DOI:
10.1039/C9RA05644K
(Paper)
RSC Adv., 2019,
9, 28768-28774
Preparation of recyclable MoO3 nanosheets for visible-light driven photocatalytic reduction of Cr(VI)
Received
22nd July 2019
, Accepted 29th August 2019
First published on 12th September 2019
Abstract
The exploitation of stable and earth-abundant photocatalysts with high catalytic activity remains a significant challenge for removing heavy metals from wastewater. Different from complex nanostructuring, this work focuses on a simple and feasible way to design catalysts. Herein, MoO3 nanosheets were fabricated and grown vertically on the surface of a quartz tablet using a one-step chemical vapor deposition method. The morphology, construction and optical properties of the MoO3 were characterized by XRD, XPS, SEM, HRTEM and UV-Vis methods, and the possible growth mechanism was also discussed. It can be found that the MoO3 nanosheets exhibited significantly enhanced visible light photocatalytic reduction capacity with stable recyclability to Cr(VI). The results show that the MoO3 nanosheets can be used as a cost-effective and recyclable photocatalyst for the removal of Cr(VI) from water. Our findings provide new inspiration for the design of new types of catalysts.
1. Introduction
Wastewater always contains heavy metals and organic compounds which endanger the environment. As is well known, chromium is widely used in many fields such as mining, chemical manufacturing, electroplating and leather making and processing, and pollution caused by chromium, mainly Cr(VI), has been ranked among the top ten worst toxic contamination issues according to a report released in 2012.1,2 Thus, the removal of Cr(VI) in water has great significance to our environment.
Environmental friendly catalytic processes are increasingly receiving attention nowadays, and photocatalytic technology provides a method for overcoming the problems.3 The photocatalysis is a physico-chemical process in which chemical reactions are driven using light energy and photocatalysts.4 Semiconducting nanomaterials have been widely used as photocatalysts because they are unique in nature of the mechanical, optical, electrical, catalytic and magnetic properties.5–8
As a n-type semiconductor, molybdenum trioxide (MoO3) can be used in catalytic, optical and electrochemical applications, and compared to other metal oxide materials, MoO3 has low cost, good structural stability and less toxicity.9–11 For example, assembled-sheets-like MoO3 was synthesized with a facile heat treatment route and used as an anode for lithium ion batteries, which maintained an improved discharge capacity over 100 cycles with capacity retention of ∼100%;12 Wang et al. prepared ultrafine β-MoO3 from industrial grade MoO3 powder via the method of sublimation and discussed its growth mechanism;13 Pandeeswari et al. fabricated nanostructured α-MoO3 thin film as a highly selective TMA sensor;14 by a solution combustion reaction, ultra-porous MoO3 can be obtained with both excellent photocatalytic and electrochemical properties.15
Therefore, the development of MoO3 nanoparticles has consequently increased in recent years.16–20 By now, many synthesis processes of MoO3 are widely investigated using electrodeposition, molten salt, hydrothermal, sol–gel methods et al., and different morphologies including nanofibers, microbelts and flower-like spheres can be fabricated.21–26
Nowadays MoO3 has been widely used as photocatalysts. As reported in ref. 27, three-dimensional MoO3 nanoflowers assembled with nanosheets were fabricated by the modified hydrothermal method for degradation of RhB under visible light; Liu et al. fabricated hierarchical porous monoliths of MoO3 nanoplates. The as-prepared MoO3 exhibited strong adsorption performance and excellent photocatalytic activity as well as promising cyclic performance for photodegradation of MB;28 MoO3 microrods were synthesized using leaf extract of Azadirachta indica and used for the cationic dye degradation;29 Ma et al. prepared MoO3 nanoflake on conjugated polymer for hydrogen evolution from water solution under solar light.30
It is known that two-dimensional materials shows very interesting physical and chemical characteristics.31–33 In this work, MoO3 nanosheets which deposited vertically on the surface of quartz tablet were fabricated by one-step chemical vapor deposition method. The morphology, construction and optical properties of MoO3 were characterized by XRD, XPS, SEM, HRTEM, UV-Vis methods, respectively. The photocatalytic and recyclable performance of the as-prepared MoO3 on the degradation Cr(VI) were analyzed. The fabrication process is low energy consumption, easy to operate and environmentally friendly. Meanwhile, the MoO3 deposited on quartz tablet is easy to be separated from water, which avoids secondary pollution during using process. Thus, the as-prepared MoO3 exhibits good application prospect for solving the pollution problems in water.
2. Experimental section
2.1 Materials
All chemicals including molybdenum disulfide (MoS2) and potassium dichromate (K2Cr2O7) are analytically pure and used as received without further purification. Deionized water was used for all procedures.
2.2 Preparation of MoO3 nanosheets
MoO3 nanosheets were prepared by chemical vapor deposition. As shown in Fig. 1, 0.2 g MoS2 were placed in a combustion boat on the middle of the tube furnace, and then a piece of quartz tablet (1 × 1 cm) was placed behind the MoS2 with a distance of about 15 cm, and 517 sccm argon gas was pumped for 40 min. After that, the tube furnace was vacuum pumping till 7.5 × 10−2 Torr, and then heated to 900 °C with the heating rate of 10 °C min−1. The reaction was kept for 20 min with Ar flow of 26 sccm and O2 flow of 4 sccm. Finally the system was cooled to room temperature and the quartz tablet coated with products was taken out for further analysis.
 |
| Fig. 1 The fabrication process for MoO3 using CVD method. | |
2.3 Characterization
Scanning electron microscope (SEM) images were obtained using a HITACHI S-4800 microscope (Japan). High-resolution transmission electron microscopy (HRTEM) images were obtained using a FEI Tecnai G2 F30 microscope (USA). The phase purity of the product was characterized by X-ray diffraction (XRD, German Bruker AXSD8 ADVANCE X-ray diffractometer) using an X-ray diffractometer with Cu KR radiation (λ = 1.5418 Å). The ultraviolet-visible (UV-Vis) diffuse reflectance spectra were obtained on an America Varian Cary 5000 spectrophotometer. Raman spectra were measured using a Britain Renishaw inVia Raman spectrometer at room temperature.
2.4 Photocatalytic experiments
The degradation of K2Cr2O7 was proceeded using the as-prepared MoO3 nanosheets as photocatalysts with visible lights. Cr(VI) stock solution (10 mg L−1) was prepared by dissolving K2Cr2O7 in distilled water. After the a piece of MoO3-coated quartz tablet was immersed in 20 mL of Cr(VI) solution, the resulting solution was magnetically stirred in the dark for 30 min at room temperature. Then the photocatalytic experiments were carried out in a GHX-2 photochemical reactor as report in ref. 34 and 35, which includes mainly four parts: light source system including a 300 W xenon lamp with a 420 nm cut-off filter and cooling attachments such as electric fan; reactor (two-layer Pyrex glass bottles of 400 mL capacity, the space between the two layers is filled with circulating water to cool the reactor); magnetic stirrer; and temperature controller. In each experiment, the distance between the xenon lamp and the reactor was set to be 5.8 cm, and the reaction temperature was 25 °C. At an interval of 10 min, 3 mL of the solution was extracted and monitored via UV-Vis spectrometer.
The photocatalytic efficiency of Cr(VI) could be calculated according to the following equation: % degradation = (1 − At/A0) × 100%, where A0 and At are the initial absorbance and absorbance at time ‘t’ of Cr(VI).36
Besides, as referential photocatalysts, 0.8 mg TiO2 (P25) and 0.8 mg MoS2 nanosheets were respectively used for degradation of Cr(VI).
3 Results and discussion
3.1 Characterizations
Fig. 2(a) displayed XRD patterns of the as-prepared MoO3. Diffraction peaks of the product is in good agreement with orthorhombic α-MoO3 (JCPDS card no. 05-0508) and the main peaks observed at 12.9°, 23.5°, 26.1°, 27.4° and 39.2° are corresponding to the (020), (110), (040), (021) and (060) planes. No other peaks of impurities were detected. The sample exhibits sharp peaks which ascribable to its highly crystalline nature.
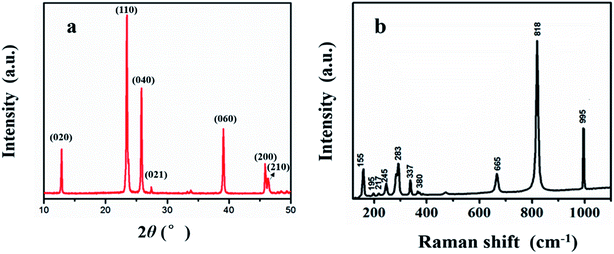 |
| Fig. 2 (a) XRD and (b) Raman spectra of MoO3. | |
Fig. 2(b) shows the typical Raman spectra of MoO3 nanosheets. The Raman peaks at 819 cm−1 and 995 cm−1 should be induced by the symmetric and asymmetric stretching vibration modes of terminal bond Mo
O. The asymmetric stretching vibration mode of O–Mo–O was assigned at 665 cm−1, while the Raman peaks at 380 cm−1, 337 cm−1, 283 cm−1 and 245 cm−1 indicated the vibrating bending modes in MoO3.11
According to XRD and Raman analytical results, it can be found that the product is well-crystallized MoO3.
XPS analysis was performed in further to confirm the presence of elements of Mo. Fig. 3(a) shows that the peaks in the spectra were assigned to Mo, O, and C. From Fig. 3(b), two peaks located at 233 eV and 236 eV can be respectively due to Mo 3d1/2 and Mo 3d3/2 signals, ensuring the Mo6+ valence state.28 Besides, the atom ratio of Mo and O is about 1
:
3, in further proving the formation of MoO3.
 |
| Fig. 3 XPS spectra of the MoO3 (a) a typical survey spectrum (b) Mo 3d core level (c) O 1s core level. | |
The morphology of the as-prepared MoO3 was characterized via SEM and TEM respectively and the results can be found in Fig. 4(a)–(d).
 |
| Fig. 4 (a and b) SEM and (c and d) TEM images of MoO3 nanosheets. | |
From SEM images, it can be found that MoO3 nanosheets were uniformly grown vertically on the surface of the substrate, which form 3D network structure and possess large surface area. Fig. 4(c) is the TEM image of MoO3 nanosheet and Fig. 4(d) is the HRTEM image. It can be found that the as-prepared MoO3 is well-crystallized and the lattice spacing is 0.375 nm, which belongs to the (110) plane.
To investigate the light absorption properties of the as-prepared MoO3, the UV-Vis absorption spectra of the MoO3 was recorded and displayed in Fig. 5. It can be seen that there is a broad absorbance peak during 200–450 nm, and band gap is estimated to be 2.9 eV from the plots of (αhν)2 versus photon energy hν.37
 |
| Fig. 5 UV-Vis spectra of MoO3. | |
3.2 Growth mechanism
In this work, the MoO3 nanosheets which grown vertically on the surface of quartz tablet were fabricated via CVD method. During the CVD process, MoS2 was steamed up in the high-temperature area. Then it was reacted with oxygen and meanwhile driven by Ar, then deposited gradually on the surface of the quartz tablet in the low-temperature area. Therefore, the growing mechanism of the morphology is based on the vapor-solid (VS) mechanism. According to VS mechanism, the size of the nanosheet is proportional to the time and growth rate, which is depend on the evaporation rate and pressure. According to
, P is the probability of nucleation, B is a constant, σ is the surface energy of solid whiskers, k is the boltzmann constant, T is the absolute temperature, α is the supersaturation ratio (defined as: α = p/p0, p and p0 are the initial pressure and equilibrium pressure at the corresponding ambient temperature respectively). Evaporation rate and system pressure may increase by affecting the supersaturated vapor pressure. Thus, MoO3 nanosheets were more easily formed under higher temperature and supersaturation.
3.3 Photocatalytic activity of Cr(VI)
Fig. 6(a) displays the photocatalytic efficiency of Cr(VI) under visible light with MoO3 as photocatalyst. It can be found that the intensity of characteristic peak of Cr(VI) gradually weakens as the irradiation time goes on, indicating the degradation of the Cr(VI), which proves that the as-prepared MoO3 can be used to degrade Cr(VI).
 |
| Fig. 6 (a) Photocatalytic efficiency of MoO3 and (b) degradation performance of the referential photocatalysts. | |
P25 and MoS2 nanosheets were used as referential photocatalysts for degradation of Cr(VI), and it can be found that both the P25 and MoS2 nanosheets have little photocatalytic performance on the degradation of Cr(VI) as shown in Fig. 6(b).
The photocatalytic mechanism of the as-prepared MoO3 nanosheets were discussed as shown in Fig. 7. The conduction band and valence band potentials of the semiconductor were calculated by ECB = ε − EH − 0.5Eg and EVB = ECB + Eg. ε is the absolute electronegativity of an atom semiconductor, which is defined as the arithmetic mean of the atomic electro affinity and the first ionization energy; EH is the energy of free electrons of hydrogen scale (4.5 eV); ECB and EVB are the potentials of the conduction and valence bands, respectively, while Eg is the band gap between the conduction and valence bands.28,38 Therefore, the conduction and valence band positions of MoO3 were calculated to be 0.4 eV and 3.3 eV, respectively.
 |
| Fig. 7 Photocatalytic mechanism of the as-prepared MoO3 nanosheets. | |
The photo-excited electrons converge on the conduction band of the MoO3 and reduce the absorbed oxygen molecules on the MoO3 surface, inducing a chain of reactions as follows:34
|
MoO3 + hν → MoO3 (h+ + e−)
| (1) |
|
6e− + Cr2O72− + 14H+ → 2Cr3+ + 7H2O
| (2) |
The recycling of MoO3 nanosheets used for photocatalytic degradation of Cr(VI) under visible-light irradiation was investigated and the results can be found in Fig. 8.
 |
| Fig. 8 (a) Recycling of MoO3 for the photocatalytic degradation of Cr(VI) and (b) TEM of MoO3 after reusing. Inset is the Raman spectra. | |
The recycling test showed that the photocatalytic activity did not exhibit obvious reduction during the recycling, and the Cr(VI) removal of 85% can still be achieved even after recycling for five times. According to the TEM and Raman analysis, it can be found that there was almost no change of the morphology and structure of MoO3, which meant the recyclable of the as-prepared photocatalyst.
4. Conclusions
MoO3 nanosheets were fabricated and deposited vertically on the surface of quartz tablet by one-step CVD method. The MoO3 nanosheets exhibited good photocatalytic efficiency for degradation of Cr(VI). The catalyst was easy to be separated from water and almost retained the catalytic efficiency after reused five times. Thus, the as-prepared MoO3 has a clear advantage for applications in environmental fields.
Conflicts of interest
There are no conflicts to declare.
Acknowledgements
This work is funded by New methods of analysis and testing of Jiangsu Association for Instrumental Analysis.
References
- J. Ye, J. Liu, Z. Huang, S. Wu, X. Dai, L. Zhang and L. Cui, Effect of reduced graphene oxide doping on photocatalytic reduction of Cr(VI) and photocatalytic oxidation of tetracycline by ZnAlTi layered double oxides under visible light, Chemosphere, 2019, 227, 505–513 CrossRef CAS PubMed
. - Y. Mu, Z. Ai, L. Zhang and F. Song, Insight into Core–Shell Dependent Anoxic Cr(VI) Removal with Fe@Fe2O3 Nanowires: Indispensable Role of Surface Bound Fe(II), ACS Appl. Mater. Interfaces, 2015, 7, 1997–2005 CrossRef CAS PubMed
. - W. Zhao, J. Li, B. Dai, Z. Cheng, J. Xu, K. Ma, L. Zhang, N. Sheng, G. Mao, H. Wu, K. Wei and D. Y. C. Leung, Simultaneous removal of tetracycline and Cr(VI) by a novel three dimensional AgI/BiVO4 p–n junction photocatalyst and insight into the photocatalytic mechanism, Chem. Eng. J., 2019, 369, 716–725 CrossRef CAS
. - T. Sakthivel, G. Venugopal, A. Durairaj, S. Vasanthkumar and X. Huang, Utilization of the internal electric field in semiconductor photocatalysis: a short review, J. Ind. Eng. Chem., 2019, 72, 18–30 CrossRef CAS
. - D. Venkatesh, S. Pavalamalar and K. Anbalagan, Selective photodegradation on dual dye system by recoverable Nano SnO2 photocatalyst, J. Inorg. Organomet. Polym. Mater., 2019, 29, 939–953 CrossRef CAS
. - T. Xu, S. Wang, L. Li and X. Liu, Dual templated synthesis of tri-modal porous SrTiO3/TiO2@ carbon composites with enhanced photocatalytic activity, Appl. Catal., A, 2019, 575, 132–141 CrossRef CAS
. - X. Tao, Y. Gao, S. Wang, X. Wang, Y. Liu, Y. Zhao, F. Fan, M. Dupuis, R. Li and C. Li, Interfacial charge modulation: an efficient strategy for boosting spatial charge separation on semiconductor photocatalysts, Adv. Energy Mater., 2019, 1803951 CrossRef
. - Z. Liu, J. Tian, D. Zeng, C. Yu, W. Huang, K. Yang, X. Liu and H. Liu, Binary-phase TiO2 modified Bi2MoO6 crystal for effective removal of antibiotics under visible light illumination, Mater. Res. Bull., 2019, 112, 336–345 CrossRef CAS
. - Y. Li, T. Liu, T. Li and X. Peng, Hydrothermal fabrication of controlled morphologies of MoO3 with CTAB: Structure and growth, Mater. Lett., 2015, 140, 48–50 CrossRef CAS
. - S. Dhanavel, E. A. K. Nivethaa, K. Dhanapal, V. K. Gupta, V. Narayanan and A. Stephen, a-MoO3/polyaniline composite for effective scavenging of rhodamine B, congo red and textile dye effluent, RSC Adv., 2016, 6, 28871–28876 RSC
. - L. Khandare and D. J. Late, MoO3-rGO nanocomposites for electrochemical energy storage, Appl. Surf. Sci., 2017, 418, 2–8 CrossRef CAS
. - J. Huang, J. Yan, J. Li, L. Cao, Z. Xu, J. Wu, L. Zhou and Y. Luo, Assembled-sheets-like MoO3 anodes with excellent electrochemical performance in Li-ion battery, J. Alloys Compd., 2016, 688, 588–595 CrossRef CAS
. - L. Wang, G. Zhang, Y. Sun, X. Zhou and K. Chou, Preparation of ultrafine β-MoO3 from industrial grade MoO3 powder by the method of sublimation, J. Phys. Chem. C, 2016, 120, 19821–19829 CrossRef CAS
. - R. Pandeeswari and B. G. Jeyaprakash, Nanostructured α-MoO3 thin film as a highly selective TMA sensor, Biosens. Bioelectron., 2014, 53, 182–186 CrossRef CAS PubMed
. - G. P. Nagabhushana, D. Samrat and G. T. Chandrappa, a-MoO3 nanoparticles: solution combustion synthesis, photocatalytic and electrochemical properties, RSC Adv., 2014, 4, 56784–56790 RSC
. - C. I. Fernandes, S. C. Capelli, P. D. Vaz and C. D. Nunes, Highly selective and recyclable MoO3 nanoparticles in epoxidation catalysis, Appl. Catal., A, 2015, 504, 344–350 CrossRef CAS
. - J. Gong, W. Zeng and H. Zhang, Hydrothermal synthesis of controlled morphologies of MoO3 nanobelts and hierarchical structures, Mater. Lett., 2015, 154, 170–172 CrossRef CAS
. - L. Su, Y. Xiong, Z. Chen, Z. Duan, Y. Luo, D. Zhu and X. Ma, MoO3 nanosheet-assisted photochemical reduction synthesis of Au nanoparticles for surface-enhanced Raman scattering substrates, Sens. Actuators, B, 2019, 279, 320–326 CrossRef CAS
. - E. D. B. Santos, F. A. Sigoli and I. O. Mazali, Structural evolution in crystalline MoO3 nanoparticles with tunable size, J. Solid State Chem., 2012, 190, 80–84 CrossRef CAS
. - R. Nadimicherla, W. Chen and X. Guo, Synthesis and characterization of a-MoO3 nanobelt composite positive electrode materials for lithium battery application, Mater. Res. Bull., 2015, 66, 140–146 CrossRef CAS
. - Q. Liu, J. Hu, Y. Liang, Z. C. Guan, H. Zhang, H. P. Wang and R. G. Du, Preparation of MoO3/TiO2 composite films and their application in photoelectrochemical anticorrosion, J. Electrochem. Soc., 2016, 163, C539–C544 CrossRef CAS
. - S. Wang, Y. Zhang, T. Chen and G. Wang, Preparation and catalytic property of MoO3/SiO2 for disproportionation of methyl phenyl carbonate to diphenyl carbonate, J. Mol. Catal. A: Chem., 2015, 398, 248–254 CrossRef CAS
. - T. Yunusi, C. Yang, W. Cai, F. Xiao, J. Wang and X. Su, Synthesis of MoO3 submicron belts and MoO2 submicron spheres via polyethylene glycol-assisted hydrothermal method and their gas sensing properties, Ceram. Int., 2013, 39, 3435–3439 CrossRef CAS
. - S. Alizadeh and S. A. Hassanzadeh-Tabrizi, MoO3 fibers and belts: molten salt synthesis, characterization and optical properties, Ceram. Int., 2015, 41, 10839–10843 CrossRef CAS
. - D. Yan, X. Luo, H. Zhang, G. Zhu, L. Chen, G. Chen, H. Xu and A. Yu, Single-crystalline a-MoO3 microbelts derived from a bio-templating method for superior lithium storage application, J. Alloys Compd., 2016, 688, 481–486 CrossRef CAS
. - A. Chithambararaj, N. S. Sanjini, S. Velmathi and A. C. Bose, Preparation of h-MoO3 and α-MoO3 nanocrystals: comparative study on photocatalytic degradation of methylene blue under visible light irradiation, Phys. Chem. Chem. Phys., 2013, 15, 14761–14769 RSC
. - L. Huang, W. Fang, Y. Yang, J. Wu, H. Yu, X. Dong, T. Wang, Z. Liu and B. Zhao, Three-dimensional MoO3 nanoflowers assembled with nanosheets for rhodamine B degradation under visible light, Mater. Res. Bull., 2018, 108, 38–45 CrossRef CAS
. - Y. Liu, P. Feng, Z. Wang, X. Jiao and F. Akhtar, Novel fabrication and enhanced photocatalytic MB degradation of hierarchical porous monoliths of MoO3 nanoplates, Sci. Rep., 2016, 7, 1845 CrossRef PubMed
. - R. Karthiga, B. Kavitha, M. Rajarajan and A. S. Registrar, Synthesis of MoO3 microrods via phytoconsituents of Azadirachta indica leaf to study the cationic dye degradation and antimicrobial properties, J. Alloys Compd., 2018, 753, 300–307 CrossRef CAS
. - C. Ma, J. Zhou, Z. Cui, Y. Wang and Z. Zou, In situ growth MoO3 nanoflake on conjugated polymer: an advanced photocatalyst for hydrogen evolution from water solution under solar light, Sol. Energy Mater. Sol. Cells, 2016, 150, 102–111 CrossRef CAS
. - J. Zhou, H. Zeng, Y. Wang and Y. Li, H+ intercalation into molybdenum oxide nanosheets under AFM tip bias, Phys. Status Solidi RRL, 2018, 12, 1700439 CrossRef
. - T. H. Chiang and H. C. Yeh, The Synthesis of α-MoO3 by ethylene glycol, Materials, 2013, 6, 4609–4625 CrossRef PubMed
. - G. Zhou, Y. Shan, Y. Hu, X. Xu, L. Long, J. Zhang, J. Dai, J. Guo, J. Shen, S. Li, L. Liu and X. Wu, Half-metallic carbon nitride nanosheets with microgrid mode resonance structure for efficient photocatalytic hydrogen evolution, Nat. Commun., 2018, 9, 3366 CrossRef PubMed
. - J. Yu, S. Zhuang, X. Xu, W. Zhu, B. Feng and J. Hu, Photogenerated electron reservoir in hetero-p–n CuO–ZnO nanocomposite device for visible-light driven photocatalytic reduction of aqueous Cr(VI), J. Mater. Chem. A, 2015, 3, 1199–1207 RSC
. - S. Zhuang, X. Xu, B. Feng, J. Hu, Y. Pang, G. Zhou, L. Tong and Y. Zhou, Photogenerated carriers transfer in dye–graphene–SnO2 composites for highly efficient visible-light photocatalysis, ACS Appl. Mater. Interfaces, 2014, 6, 613–621 CrossRef CAS PubMed
. - N. Qureshi, M. Shinde, S. Arbuj, S. Rane, A. Bhalerao, H.-U. Kim, T. Kim and D. Amalnerkar, Sol–Gel assisted isotropic morphological progression in nanostructured MoO3 and allied investigations on photocatalytic dye-degradation, J. Nanosci. Nanotechnol., 2019, 19, 3479–3486 CrossRef CAS PubMed
. - J. Y. Bae and S. G. Jang, Preparation and Characterization of CuO-TiO2 composite hollow nanospheres with enhanced photocatalytic activity under visible light irradiation, J. Nanosci. Nanotechnol., 2019, 19, 6363–6368 CrossRef PubMed
. - G. Zhou, X. Xu, J. Yu, B. Feng, Y. Zhang, J. Hu and Y. Zhou, Vertically aligned MoS2/MoOx heterojunction nanosheets for enhanced visible-light photocatalytic activity and photostability, CrystEngComm, 2014, 38, 9025–9032 RSC
.
|
This journal is © The Royal Society of Chemistry 2019 |
Click here to see how this site uses Cookies. View our privacy policy here.