DOI:
10.1039/C9RA04926F
(Paper)
RSC Adv., 2019,
9, 28764-28767
N-Heterocyclic carbene copper catalyzed quinoline synthesis from 2-aminobenzyl alcohols and ketones using DMSO as an oxidant at room temperature†
Received
30th June 2019
, Accepted 29th August 2019
First published on 12th September 2019
Abstract
A facile and practical process for the synthesis of quinolines through an N-heterocyclic carbene copper catalyzed indirect Friedländer reaction from 2-aminobenzyl alcohol and aryl ketones using DMSO as an oxidant at room temperature is reported. A series of quinolines were synthesized in acceptable yields.
Introduction
Quinolines are an important class of heterocyclic molecules that are widely found in natural products, especially in alkaloids, and in synthetic pharmacologically active substances, due to their biological activities, such as anticancer, antiviral, antibacterial, antifungal, anti-inflammatory and antiplatelet aggregation.1 For example, cinchophen (A) is a quinoline carboxylic acid used as an analgesic drug, quinoline derivatives exemplified by B could inhibit DNA gyrase in antibacterial tests,2 and quinoline compound C has been developed as a 6-phosphofructo-2-kinase/fructose-2,6-biphosphatase 3 (PFKFB3) inhibitor with an IC50 137 nM for cancer treatment (Fig. 1).3
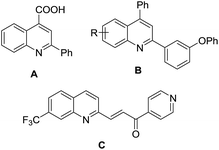 |
| Fig. 1 Examples of bioactive quinolines. | |
In view of the importance of quinolines, great endeavors have been made to develop the procedures for the synthesis of quinoline derivatives.4 The classical strategies include Skraup, Doebner–von Miller, Conrad–Limpach, Pfitzinger, and Friedländer syntheses.5 Among them, Friedländer quinoline synthesis, featuring cyclization of 2-aminobenzaldehydes with ketones promoted by base or acid, is considered to be the simplest method, although it suffers from the drawback that the starting materials 2-aminobenzaldehydes are unstable and easily prone to self-condensation.6 The indirect Friedländer quinoline synthesis is subsequently developed to improve Friedländer synthesis through the oxidative cyclization of 2-aminobenzylic alcohols instead of o-aminobenzaldehydes with ketones or their precursor, alcohols. In general, there are three strategies for the indirect Friedländer quinoline synthesis (Scheme 1): (a) using ketone as the oxidant through Pfitzner–Moffatt oxidation by adding at least 2 equivalent of starting material, ketone7 or another ketone, for example, benzophenone.8 (b) Using oxygen or air as the oxidant at high reaction temperature.9 (c) Through catalytic dehydrogenation, usually under the catalysis of unique metal complex.10
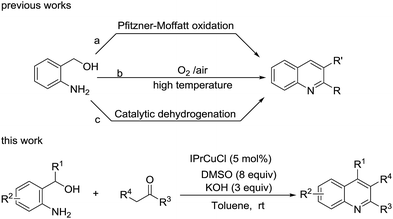 |
| Scheme 1 The synthesis of quinolines from 2-aminobenzylic alcohols. | |
Although these reported processes facilitate alternative for quinolines synthesis, they suffer from the drawbacks such as a waste of starting materials, harsh conditions and/or the need of special catalyst, which is not easy to prepare. Therefore, the development of an alternative method for the indirect Friedländer quinoline synthesis is still in demand. Herein, we report a process for the synthesis of quinoline from 2-aminobenzyl alcohol and aryl ketones under the catalysis of N-heterocyclic carbene copper complex using DMSO as an oxidant at room temperature (Scheme 1).
Results and discussion
Our studies began by optimizing the reaction conditions for the synthesis of quinolines, choosing 2-aminobenzyl alcohol (1a) and acetophenone (2a) as model substrates. The results are collected in Table 1. When this reaction was conducted at room temperature for 6 h under the catalysis of CuCl2 using molecular oxygen as the oxidant in the presence of 3.0 equivalent of KOH in dioxane, which is the solvent usually employed in the oxidative indirect Friedländer quinolines synthesis, trace amount of the desired 2-phenylquinoline (3aa) was detected by GC (Entry 1). The results of screening the conventional solvents suggested that DMSO benefited this transformation most (Entries 2–4). Unexpectedly, this reaction could take place efficiently in the absence of oxygen, and the yield of desired product was further improved by running the reaction in toluene using 8.0 equivalent of DMSO as an oxidant (Entries 5 and 6). When 10 mol% 1,10-phenanthroline was added, the corresponding quinolone was formed with a higher yield (Entry 7). Probably, the ligand could coordinate copper to form in situ a homogeneous copper catalysts, which could prevent the formation of heterogeneous CuO. Encouraged by these promising results, we turned our attention to copper N-heterocyclic carbene complexes in consideration of their stability and activity under basic conditions.11 The testing results suggested that more sterically demanding IPrCuCl was more beneficial to this transformation compared with IMesCuCl (Entries 8 and 9). Catalyst loading was finally investigated. It was found that the yield of 3aa was increased with the increase of copper complex loading and 5 mol% was optimal for this process to synthesize quinolines (Entries 10 and 11). In contrast, the dosage of IPrCuCl must be increased to 15 mol% when toluene, DMSO and KOH were used without drying, for the possible reason that the inclusive water could make the copper complex decompose (Entry 12). Performing this reaction in absence of copper led to a plunge of the yield of expected quinolone (Entry 13), and no corresponding product was isolated with starting materials recovered when this reaction took place without DMSO (Entry 14).
Table 1 Optimizing the reaction conditionsa
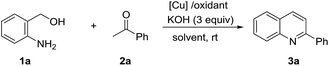
|
Entry |
Catalyst (mol%) |
Oxidant (equiv.) |
Solvent |
Yieldb (%) |
Reaction conditions: 1a (0.5 mmol), 2a (0.5 mmol), KOH (3.0 equiv.), catalyst and oxidant (indicated amount) in 3 mL solvent at room temperature for 12 h. GC yield. IMesCuCl = chloro(1,3-dimesitylimidazol-2-ylidene)copper(I). IPrCuCl = chloro[1,3-bis(2,6-diisopropylphenyl)imidazol-2-ylidene]copper(I). The value in bracket is isolated yield. |
1 |
CuCl2 (5) |
O2 |
Dioxane |
Trace |
2 |
CuCl2 (5) |
O2 |
Toluene |
Trace |
3 |
CuCl2 (5) |
O2 |
CH2Cl2 |
0 |
4 |
CuCl2 (5) |
O2 |
DMSO |
21 |
5 |
CuCl2 (5) |
— |
DMSO |
31 |
6 |
CuCl2 (5) |
DMSO (8) |
Toluene |
43 |
7 |
CuCl2/Phen (5/10) |
DMSO (8) |
Toluene |
54 |
8c |
IMesCuCl (1) |
DMSO (8) |
Toluene |
65 |
9d |
IPrCuCl (1) |
DMSO (8) |
Toluene |
73 |
10 |
IPrCuCl (3) |
DMSO (8) |
Toluene |
86 |
11e |
IPrCuCl (5) |
DMSO (8) |
Toluene |
94 (89) |
12 |
IPrCuCl (15) |
DMSO (8) |
Toluene |
93 |
13 |
— |
DMSO (8) |
Toluene |
20 |
14 |
IPrCuCl (5) |
— |
Toluene |
0 |
The scope of the protocol was further investigated after the optimal reaction conditions were established. 1a was reacted with various ketones under the best reaction conditions, and the results are listed in Table 2. Generally, acetophenones substituted by either electron-donating groups or electron withdrawing groups on the aromatic ring could smoothly go through the cyclization reaction and afforded the corresponding products in moderate to excellent yields (Table 2, 3ba–3ea). The experimental results indicated that steric hindrance of the group on the phenyl ring of acetophenones had a significant effect on the transformation and ortho-substituted acetophenone gave the worst result compared with meta- or para-substituted ones (Table 2, 3ga–3ia). Heteroaryl methyl ketones were found to be good partners of 2-aminobenzyl alcohol, providing the target quinolines in high yields (Table 2, 3ja–3ka). 5, 6-Dihydrobenzo[c]acridine 3la was formed in 86% yield when 2l was subjected to the optimized reaction conditions. However, aryl ketone with a long-chain substituent furnished the desired quinolines in lower yields (Table 2, 3ma).
Table 2 Scope of ketonesa,b

|
Reactions were performed with 1a (0.5 mmol), 2 (0.5 mmol), IPrCuCl (5 mol%), DMSO (8 equiv.) and KOH (3 equiv.) in Toluene (3 mL) at room temperature for 6 h. Isolated yield. |
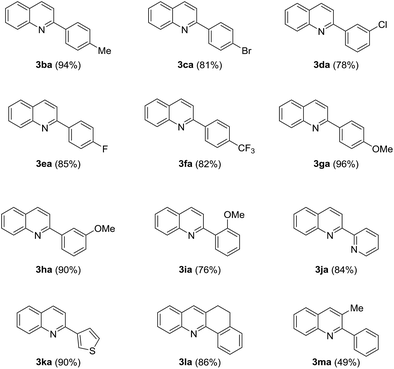 |
A variety of 2-aminobenzylic alcohols were then probed, and the results are summarized in Table 3. We found that 2-aminobenzylic alcohols substituted by electron-donating groups and halogens on the phenyl ring could all undergo the transformation and produce the target compounds in good to excellent yields (Table 3, 3ab–3ag). However, 2-aminobenzylic alcohols whose α-position was substituted by methyl or phenyl performed poorly under the standard conditions, delivering the corresponding products in 19% and 13% yield, respectively. After further optimization, it was found that they could smoothly go across the cyclization reaction in the mixture of DMSO and toluene (2
:
1) and provide the target quinolones in acceptable yields (Table 3, 3ah–3ai), which demonstrated that steric effects of α-substituents of hydroxyls influenced the reaction considerably and a more polar medium benefited this reaction.
Table 3 Scope of 2-aminobenzylic alcoholsa,b
To gain insight into the mechanistic profile, some control experiments were conducted (Scheme 2). When 2-aminobenzyl alcohol (1a) was subjected to the standard conditions in the absence of ketone, 2-aminobenzaldehyde was formed in 20% with a 100% conversion of 1a determined by GC-MS (eqn (1), see ESI†). Under the standard conditions, α-methylbenzyl alcohol was able to react with 2-aminobenzyl alcohol (1a) to provide quinoline 3aa in 83% isolated yield (eqn (2)). The reaction between 2-amino-5-chlorobenzaldehyde and acetophenone (2a) under the standard conditions afforded target quinoline 3ae in 93% yield (eqn (3)).
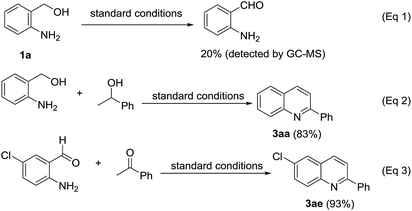 |
| Scheme 2 Control experiments. | |
On the basis of the above mentioned results and relevant reports in the literature, a reasonable reaction pathway for N-heterocyclic carbene copper complex catalyzed indirect Friedländer quinoline synthesis is illustrated in Scheme 3. Firstly, under the catalysis of N-heterocyclic carbene copper complex, 1a is oxidized into 2-aminobenzaldehyde A by DMSO in the presence of KOH.12 Aldol condensation between 2a and A then takes place under a basic condition to furnish an α,β-unsaturated ketone B, which goes through the cyclodehydration reaction to give the final quinoline product.
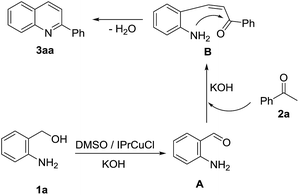 |
| Scheme 3 Possible reaction pathway. | |
Conclusions
In conclusion, we have developed a practical and facile process for the synthesis of quinolines through N-heterocyclic carbene copper complex catalyzed indirect Friedländer reaction from 2-aminobenzylic alcohols and aryl ketones using DMSO as an oxidant at room temperature. This method has broad substrate scope, tolerating aryl chloride, aryl bromide and 2-aminobenzylic alcohols substituted at α-position of hydroxyl.
Conflicts of interest
There are no conflicts to declare.
Acknowledgements
The authors thank the National Natural Science Foundation of China (21572040), Guangdong Natural Science Foundation (2015A030310371) and Scientific Research Foundation of Guangdong Medical University (M2015005) for financial support.
References
-
(a) S. M. Hussaini, Expert Opin. Ther. Pat., 2016, 26, 1201 CrossRef CAS PubMed;
(b) P. Chung, Z. Bian, H. Pun, D. Chan, A. Chan, C. Chui, J. Tang and K. Lam, Future Med. Chem., 2015, 7, 947 CrossRef CAS PubMed.
- M. Alagumuthu and S. Arumugam, Bioorg. Med. Chem., 2017, 25, 1448 CrossRef CAS PubMed.
- P. Chand and G. H. Tapolsky, WO2013148228A1, 2013.
-
(a) C. Li, J. Li, Y. An, J. Peng, W. Wu and H. Jiang, J. Org. Chem., 2016, 81, 12189 CrossRef CAS PubMed;
(b) M. Zhong, S. Sun, J. Cheng and Y. Shao, J. Org. Chem., 2016, 81, 10825 CrossRef CAS PubMed;
(c) H. Wang, Q. Xu, S. Shen and S. Yu, J. Org. Chem., 2017, 82, 770 CrossRef CAS PubMed.
- S. Prajapati, K. Patel, R. Vekariya, S. Panchal and H. Patel, RSC Adv., 2014, 4, 24463 RSC.
- J. Marco-Contelles, E. Pérez-Mayoral, A. Samadi, M. Carreiras and E. Soriano, Chem. Rev., 2009, 109, 2652 CrossRef CAS PubMed.
-
(a) H. V. Mierde, P. V. D. Voort and F. Verpoort, Tetrahedron Lett., 2008, 49, 6893 CrossRef;
(b) Y. Liang, X. Zhou, S. Tang, Y. Huang, Y. Feng and H. Xu, RSC Adv., 2013, 3, 7739 RSC;
(c) Y. Zhu and C. Cai, RSC Adv., 2014, 4, 52911 RSC;
(d) C. Cho, B. Kim, T. Kim and S. Shim, Chem. Commun., 2001, 2001, 2576 RSC;
(e) A. Porcheddu and G. Chelucci, Chem. Rec., 2019, 19, 1 CrossRef.
-
(a) R. Martínez, D. J. Ramón and M. Yus, J. Org. Chem., 2008, 73, 9778 CrossRef PubMed;
(b) R. Martínez, D. J. Ramón and M. Yus, Eur. J. Org. Chem., 2007, 2007, 1599 CrossRef.
-
(a) B. Chen, L. Chng, J. Yang, Y. Wei, J. Yang and J. Ying, ChemCatChem, 2013, 5, 277 CrossRef CAS;
(b) N. Anand, S. Koley, B. J. Ramulu and M. S. Singh, Org. Biomol. Chem., 2015, 13, 9570 RSC;
(c) C. Cho, W. Ren and S. Shim, Tetrahedron Lett., 2006, 47, 6781 CrossRef CAS;
(d) C. Cho, W. Ren and N. Yoon, J. Mol. Catal. A: Chem., 2009, 299, 117 CrossRef CAS;
(e) S. Yao, K. Zhou, J. Wang, H. Cao, L. Yu, J. Wu, P. Qiu and Q. Xu, Green Chem., 2017, 19, 2945 RSC.
-
(a) G. Chakraborty, R. Sikari, S. Das, R. Mondal, S. Sinha, S. Banerjee and N. Paul, J. Org. Chem., 2019, 84, 2626 CrossRef CAS PubMed;
(b) B. Pan, B. Liu, E. Yue, Q. Liu, X. Yang, Z. Wang and W. Sun, ACS Catal., 2016, 6, 1247 CrossRef CAS;
(c) D. Tan, H. Li, D. Zhu, H. Li, D. Young, J. Yao and J. Lang, Org. Lett., 2018, 20, 608 CrossRef CAS PubMed;
(d) G. Zhang, J. Wu, H. Zeng, S. Zhang, Z. Yin and S. Zheng, Org. Lett., 2017, 19, 1080 CrossRef CAS PubMed;
(e) R. Wang, H. Fan, W. Zhao and F. Li, Org. Lett., 2016, 18, 3558 CrossRef CAS PubMed;
(f) M. Maji, K. Chakrabarti, B. Paul, B. C. Roy and S. Kundu, Adv. Synth. Catal., 2018, 360, 722 CrossRef CAS.
-
(a) D. Nelson and S. Nolan, Coord. Chem. Rev., 2017, 353, 278 CrossRef CAS;
(b) F. Lazreg, F. Nahra and C. Cazin, Coord. Chem. Rev., 2015, 293-294, 48 CrossRef CAS.
-
(a) L. Zhan, L. Han, P. Xing and B. Jiang, Org. Lett., 2015, 17, 5990 CrossRef CAS PubMed;
(b) K. Yang and Q. Song, Org. Biomol. Chem., 2015, 13, 2267–2272 RSC.
Footnote |
† Electronic supplementary information (ESI) available. See DOI: 10.1039/c9ra04926f |
|
This journal is © The Royal Society of Chemistry 2019 |