DOI:
10.1039/C9RA05615G
(Paper)
RSC Adv., 2019,
9, 33922-33930
Effective removal of calcium and magnesium sulfates from wastewater in the rare earth industry†
Received
22nd July 2019
, Accepted 10th October 2019
First published on 22nd October 2019
Abstract
The wastewater discharged from the rare earth (RE) industry generally contains a high level of calcium and magnesium sulfates, which confers permanent hardness and causes difficulties in recycling this wastewater. In this study, the alkyl phenoxy acetic acid derivatives including 4-methyl phenoxy acetic acid (M-POAA), 4-tert-butyl phenoxy acetic acid (B-POAA) and 4-tert-octyl phenoxy acetic acid (O-POAA), were synthesized via the Williamson reaction and characterized by nuclear magnetic resonance (NMR), infrared (IR), and ultra-violet (UV) spectroscopy, as well as elemental analysis and X-ray diffraction (XRD). Synthesis of the POAAs were simple and green, and the raw materials used for their production are widely available and low-cost. The potential for removal of Ca and Mg sulfates from industrial wastewater using POAAs as the organic precipitants was assessed. The total precipitation efficiencies of Ca and Mg from wastewater with the use of POAAs increased with the following order: M-POAA < B-POAA < O-POAA. The residual concentrations of Ca and Mg using O-POAA as the precipitant were lower than 0.099 and 0.089 g L−1, respectively. The O-POAA could be regenerated five times without any significant change in its structure and precipitation performance. Thus, the use of the novel precipitants is a prospective alternative to the conventional processes for softening wastewater.
1 Introduction
The unique physical and chemical properties of rare earth (RE) elements make them irreplaceable, as they can be used not only in the traditional fields of metallurgy, agriculture, and ceramics, but also in high-tech fields, such as magnetic materials, catalysts, laser materials and so on.1,2 RE resource are abundant in China, accounting for 30% of the world's reserves where China dominates the world's RE production at 85%.3 However, it is not widely known that mining RE elements discharges considerable amounts of wastewater with high hardness.4 The generation of wastewater containing Ca and Mg during the RE production process is illustrated in the ESI (Fig. S1†). RE sulfates can be obtained after enhanced roasting of a mixture of bastnaesite and monazite with concentrated sulfuric acid and leaching with a large amount of fresh or reused water. The wastewater with Ca and Mg sulfate is generated in the acid/base neutralization and extraction transformation processes. It is reported that the annual discharge of wastewater from RE production in Inner Mongolia, China is about 2.5 million tonnes.5 The wastewater derived from the hydrometallurgical process generally confers permanent hardness and affects the subsequent recycling of the wastewater.6,7 Techniques for eliminating permanent hardness in wastewater are of particular importance. The four main methods for softening hard wastewater and removing Ca and Mg are summarized as follows:
(1) Inhibiting scaling with anti-scalants
Anti-scalants, also called chemical inhibitors, such as sodium tri-polyphosphate, organic phosphoric acid and phosphine-based polyacetic acid, are used to inhibit the growth of scale crystals.8 Studies have shown that anti-scalants can decrease the particle sizes of precipitates and change the shapes of the particles. Smaller particles can cause a greater decline in the microfiltration flux during the solid/liquid separation steps.9 The presence of anti-scalants during precipitation can also decrease the mass of precipitated calcium carbonate.10 With the restrictions on the use of phosphorus chemicals, anti-scalants without phosphorus have been developed, such as polyacrylic acid and polyepoxide succinic acid. However, these kinds of anti-scalants readily generate polymer calcium gels which are difficult to dissolve in water and do not possess scale-inhibiting effects.11
(2) Chemical precipitation method
Chemical precipitation is one of the conventional methods for softening hard water in industrial applications.12,13 Chemical additives such as lime or soda induce the formation of insoluble precipitates that are readily discharged from the water to obtain soft water.14 Taking lime and soda as example, the precipitation reaction can be expressed as eqn (1) and (2). |
MgSO4 + Ca(OH)2 → Mg(OH)2↓ + CaSO4
| (1) |
|
CaSO4 + Na2CO3 → CaCO3↓ + Na2SO4
| (2) |
Considering that the solubility product constant, Ksp, of CaCO3 (8.7 × 10−9) is far less than that of MgCO3 (2.6 × 10−5), Ca2+ is more easily precipitated than Mg2+ in carbonate medium.15 One study reported that the use of 12% ammonia water as the precipitant to remove Ca and Mg resulted in removal efficiencies of 75 and 52%, respectively.16
Oxalic acid saponified with an alkali can also be used to soften high-hardness wastewater, where Ca2+ can be precipitated and a small amount of Mg2+ is co-precipitated when oxalic acid used as the precipitant. The particle sizes of CaC2O4 or MgC2O4 were extremely small, resulting in difficulty of the solid/liquid separation. Another disadvantage of this method is that oxalic acid is highly water soluble (9.52 g/100 g water), leading to a high residual concentration in the aqueous phase. Oxalic acid and the oxalates exert biological toxicity with harmful effects upon contact. The reported oral lowest published lethal dose (LDLO) of oxalic acid was 600 mg kg−1.17 The precipitation reactions of Ca and Mg with oxalates are presented in eqn (3) and (4).
|
CaSO4 + Na2C2O4 → CaC2O4↓ + NaSO4
| (3) |
|
MgSO4 + Na2C2O4 → MgC2O4↓ + NaSO4
| (4) |
As is evident from eqn (5) and (6), the precipitation of magnesium ammonium phosphate (MAP, MgNH4PO4·6H2O) from wastewater has attracted considerable attention as this technique offers the potential for recovering phosphorus for fertilization.18 The MAP crystallization process includes nucleation and crystal growth processes that are affected by physicochemical factors such as the pH, super saturation ratio, temperature, and the presence of foreign ions.19,20 It is a promising method for removing Ca and Mg from wastewater based on the low Ksp value of MAP (2.5 × 10−13).21,22
|
3Ca2+ + 2PO43− → Ca3(PO4)2↓
| (5) |
|
Mg2+ + NH4+ + PO43− → MgNH4PO4↓
| (6) |
(3) Membrane separation
Membrane separation methods including reverse osmosis (RO), ultrafiltration (UF), nanofiltration (NF) and so on have been used for several years to remove suspended particles or dissolved salts from aqueous media.23–25 This method not only removes calcium and magnesium ions, but also intercepts other inorganic salts, carbohydrates, amino acids, etc., in wastewater. The radii of intercepted particles or salts are greater than 1 nm and the intercept rates are as high as 10–90%.
(4) Ion exchange
Ion exchange resins are frequently used to remove ions such as Ca and Mg from aqueous media. It is reported that the hardness of the industrial wastewater from Inner Mongolia, China was reduced from 5.42 × 103 to 71.2 mg L−1 with a total removal efficiency of 98%.26
Recently, mono-substituted alkylphenoxy acetic acid derivatives (POAAs) were reported.27–29 The former can be used for the separation of high-purity yttrium from RE mixture,30 whereas the latter plays an important role in the enrichment and transformation of RE elements.31,32 To the authors' knowledge, the coordination characteristics of Ca and Mg ions with carboxyl groups are similar to those of RE ions. To date, the study of precipitation of Ca and Mg using POAAs has not been reported based on a literature survey. The objectives of this study are to synthesize mono-substituted alkyl phenoxy acetic acids including 4-methyl phenoxy acetic acid (M-POAA), 4-tert-butyl phenoxy acetic acid (B-POAA) and 4-tert-octyl phenoxy acetic acid (O-POAA) and to discuss the application to the selective precipitation of Ca and Mg sulfates from wastewater in RE industry.
2 Experimental
2.1 Chemical reagents and wastewater samples
4-Methyl-octylphenol, 4-tert-butyl-octylphenol, 4-tert-octylphenol, chloroacetic acid, dimethyl sulfoxide (DMSO) and NaOH were purchased from Chendu Xiya Reagent Chemical Technology Co., Ltd. Concentrated hydrochloric acid, oxalic acid, calcium hydroxide, sodium phosphate, ammonium chloride, calcium sulfate and magnesium sulfate were purchased from Sino-pharm Chemical Reagent Co., Ltd., China. All other chemicals were used without further purification.
Real wastewater sample was collected from the RE industry in Inner Mongolia, China. The typical compositions of the wastewater are listed as follows, CaSO4 0.0113 mol L−1, MgSO4 0.124 mol L−1 and (NH4)2SO4 0.325 mol L−1. In order to reduce the influence of impurities, the synthetic wastewater samples containing CaSO4, MgSO4 and (NH4)2SO4 were prepared and used in the parts of mechanism research.
2.2 Apparatus and analytical methods
Elemental (C, H and N) analysis of the organic compounds was performed using an Elementar Vari EL Cube. Inductively Coupled Plasma Optical Emission Spectroscopy (ICP-OES) was performed with a JY-Horiba Ultima 2 instrument to determine the concentrations of Ca2+ and Mg2+. Proton and carbon nuclear magnetic resonance (1H and 13C NMR) spectra were obtained with an AV III-500 Bruker spectrometer. Scanning electron microscope (SEM) images were collected on a Hitachi SU1510 instrument at 30 kV. Particle sizes were measured with the aid of the back-scattering principle by Brookhaven NanoBrook Omni, USA. Fourier-transform infra-red (FT-IR) spectra were obtained by using a Nicolet iS50 spectrometer (Thermo Scientific) in the range of 600–4000 cm−1.
The method for analysis of the trace POAAs in the aqueous phase was developed based on the characteristic ultraviolet spectral absorption of the benzene ring in the POAA molecule. There is a quantitative relationship between the concentration of the POAA and the ultraviolet spectral absorbance in the wavelength range of 274–276 nm (ESI, Fig. S2†). The logarithmic acid dissociation constant (pKa) and purity of the POAA can be determined by acid–base titration in 75 vol% alcohol using 0.01 mol L−1 NaOH as the standard solution and 0.5 vol% bromothymol blue as the indicator.33 The water content of solid complexes was determined from the weight change before and after drying at 100 °C for 5 h.
2.3 Synthesis and characterization of POAAs
Molecular structures of M-, B- and O-POAA are illustrated in Fig. 1. Crystal data and structure refinement for M-POAA (CCDC 1105571), B-POAA (CCDC 1844628) and O-POAA (CCDC 1587656) are available (ESI, Table S1†). The compounds were prepared in laboratory scale via Williamson reaction and the synthetic routes are as following: (1) 0.2 mol 4-methyl/4-tert-butyl/octyl phenol, 0.21 mol chloroacetic acid, 0.41 mol NaOH were mixed together in the solvent dimethyl sulfoxide (DMSO). The mixture was stirred at 110 °C for 2 h and a large amount of white solid phase was formed. A certain amount of NaOH was added to control pH > 11 throughout the reaction. (2) The resultant solution was sequentially neutralized with 6.0 mol L−1 HCl, extracted with mineral ether, washed with deionized water, and evaporated on a rotary evaporator. (3) The products were further purified by recrystallization in high purity toluene.
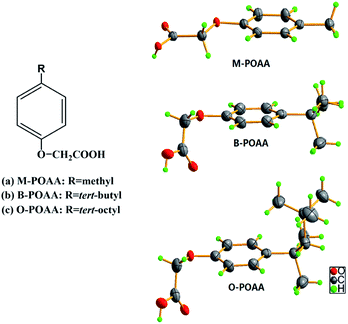 |
| Fig. 1 Molecular structures of 4-methyl phenoxy acetic acid (M-POAA), 4-tert-butyl phenoxy acetic acid (B-POAA), and 4-tert-octyl phenoxy acetic acid (O-POAA). | |
As for a large-scale preparation, the irritating solvent DMSO can be omitted without distinct influence of the final yield of product, which leads to the synthetic routes relatively simple and green. The raw material including 4-methyl/4-tert-butyl/octyl phenol, chloroacetic acid and NaOH, of POAAs have a wide range of sources and low production costs. Thus, the POAAs are suitable for large-scale industrial usage.
4-Methyl phenoxy acetic acid (M-POAA). Final yield 95%. Purity > 99% (acid–base titration method). 1H NMR (500 MHz, DMSO) δ 12.97 (s, 1H), 7.08 (d, J = 8.2 Hz, 2H), 6.85–6.75 (m, 2H), 4.62 (s, 2H), 2.23 (s, 3H). 13C NMR (125 MHz, DMSO) δ 170.79, 156.10, 130.13, 130.09, 114.68, 64.95, 20.52. C9H10O3(166.18): found (anal. calc.)%, C 64.91(65.05), H 6.07(6.07). Water solubility 1.51 g L−1 at 25 °C (UV-Vis Spectrophotometry). UV characteristic peak at 276 nm. pKa = 5.05.
4-tert-Butyl phenoxy acetic acid (B-POAA). Final yield 90%. Purity > 99% (acid–base titration method). 1H NMR (500 MHz, DMSO) δ 12.98 (s, 1H), 7.29 (d, J = 8.9 Hz, 2H), 6.85–6.79 (m, 2H), 4.63 (s, 2H), 1.25 (s, 9H). 13C NMR (125 MHz, DMSO) δ 170.82, 155.94, 143.58, 126.51, 114.29, 64.90, 34.24, 31.79. C12H16O3 (208.26): found (anal. calc.)%, C 69.50(69.21), H 7.92(7.74). Water solubility 0.872 g L−1 at 25 °C. UV characteristic peak at 274 nm. pKa = 5.07.
4-tert-Octyl phenoxy acetic acid (O-POAA). Final yield 83%. Purity > 99% (acid–base titration method). 1H NMR (500 MHz, DMSO) δ 0.68 (s, 9H), 1.30 (s, 6H), 1.69 (s, 2H), 4.62 (s, 2H), 6.89 (d, J = 8.7 Hz, 2H), 7.27 (d, J = 8.6 Hz, 2H), 12.95 (s, 1H); 13C NMR (125 MHz, DMSO) δ 32.0, 32.1, 32.5, 38.1, 56.8, 64.9, 114.0, 127.3, 142.4, 155.8, 170.8. C16H24O3 (264.36): found (anal. calc.)%, C 72.70(72.69), H 9.25(9.15). Water solubility 33.3 mg L−1 at 25 °C. UV characteristic peak at 275 nm. pKa = 4.88.
2.4 Precipitation and stripping procedure
The precipitation experiments were carried out by mixing the saponified organic precipitants and Ca/Mg solution together to form a solid complex phase at room temperature. The detailed precipitation routes are as follows: (1) precipitants were saponified with 5.0 mol L−1 NaOH which transformed the solid precipitant into a transparent suspension. (2) Solid complexes containing Ca and Mg were formed by adding the saponified precipitants to aqueous phase. The solid complex was separated from the aqueous phase with liquid/solid centrifugal separator. The saponification degree can be defined as the proportion of compounds saponified into mono-carboxylate relative to the total amount of the compounds. The mixture was centrifuged or filtered, and the solid phase was separated from the liquid phase. In order to make the precipitants loaded with Ca and Mg recyclable, the stripping experiments were conducted by immersing the solid complex in a series of concentrations of hydrochloric acid for 0.5 h. To ensure data reliability, the experiments were repeated at least twice. The precipitation rate (P), distribution ratio (D), separation factor (β) and stripping rate (S) are defined as follows: |
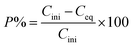 | (7) |
|
 | (8) |
|
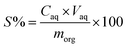 | (10) |
where Cini and Ceq are the initial and equilibrium concentration of the metal in the aqueous phase, respectively. D1 and D2 represent the distribution ratio of metals 1 and 2, respectively. Caq is the metal concentration in the stripping section. Vaq and morg represent the volume of the aqueous phase in the stripping section and the initial mass of metal in the solid complex, respectively.
3 Results and discussion
3.1 Comparison of oxalic acid and POAAs
As mentioned above, oxalic acid can be used for soften wastewater with high hardness. However, the precipitant cannot be reused for the next time. In this paper, we designed the precipitation strategy as follows. Firstly, the novel precipitants were saponified into anionic surfactants with alkali, such as sodium hydroxide and ammonia, which could be contacted with the aqueous phase thoroughly. Secondly, insoluble solid complexes containing Ca and Mg sulphates were formed by adding the saponified precipitants into wastewater with high hardness. Therefore, Ca and Mg were transferred from aqueous phase into solid phase. Solid complexes could be separated from wastewater easily with liquid/solid centrifugal separator, plate frame filter, or other filter equipment, leaving water with low hardness. Finally, the precipitants were designed to be regenerated by the stripping of solid complexes with concentrated HCl. Thus, low concentration of slightly soluble Ca and Mg sulphates transitioned to high concentration of soluble Ca and Mg chloride.
Total precipitation efficiency of Ca and Mg sulphates with oxalic acid and POAAs were compared. As can be seen in Fig. 2, POAAs indicates better precipitation abilities for the Ca and Mg sulphates than oxalic acid. With the increase of alkyl chain carbon atoms in POAAs, the precipitation rates of Ca and Mg sulphates increased. The results indicate that the total precipitation rates of Ca and Mg sulphates from hardness wastewater reached 97.7% by the use of O-POAA and the residual concentration of Ca and Mg sulphates were lower than 27.8 and 15.6 mg L−1, respectively. Total precipitation efficiency of Ca and Mg sulphates with oxalic acid reached only 58.1%.
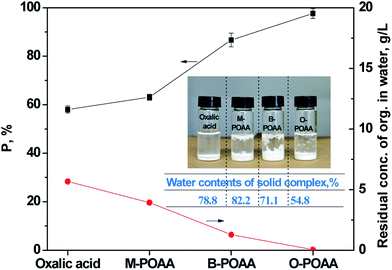 |
| Fig. 2 Total precipitation efficiency of Ca and Mg with oxalic acid, M-, B- and O-POAA and residual concentration of organic precipitants in aqueous phase. Aqueous phase: CaSO4 = 0.0141 mol L−1, MgSO4 = 0.0434 mol L−1, VFeed = 20.0 mL. Solid phase: oxalic acid = 0.0155 mol, M-POAA = B-POAA = O-POAA = 0.00310 mol, saponification degree = 80%. | |
Residual concentration of organic precipitants in aqueous phase during the precipitation process have also been investigated. As the simplest dicarboxylic acid, oxalic acid is the organic compound with the saturated solubility in water of 143 g/100 g (20 °C).34 In the current precipitation process, residual concentration of oxalic acid reached 5.67 g L−1. With the increase of alkyl chain carbon atoms in POAAs, the residual concentration in water significantly decreased. The solubility order of M-POAA, B-POAA and O-POAA at room temperature follows the reverse sequence as, M-POAA (3.93 g L−1) > B-POAA (1.28 g L−1) > O-POAA (0.033 g L−1). Water contents of solid complexes including oxalic acid and POAA complexes were tested as 78.8, 82.2, 71.1 and 54.8%, respectively. It can be explained that, with the increase of the alkyl chain in the molecular structure, the hydrophobicity of the molecule is increased. Correspondingly, lower water content can lead to the faster separation of solid complexes from aqueous phase.
To evaluate the precipitation abilities and selectivities using M-, B- and O-POAA, their precipitation rates for Ca and Mg ions were compared. As shown in Table 1, oxalic acid reveals better precipitation abilities for Ca than Mg with the precipitation rates of 95.8% and 45.8%, respectively. The separation factor between Ca and Mg, βCa/Mg, can be deduced as 26.9, correspondingly. This can be explained that, the solubility product constant, Ksp, of CaC2O4 (2.57 × 10−9) is far less than that of MgC2O4 (4.83 × 10−6). In a diametrically opposite way, O-POAA indicates better precipitation abilities for the Mg than Ca and demonstrated βCa/Mg value of 0.293. Comprehensive considering the maximum precipitation rate, minimum water content, we can assume that O-POAA among POAAs might be used in parallel to remove Ca and Mg from wastewater streams.
Table 1 Precipitation rate of mixed Ca and Mg ions with oxalic acid, M-, B- and O-POAA. CaSO4 = 0.0141 mol L−1, MgSO4 = 0.0434 mol L−1, VFeed = 20.0 mL. Solid phase: oxalic acid = 0.0155 mol, M-POAA = B-POAA = O-POAA = 0.00310 mol, saponification degree = 80%
Precipitants |
P% |
D |
βCa/Mg |
Ca |
Mg |
Ca |
Mg |
Oxalic acid |
95.8 |
45.8 |
22.7 |
0.844 |
26.9 |
M-POAA |
63.1 |
63.0 |
1.72 |
1.70 |
1.01 |
B-POAA |
92.8 |
84.7 |
12.8 |
5.54 |
2.32 |
O-POAA |
95.1 |
98.5 |
19.3 |
65.8 |
0.293 |
3.2 Precipitation mechanism
Unlike oxalic acid, the POAAs are completely non-dissociated in water and the precipitation reactions between POAA and Ca/Mg sulfates cannot be directly carried out through direct contact. As the typical weak mono acids, logarithmic acid dissociation constant, pKa, values of M-, B- and O-POAA were determined as 5.05, 5.07 and 4.88 in 75 vol% ethanol, respectively, much lower than first-order dissociation of oxalic acid (pKa1 = 1.2). The structures of the POAAs showed dimer compounds via XRD single crystal characterization (Bruker D8 Venture diffractometer). In the solvent extraction system, the acidic extractants were diluted in inert solvents to reduce the dimerization tendency. In the current precipitation strategy, quantitative precipitation of Ca and Mg could be achieved when the POAAs were saponified by acid–base neutralization reactions to break the dimeric connections between the molecules.
Taking O-POAA as an example, the effect of saponification degree on the precipitation of Ca and Mg was investigated and the results are shown in Fig. 3. The precipitation efficiencies of Ca and Mg with the POAA increased quantitatively with an increase in the degree of saponification. In addition, the formation of the solid complex, ΔM2+ (M = Ca or Mg), and the reduction of the H+ concentration in the solid precipitants, ΔH+, were calculated; the results are also shown in Table 2. The ratio of ΔH+/ΔM2+ remained at about 2 for different saponification degrees, which means that the precipitation efficiencies of Ca or Mg was quantitative under the current saponification conditions. The water solubility of O-POAA was determined as 33.3 mg L−1 at 25 °C. With an increase in saponification degrees during the precipitation processes, the water solubility of O-POAA increased slightly. The saponification and precipitation reactions can be expressed in terms of the following equations:
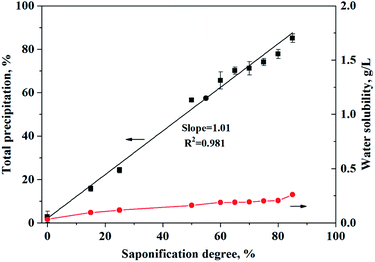 |
| Fig. 3 The effect of saponification degree on the precipitation of Ca and Mg with O-POAA. Synthetic wastewater sample: CaSO4 = 0.00951 mol L−1, MgSO4 = 0.0223 mol L−1, (NH4)2SO4 = 0.0584 mol L−1, VFeed = 24.0 mL, pH = 6. Solid phase: O-POAA = 0.00154 mol, saponification degree = 0–85%. | |
Table 2 The relationship between the formation of solid complex, ΔM2+, and the reduction of H+ in the solid precipitants, ΔH+
Sap. rate% |
ΔH+ × 10−4, mol |
ΔM2+ × 10−4, mol |
ΔH+/ΔM2+ |
0 |
0 |
0 |
N/A |
15 |
2.31 |
1.20 |
1.92 |
25 |
3.85 |
1.85 |
2.08 |
50 |
7.70 |
4.32 |
1.78 |
60 |
9.24 |
5.01 |
1.85 |
65 |
10.0 |
5.35 |
1.87 |
70 |
10.8 |
5.44 |
1.98 |
75 |
11.6 |
5.65 |
2.04 |
80 |
12.3 |
5.94 |
2.08 |
85 |
13.1 |
6.49 |
2.02 |
The total reaction can be represented as:
|
M2+ + 2HL + OH− → ML2↓ + H2O
| (13) |
Therefore, the precipitation of Ca and Mg with POAA proceeds via a cation exchange mechanism. In practical operation, the saponification degree of the precipitants and the acidity of the aqueous phase are the key factors that impact the effectiveness of the precipitants and the precipitation efficiencies of Ca and Mg, respectively.
3.3 Temperature effects
In earlier publications, temperature was found to affect the equilibrium of the extraction reaction.35 Increasing the temperature should result in an increase in the reaction rate in many cases. In industrial production, extraction and separation are generally carried out at temperatures above 15 °C. However, the effects of temperature on the precipitation rates of Ca and Mg have seldomly been reported. The effect of temperature on the precipitation of Ca and Mg with O-POAA was thus investigated. As shown in Fig. 4, the total precipitation rate decreased slightly with an increase in the temperature from 5 °C to 65 °C, indicating the insignificant effect of temperature on Ca and Mg precipitation. However, with an increase in the temperature during the precipitation processes, the water solubility of O-POAA obviously increased. The recommended working temperature is below 35 °C, where the water solubility of the precipitant can be controlled to below 242 mg L−1. This precipitation method is especially suitable for cold areas with annual temperatures below 35 °C.
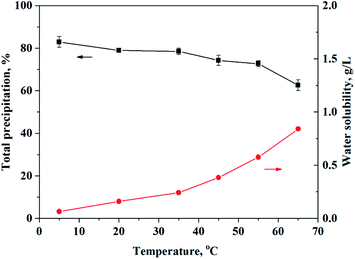 |
| Fig. 4 The effect of temperature on the precipitation of Ca and Mg with O-POAA. Synthetic wastewater sample: CaSO4 = 0.00945 mol L−1, MgSO4 = 0.0226 mol L−1, (NH4)2SO4 = 0.0586 mol L−1, VFeed = 24.0 mL, pH = 6. Solid phase: O-POAA = 0.00154 mol, saponification degree = 80%. | |
3.4 Stripping studies
Conventional precipitating agents, i.e. oxalic acid or a mixture of NH4Cl and Na3PO4, cannot be recycled for Ca and Mg precipitation. The definite advantage of this method is the possibility of reducing the production cost by recycling the precipitant. Therefore, stripping Ca and Mg from the loaded solid complex using hydrochloric acid is the key step to achieving recyclability of the precipitant. The effect of HCl concentration on the stripping of Ca and Mg from the loaded O-POAA was investigated as shown in Fig. 5. When the concentration of HCl exceeded 1.2 and 2.4 mol L−1, stripping efficiencies of Ca and Mg from the loaded solid complex could be achieved as 94.5 and 99.7%, respectively. The water solubility of POAA during the stripping step was lower than 31.7 mg L−1 when [HCl] > 1.2 mol L−1. O-POAA could be regenerated after the stripping steps and there was no significant change in its precipitation performance.
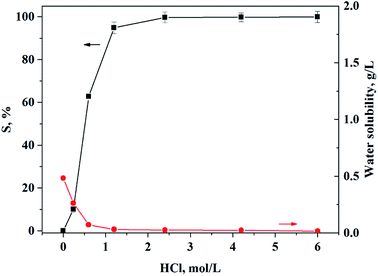 |
| Fig. 5 The effect of HCl concentration on the stripping of Ca and Mg from loaded POAA. Aqueous phase: VHCl = 6.0 mL, HCl concentration = 0.24–6.0 mol L−1. Solid phase: 2.0 g O-POAA loaded with 0.000302 mol Ca and 0.00278 mol Mg. | |
3.5 Pilot tests
There are abundant RE resources in Bayan Obo in Inner Mongolia, China. However, the industrial production of REs, including the decomposition of bastnaesite and monazite concentrates, separation of the RE elements, and manufacture of high-purity RE products requires the use of a large amount of chemicals, resulting in a large amount of waste gas, wastewater and radioactive residue, which are serious threats to the environment. In order to further recycle the ammonium sulfate wastewater from the process of RE smelting, the ammonium sulfate wastewater can be mixed with Ca(OH)2 and more than 17% of the ammonia can be obtained in the distillation column. If the content of Ca and Mg is too high, this will directly affect the recycling of wastewater.
Pilot tests were carried out to verify the efficacy of current the precipitation strategy for the removal of high-concentration Ca and Mg from wastewater in the RE industry (process-I); the flow chart presented in Fig. 6. The residual concentration of Ca and Mg in the treated water (process-I) were lower than 0.099 and 0.089 g L−1, respectively, satisfying the requirements of local RE production enterprises (Baotou Iron and Steel Co., Ltd., Ca < 0.1 g L−1, Mg < 0.5 g L−1). The precipitants were regenerated by stripping the solid complexes with 6 mol L−1 HCl. The mass-to-volume ratio of the solid phase and aqueous phase was 1
:
1. The total concentration of 0.656 mol L−1 CaCl2 + MgCl2 discharged from the regeneration process can be locally used as snow melting agent after further purification.
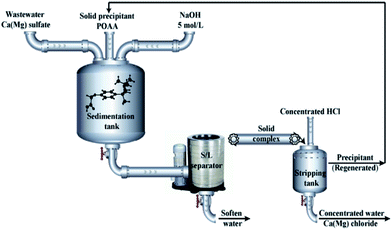 |
| Fig. 6 Process flow chart used for the removal of Ca and Mg sulfates with POAA from wastewater in RE industry. | |
For comparison, the precipitation of Ca and Mg from wastewater with oxalic acid was also evaluated (process-II). Detailed material balance calculations of Ca and Mg are presented in Table 3. The residual concentration of Ca and Mg in the treated water (process-II) were determined as 0.0981 and 2.41 g L−1, respectively. Thus, the residual Mg from process-II does not meet the requirements of the local RE production enterprises.
Table 3 Material balance calculation of Ca and Mg during the current precipitation strategy. The molecular weight of Mg (24) is set to that of Ca (40) according to the definition of the hardness of water. (a) Process-I. O-POAA = 0.0742 mol, saponification degree = 80%. Real wastewater sample: CaSO4 = 0.0113 mol L−1, MgSO4 = 0.124 mol L−1, (NH4)2SO4 = 0.325 mol L−1, VFeed = 200 mL. Mass-to-volume ratio of solid and aqueous phase = 1
:
1 in stripping section. (b) Process-II. Oxalic acid = 0.0371 mol. (c) Process-III. Ca(OH)2 + Na2CO3 = 0.0810 mol. (d) Process-IV. Na3PO4 + NH4Cl = 0.0720 mol
(a) |
Procedure |
Ca + Mg, mol L−1 |
V, mL |
Material balance% |
Precipitation/stripping efficiency% |
Precipitation section |
Feed |
0.135 |
200 |
(100) |
95.4 |
|
Raffinates |
0.00620 |
200 |
4.60 |
Stripping section |
Solid complex |
0.586 |
44.1 |
(100) |
101.9 |
|
Stripping solution |
0.656 |
40 |
101.9 |
(b) |
Procedure |
Ca + Mg, mol L−1 |
V, mL |
Material balance% |
Precipitation efficiency% |
Precipitation section |
Feed |
0.135 |
200 |
(100) |
24.0 |
Raffinates |
0.103 |
200 |
76.0 |
(c) |
Procedure |
Ca + Mg, mol L−1 |
V, mL |
Material balance% |
Precipitation efficiency% |
Precipitation section |
Feed |
0.132 |
200 |
(100) |
37.6 |
Raffinates |
0.0824 |
200 |
62.4 |
(d) |
Procedure |
Ca + Mg, mol L−1 |
V, mL |
Material balance% |
Precipitation efficiency% |
Precipitation section |
Feed |
0.132 |
200 |
(100) |
60.1 |
Raffinates |
0.0528 |
200 |
39.9 |
The morphologies and sizes of the particles of the POAA complexes and Ca + Mg C2O4 produced in process I and II were measured, and the results are shown in Fig. 7. The figure shows the surface morphologies of POAA-Ca(Mg) with particle sizes of about 50 μm. In the case of Ca(Mg)C2O4, particles with sizes of 2–5 μm accounted for only 1/10 or lower of the proportion. The larger particle size of POAA-Ca(Mg) significantly facilitated separation of the solid phase from the liquid phase.
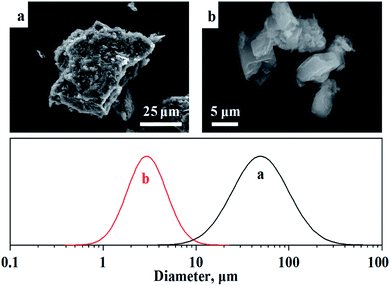 |
| Fig. 7 Particle morphologies and sizes of the solid precipitates. (a) POAA complexes loaded with Ca and Mg in process I. (b) Ca + Mg C2O4 produced in process II. | |
Further experiments were carried out to remove Ca and Mg from wastewater with Ca(OH)2 + Na2CO3 (process-III) and Na3PO4 + NH4Cl (process-IV). The total precipitation efficiencies of Ca and Mg were tested as 37.6 and 60.1% in process III and IV, respectively.
POAA was recycled five times on the basis of process I, which lasted for one month, and the result is shown in Fig. 8. The total precipitation efficiency of Ca and Mg was above 95% and the precipitant concentration in the treated (softened) wastewater was below 37.5 mg L−1. The average weight loss of POAA in the regeneration process was determined as 3.9%. Original POAA (a) and POAA regenerated five times (b) were compared after the stripping steps and the results are shown in Fig. 9. Elemental analysis of the original and recycled POAA, analyzed using an Elementar Vario Micro Cube instrument showed absolute discrepancies of less than 0.4% between the experimental and calculated values. IR, UV and XRD characterization showed that the crystal structure, infrared and UV spectra of the original sample were all consistent with that of the recycled sample. The acid values were determined by acid–base titration and the purity of the original and reused samples was consistent. There is a slight difference in the chromatic properties between (a) and (b). In summary, there was no significant change of the precipitant before and after regeneration. Bio-toxicological evaluation of POAA was also carried out, and the median lethal dose, LD50, was determined as 3160 mg kg−1, which means that POAA can be classified as mildly toxic (500 mg kg−1 < LD50 < 5000 mg kg−1).
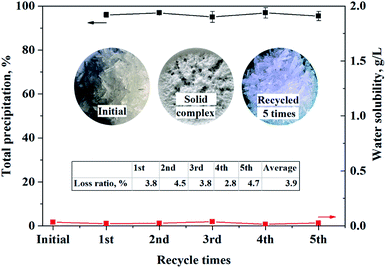 |
| Fig. 8 Comparison of (a) the initial O-POAA and (b) regenerated O-POAA for five times. Real wastewater sample: CaSO4 = 0.0113 mol L−1, MgSO4 = 0.124 mol L−1, (NH4)2SO4 = 0.325 mol L−1, VFeed = 200 mL. Solid phase: O-POAA = 0.0742 mol, saponification degree = 80%. Mass-to-volume ratio of solid and aqueous phase = 1 : 1 in stripping section. | |
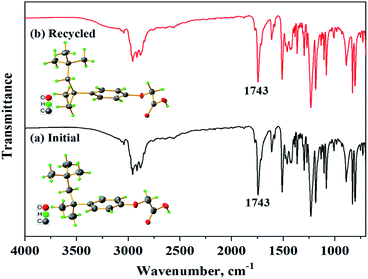 |
| Fig. 9 IR and XRD characterization of (a) the initial O-POAA and (b) regenerated O-POAA for five times. | |
This method is also applicable to high hardness wastewater produced in other regions or other fields containing calcium sulfate and magnesium sulfate, whether or not containing ammonium sulfate. Because the residual concentration of POAA in the softened water is about 20–30 mg L−1, this method is not suitable for softening of drinking water.
4 Conclusions
In this study, alkylphenoxy acetic acid (POAA) derivatives were determined to be effective for softening (removing the permanent hardness of) wastewater from the RE industry. The hardness was controlled by precipitating Ca and Mg sulfates from the wastewater as insoluble solid complexes via the addition of POAA. In a sense, this precipitation method is an extraction method without a diluent. Pilot tests were carried out using wastewater samples collected from the RE industry in Inner Mongolia, China as aqueous phase and using saponified 4 -tert-octyl phenoxy acetic acid (O-POAA) as the precipitant, demonstrating its superiority to oxalic acid. Insoluble solid complexes containing Ca and Mg were formed with a total precipitation efficiency exceeding 95%. The residual concentration Ca and Mg in the treated water were lower than 0.099 and 0.089 g L−1, respectively, satisfying the requirements of local RE production enterprises. The precipitant can be regenerated by stripping the solid complexes and there was no significant change in its performance based on characterization by elemental analysis, IR, XRD, UV, acid–base titration and chromometry. In view of the low-cost and easy synthesis of this precipitant, large-scale application in the removal of Ca and Mg sulfates from wastewater generated by the RE industry in Inner Mongolia, China is anticipated, and the problem of the water shortage in the area is expected to be effectively alleviated.
Conflicts of interest
There are no conflicts to declare.
Acknowledgements
This work was supported by National Key R&D Program of China (2017YFE0106900), Science and Technology Major Projects of Fujian Province (2015HZ0001-3), Natural Science Foundation of Fujian Province (2016J05058), ‘Hundreds of Talents Program’ and Science and Technology Service Network Initiative from Chinese Academy of Sciences.
References
- G. X. Xu, The Rare Earths, Metallurgical Industry Press, Beijing, 1995 Search PubMed.
- S. Riaño, M. Petranikova, B. Onghena, T. Vander Hoogerstraete, D. Banerjee, M. R. S. Foreman, C. Ekberg and K. Binnemans, RSC Adv., 2017, 7, 32100–32113 RSC.
- S. Maes, W. Q. Zhuang, K. Rabaey, C. L. Alvarez and T. Hennebel, Environ. Sci. Technol., 2017, 51, 1654–1661 CrossRef CAS.
- Y. Fujita, J. Barnes, A. Eslamimanesh, M. M. Lencka, A. Anderko, R. E. Riman and A. Navrotsky, Environ. Sci. Technol., 2015, 49, 9460–9468 CrossRef CAS PubMed.
- L. Liu, J. Xie, H. Li, L. Y. Liu, S. C. Hou, R. Sa and L. J. Yao, Hydrometall. China, 2015, 34, 242–244 CAS.
- Q. Q. Lin, G. H. Gu, H. Wang, C. Q. Wang, Y. C. Liu, R. F. Zhu and J. G. Fu, Trans. Nonferrous Met. Soc. China, 2016, 26, 1118–1125 CrossRef CAS.
- Y. H. Xu, G. Tian, Y. J. Wang, X. X. Zhang and S. F. Ma, Chin. Pat., CN201410538625, 2014.
- F. E. Heakal, S. K. Attia, S. A. Rizk, M. A. Abou and A. E. Elkholy, J. Mol. Struct., 2017, 1147, 714–724 CrossRef.
- L. F. Greenlee, F. Testa, D. F. Lawler, B. D. Freeman and P. Moulin, Water Res., 2010, 44, 2957–2969 CrossRef CAS.
- H. L. Yan and K. Shih, Water Res., 2016, 95, 310–318 CrossRef CAS.
- Z. H. Shen, J. L. Shi, S. Z. Zhang, J. Fan and J. S. Li, Water Sci. Technol., 2017, 75, 255–262 CrossRef CAS.
- E. V. Musvoto, M. C. Wentzel and G. A. Ekama, Water Res., 2000, 34, 1868–1880 CrossRef CAS.
- J. Pakarinen and E. Paatero, Miner. Eng., 2011, 24, 1421–1429 CrossRef CAS.
- K. M. Christian, T. Stephan, F. A. Xavier and D. J. Batstone, Water Res., 2015, 85, 359–370 CrossRef.
- J. Leentvaar and M. Rebhun, Water Res., 1982, 16, 655–662 CrossRef CAS.
- Y. Q. Hu, L. G. Wu, X. Q. Ye, C. J. Wu and D. M. Xue, Technology of Water Treatment, 2005, 31, 61–62 CAS.
- B. R. Von, J. Appl. Toxicol., 1994, 14, 233–237 CrossRef.
- H. M. Huang, J. H. Liu, J. Xiao, P. Zhang and F. M. Gao, ACS Sustainable Chem. Eng., 2016, 4, 3688–3696 CrossRef CAS.
- L. Birnhack, O. Nir, M. Telzhenski and O. Lahav, Environ. Technol., 2015, 36, 1892–1901 CrossRef CAS.
- E. Kirinovic, A. R. Leichtfuss, C. Navizaga, H. Y. Zhang, J. D. Schuttlefield Christus and J. Baltrusaitis, ACS Sustainable Chem. Eng., 2017, 5, 1567–1577 CrossRef CAS.
- Y. H. Xu, W. L. Guo, Y. Ma, X. H. Ping and H. W. Hong, Chin. Rare Earths, 2008, 23, 82–85 Search PubMed.
- Y. H. Xu, Y. Ma, H. W. Hong, X. H. Ping and Q. Jun, J. Chin.
Rare Earth Soc., 2005, 23, 271–274 Search PubMed.
- A. Giwa, N. Akther, V. Dufour and S. W. Hasan, RSC Adv., 2016, 6, 8134–8163 RSC.
- W. Zhao, L. Liu, L. Wang and N. Li, RSC Adv., 2016, 6, 72133–72140 RSC.
- X. Dong, S. Li, Q. Zhang and S. Zhang, RSC Adv., 2014, 4, 22625–22631 RSC.
- Y. Zhou, X. H. Zhu and M. Y. Ban, Chin. Rare Earths, 2006, 27, 81–83 CrossRef CAS.
- X. G. Guo, R. O. Yang, Y. Gong, Z. Jiang, Y. M. Dong and X. Q. Sun, Eur. J. Inorg. Chem., 2017, 17, 2332–2339 CrossRef.
- Y. L. Wang, H. Y. Zhou, Y. B. Wang, F. J. Li and X. Q. Sun, Separ. Purif. Technol., 2017, 184, 280–287 CrossRef CAS.
- Y. Wang, Y. Wang, H. Zhou, F. Li and X. Sun, RSC Adv., 2017, 7, 39556–39563 RSC.
- W. Li, X. L. Wang, S. L. Meng, D. Q. Li and Y. Xiong, Sep. Purif. Technol., 2007, 54, 164–169 CrossRef CAS.
- Y. L. Wang, X. G. Guo, Y. F. Bi, J. Su, W. C. Kong and X. Q. Sun, Green Chem., 2018, 20, 1998–2006 RSC.
- Y. Wang, J. Su, X. Guo, J. Cui, Y. Bai, F. Li and X. Sun, J. Rare Earths, 2019 DOI:10.1016/j.jre.2019.03.019.
- J. L. Wang, M. Y. Xie, H. J. Wang and S. M. Xu, Hydrometallurgy, 2017, 167, 39–47 CrossRef CAS.
- S. T. Hussain, G. A. Khan and M. Shabeer, Asian J. Res. Chem., 2012, 5, 1323–1330 Search PubMed.
- G. Xu and C. Yuan, Solvent Extraction of Rare Earth, Science Press, Beijing, 1987 Search PubMed.
Footnote |
† Electronic supplementary information (ESI) available. See DOI: 10.1039/c9ra05615g |
|
This journal is © The Royal Society of Chemistry 2019 |
Click here to see how this site uses Cookies. View our privacy policy here.