DOI:
10.1039/C9RA05349B
(Paper)
RSC Adv., 2019,
9, 24942-24950
Biological activity evaluation and action mechanism of chalcone derivatives containing thiophene sulfonate†
Received
12th July 2019
, Accepted 6th August 2019
First published on 12th August 2019
Abstract
A series of novel chalcone derivatives containing a thiophene sulfonate group were designed and synthesized. The structures of all title compounds were determined by 1H-NMR, 13C-NMR and HRMS. Antibacterial bioassays indicated that, compound 2l demonstrated excellent antibacterial activities against Xanthomonas axonopodis pv. citri (Xac), with an EC50 value of 11.4 μg mL−1, which is significantly superior to those of bismerthiazol (BT) (51.6 μg mL−1) and thiodiazole-copper (TC) (94.7 μg mL−1). Meanwhile, the mechanism of action of compound 2l was confirmed by using scanning electron microscopy (SEM). In addition, compound 2e showed remarkable inactivation activity against Tobacco mosaic virus (TMV), with an EC50 value of 44.3 μg mL−1, which was superior to that of ningnanmycin (120.6 μg mL−1). Microscale thermophoresis (MST) also showed that the binding of compounds 2e and 2h to Tobacco mosaic virus coat protein (TMV-CP) yielded Kd values of 0.270 and 0.301 μmol L−1, which are better than that of ningnanmycin (0.596 μmol L−1). At the same time, molecular docking studies for 2e and 2h with TMV-CP (PDB code: 1EI7) showed that the compound was embedded well in the pocket between the two subunits of TMV-CP in each case. These results suggested that chalcone derivatives containing a thiophene sulfonate group may be considered as activators in the design of antibacterial and antiviral agents.
1. Introduction
The bacterial and virus infections of crops have become some of the world's most important agricultural problems, and the threat they pose is not only about agricultural production but also about human health.1–3 Pathogens that cause bacterial diseases, such as Xanthomonas oryzae pv. oryzae (Xoo), Ralstonia solanacearum (Rs) and Xanthomonas axonopodis pv. citri (Xac)4–6 are the world's most important and well-known plant bacteria.7–11 These bacteria can actively suppress the crop yields worldwide. Besides, Tobacco mosaic virus (TMV), one of the world's most severe pathogenic viruses can infect a variety of crops, such as tobacco, cucumbers and other economic crops, resulting in a significant loss of crops each year.12–14 To overcome these serious problems, antibacterial drugs such as bismerthiazol (BT), thiodiazole-copper (TC), and ningnanmycin have been widely developed and used to reduce the infection by bacteria and plant viruses.15–17 However, prolonged use and abuse of traditional antibacterial drugs lead to increased resistance of pathogenic genes, which not only enhances the resistance of target pathogens but is also harmful to the environment and health of the plant.18–20 Therefore, the development of new antibacterial and antiviral agents is an important task in pesticide science.
Recent studies have shown that chalcone readily binds to receptors of different structures to form chalcone derivatives due to its flexible structure.21–23 Its derivatives have bactericidal,24,25 insecticidal,26,27 anticancer,28 antiviral,29,30 and anti-inflammatory31 activities, thus demonstrating a wide range of applications in chemical and biological research. Thiophene sulfonate plays a prominent role in the studies and applications of organic chemistry.32,33 As a biologically important active intermediate, it is often used to synthesize thiophene sulfonate compounds with various biological activities.34–36 Therefore, thiophene sulfonate and its derivatives have a wide range of applications in industry, agriculture, pharmaceuticals and have a broad spectrum of biological activities, such as insecticidal,37,38 anti-inflammatory,39,40 antiviral,41,42 and anticancer.43
However, to the best of our knowledge, there have been no reports on chalcone-based thiophene sulfonates so far. To find excellent biological activities and environment-friendly small organic molecules, thiophene sulfonate structure with excellent biological activity is combined with the structure of chalcone by active splicing to design and synthesize a series of novel molecules. Through biological activity screening, it is expected to find chalcone compounds with high anti-plant viral and antibacterial activities, which lays a theoretical foundation for the creation of new pesticides.
2. Experimental
2.1. Instrument, chemicals and reagents
The molecular docking was performed by using DS-CDocker implemented in Discovery Studio (version 4.5). Melting points of the synthesized compounds (2a–2v) were measured using XT-4 Binocular Microscope (Beijing Tech. Instrument, China) without correction. 1H-NMR, 13C-NMR and 19F-NMR spectra were obtained on a Bruker Ascend-400 spectrometer (Bruker Optics, Switzerland) and DMSO-d6 or CDCl3 were used as a solvent and TMS was used as an internal standard. HRMS data were obtained using Thermo Scientific Q Exactive Hybrid Quadrupole Mass Spectrometer (Thermo Scientific Inc., St Louis, MO, USA). The microscale thermophoresis (MST) of the compounds to check the interaction with TMV-CP (Tobacco mosaic virus coat protein) was determined using a micro thermophoresis instrument (NanoTemper Technologies GmbH, Germany). All reagents and solvents were purchased from Chinese Chemical Reagent Company and were chemically pure analytical grade reagents. The synthetic route of chalcone derivatives containing thiophene sulfonate is shown in Scheme 1. The intermediate 1 were prepared according to the methods already reported in the literature.44
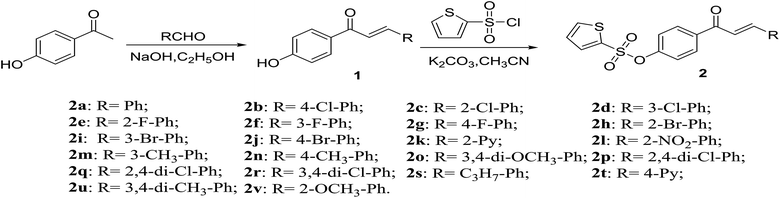 |
| Scheme 1 Synthetic route of title compounds 2a–2v. | |
2.2. General procedure for the synthesis of intermediate 1
At first, aqueous sodium hydroxide solution (5% NaOH, 5 mmol) was added to a round-bottomed flask containing 1-(4-hydroxyphenyl)ethan-1-one (4 mmol) and differently substituted aldehydes (6 mmol). The mixture was stirred at ambient temperature for 12 h. The resulting dark-yellow mixture was acidified by HCl (5% HCl, 5 mmol) after the reaction was completed. Finally, the mixture was filtered under vacuum, and the residue was dried to yield intermediate 1.
2.3. General procedure for the synthesis of title compounds 2a–2v
A solution of intermediate 1 (6.5 mmol) and 2-thiophenesulfonyl chloride (5.5 mmol) in acetonitrile (50 mL) was stirred until dissolved, the reaction mixture was refluxed at 90 °C for 10 h. After completion of the reaction, the whole reaction system was poured into water, extracted with ethyl acetate, the solvent was removed under depressurization, and the residue was purified by column chromatography on silica gel with a mixture (v(petroleum ether)
:
v(ethyl acetate) = 3
:
1) to yield the title compounds 2a–2v.45 The 1H-NMR, 13C-NMR, 19F-NMR and HMRS spectra of the designated title compounds 2a–2v are also provided in the ESI.†
3. Results and discussion
3.1. Spectral properties
The structures of all the compounds were confirmed using 1H-NMR, 13C-NMR and HRMS. In 1H-NMR spectra, multiplet signals at δ 8.27–7.26 ppm indicate the presence of protons in olefinic bonds and aromatic nuclei. The 13C-NMR spectra of the title compounds exhibited a characteristic (C
O) signal at δ 186.79–188.77 ppm. The 13C-NMR spectra of the title compounds exhibited a characteristic (thiophene-2-C) signal at δ 142.22–145.08 ppm. The strong presence of [M + H]+ ions indicates that the title compounds are in the steady state.
3.2. Antibacterial activity of title compounds against Xac, Xoo and Rs in vitro
The in vitro antibacterial activities of the title compounds 2a–2v against three phytopathogenic bacteria (Xac, Xoo and Rs) were tested by using turbidimeter.46–48 Commercial agricultural bactericides (TC and BT) were used as the control, as shown in Table 1, and most of the compounds exhibited significant antibacterial activities against Xac, Xoo and Rs at 100 or 50 μg mL−1. Among these, the antibacterial activities of the compounds 2e, 2l and 2p against Xac at 100 μg mL−1 were 77.2, 95.2 and 79.6%, respectively, which exceeded both TC (57.2%) and BT (65.3%). Compounds 2a, 2f, 2j, 2l, 2m, 2o, 2q and 2u against Xoo at 50 μg mL−1 were 65.6, 60.8, 46.4, 58.0, 58.4, 58.5, 56.9 and 62.2%, respectively, which were better compared to TC (37.2%) and BT (45.2%). In particular, the antibacterial activities of the compounds 2m, 2o and 2p against Rs at 100 and 50 μg mL−1 were 91.4 and 77.5%, 92.4 and 63.3%, 85.2 and 56.6%, respectively, which were significantly superior to TC (45.2 and 20.6%) and BT (53.7 and 32.2%).
Table 1 Antibacterial activities of title compounds (2a–2v) against plant pathogens Xac, Xoo and Rs in vitro
Compounds |
R |
Xac (%) |
Xoo (%) |
Rs (%) |
100 μg mL−1 |
50 μg mL−1 |
100 μg mL−1 |
50 μg mL−1 |
100 μg mL−1 |
50 μg mL−1 |
The commercial agricultural antibacterial agents thiodiazole copper (TC) and bismerthiazol (BT) were used as control agents. |
2a |
Ph |
67.5 ± 1.7 |
53.4 ± 1.6 |
72.4 ± 1.7 |
65.6 ± 1.4 |
76.6 ± 1.4 |
48.8 ± 1.4 |
2b |
4-Cl-Ph |
59.3 ± 2.5 |
22.6 ± 3.4 |
62.6 ± 1.3 |
43.8 ± 2.4 |
47.1 ± 3.7 |
40.3 ± 1.2 |
2c |
2-Cl-Ph |
45.3 ± 3.1 |
23.4 ± 2.9 |
52.9 ± 3.6 |
37.5 ± 2.9 |
53.3 ± 2.9 |
48.9 ± 2.5 |
2d |
3-Cl-Ph |
51.6 ± 0.9 |
36.7 ± 1.8 |
48.6 ± 2.1 |
29.5 ± 4.6 |
47.5 ± 1.0 |
36.8 ± 2.5 |
2e |
2-F-Ph |
77.2 ± 1.1 |
44.3 ± 2.1 |
50.5 ± 1.4 |
37.6 ± 1.6 |
71.1 ± 2.4 |
52.3 ± 2.4 |
2f |
3-F-Ph |
66.5 ± 1.5 |
44.6 ± 2.4 |
88.1 ± 1.7 |
60.8 ± 2.6 |
67.8 ± 0.6 |
55.1 ± 1.6 |
2g |
4-F-Ph |
63.5 ± 1.5 |
55.2 ± 1.9 |
43.2 ± 1.3 |
39.4 ± 1.6 |
58.3 ± 1.5 |
48.8 ± 1.3 |
2h |
2-Br-Ph |
52.9 ± 2.2 |
36.8 ± 0.9 |
53.3 ± 0.9 |
35.7 ± 3.7 |
52.6 ± 3.8 |
33.0 ± 0.8 |
2i |
3-Br-Ph |
46.5 ± 2.9 |
32.4 ± 3.5 |
36.0 ± 2.1 |
24.3 ± 1.4 |
40.8 ± 5.6 |
33.5 ± 1.9 |
2j |
4-Br-Ph |
52.5 ± 2.7 |
25.6 ± 1.7 |
77.3 ± 0.4 |
46.4 ± 2.6 |
56.7 ± 2.4 |
43.4 ± 2.5 |
2k |
2-Py |
59.7 ± 1.2 |
32.6 ± 1.8 |
43.2 ± 0.6 |
32.9 ± 1.2 |
73.7 ± 7.9 |
56.3 ± 2.0 |
2l |
3-NO2-Ph |
95.2 ± 0.7 |
72.3 ± 1.1 |
82.3 ± 1.1 |
58.0 ± 0.6 |
44.3 ± 3.5 |
21.3 ± 1.2 |
2m |
3-CH3-Ph |
44.4 ± 1.5 |
25.8 ± 1.7 |
65.3 ± 2.4 |
58.4 ± 1.5 |
91.4 ± 1.6 |
77.5 ± 1.3 |
2n |
4-CH3-Ph |
40.3 ± 2.8 |
22.4 ± 1.3 |
51.4 ± 2.5 |
22.6 ± 1.6 |
67.1 ± 1.9 |
53.3 ± 2.1 |
2o |
3,4-di-OCH3-Ph |
58.3 ± 0.5 |
38.7 ± 1.1 |
72.3 ± 1.5 |
58.5 ± 1.6 |
92.4 ± 1.2 |
63.3 ± 2.0 |
2p |
2,4-di-Cl-Ph |
79.6 ± 0.5 |
56.4 ± 1.1 |
44.6 ± 1.8 |
34.2 ± 0.9 |
85.2 ± 0.5 |
56.6 ± 2.0 |
2q |
3,4-di-Cl-Ph |
55.6 ± 2.5 |
18.7 ± 1.4 |
68.2 ± 1.1 |
56.9 ± 1.8 |
57.2 ± 1.3 |
47.2 ± 2.6 |
2r |
C3H7-Ph |
59.4 ± 1.4 |
21.4 ± 0.2 |
44.6 ± 1.8 |
34.2 ± 0.9 |
52.8 ± 1.6 |
32.2 ± 1.3 |
2s |
2-Th |
52.3 ± 2.1 |
38.7 ± 1.6 |
55.6 ± 0.4 |
41.8 ± 2.7 |
50.6 ± 1.7 |
42.5 ± 1.8 |
2t |
4-Py |
46.3 ± 0.8 |
26.1 ± 3.8 |
36.7 ± 1.9 |
25.9 ± 4.5 |
45.3 ± 3.2 |
37.6 ± 2.4 |
2u |
3,4-di-CH3-Ph |
52.4 ± 1.2 |
32.5 ± 1.4 |
83.3 ± 0.8 |
62.2 ± 1.0 |
66.3 ± 0.3 |
58.2 ± 0.9 |
2v |
2-OCH3-Ph |
56.2 ± 1.8 |
31.1 ± 1.9 |
56.7 ± 2.4 |
43.4 ± 2.5 |
42.3 ± 1.3 |
28.3 ± 2.5 |
TCa |
— |
57.2 ± 1.3 |
27.8 ± 3.8 |
50.2 ± 0.9 |
37.2 ± 3.2 |
45.2 ± 4.3 |
20.6 ± 2.7 |
BTa |
— |
65.3 ± 2.8 |
54.9 ± 5.5 |
64.9 ± 3.9 |
45.2 ± 2.0 |
53.7 ± 3.6 |
32.2 ± 2.8 |
The antibacterial activities of the compounds were further confirmed by determining their EC50 values, and the obtained results are shown in Table 2. Compounds 2e, 2l and 2p exhibited remarkable antibacterial activities against Xac, with EC50 values of 46.5, 11.4, and 27.1 μg mL−1, which were much better compared to TC (94.7 μg mL−1) and BT (51.6 μg mL−1). Compounds 2a, 2f, 2j, 2l, 2m, 2o, 2q and 2u exhibited excellent antibacterial activities against Xoo, with EC50 values of 19.8, 19.1, 42.6, 28.1, 21.8, 32.0, 39.9 and 29.8 μg mL−1, respectively which were significantly superior to TC (97.8 μg mL−1) and BT (71.7 μg mL−1). Compounds 2a, 2e, 2f, 2k, 2m, 2n, 2o, 2p and 2u exhibited notable antibacterial activities against Rs, with EC50 values of 38.4, 38.9, 43.6, 38.7, 11.6, 38.4, 25.0, 31.9 and 36.3 μg mL−1, respectively, which were much better than TC (98.6 μg mL−1) and BT (78.8 μg mL−1). These results indicate that such compounds should be further studied as potential alternative templates in the search for novel antibacterial agents.
Table 2 EC50 values of the title compounds against plant pathogenic bacteria in vitro
Bacterial |
Compounds |
R |
Toxic regression equation |
r |
EC50/(μg mL−1) |
The commercial agricultural antibacterial agents thiodiazole copper (TC) and bismerthiazol (BT) were used as control agents. |
Xac |
2e |
2-F-Ph |
y = 1.2997x + 2.9498 |
0.9862 |
46.5 |
2l |
3-NO2-Ph |
y = 1.5536x + 2.7744 |
0.9872 |
11.4 |
2p |
2,4-di-Cl-Ph |
y = 1.1393x + 3.3968 |
0.9801 |
27.1 |
TCa |
— |
y = 1.1576x + 2.7122 |
0.9859 |
94.7 |
BTa |
— |
y = 1.0698x + 3.1679 |
0.9831 |
51.6 |
Xoo |
2a |
Ph |
y = 0.8781x + 3.8623 |
0.9842 |
19.8 |
2f |
3-F-Ph |
y = 1.4287x + 3.1693 |
0.9869 |
19.1 |
2j |
4-Br-Ph |
y = 1.4836x + 2.5829 |
0.9756 |
42.6 |
2l |
3-NO2-Ph |
y = 1.4387x + 2.9155 |
0.9778 |
28.1 |
2m |
3-CH3-Ph |
y = 1.0376x + 3.3799 |
0.9891 |
21.8 |
2o |
3,4-di-OCH3-Ph |
y = 1.1362x + 3.2891 |
0.9981 |
32.0 |
2q |
3,4-di-Cl-Ph |
y = 1.3694x + 2.8072 |
0.9876 |
39.9 |
2u |
3,4-di-CH3-Ph |
y = 1.6774x + 2.5248 |
0.9928 |
29.8 |
TCa |
— |
y = 1.2058x + 2.2869 |
0.9971 |
97.8 |
BTa |
— |
y = 0.9913x + 3.1606 |
0.9802 |
71.7 |
Rs |
2a |
Ph |
y = 1.3445x + 2.8696 |
0.9812 |
38.4 |
2e |
2-F-Ph |
y = 1.2021x + 3.0881 |
0.9907 |
38.9 |
2f |
3-F-Ph |
y = 1.4532x + 2.6183 |
0.9729 |
43.6 |
2k |
2-Py |
y = 1.4622x + 2.6783 |
0.9975 |
38.7 |
2m |
3-CH3-Ph |
y = 1.3326x + 3.5835 |
0.9814 |
11.6 |
2n |
4-CH3-Ph |
y = 0.9189x + 3.5445 |
0.9775 |
38.4 |
2o |
3,4-di-OCH3-Ph |
y = 1.9357x + 2.2953 |
0.9886 |
25.0 |
2p |
2,4-di-Cl-Ph |
y = 1.7032x + 2.4391 |
0.9758 |
31.9 |
2u |
3,4-di-CH3-Ph |
y = 1.0373x + 3.3816 |
0.9864 |
36.3 |
TCa |
— |
y = 1.4682x + 1.8274 |
0.9758 |
78.8 |
BTa |
— |
y = 1.2581x + 2.4918 |
0.9746 |
98.6 |
3.3. Structure–activity relationships of antibacterial activities
Tables 1 and 2 show that the changes in the substituted groups could significantly impact the inhibitory effects against plant bacteria, and analysis on the structure–activity relationships is discussed below. For instance, the designated compounds 2e (R = 2-F-Ph), 2l (R = 3-NO2-Ph) and 2p (R = 2,4-di-Cl-Ph) exhibited significant anti Xac at 100 μg mL−1, with the inhibition rates of 77.2, 95.2 and 79.6%, respectively. These compounds were found to be more active compared to other tested compounds. However, when R was substituted with Ph, 3-F-Ph, 4-Br-Ph, 3-NO2-Ph, 3,4-di-OCH3-Ph, and 3,4-di-CH3-Ph groups, the activities of the corresponding compounds 2a, 2f, 2j, 2l, 2o and 2u, against Xoo at 100 μg mL−1 were 72.4, 88.1, 77.3, 82.3, 72.3 and 83.3%, respectively, which were higher than that of bismerthiazol (64.9%) and thiadiazole-copper (50.2%). Notably, when R groups was Ph (2a), 3-F-Ph (2e), 2-Py (2k), 3-CH3-Ph (2m), 3,4-di-OCH3-Ph (2o) and 2,4-di-Cl-Ph (2p) excellent biological activity was found anti Rs at 100 μg mL−1, inhibition rates of 76.6, 71.1, 73.7, 91.4, 92.4 and 85.2%, respectively.
3.4. Scanning electron microscopy (SEM) study
Following the antibacterial activities of the compounds, as shown in Tables 1 and 2, the antibacterial mechanism of Xac was further studied through SEM analysis.49 It can be seen that the compound destroys the cell membrane, and more importantly, as the concentration of the compound increases, the damage of the cell membrane becomes more serious. For example, when the concentration was 50 μg mL−1, a part of the cell membrane begins to destroy (Fig. 1B). However, when the concentration was further increased to 100 μg mL−1, most of the cell membrane was damaged, and only a small number of cells was present (Fig. 1C). In contrast, in the absence of treatment with compound 2l, the cells were smooth and well stacked with an intact membrane (Fig. 1A). These SEM images further confirm that compound 2l destroys bacterial cells and may eventually kill the cells.
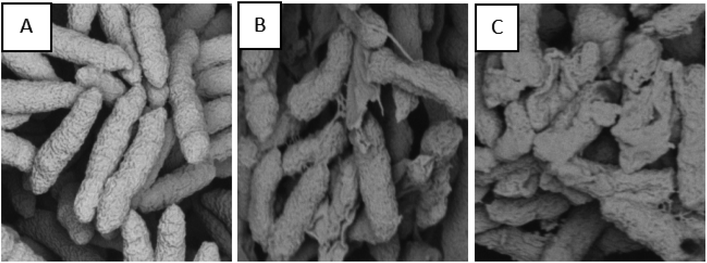 |
| Fig. 1 SEM images for Xac after incubated using different concentrations of compound 2l, (A) 0 μg mL−1, (B) 50 μg mL−1 and (C) 100 μg mL−1. Scale bar for (A), (B) and (C) are 2 μm. | |
3.5. Antiviral activity of title compounds against TMV in vivo
Using the same age tobacco leaves as the test subjects, the in vivo healing and protective activities of TMV at 500 μg mL−1 were assessed by the half-blight spot method.50–52 For this, a commercial agricultural antiviral agent ningnanmycin was used as a control, and the obtained preliminary bioassay results are shown in Table 3. The compounds exhibited significant antiviral activity against TMV. Among them, compounds 2a, 2e, 2h, 2l, 2m and 2o showed excellent curative activities against TMV, with the inhibition of 72.5, 84.2, 73.5, 81.8, 72.6 and 75.3%, which were better than ningnanmycin (53.3%). The protective activities of 2a, 2e, 2h and 2l (70.0, 75.2, 71.8 and 72.8%, respectively) against TMV were more effective than ningnanmycin (62.6%). In particularly, compounds 2a, 2e, 2h and 2o showed excellent inactivation activities against TMV, with the inhibition of 80.1, 87.3, 81.1 and 79.8%, which were better than ningnanmycin (78.3%).
Table 3 Antiviral activities of the test compounds against TMV in vivo at 500 μg mL−1
Compounds |
R |
Curative activitya (%) |
Protective activitya (%) |
Inactivation activitya (%) |
Average of three replicates. The commercial antiviral agent ningnanmycin. |
2a |
Ph |
72.5 ± 2.5 |
67.0 ± 2.1 |
80.1 ± 1.7 |
2b |
4-Cl-Ph |
46.4 ± 2.6 |
58.3 ± 0.9 |
53.8 ± 2.2 |
2c |
2-Cl-Ph |
52.4 ± 1.2 |
29.9 ± 1.0 |
62.3 ± 1.8 |
2d |
3-Cl-Ph |
45.3 ± 3.8 |
51.9 ± 2.2 |
59.7 ± 2.3 |
2e |
2-F-Ph |
76.2 ± 1.2 |
70.2 ± 1.9 |
87.3 ± 0.7 |
2f |
3-F-Ph |
48.5 ± 0.9 |
35.3 ± 2.2 |
60.2 ± 1.5 |
2g |
4-F-Ph |
52.3 ± 1.8 |
58.1 ± 0.4 |
58.7 ± 0.6 |
2h |
2-Br-Ph |
73.5 ± 2.1 |
68.8 ± 4.9 |
81.1 ± 1.9 |
2i |
3-Br-Ph |
26.9 ± 1.8 |
54.6 ± 4.4 |
65.3 ± 3.1 |
2j |
4-Br-Ph |
48.4 ± 0.8 |
61.9 ± 2.5 |
58.9 ± 1.7 |
2k |
2-Py |
45.6 ± 1.6 |
42.9 ± 1.9 |
66.1 ± 1.8 |
2l |
3-NO2-Ph |
74.8 ± 1.7 |
69.8 ± 1.6 |
75.3 ± 2.4 |
2m |
3-CH3-Ph |
72.6 ± 1.9 |
52.8 ± 0.7 |
69.2 ± 0.7 |
2n |
4-CH3-Ph |
30.3 ± 1.6 |
35.4 ± 2.9 |
45.3 ± 2.7 |
2o |
3,4-di-OCH3-Ph |
65.3 ± 0.8 |
60.2 ± 1.8 |
79.8 ± 2.1 |
2p |
2,4-di-Cl-Ph |
52.8 ± 2.7 |
40.9 ± 1.6 |
35.2 ± 1.9 |
2q |
3,4-di-Cl-Ph |
52.1 ± 2.0 |
46.2 ± 2.8 |
55.1 ± 3.3 |
2r |
C3H7-Ph |
27.2 ± 1.6 |
41.6 ± 1.9 |
58.1 ± 0.9 |
2s |
2-Th |
48.7 ± 4.3 |
32.1 ± 1.9 |
54.2 ± 1.7 |
2t |
4-Py |
43.9 ± 2.8 |
57.6 ± 1.7 |
56.2 ± 3.8 |
2u |
3,4-di-CH3-Ph |
49.3 ± 1.9 |
36.3 ± 1.8 |
61.2 ± 0.9 |
2v |
2-OCH3-Ph |
53.0 ± 1.8 |
51.1 ± 2.6 |
45.2 ± 1.4 |
Ningnanmycinb |
— |
53.3 ± 1.2 |
62.6 ± 1.3 |
78.3 ± 1.5 |
To compare the antiviral activity of the synthesized compounds (2a–2v), EC50 values of 2a, 2e, 2h, 2l, 2m and 2o were calculated and have been summarized in Table 4. It could be seen that compounds 2a, 2e, 2h and 2l exhibited higher curative activity than ningnanmycin (403.7 μg mL−1), with EC50 values of 256.1, 178.0, 279.2 and 258.5 μg mL−1, respectively. Compounds 2e and 2l exhibited the best protective activity against TMV, with EC50 values of 269.0 and 282.6 μg mL−1, respectively, which were better than that of ningnanmycin (317.0 μg mL−1). Among them, compounds 2e and 2h exhibited the best inactivation activity against TMV, with EC50 values of 44.3 and 62.2 μg mL−1, respectively, which were better than that of ningnanmycin (120.6 μg mL−1). It can be indicated from these results that most of these novel chalcone derivatives containing thiophene sulfonate could be further studied as a potential alternative template in the search for novel antiviral agents (Fig. 2).
Table 4 EC50 values of some compounds against TMV
|
Compounds |
R |
Toxic regression equation |
r |
EC50a/(μg mL−1) |
Average of three replicates. The commercial antiviral agent ningnanmycin. |
Curative |
2a |
Ph |
y = 1.5764x + 1.2033 |
0.9948 |
256.1 |
2e |
2-F-Ph |
y = 1.2516x + 2.1833 |
0.9797 |
178.0 |
2h |
2-Br-Ph |
y = 1.6378x + 0.9941 |
0.9981 |
279.2 |
2l |
3-NO2-Ph |
y = 1.5526x + 1.2543 |
0.9958 |
258.5 |
2m |
3-CH3-Ph |
y = 1.3411x + 1.6219 |
0.9740 |
330.3 |
2o |
3,4-di-OCH3-Ph |
y = 1.2168x + 1.9170 |
0.9829 |
341.7 |
Ningnanmycinb |
y = 1.1975x + 1.8793 |
0.9956 |
403.7 |
Protection |
2a |
Ph |
y = 1.3994x + 1.4998 |
0.9745 |
317.1 |
2e |
2-F-Ph |
y = 1.3560x + 1.7053 |
0.9767 |
269.0 |
2h |
2-Br-Ph |
y = 1.2375x + 1.9576 |
0.9913 |
287.4 |
2l |
3-NO2-Ph |
y = 1.5374x + 1.2315 |
0.9901 |
282.6 |
Ningnanmycinb |
y = 1.2081x + 1.9784 |
0.9804 |
317.0 |
Inactivation |
2a |
Ph |
y = 1.0219x + 3.0517 |
0.9985 |
80.6 |
2e |
2-F-Ph |
y = 1.0379x + 3.2916 |
0.9894 |
44.3 |
2h |
2-Br-Ph |
y = 0.9258x + 3.3392 |
0.9973 |
62.2 |
2o |
3,4-di-OCH3-Ph |
y = 0.9448x + 3.2055 |
0.9935 |
79.3 |
Ningnanmycinb |
y = 1.0209x + 2.8752 |
0.9818 |
120.6 |
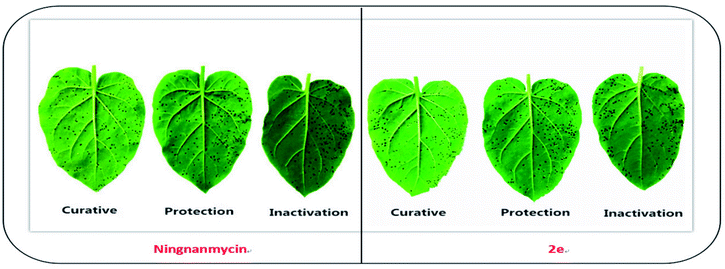 |
| Fig. 2 Tobacco leaf morphology effects of the ningnanmycin and 2e against TMV in vivo. (Right leaf: not treated with compound; left leaf: smeared with compound). | |
3.6. Structure–activity relationships of antiviral activities
As indicated in Tables 3 and 4, most of the thiophene sulfonate chalcone derivatives showed significant antiviral activities against TMV, and some of the structure–activity relationships can be analyzed and summarized. First, the Ph, 2-F-Ph, 2-Br-Ph, 3-NO2-Ph and 3-CH3-Ph groups at –R position greatly improved the curative activities of the title compounds against TMV. For instance, the curative activities of the target compounds 2a, 2e, 2h, 2l and 2m, which were better than that of other substituent groups. In addition, when –R was Ph, 2-F-Ph, 2-Br-Ph and 3-NO2-Ph groups, the protective activities of the corresponding compounds 2a, 2e, 2h and 2l at 500 μg mL−1 were better than that of ningnanmycin. When R was with Ph (2a), 2-F-Ph (2e), 2-Br-Ph (2h) and 3,4-di-OCH3-Ph (2o) groups, the corresponding compounds presented excellent inactivation activity against TMV. For instance, some target compounds the curative, protection and inactivation effects which were better than that of ningnanmycin following the order of 2e (R = 2-F-Ph) > 2h (R = 2-Br-Ph) > 2a (R = Ph). The detailed values corresponding to these results can be seen in Table 3.
3.7. Binding sites of 2e, 2h, 2n and ningnanmycin to TMV-CP
To further analyze the interactions between the title compounds and TMV coat protein (TMV-CP), the microscale thermophoresis (MST) analysis was used.53,54 The MST results as summarized in Fig. 3 show that the binding of compounds 2e, 2h, 2n and ningnanmycin to TMV-CP protein yielded Kd values of 0.270 ± 0.190 μmol L−1, 0.301 ± 0.121 μmol L−1, 56.401 ± 11.298 μmol L−1 and 0.596 ± 0.338 μmol L−1, respectively. As indicated in MST, 2e or 2h with ningnanmycin share strong affinity, which is contrary to 2n as it shares weak affinity. These results exhibited that the combining capacity in the following order of 2e > 2h > ningnanmycin > 2n is consistent with the trends of the screening of antiviral activities. Based on the experimental results it can be predicted that compounds 2e and 2h may interact with TMV-CP. As depicted in Fig. 3 the bioactivity was mainly determined by the electrostatic interactions, and the activity could have been enhanced by the presence of aromatic ring which is rich in electrons for absorption. For example, when R was substituted with 2-F-Ph and 2-Br-Ph groups, the corresponding compounds 2e and 2h exhibited a stronger combining capacity, with Kd values of 0.270 ± 0.190 μmol L−1 and 0.301 ± 0.121 μmol L−1, as compared to that of compound 2h (56.401 ± 11.298 μmol L−1), which was substituted with a 4-CH3-Ph.
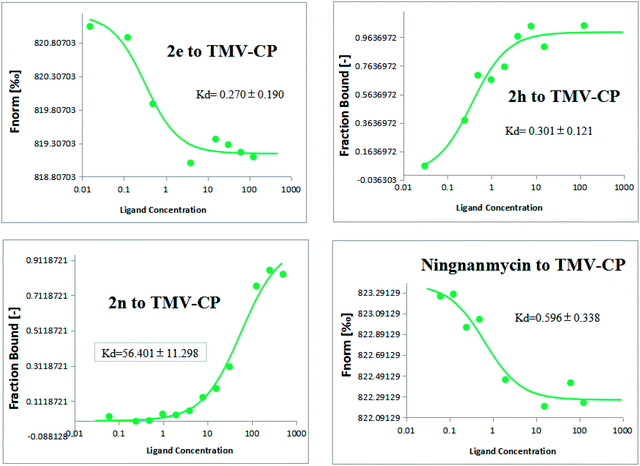 |
| Fig. 3 Microscale thermophoresis (MST) results of compounds 2e, 2h, 2n and ningnanmycin. | |
3.8. Molecular docking of 2e or 2h and TMV-CP
To identify the 2e and 2h recognition sites in TMV-CP (PDB code: 1EI7), we performed molecular docking using the Gold method with 200 cycles. As depicted in Fig. 4, the three compounds were well-embedded in the activity pocket (ARG-46, THR-42, ARG-90, GLN-34, THR-37, etc.) between the two subunits of TMV-CP. Among them, ARG-46 had strong hydrogen bond with 2e (1.620 Å and 1.653 Å), C
O (2e) demonstrates three hydrogens with the THR-42 (O–H = 2.332 Å), ARG-90 (O–H = 1.915 Å and 1.935 Å). Moreover, thiophene sulfonate demonstrates strong hydrogen bond with GLN-34 (O–H = 1.819 Å), THR-37 showed one hydrogen bond 2h (1.940 Å), there was also one hydrogen bond between the C
O and the residue ARG-90 (O–H = 2.206 Å). In addition, GLN-34 had strong hydrogen bond with ningnanmycin (1.798 Å), C
O (ningnanmycin) demonstrates one hydrogens with the ARG-90 (2.231 Å). It can be seen that the combination of 2e and 2h with TMV-CP has several more stable hydrogen bonds than ningnanmycin and TMV-CP, thus indicating that 2e and 2h have better antiviral activity than ningnanmycin. These interactions between molecules and TMV-CP are likely to weaken the interaction of two subunits of TMV-CP, thereby preventing the self-assembly of TMV particle, as well as the binding capability with TMV-CP. The results of molecular docking studies were consistent with the experimental results (protection and curative activities) and support that the chalcone derivatives containing thiophene sulfonate may be potential lead structures for developing novel anti-TMV agents.
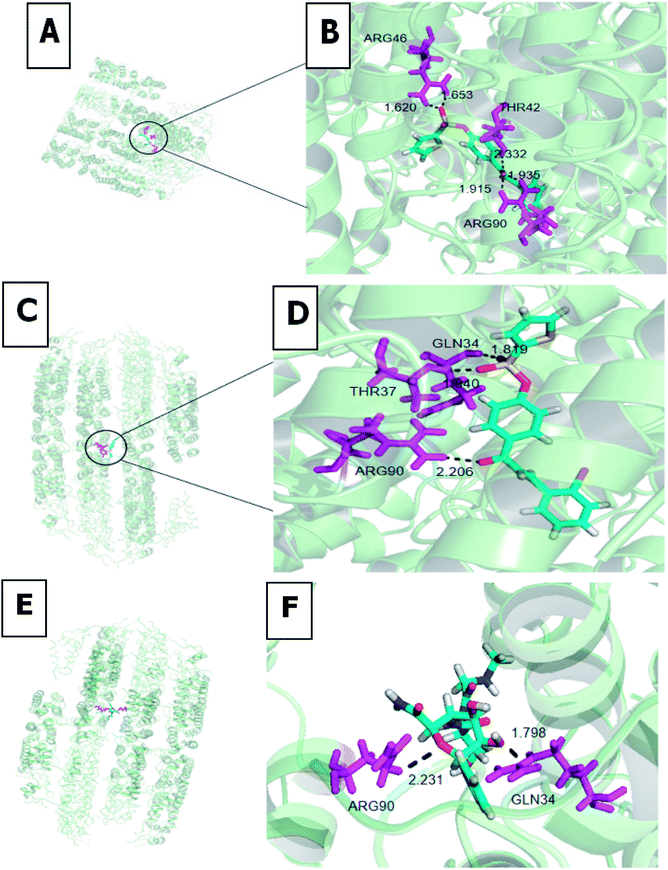 |
| Fig. 4 Molecular docking studies of compounds 2e (A and B), 2h (C and D) and ningnanmycin (E and F). | |
4. Conclusions
In summary, with the aim of developing a novel, highly efficient, and environmentally benign virucide, in this study a thiophene sulfonate group was introduced into chalcone derivatives to synthesize 22 derivatives. Also, their in vitro antibacterial activities against Xac, Xoo, and Rs and their in vivo antiviral activity against TMV were evaluated. Bioassay results showed that several of the title compounds exhibited good antibacterial and antiviral activities. In particular, compound 2l showed remarkable activity against Xac. Among them, compounds 2a, 2e, 2h, 2l, 2m and 2o showed excellent curative activities against TMV. Based on the above results, these novel thiophene sulfonate chalcone derivatives should be further studied as potential alternative templates in the search for novel antibacterial and antiviral agents.
Conflicts of interest
The authors declare that they have no competing interests.
Acknowledgements
This research was funded by the National Key Research and Development Program of China (No. 2017YFD0200506) and the Science and Technology Project of Guizhou Province (No. 20185781).
References
- Z. F. Li, S. L. Wu, X. F. Bai, Y. Liu, J. F. Lu, Y. Liu, B. G. Xiao, X. P. Lu and L. Fan, J. Bacteriol., 2011, 193, 6088–6089 CrossRef CAS PubMed.
- F. D. Lorenzo, A. Palmigiano, A. Silipo, Y. Desaki, D. Garozzo, R. Lanzetta, N. Shibuya and A. Molinaro, Carbohydr. Res., 2016, 427, 38–43 CrossRef PubMed.
- B. Li, B. P. Liu, C. L. Shan, M. Ibrahim, Y. S. Lou, Y. L. Wang, G. L. Xie, H. Y. Li and G. H. Sun, Pest Manage. Sci., 2013, 69, 312–320 CrossRef CAS PubMed.
- S. Perumalsamy, M. Bharani, M. Sudha, P. Nagarajan, L. Arul, R. Saraswathi, P. Balasubramanian and J. Ramalingam, Plant Breed., 2010, 129, 400–406 CAS.
- P. Y. Wang, L. Zhou, J. Zhou, Z. B. Wu, W. Xue, B. A. Song and S. Yang, Bioorg. Med. Chem. Lett., 2016, 26, 1214–1217 CrossRef CAS PubMed.
- A. G. De Oliveira, L. S. Murate, F. R. Spago, L. D. Lopes, J. P. D. Beranger, J. A. B. S. Martin, M. A. Nogueira, J. C. P. Mello, C. G. T. D. Andrade and G. Andrate, Biol. Control, 2011, 56, 125–131 CrossRef.
- T. R. Gottwald, X. Sun, T. Riley, J. H. Graham, F. Ferrandino and E. L. Taylor, Phytopathology, 2002, 92, 361–377 CrossRef PubMed.
- T. B. Adhikari, R. C. Basnyat and T. W. Mew, Plant Dis., 1999, 83, 46–50 CrossRef PubMed.
- Z. Ji, C. Ji, B. Liu, L. Zou, G. Chen and B. Yang, Nat. Commun., 2016, 7, 13435–13444 CrossRef CAS PubMed.
- L. F. Zou, Y. R. Li and G. Y. Chen, Agric. Sci. China, 2011, 10, 1139–1150 CrossRef CAS.
- L. F. Zou, X. P. Wang, Y. Xiang, B. Zhang, Y. R. Li and J. S. Wang, Appl. Environ. Microbiol., 2006, 72, 6212–6224 CrossRef CAS PubMed.
- M. H. Chen, Z. Chen, B. A. Song, P. S. Bhadury, S. Yang, X. J. Cai, D. Y. Hu, W. Xue and S. Zeng, J. Agric. Food Chem., 2009, 57, 1383–1388 CrossRef CAS PubMed.
- X. H. Gan, D. Y. Hu, P. Li, J. Wu, X. W. Chen, W. Xue and B. A. Song, Pest Manage. Sci., 2016, 72, 534–543 CrossRef CAS PubMed.
- X. H. Gan, D. Y. Hu, Y. J. Wang, L. Yu and B. A. Song, J. Agric. Food Chem., 2017, 35, 665–672 CAS.
- P. Y. Wang, L. Zhou, J. Zhou, Z. B. Wu, W. Xue, B. A. Song and S. Yang, Bioorg. Med. Chem. Lett., 2016, 26, 1214–1217 CrossRef CAS PubMed.
- X. Wang, P. Li, Z. Li, J. Yin, M. He, W. Xue, Z. Chen and B. A. Song, J. Agric. Food Chem., 2013, 61, 9575–9582 CrossRef CAS PubMed.
- X. M. Zhong, X. B. Wang, L. J. Chen and W. Xue, Chem. Cent. J., 2017, 11, 106 CrossRef PubMed.
- S. Perumalsamy, M. Bharani, M. Sudha, P. Nagarajan, L. Arul, R. Saraswathi, P. Balasubramanian and J. Ramalingam, Plant Breed., 2010, 129, 400–406 CAS.
- Y. Q. Hu, S. Zhang, Z. Xu, Z. S. Lv, M. L. Liu and L. S. Feng, Eur. J. Med. Chem., 2017, 141, 335–345 CrossRef CAS PubMed.
- Y. J. Lin, Z. L. He, E. N. Rosskopf, K. L. Conn, C. A. Powell and G. Lazarovits, Plant Dis., 2010, 94, 201–206 CrossRef CAS PubMed.
- R. Schobert, B. Biersack and A. Dietrich, J. Med. Chem., 2009, 52, 241–246 CrossRef CAS PubMed.
- S. Vogel, M. Barbic and J. Guido, Eur. J. Med. Chem., 2010, 45, 2206–2213 CrossRef CAS PubMed.
- V. Tomar and G. Bhattacharjee, Eur. J. Med. Chem., 2010, 45, 745–751 CrossRef CAS PubMed.
- P. L. Zhao, C. L. Liu, W. Huang, Y. Z. Wang and G. F. Yang, J. Agric. Food Chem., 2007, 55, 5697–5700 CrossRef CAS PubMed.
- R. Sribalan, G. Banuppriya, M. Kirubavathi, A. Jayachitra and G. Padmini, Bioorg. Med. Chem. Lett., 2016, 26, 5624–5630 CrossRef CAS PubMed.
- G. Pasquale, G. P. Romanelli, J. C. Autino, J. García, V. O. Erlinda and P. R. Duchowicz, J. Agric. Food Chem., 2012, 60, 692–697 CrossRef CAS PubMed.
- S. Attar, Z. O'Brien, H. Alhaddad, M. L. Golden and A. G. Urrea, Bioorg. Med. Chem., 2011, 19, 2055–2073 CrossRef CAS PubMed.
- D. Coskun, M. Erkisa, E. Ulukaya, M. F. Coskun and F. Ari, Eur. J. Med. Chem., 2017, 136, 212–222 CrossRef CAS PubMed.
- X. H. Gan, D. Y. Hu, Y. J. Wang, L. Yu and B. A. Song, J. Agric. Food Chem., 2017, 65, 4367–4377 CrossRef CAS PubMed.
- A. L. Cole, S. Hossain and A. M. Cole, Bioorg. Med. Chem., 2016, 24, 2768–2776 CrossRef CAS PubMed.
- J. F. Li, D. Li, Y. M. Xu, Z. B. Guo, X. Liu, H. Yang, L. C. Wu and L. S. Wang, Bioorg. Med. Chem. Lett., 2017, 27, 602–606 CrossRef CAS PubMed.
- S. J. William, J. H. Melanie, A. S. Stacey and E. M. Rockafellow, J. Org. Chem., 2009, 74, 2765–2770 CrossRef PubMed.
- M. K. Parai, G. Panda, V. Chaturvedi, Y. K. Manju and S. Sinha, Bioorg. Med. Chem. Lett., 2007, 18, 289–292 CrossRef PubMed.
- A. M. Isloor, B. Kalluraya and K. Sridhar, Eur. J. Med. Chem., 2010, 45, 825–830 CrossRef CAS PubMed.
- M. B. Félix, E. R. Souza, M. de Lima, D. K. G. Frade, V. d. L. Serafim and M. R. de Oliveira, Bioorg. Med. Chem. Lett., 2016, 24, 3972–3977 CrossRef PubMed.
- D. H. Pandya, J. A. Sharma, H. B. Jalani, A. N. Pandya, V. Sudarsanam, S. Kachler and K. K. Vasu, Bioorg. Med. Chem. Lett., 2015, 25, 1306–1309 CrossRef CAS PubMed.
- R. Wang, X. Y. Zhi, J. Li and H. Xu, J. Agric. Food Chem., 2015, 63, 6668–6674 CrossRef CAS PubMed.
- R. F. Sun, Z. W. Wang, Y. Q. Li, L. X. Xiong, Y. X. Liu and Q. M. Wang, J. Agric. Food Chem., 2013, 61, 517–522 CrossRef CAS PubMed.
- K. A. K. Musa and L. A. Eriksson, J. Phys. Chem. B, 2009, 113, 11306–11313 CrossRef CAS PubMed.
- R. M. Mohareb, M. Y. Zaki and N. S. Abbas, Steroids, 2015, 98, 80–91 CrossRef CAS PubMed.
- S. G. Gallego, M. J. Serramía, E. Arnaiz, L. Díaz, M. A. Muñoz-Fernández, P. Gómez-Sal, M. F. Ottaviani and R. Gómez, Eur. J. Med. Chem., 2011, 10, 1657–1665 Search PubMed.
- Z. Wang, D. W. Kang, M. Chen, G. C. Wu, D. Feng, T. Zhao, Z. X. Zhou, Z. P. Huo and X. Y. Liu, Chem. Biol. Drug Des., 2018, 92, 2009–2021 CrossRef CAS PubMed.
- N. K. Duddukuri, S. Thatikonda, C. Godugu, R. A. Kumar and N. Doijad, ChemistrySelect, 2018, 3, 6859–6864 CrossRef CAS.
- J. Zhang, F. Yang, Z. T. Qiao, M. Y. Zhu and H. C. Zhou, Bioorg. Med. Chem. Lett., 2016, 26, 5797–5801 CrossRef CAS PubMed.
- B. Prasad, R. Adepu, S. Sandra, D. Rambabu, G. Rama Krishna, C. M. Reddy, G. S. Deora, P. Misra and M. Pal, Chem. Commun., 2012, 48, 10434–10436 RSC.
- X. Wang, P. Li, Z. N. Li, J. Yin, M. He, W. Xue, Z. W. Chen and B. A. Song, J. Agric. Food Chem., 2013, 61, 9575–9582 CrossRef CAS PubMed.
- P. Li, D. Y. Hu, D. D. Xie, J. X. Chen, L. H. Jin and B. A. Song, J. Agric. Food Chem., 2018, 66, 3093–3100 CrossRef CAS PubMed.
- X. H. Ruan, C. Zhang, S. C. Jiang, T. Guo, R. J. Xia, Y. Chen, X. Tang and W. Xue, Molecules, 2018, 23, 3132–3142 CrossRef PubMed.
- J. Chen, J. Shi, L. Yu, D. Liu, X. H. Gan, B. A. Song and D. Y. Hu, J. Agric. Food Chem., 2018, 66, 5335–5345 CrossRef CAS PubMed.
- Z. X. Wu, J. Zhang, J. X. Chen, J. K. Pan, L. Zhao, D. Y. Liu, A. W. Zhang, J. Chen, D. Y. Hu and B. A. Song, Pest Manage. Sci., 2017, 73, 2079–2089 CrossRef CAS PubMed.
- L. J. Chen, T. Guo, R. J. Xia, X. Tang, Y. Chen, C. Zhang and W. Xue, Molecules, 2019, 24, 925–937 CrossRef PubMed.
- J. Zhou, Q. Q. Tao, P. Y. Wang, W. B. Shao, Z. B. Wu, Z. Li and S. Yang, Bioorg. Med. Chem. Lett., 2018, 28, 1742–1746 CrossRef CAS PubMed.
- X. Y. Li, J. Liu, X. Yang, Y. Ding, J. Wu, D. Y. Hu and B. A. Song, Bioorg. Med. Chem., 2015, 23, 3629–3637 CrossRef CAS PubMed.
- C. J. Wienken, P. Baaske, U. Rothbauer, D. Braun and S. Duhr, Nat. Commun., 2010, 1, 100–107 CrossRef PubMed.
Footnote |
† Electronic supplementary information (ESI) available. See DOI: 10.1039/c9ra05349b |
|
This journal is © The Royal Society of Chemistry 2019 |
Click here to see how this site uses Cookies. View our privacy policy here.