. Then, 4-hydroxycoumarin is added to intermediate I to give intermediate II. Then, migration of the hydrogen atom provides the final product (Scheme 2).
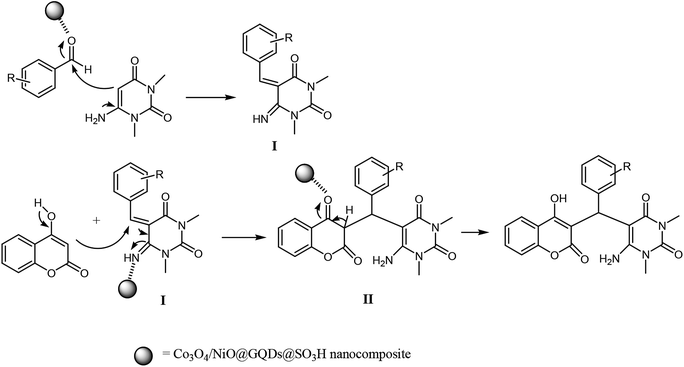 |
| Scheme 2 Proposed mechanism for the three-component reaction. | |
To study the applicability of this method for large-scale synthesis, we performed selected reactions at the 10 mmol scale. As can be seen, the reactions on a large scale gave the product with a gradual decrease in reaction yield (Table 3).
Table 3 The large-scale synthesis of some chromenpyrimidines using the Co3O4/NiO@GQD@SO3H nanocomposite (5 mg)
Entry |
Product |
Time (min) |
Yielda (%) |
Isolated yield. |
1 |
4a |
90 |
86 |
2 |
4c |
95 |
83 |
3 |
4d |
80 |
90 |
4 |
4i |
95 |
82 |
5 |
4k |
80 |
87 |
To compare the efficiency of the Co3O4/NiO@GQD@SO3H nanocomposite with the reported catalysts for the synthesis of chromenpyrimidines, we tabulated the results in Table 4. As indicated in Table 4, the Co3O4/NiO@GQD@SO3H nanocomposite is superior to the reported catalysts. As expected, the increased surface area due to the small particle size increased the reactivity of the catalyst, which is responsible for the accessibility of the substrate molecules on the catalyst surface.
Table 4 Comparison of the catalytic activity of the Co3O4/NiO@GQD@SO3H nanocomposite (5 mg) with that of other reported catalysts for the synthesis chromenpyrimidines (4b)
Entry |
Catalyst (conditions) |
Time (min) |
Conversion efficiency |
Yielda (%) |
Ref. |
Isolated yield. |
1 |
L-proline, 20 mol%, EtOH, reflux |
360 |
75 |
83 |
13 |
2 |
Bifunctional thiourea-based organocatalyst, 20 mol%, H2O, reflux |
240 |
79 |
86 |
14 |
3 |
Sulfamic acid, 30 mol%, (EtOH : H2O: 1 : 1), ultrasound irradiation |
30 |
77 |
85 |
11 |
4 |
Co3O4/NiO@GQD@SO3H nanocomposite (5 mg, EtOH, reflux) |
80 |
82 |
90 |
This work |
The activity of catalysts is influenced by their acid–base properties and many other factors such as surface area, geometric structure (particularly pore structure), distribution of sites and polarity of the surface sites.41,42 The SO3H groups distributed on the surface of Co3O4/NiO@GQDs activate the groups of the substrates. In this mechanism, the surface atoms of Co3O4/NiO@GQD@SO3H activate the C
O and C
N groups for better reaction with nucleophiles. The Co3O4/NiO@GQD@SO3H nanocomposite has Lewis and Brønsted acid properties, which increase the activity of the catalyst.
3. Experimental
3.1. Chemicals and apparatus
NMR spectra were recorded on a Bruker Avance-400 MHz spectrometer in the presence of tetramethylsilane as an internal standard. IR spectra were recorded on an FT-IR Magna 550 spectrometer using KBr plates. Melting points were determined on an Electrothermal 9200, and are not corrected. Elemental analyses (C, H, and N) were performed on a Carlo ERBA Model EA 1108 analyzer. Powder X-ray diffraction (XRD) was performed on a Philips X'pert diffractometer with monochromatized Cu Kα radiation (λ = 1.5406 Å). The microscopic morphology of the nanocatalyst was visualized by SEM (MIRA3). Thermogravimetric analysis (TGA) curves were measured on a V5.1A DUPONT 2000. The magnetic measurement of the samples was performed in a vibrating sample magnetometer (VSM) (Meghnatis Daghigh Kavir Co.; Kashan Kavir; Iran).
3.2. Preparation of Co3O4/NiO nanoparticles
Co(NO3)3 and NiCl2 with a 3
:
1 molar ratio were dissolved in ethylene glycol. Afterward, the appropriate amount of aqueous ammonia solution (28 wt%) was added to the above solution until the pH reached 10. Then, the transparent solution was placed in an autoclave at 150 °C for 4 h. The obtained precipitate was washed twice with methanol and dried at 60 °C for 8 h. Finally, the product was calcined at 500 °C for 2 h.
3.3. Preparation of Co3O4/NiO@N-GQD nanocomposite
1 g citric acid and was dissolved in 20 mL deionized water, and stirred to form a clear solution. Subsequently, 0.3 mL ethylenediamine was added to the above solution and mixed to obtain a clear solution. Then, 0.1 g Co3O4/NiO nanoparticles was added to the mixture. The mixture was stirred at room temperature for 5 min. Then the solution was transferred to a 50 mL Teflon-lined stainless autoclave, which was sealed and heated to 180 °C for 12 h in an electric oven. Finally, the as-prepared nanostructured Co3O4/NiO@GQDs was obtained, which was washed several times with deionized water and ethanol, and then dried in an oven until a constant weight was achieved.
3.4. Preparation of Co3O4/NiO@GQD@SO3H nanocomposite
1 g of Co3O4/NiO@N-GQD nanocomposite was dispersed in dry CH2Cl2 (10 mL) and sonicated for 5 min. Then, chlorosulfonic acid (0.8 mL in dry CH2Cl2) was added dropwise to a cooled (ice-bath) mixture of Co3O4/NiO@N-GQDs for 30 min under N2 with vigorous stirring. The mixture was stirred for 120 min, while the residual HCl was removed by suction. The resulted Co3O4/NiO@GQD@SO3H nanocomposite was separated, washed several times with dried CH2Cl2 before drying under vacuum at 60 °C.
3.5. General procedure for the preparation of chromenpyrimidines
A mixture of aldehyde (1 mmol), 6-amino-1,3-dimethyluracil (1 mmol), 4-hydroxycoumarin (1 mmol) and 5 mg Co3O4/NiO@GQD@SO3H nanocomposite was stirred in 5 mL ethanol under reflux. The reaction was monitored by TLC. After completion of the reaction, the solution was filtered, and the heterogeneous catalyst was recovered. Water was added, and the precipitate was collected by filtration and washed with water. The crude product was recrystallized or washed with ethanol to give the pure product.
3.6. Spectral data
6-Amino-5-((4-hydroxy-2-oxo-2H-chromen-3-yl)(phenyl) methyl)-1,3-dimethylpyrimidine-2,4(1H,3H)-dione (4a). White solid; m.p. 220–222 °C; IR (KBr): 3432, 3234, 2964, 1695, 1664, 1616, 1569, 1444, 1356, 754 cm−1; 1H NMR (400 MHz, DMSO-d6) δ: 3.14 (s, 3H, –C
3), 3.40 (s, 3H, –C
3), 5.62 (s, 1H, –C
), 7.16–7.85 (m, 11H, ArH, –N
2), 13.99 (s, 1H, –O
); 13C NMR (100 MHz, DMSO-d6) δ: 28.68, 31.02, 36.53, 87.26, 105.11, 116.62, 117.41, 124.17, 124.76, 126.14, 126.80, 128.55, 132.87, 138.74, 150.50, 152.44, 155.63, 164.27, 164.55, 166.27; anal. calcd for C22H19N3O5: C, 65.18%; H, 4.72%; N, 10.37%. Found: C, 65.20%; H, 4.59%; N, 10.25%.
6-Amino-5-((4-hydroxy-2-oxo-2H-chromen-3-yl)(4-chlorophenyl) methyl)-1,3-dimethylpyrimidine-2,4(1H,3H)-dione (4b). White solid; m.p. 218–220 °C; IR (KBr): 3405, 3211, 2921, 1695, 1663, 1617, 1493, 1448, 763 cm−1; 1H NMR (400 MHz, DMSO-d6) δ: 3.14 (s, 3H), 3.37 (s, 3H), 5.61 (s, 1H), 7.17–7.85 (m, 10H, ArH and NH2), 13.98 (s, 1H, OH); 13C NMR (100 MHz, DMSO-d6) δ: 28.72, 31.14, 36.17, 86.98, 104.96, 116.60, 117.35, 124.20, 124.82, 128.43, 128.96, 130.78, 132.96, 138.02, 150.52, 152.40, 155.62, 164.25, 164.52, 166.14; anal. calcd for C22H18ClN3O5: C, 60.07%; H, 4.12%; N, 9.55%. Found: C, 60.15%; H, 4.18%; N, 9.42%.
6-Amino-5-((4-hydroxy-2-oxo-2H-chromen-3-yl)(4-methoxy phenyl) methyl)-1,3-dimethylpyrimidine-2,4(1H,3H)-dione (4c). Light yellow solid, m.p.: 178–180 °C; IR (KBr): 3413, 3238, 2952, 1695, 1613, 1568, 1508, 1450, 1253, 767 cm−1, 1H NMR (400 MHz, DMSO-d6) δ: 3.14 (s, 3H), 3.37 (s, 3H), 3.69 (s, 3H), 5.61 (s, 1H), 6.78–6.80 (d, J = 8.0 Hz, 2H), 7.03–7.05 (d, J = 8.0 Hz, 2H), 7.32–7.84 (m, 6H), 13.98 (s, 1H, OH); 13C NMR (100 MHz, DMSO-d6) δ: 28.66, 31.02, 35.83, 55.43, 87.45, 105.43, 113.95, 116.62, 117.42, 124.15, 124.75, 127.90, 130.32, 132.82, 150.56, 152.42, 155.57, 157.88, 164.20, 164.54, 166.29; anal. calcd for C23H21N3O6: C, 63.44%; H, 4.86%; N, 9.65%. Found: C, 63.49%; H, 4.74%; N, 9.59%.
6-Amino-5-((4-hydroxy-2-oxo-2H-chromen-3-yl)(4-nitrophenyl) methyl)-1,3-dimethylpyrimidine-2,4(1H,3H)-dione (4d). Light yellow solid; m.p.: 240–242 °C; IR (KBr): 3396, 3210, 2903, 1685, 1619, 1571, 1514, 1346, 769 cm−1; 1H NMR (400 MHz, DMSO-d6) δ: 3.14 (s, 3H), 3.49 (s, 3H), 5.75 (s, 1H), 7.35–8.10 (m, 10H), 13.96 (s, 1H, OH); 13C NMR (100 MHz, DMSO-d6) δ: 28.69, 31.05, 36.52, 86.48, 104.72, 117.30, 121.45, 121.63, 124.22, 130.00, 133.04, 134.12, 141.74, 148.41, 150.46, 152.47, 155.80, 164.34, 164.55, 165.98; anal. calcd for C22H18N4O7: C, 58.67%; H, 4.03%; N, 12.44%; found C, 58.72%; H, 4.09%; N, 12.52%.
6-Amino-5-((4-hydroxy-2-oxo-2H-chromen-3-yl)(3-nitrophenyl) methyl)-1,3-dimethylpyrimidine-2,4(1H,3H)-dione (4e). Light yellow solid; m.p.: 230–232 °C; IR (KBr): 3460, 3209, 2923, 1699, 1673, 1523, 1437, 1343, 772 cm−1; 1H NMR (400 MHz, DMSO-d6) δ: 3.14 (s, 3H), 3.38 (s, 3H), 5.76 (s, 1H), 7.36–8.06 (m, 10H), 13.97 (s, 1H, OH); 13C NMR (100 MHz, DMSO-d6) δ: 28.69, 31.05, 36.52, 86.48, 104.70, 116.54, 117.35, 121.42, 121.62, 124.80, 124.24, 130.14, 133.24, 134.22, 141.75, 148.45, 150.49, 152.42, 155.92, 164.42, 164.45, 165.85; anal. calcd for C22H18N4O7: C, 58.67%; H, 4.03%; N, 12.44%; found C, 58.75%; H, 4.12%; N, 12.54%.
6-Amino-5-((4-hydroxy-2-oxo-2H-chromen-3-yl)(4-hydroxyphenyl) methyl)-1,3-dimethylpyrimidine-2,4(1H,3H)-dione (4f). White solid; m.p. 230–232 °C; IR (KBr): 3364, 3219, 1695, 1671, 1569, 1509, 1444, 1358, 769 cm−1; 1H NMR (400 MHz, DMSO-d6) δ: 3.14 (s, 3H), 3.49 (s, 3H), 5.51 (s, 1H), 6.60–6.62 (d, J = 8.0 Hz, 2H), 6.90–6.92 (d, J = 8.0 Hz, 2H), 7.29–7.84 (m, 6H), 9.14 (s, 1H, OH), 13.97 (s, 1H, OH); 13C NMR (100 MHz, DMSO-d6) δ: 28.64, 30.96, 35.74, 87.54, 105.45, 115.35, 116.55, 117.40, 124.12, 124.73, 127.77, 128.41, 132.79, 150.50, 152.35, 155.43, 155.76, 164.17, 164.49, 166.27. Anal. calcd for C22H19N3O6: C, 62.70%; H, 4.54%; N, 9.97%; found C, 62.78%; H, 4.59%; N, 10.05%.
6-Amino-5-((4-hydroxy-2-oxo-2H-chromen-3-yl)(3-hydroxyphenyl) methyl)-1,3-dimethylpyrimidine-2,4(1H,3H)-dione (4g). White solid; m.p. 232–234 °C; IR (KBr): 3429, 3306, 2957, 1695, 1670, 1617, 1569, 1447, 1354, 760 cm−1; 1H NMR (400 MHz, DMSO-d6) δ: 3.15 (s, 3H), 3.38 (s, 3H), 5.55 (s, 1H), 6.53–7.67 (m, 10H), 9.12 (s, 1H, OH), 14.03 (s, 1H, OH); 13C NMR (100 MHz, DMSO-d6) δ: 28.72, 30.94, 35.72, 87.55, 104.55, 115.34, 116.52, 116.58, 117.42, 124.14, 124.25, 124.78, 127.75, 128.43, 132.79, 151.22, 152.34, 155.42, 155.78, 164.15, 164.45, 166.28. Anal. calcd for C22H19N3O6: C, 62.70%; H, 4.54%; N, 9.97%; found C, 62.72%; H, 4.55%; N, 10.09%.
6-Amino-5-((4-hydroxy-2-oxo-2H-chromen-3-yl)(2-hydroxyphenyl) methyl)-1,3-dimethylpyrimidine-2,4(1H,3H)-dione (4h). White solid; m.p. 240–242 °C; IR (KBr): 3432, 3306, 2958, 1695, 1607, 1617, 1447, 760 cm−1; 1H NMR (400 MHz, DMSO-d6) δ: 3.15 (s, 3H), 3.38 (s, 3H), 5.55 (s, 1H), 6.63–7.87 (m, 10H), 9.11 (s, 1H, OH), 14.03 (s, 1H, OH); 13C NMR (100 MHz, DMSO-d6) δ: 28.67, 31.02, 36.40, 87.35, 104.98, 113.16, 113.69, 116.58, 116.59, 117.48, 124.23, 124.81, 124.83, 129.47, 132.93, 140.27, 150.51, 152.36, 155.52, 157.72, 164.48, 166.23. Anal. calcd for C22H19N3O6: C, 62.70%; H, 4.54%; N, 9.97%; found C, 62.82%; H, 4.62%; N, 9.98%.
6-Amino-5-((4-hydroxy-2-oxo-2H-chromen-3-yl)(4-methylphenyl) methyl)-1,3-dimethylpyrimidine-2,4(1H,3H)-dione (4i). White solid; m.p. 202–204 °C; IR (KBr): 3411, 3212, 2922, 1697, 1665, 1618, 1569, 1353, 764 cm−1; 1H NMR (400 MHz, DMSO-d6) δ: 2.24 (s, 3H), 3.14 (s, 3H), 3.37 (s, 3H), 5.57 (s, 1H), 6.96–7.85 (m, 10H), 13.98 (s, 1H, OH); 13C NMR (100 MHz, DMSO-d6) δ: 20.60, 28.32, 30.64, 35.74, 87.30, 104.72, 116.25, 123.72, 124.17, 124.42, 126.37, 128.82, 132.58, 134.74, 135.13, 150.18, 152.02, 155.12, 164.15, 165.93, 167.75; anal. calcd for C23H21N3O5: C, 65.86%; H, 5.05%; N, 10.02%; found C, 65.92%; H, 5.15%; N, 10.08%.
6-Amino-5-((4-hydroxy-2-oxo-2H-chromen-3-yl)(3-methylphenyl) methyl)-1,3-dimethylpyrimidine-2,4(1H,3H)-dione (4j). White solid; m.p. 200–202 °C; IR (KBr): 3403, 3229, 2923, 1699, 1695, 1445, 758 cm−1; 1H NMR (400 MHz, DMSO-d6) δ: 2.21 (s, 3H), 3.15 (s, 3H), 3.38 (s, 3H), 5.59 (s, 1H), 6.92–7.86 (m, 10H), 13.97 (s, 1H, OH); 13C NMR (100 MHz, DMSO-d6) δ: 21.63, 28.66, 30.98, 36.37, 87.34, 105.11, 116.58, 117.37, 123.91, 124.18, 124.77, 126.87, 127.29, 128.42, 132.85, 137.50, 138.66, 150.50, 152.37, 155.52, 164.26, 164.54, 166.25; anal. calcd for C23H21N3O5: C, 65.86%; H, 5.05%; N, 10.02%; found C, 65.96%; H, 5.18%; N, 10.12%.
6-Amino-5-((4-hydroxy-2-oxo-2H-chromen-3-yl)(4-bromophenyl) methyl)-1,3-dimethylpyrimidine-2,4(1H,3H)-dione (4k). White solid; m.p. 236–238 °C; IR (KBr): 3404, 3212, 2921, 1675, 1663, 1616, 1488, 1446, 761 cm−1; 1H NMR (400 MHz, DMSO-d6) δ: 3.13 (s, 3H), 3.37 (s, 3H), 5.58 (s, 1H), 7.11–7.84 (m, 10H), 13.97 (s, 1H, OH); 13C NMR (100 MHz, DMSO-d6) δ: 28.75, 31.17, 36.19, 86.95, 104.93, 116.63, 117.34, 124.22, 124.85, 128.40, 128.93, 130.79, 132.94, 138.08, 150.55, 152.42, 155.67, 164.28, 164.54, 166.16; anal. calcd for C22H18BrN3O5: C, 54.56%; H, 3.75%; N, 8.68%. Found: C, 54.62%; H, 3.83%; N, 8.75%.
6-Amino-5-((4-hydroxy-2-oxo-2H-chromen-3-yl)(4-isopropylphenyl) methyl)-1,3-dimethylpyrimidine-2,4(1H,3H)-dione (4l). White solid; m.p. 257–259 °C; IR (KBr): 3463, 3230, 2958, 1695, 1671, 1616, 1569, 1446, 1343, 762 cm−1; 1H NMR (400 MHz, DMSO-d6) δ: 1.15 (s, 6H), 2.77 (m, 1H), 3.15 (s, 3H), 3.38 (s, 3H), 5.57 (s, 1H), 7.02–7.85 (m, 10H), 13.99 (s, 1H, OH); 13C NMR (100 MHz, DMSO-d6) δ: 23.8, 28.1, 30.3, 32.9, 35.6, 87.1, 104.4, 115.8, 116.9, 123.7, 123.9, 125.8, 126.1, 131.9, 135.2, 145.5, 149.9, 151.9, 155.0, 163.8, 164.1, 168.3; anal. calcd for C25H25N3O5: C, 67.10%; H, 5.63%; N, 9.39%; found C, 67.19%; H, 5.75%; N, 9.45%.
6-Amino-5-((4-hydroxy-2-oxo-2H-chromen-3-yl)(2-bromophenyl) methyl)-1,3-dimethylpyrimidine-2,4(1H,3H)-dione (4m). White solid; m.p. 210–212 °C; IR (KBr): 3402, 3210, 2925, 1676, 1665, 1618, 1489, 1447, 765 cm−1; 1H NMR (400 MHz, DMSO-d6) δ: 3.12 (s, 3H), 3.35 (s, 3H), 5.57 (s, 1H), 7.12–7.86 (m, 10H), 13.96 (s, 1H, OH); 13C NMR (100 MHz, DMSO-d6) δ: 28.74, 31.16, 36.10, 86.94, 104.92, 116.63, 117.29, 124.25, 124.82, 126.35, 127.52, 128.40, 128.92, 130.75, 132.96, 138.05, 150.56, 152.47, 155.65, 164.22, 164.55, 166.14; anal. calcd for C22H18BrN3O5: C, 54.56%; H, 3.75%; N, 8.68%. Found: C, 54.65%; H, 3.86%; N, 8.77%.
6-Amino-5-((4-hydroxy-2-oxo-2H-chromen-3-yl)(3-bromophenyl) methyl)-1,3-dimethylpyrimidine-2,4(1H,3H)-dione (4n). White solid; m.p. 245–247 °C; IR (KBr): 3405, 3210, 2924, 1673, 1665, 1617, 1489, 1445, 764 cm−1; 1H NMR (400 MHz, DMSO-d6) δ: 3.13 (s, 3H), 3.35 (s, 3H), 5.59 (s, 1H), 7.10–7.89 (m, 10H), 13.98 (s, 1H, OH); 13C NMR (100 MHz, DMSO-d6) δ: 28.74, 31.18, 36.17, 86.95, 104.94, 116.62, 117.31, 124.26, 124.85, 125.33, 126.54, 128.42, 128.94, 130.72, 132.90, 138.07, 150.52, 152.43, 155.64, 164.22, 164.50, 166.12; anal. calcd for C22H18BrN3O5: C, 54.56%; H, 3.75%; N, 8.68%. Found: C, 54.65%; H, 3.87%; N, 8.79%.
4. Conclusions
In conclusion, we reported an efficient method for the synthesis of chromenpyrimidines using the Co3O4/NiO@GQD@SO3H nanocomposite as a superior catalyst under reflux conditions. The new catalyst was characterized via FT-IR, SEM, XRD, EDS, TGA, BET and VSM. The current method provides obvious advantages, including environmental friendliness, short reaction time, reusability of the catalyst, low catalyst loading and simple workup procedure.
Conflicts of interest
There are no conflicts to declare.
Acknowledgements
The authors acknowledge a reviewer who provided helpful insights. The authors are grateful to University of Kashan for supporting this work by grant no.: 159196/XXI.
References
- G. M. Ziarani, N. H. Nasab and N. Lashgari, RSC Adv., 2016, 6, 38827–38848 RSC.
- R. S. Keri, K. M. Hosamani, R. V. Shingalapur and M. H. Hugar, Eur. J. Med. Chem., 2010, 45, 2597–2605 CrossRef CAS PubMed.
- U. S. Rai, A. M. Isloor, P. shetty, A. M. Vijesh, N. Prabhu, S. Isloor, M. Thiageeswaran and H. K. Fun, Eur. J. Med. Chem., 2010, 45, 2695–2699 CrossRef PubMed.
- S. V. Laxmi, B. S. Kuarm and B. Rajitha, Med. Chem. Res., 2013, 22, 768–774 CrossRef.
- N. R. Emmadi, K. Atmakur, C. Bingi, N. R. Godumagadda, C. G. Kumar and J. B. Nanubolu, Bioorg. Med. Chem. Lett., 2014, 24, 485–489 CrossRef CAS PubMed.
- A. A. Abu-Hashem and M. M. Youssef, Molecules, 2011, 16, 1956–1972 CrossRef CAS PubMed.
- C. Sun, C. Chen, S. Xu, J. Wang, Y. Zhu, D. Kong, H. Tao, M. Jin, P. Zheng and W. Zhu, Bioorg. Med. Chem., 2016, 24, 3862–3869 CrossRef CAS PubMed.
- N. R. Kamdar, D. D. Haveliwala, P. T. Mistry and S. K. Patel, Med. Chem. Res., 2011, 20, 854–864 CrossRef CAS.
- T. P. Selvam, C. R. James, P. V. Dniandev and S. K. Valzita, Res. Pharm., 2012, 2, 01–09 Search PubMed.
- G. Basumatary, R. Mohanta and G. Bez, Catal. Lett., 2019, 149, 2776–2786 CrossRef CAS.
- G. Brahmachari, M. Mandal, I. Karmakar, K. Nurjamal and B. Mandal, ACS Sustainable Chem. Eng., 2019, 7, 6369–6380 CrossRef CAS.
- S. Abdolmohammadi, S. Balalaie, M. Barari and F. Rominger, Comb. Chem. High Throughput Screening, 2013, 16, 150–159 CAS.
- R. Bharti and T. Parvin, Synth. Commun., 2015, 45, 1442–1450 CrossRef CAS.
- R. Bharti and T. Parvin, RSC Adv., 2015, 5, 66833–66839 RSC.
- X. T. Zheng, A. Ananthanarayanan, K. Q. Luo and P. Chen, Small, 2015, 11(14), 1620–1636 CrossRef CAS PubMed.
- B. Senel, N. Demir, G. Büyükköroglu and M. Yildiz, Saudi Pharm. J., 2019, 27, 846–858 CrossRef PubMed.
- M. J. Molaei, RSC Adv., 2019, 9, 6460–6481 RSC.
- T. F. Yeh, C. Y. Teng, S. J. Chen and H. Teng, Adv. Mater., 2014, 26, 3297–3303 CrossRef CAS PubMed.
- F. Xi, J. Zhao, C. Shen, J. He, J. Chen, Y. Yan, K. Li, J. Liu and P. Chen, Carbon, 2019, 153, 127–135 CrossRef CAS.
- Y. Wang, Y. Shao, D. W. Matson, J. Li and Y. Lin, ACS Nano, 2010, 4, 1790–1798 CrossRef CAS PubMed.
- Q. Li, S. Zhang, L. Dai and L. S. Li, J. Am. Chem. Soc., 2012, 134, 18932–18935 CrossRef CAS PubMed.
- A. L. M. Reddy, A. Srivastava, S. R. Gowda, H. Gullapalli, M. Dubey and P. M. Ajayan, ACS Nano, 2010, 4, 6337–6342 CrossRef CAS PubMed.
- M. T. Hasan, R. Gonzalez-Rodriguez, C. Ryan, K. Pota, K. Green, J. L. Coffer and A. V. Naumov, Nano Res., 2019, 12, 1041–1047 CrossRef CAS.
- F. Temerov, A. Beliaev, B. Ankudze and T. T. Pakkanen, J. Lumin., 2019, 206, 403–411 CrossRef CAS.
- S. Zhu, Y. Song, X. Zhao, J. Shao, J. Zhang and B. Yang, Nano Res., 2015, 8, 355–381 CrossRef CAS.
- D. Qu, M. Zheng, J. Li, Z. Xie and Z. Sun, Light: Sci. Appl., 2015, 4, 364–371 CrossRef.
- S. Sajjadi, A. Khataee, R. D. C. Soltani and A. Hasanzadeh, J. Phys. Chem. Solids, 2019, 127, 140–150 CrossRef CAS.
- Y. Du and S. Guo, Nanoscale, 2016, 8, 2532–2543 RSC.
- Y. Yan, J. Gong, J. Chen, Z. Zeng, W. Huang, K. Pu, J. Liu and P. Chen, Adv. Mater., 2019, 31, 1808283–1808305 CrossRef PubMed.
- M. Li, T. Chen, J. J. Gooding and J. Liu, ACS Sens., 2019, 4, 1732–1748 CrossRef CAS PubMed.
- Z. Wang, H. Zeng and L. Sun, J. Mater. Chem. C, 2015, 3, 1157–1165 RSC.
- E. Hu, X. Y. Yu, F. Chen, Y. Wu, Y. Hu, X. Wen and D. Lou, Adv. Energy Mater., 2018, 8, 1702476–1702483 CrossRef.
- E. Hu, J. Ning, D. Zhao, C. Xu, Y. Lin, Y. Zhong, Z. Zhang, Y. Wang and Y. Hu, Small, 2018, 14, 1704233–1704241 CrossRef PubMed.
- E. Hu, Y. Feng, J. Nai, D. Zhao, Y. Hu, X. Wen and D. Lou, Energy Environ. Sci., 2018, 11, 872–880 RSC.
- Q. Li, W. Lu, Z. Li, J. Ning, Y. Zhong and Y. Hu, Chem. Eng. J., 2020, 380, 122544–122552 CrossRef CAS.
- E. Hu, J. Ning, B. He, Z. Li, C. Zheng, Y. Zhong, Z. Zhang and Y. Hu, J. Mater. Chem. A, 2017, 5, 2271–2279 RSC.
- D. Qu, M. Zheng, P. Du, Y. Zhou, L. Zhang, D. Li, H. Tan, Z. Zhao, Z. Xie and Z. Sun, Nanoscale, 2013, 5, 12272–12277 RSC.
- K. Li, J. Chen, Y. Yan, Y. Min, H. Li, F. Xi, J. Liu and P. Chen, Carbon, 2018, 136, 224–233 CrossRef CAS.
- N. Limchoowong, P. Sricharoen, Y. Areerob, P. Nuengmatcha, T. Sripakdee, S. Techawongstien and S. Chanthai, Food Chem., 2017, 230, 388–397 CrossRef CAS PubMed.
- F. Alemi-Tameh, J. Safaei-Ghomi, M. Mahmoudi-Hashemi and R. Teymuri, Res. Chem. Intermed., 2016, 42, 6391–6406 CrossRef CAS.
- J. Safaei-Ghomi, H. Shahbazi-Alavi and E. Heidari-Baghbahadorani, RSC Adv., 2014, 4, 50668–50677 RSC.
- J. Safaei-Ghomi and H. Shahbazi-Alavi, J. Saudi Chem. Soc., 2017, 21, 698–707 CrossRef CAS.
Footnote |
† Electronic supplementary information (ESI) available. See DOI: 10.1039/c9ra05896f |
|
This journal is © The Royal Society of Chemistry 2019 |