DOI:
10.1039/C9RA05256A
(Paper)
RSC Adv., 2019,
9, 27439-27448
Remarkably selective biocompatible turn-on fluorescent probe for detection of Fe3+ in human blood samples and cells†
Received
10th July 2019
, Accepted 18th August 2019
First published on 2nd September 2019
Abstract
The robust nature of a biocompatible fluorescent probe is demonstrated, by its detection of Fe3+ even after repeated rounds of quenching (reversibility) by acetate in real human blood samples and cells in vitro. Significantly trace levels of Fe3+ ions up to 8.2 nM could be detected, remaining unaffected by the existence of various other metal ions. The obtained results are validated by AAS and ICP-OES methods. A portable test strip is also fabricated for quick on field detection of Fe3+. As iron is a ubiquitous metal in cells and plays a prominent role in biological processes, the use of this probe to image Fe3+ in cells is a substantial development towards biosensing. Cytotoxicity studies also proved the nontoxic nature of this probe.
1. Introduction
Over the past few decades, traditional techniques like atomic absorption spectroscopy (AAS), inductively coupled plasma atomic emission spectroscopy (ICP-OES) voltammetry and colorimetric techniques1 have been used for the detection of Fe3+. Nonetheless, these techniques require advanced equipment, tiresome sample preparation procedures and trained professionals. Fluorescence techniques have been extensively applied by research groups to avoid these disadvantages. A number of fluorescent probes for selective sensing of Fe3+ ion have been developed.2 As Fe3+ possesses paramagnetic nature in its 3d orbital it leads to fluorescence quenching.3 Most of the early reported probes exhibited turn-off (fluorescence quenching) response because of the paramagnetic nature of Fe3+ ion that restricts its application in biological systems.4 Turn on signals are dominant in biosensing compared to turn-off ones because of their good processing of signals in biological media.5
Highly optically active metal chalcogenide/quantum dots (QDs) have been reported as fluorescence based sensors/receptors with excellent stability and photophysical properties.6 Due to their tunable optical properties6a,7 they have gained importance in numerous fields such as photovoltaics,8 bioimaging9 and as sensors10,11. In the early years Jiang et al. reported a gallic acid modified nanometer sized alumina micro-column separations and were able to detect up to 52.1 μM Fe3+ in real water samples using ICP-MS.12 With time, in 2019 Mohammadi et al. developed a MBTBA-Fe3O4@SiO2 nanocomposite fluorescent ligand capable of sensing Fe3+ with a detection limit of 43.09 nM.13 Further Nibu et al. reported a dual responsive colorimetric/fluorescent turn-on sensor with detection limit up to 7.49 μM concentration.14 All these probes were for iron detection in water samples. Reports on detection of metal ions other than Fe3+ in blood samples can also be found. In 2009, Jung et al. reported a BODIPY-functionalized magnetic silica nanoparticle fluorescent receptor for probing Pb2+ in children's blood15 and the same group in 2010 reported a nitrobenzene-functionalized Ni@SiO2 core/shell magnetic nanoparticles as a fluorogenic chemosensor that removed about 96% of Cu2+ in human blood.16 Subsequently in 2011 a macrocyclic dioxotetraamine probe of two-photon excited chemosensor was designed by Liu et al. for determination of copper ions with a detection limit of 0.007 μM in human blood serum samples17 based on the principle of ICT. Bandyopadhyay et al. reported a fluorescent ligand to determine the concentration of inorganic phosphate and detected about 1.82 mM of phosphate in chicken serum.18 Recently, Vishaka et al. designed a colorimetric chemosensor for the sensing of Cu2+ with a detection limit of 1.31 μM in human blood serum samples.19 For the first time in 2016 Wei et al. reported a Fe3O4@ZnO based fluorescent chemosensor for the detection of Fe3+ in human blood serum samples with 92.6–108.4% recovery20 and no reports since then have been reported for such chemosensing of Fe3+ in blood. Interference of other transition metal ions, like Al3+, Pb2+, Hg2+, Cu2+ with Fe3+ results in poor selectivity and sensitivity of the chemosensor.21 However many colorimetric and fluorometric chemosensors are described in the literature for intracellular imaging of cells.22 Most of the reported probes cannot be used in live cells due to their cellular toxicity and many of them are susceptible to be affected by the background fluorescence since they possess a shorter emission wavelength below 550 nm.
Xanthene and its derivatives23 with their spirocyclic structure being responsible for off and on fluorescence when the specific ion unbinds and binds to the probe respectively have been reported for detection of Fe3+ ions in water samples based on this mechanism.24,25 Still most of the reported probes show some fragility and insufficiency like interference of other metal ions and poor sensitivity.26 However, the present work on such probes for Fe3+ detection in human blood samples is first of its kind. Turn-on fluorescent probes favor high selectivity, sensitivity and anti-interference, hence it demands the synthesis of new fluorescent probes for the detection of Fe3+ ions.27 Herewith, we report an excellent biocompatible rhodamine 6G-derivative fluorescent probe named RG5NC as a turn-on fluorescent sensor with high selectivity and sensitivity (in nM range) towards Fe3+ in the existence of various other metal ions. The probe exhibited negligible cytotoxicity.
2. Experimental
The materials and methods used for synthesis of probe (RG5NC) and its Fe3+ complex can be found in ESI 1–9,† wherein Schemes 1 and 2† explains the reactions involved. Details of preparation of stock solution for spectral determination, absorption and emission studies are explained in ESI 10 and 11.†
2.1 Detection of Fe3+ in real samples and human blood samples
Industrial waste water was collected from paper and plastic industry from Harohalli Industrial Area, Bangalore rural, Karnataka, India. Human blood samples were collected from Arunodaya Polyclinic, Harohalli, Bangalore and samples were digested as per the established standard protocol28 and the obtained blood serum was used for further studies. Details of the certified reference material is attached in ESI 12.†
2.2 Cell viability assessment
The NIH 3T3 mouse embryonic fibroblast cells (ATCC) were used for the cell culture experiments for testing cytocompatibility of the composite. The cells were grown in 25 cm2 culture flasks with DMEM, supplemented with 10% FBS and 1% antibiotic/antimycotic solution. Trypsinization of confluent cells in the culture flask was carried out using trypsin (0.25% trypsin–EDTA solution). The cells were counted using a hemocytometer (Improved Neubauer cell counter). Tissue culture polystyrene (TCPS) well plates were used as control in all the cell culture experiments.
The cellular viability after incubation of cells with different Fe complex concentration was quantified by MTT assay. In this assay, formazan crystals are formed when metabolically active cells react with MTT salt by the activity of oxidoreductase enzymes in the mitochondria. The concentration of the color formed by the formazan is directly proportional to the percentage of viable cells. Before adding MTT solution to the cell culture dishes, the cells were washed with PBS in order to remove any probe/complex present in the cell culture solution. Additionally, after incubation of cells with MTT solution, formed formazan crystals were dissolved in DMSO followed by centrifugation and OD was taken of the supernatant to avoid any interference during OD measurement. For MTT assay, 10
000 cells were seeded in each well of 96 well plate and incubated with Fe complex with various concentrations. After 24 h and 72 h of incubation, 0.5 mg mL−1 of the MTT solution was added to each well and incubated for a period of 2 h. The formazan crystals formed were later solubilized in DMSO and the optical density was recorded at 570 nm using an ELISA plate reader (Tecan InfiniteVR M1000 PRO). The MTT assay was repeated at least three times.
The cellular morphology and intracellular fluorescence after uptake of Fe complex were observed using fluorescence microscope (INCell Analyzer 6000, GE Healthcare Life Sciences, USA). The cells were grown in 24 well plate with Fe complex or ligand for 24 h and thereafter fixed with 3.7% formaldehyde in 1× PBS for 20 min and Hoechst 33258 dye was used to stain the nucleus of the cells. The cells were stained only using Hoechst 33258 dye which binds DNA of the cells and gives blue fluorescence. The fluorescence in green and red channels by cells is resulting in cellular uptake of the complex which is absent in the cells incubating without complex. All the experimental data, obtained using MTT assay are expressed as mean ± standard deviation (SD) and were analyzed by one-way ANOVA (SPSS 16.0) for the calculation of significance level of the experimental data. The differences were considered statistically significant, when p ≤ 0.05.
3. Results and discussions
3.1 Selectivity study
The selectivity of RG5NC in MeCN solution was examined with different metal ions (like Al3+, Ca2+, Zn2+, Cu2+, Ni2+, Cd2+, Pb2+, Fe3+, Cr3+, Hg2+ and Co2+). As shown in Fig. 1a, after coordination of Fe3+ with RG5NC, the probe exhibited notably strong and high selective ‘OFF–ON’ absorption at λmax = 529 nm. The free probe remained colorless when no Fe3+ ions were added into the solution. The solution color changed from colorless to orangish pink upon addition of Fe3+ ions into the RG5NC solution (inset Fig. 1b). Whereas, the other metal ions did not incite any visible color change. These results designates that RG5NC shows excellent selectivity for Fe3+ in MeCN solution.
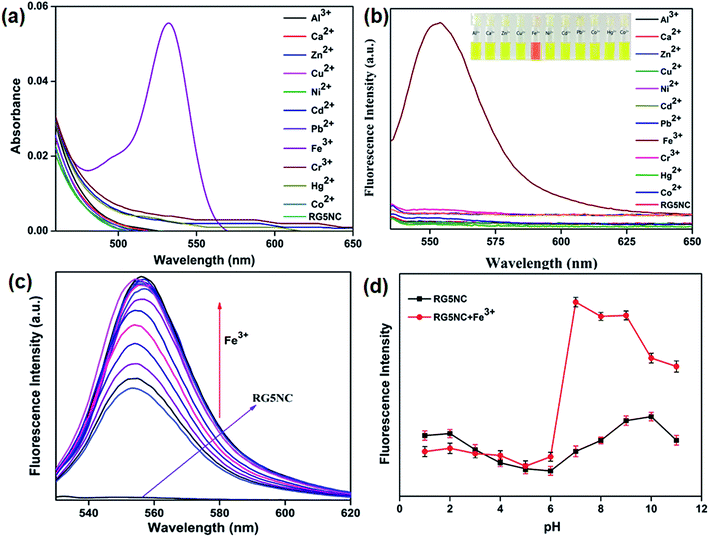 |
| Fig. 1 (a) UV-vis spectra of RG5NC with different metal ions in MeCN. (b) Fluorescence spectra of RG5NC with different metal ions in MeCN solution. Inset: Picture depicting color change of RG5NC upon addition of various metal ions (c) fluorescence spectra of RG5NC for different concentrations of Fe3+ in MeCN solution. (d) Maximum fluorescence intensity of RG5NC at 553 nm in the absence and presence of Fe3+ at different pH. | |
To attain further thorough analysis on the selectivity of RG5NC for different metal ions, the change in the fluorescence intensity upon the addition of metal ions under the same condition was also examined by using fluorescence spectra. The fluorescence spectra of RG5NC in MeCN exhibited no fluorescence at 553 nm for free probe. Upon addition of Fe3+ to the RG5NC solution, a significant fluorescence enhancement was observed as shown in Fig. 1b. All other metal ions did not display any fluorescence enhancement under similar conditions. These aspects indicate that opening of RG5NC spirolactam ring is due to the Fe3+ induced delocalization of xanthene moiety by chelation enhanced fluorescence (CHEF) mechanism and this reveal that RG5NC is a highly selective fluorescence chemosensor for Fe3+ and can be applied to biological sensing. The fluorescence quantum yield were calculated to be 2% and 78% in the absence and presence of Fe3+ ions (300 μM) respectively with rhodamine 6G as standard (ΦF = 0.95 in ethanol),.29
To gain more insight into the binding behavior of RG5NC with Fe3+, fluorescence titrations of Fe3+ against RG5NC was monitored in MeCN solution. As seen in Fig. 1c, the free probe RG5NC did not show any fluorescence at 553 nm. Upon addition of Fe3+, it leads to a remarkable increase in the emission intensities and reaches maximum at 300 μM. Since our main focus of the study was for sensing of biological samples and bio imaging it was necessary that the sensor is acceptable for physiological pH, hence, we evaluated the fluorescence response of RG5NC in presence and absence of Fe3+ at different pH values ranging from 1 to 11 as shown in Fig. 1d. The fluorescence OFF–ON worked well in the pH range 6 to 9, suggesting that the sensor RG5NC could be easily used for determining Fe3+ in biological samples at physiological pH.
The limit of detection for this fluorescent probe was evaluated. Each spectrum was recorded at λex/em = 550/570 nm. As shown in Fig. 2a, the Fe3+ concentration was varied over the range of 0.10–20 μM. The calculated detection limit of Fe3+ is 8.2 × 10−9 M with a good linear regression (R = 0.992).30
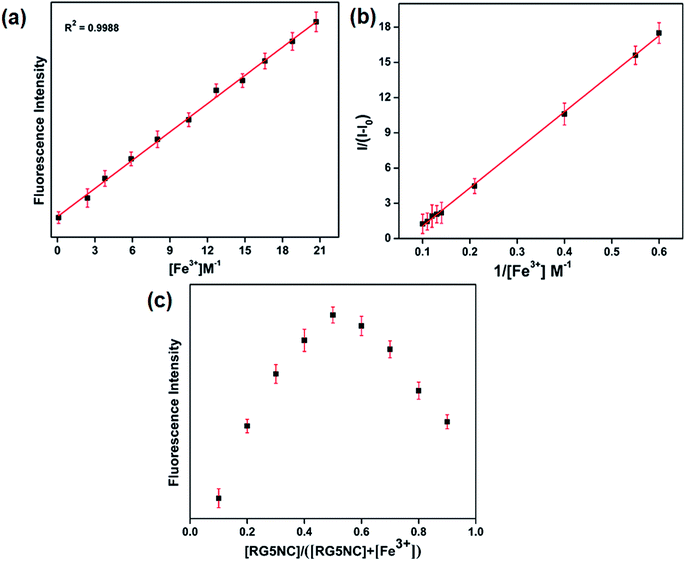 |
| Fig. 2 (a) Fluorescence spectra of RG5NC with various concentration of Fe3+ in MeCN solution varying from 0–20 μM (b) Benesi–Hildebrand plot to evaluate binding constant. (c) Job's plot with a total concentration of [RG5NC] + [Fe3+] = 20 μM. | |
Further, binding constant of RG5NC with Fe3+ was calculated using a Benesi–Hildebrand plot.31 The results in Fig. 2b shows a plot of 1/(I − I0) versus 1/[Fe3+] and yields a binding constant (Ka) value as 3.3 × 104 M−1 (R2 = 0.992) suggesting the sensor binding ability between the probe and Fe3+ ion. The complexation ratio of RG5NC with Fe3+ was also explained in MeCN using Job's plot. The molar concentration of Fe3+ was varied from 0 to 0.9 in a solution containing [Fe3+] + [RG5NC] and the total concentration of RG5NC with Fe3+ was 20 μM. The obtained results suggests that the fluorescence intensity reached a maximum value when the molar fraction of Fe3+ is 0.5 (Fig. 2c), indicating that the Fe3+ complexes with RG5NC is in 1
:
1 binding ratio.
In order to further investigate the selectivity of RG5NC for Fe3+, its selectivity for Fe3+ in presence of other competitive metal cations were examined under same conditions. Fig. 3a depicts changes in the emission spectra of RG5NC. The detection was experimented in the presence/absence of other competitive metal cations. These results evidently indicates the noninterference of other competitive metal cations during selective sensing of Fe3+. One of the important parameters in developing a novel probe for practical and on field applications is its reversibility. The reversible interaction between RG5NC and Fe3+ was confirmed by the addition of AcO− ions into the [RG5NC–Fe3+] complex. The experimental results exhibited color change from orangish red to colorless upon introduction of AcO− ions forming iron acetate. Concurrently, about 95% of the fluorescence intensity was quenched. Then the fluorescence intensity was originally regained on addition of Fe3+ into the mixture. This process was repeated at least five times to suggest the reversibility of probe (Fig. 3b).
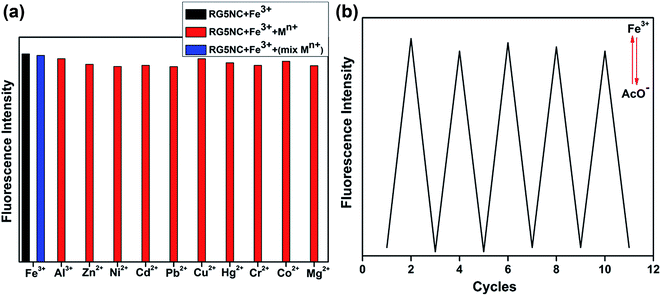 |
| Fig. 3 (a) Selectivity of RG5NC for Fe3+ in presence of other metal cations. (b) Fluorescence spectra of RG5NC after sequential addition of Fe3+ and 1 : 1 AcO− solution. | |
4. Cytocompatibility and cell imaging studies
The cytocompatibility of [RG5NC–Fe3+] complex was confirmed with NIH 3T3 cells in vitro. No significant difference was observed in the cell viability up to 80 μM concentration of [RG5NC–Fe3+] complex in the media (after 1 day of incubation) when compared to the control (Fig. 4). However, at higher concentration (100 μM) the viability of cells was less compared to control. Interestingly, this difference in the viability disappeared when cells were allowed to grow with the [RG5NC–Fe3+] complex for 3 days. Initially, intracellular stress is induced because of higher concentration of [RG5NC–Fe3+] complex. However, with time, cells recover and start growing like control even at higher concentration of [RG5NC–Fe3+] complex.
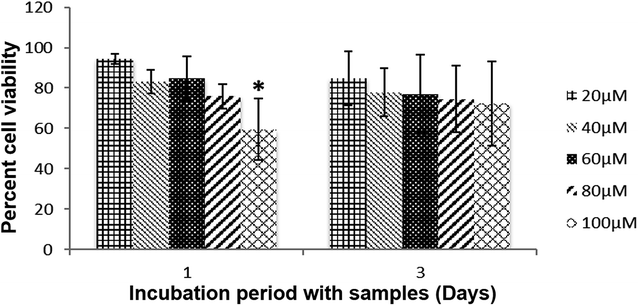 |
| Fig. 4 NIH 3T3 cell viability grown with Fe complex. Error bars represent mean ± SD. Asterisk shows the significant difference at p ≤ 0.05 with respect to control. | |
The main aspect of the study was to detect the presence of Fe3+ in NIH 3T3 mouse embryonic fibroblast cells in vitro. Two different sets of cells, one were incubated with [RG5NC–Fe3+] and the other treated with just the probe. The latter set of cells did not show any fluorescence signal (Fig. 5) while the other exhibited fluorescence. Additionally the intensity of the signal was observed to increase with increase in concentration of the [RG5NC–Fe3+] uptake. The cells could efficiently uptake the probe and fluoresce illustrating its ability to act as sensor to detect Fe3+ in vitro.
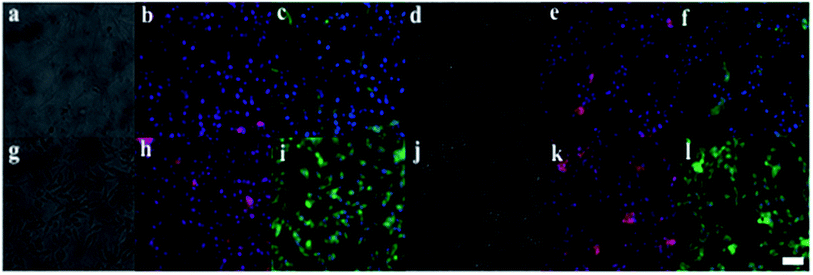 |
| Fig. 5 Representative fluorescence images of NIH 3T3 cells after 24 h grown with 20 μM RG5NC (a) bright field, (b) red channel (c) green channel; 100 μM (d) bright field, (e) red channel (f) green channel; 20 μM [RG5NC–Fe3+] complex (g) bright field, (h) red channel (i) green channel and 100 μM Fe complex (j) bright field, (k) red channel (l) green channel. Scale bar is 50 μm. | |
4.1 Detection of Fe3+ in real samples
Further, we tested the applicability of this probe RG5NC for the detection of Fe3+ ions in real samples. We have used this probe to detect Fe3+ in industrial effluents, tap water, and Iron tablet (Irozorb) and Iron syrup (Orofex XT). In all the real samples significant fluorescence dependence on concentration was observed with a good liner response. Importantly, trace levels of metal ions could be detected using this probe RG5NC which ensured the potential analysis of Fe3+ in real sample sources. The obtained results were also validated by AAS and ICP-OES methods respectively. It is important to mention that testing of commercially available Iron syrup and Iron tablet for Fe3+ was examined using this probe. Each sample was analyzed with three of their replicates. The estimated detection limits have been tabulated in Table 1.
Table 1 Determination of Fe3+ in real samples
Samples |
Fe3+ found by AAS method (M) |
RSD (%) |
Fe3+ found by present method (M) |
SE |
Iron syrup |
9.12 × 10−5 |
2.24 |
6.08 × 10−5 |
2.11 |
Iron tablet |
1.16 × 10−4 |
2.10 |
1.05 × 10−5 |
1.91 |
Industrial effluent1 |
8.38 × 10−4 |
2.81 |
7.50 × 10−4 |
1.17 |
Industrial effluent2 |
6.24 × 10−4 |
1.62 |
6.28 × 10−4 |
1.53 |
Tap water |
2.21 × 10−4 |
2.54 |
1.55 × 10−4 |
2.76 |
4.2 Response of RG5NC towards human blood serum
We have investigated the use of the probe towards the detection of Fe3+ ion in blood serum samples. In order to investigate the sensitivity of the probe RG5NC towards Fe3+ similar titration protocol was followed. Even in high serum condition the probe showed effective detection response towards Fe3+. A linear change in the emission with good linear regression at 553 nm was observed. The control experiment was also performed to study the interaction of the probe RG5NC with human blood serum under similar conditions. The obtained values detected from this method matched well with the mM values of Fe3+ ion given in certified reference material (CRM). The results were also validated by AAS and ICP-OES methods respectively. The experiments were conducted in triplicates. The determined Fe3+ content in human blood samples have been tabulated in Table 2.
Table 2 Determination of Fe3+ in various human blood serum samples using RG5NCa
Samples |
Fe3+ found by ICP_OES method (M) |
RSD (%) |
Fe3+ found by present method (M) |
SE |
CBRM: certified blood reference material, BS: blood serum samples. |
CBRM1 |
5.96 × 10−2 |
3.64 |
5.67 × 10−2 |
1.81 |
CBRM2 |
5.94 × 10−2 |
1.70 |
5.63 × 10−2 |
1.23 |
CBRM3 |
5.92 × 10−2 |
2.02 |
5.62 × 10−2 |
1.57 |
BS1 |
5.99 × 10−2 |
1.94 |
5.87 × 10−2 |
0.76 |
BS2 |
6.01 × 10−2 |
1.17 |
5.83 × 10−2 |
0.95 |
BS3 |
6.05 × 10−2 |
1.63 |
5.85 × 10−2 |
1.84 |
5. Fast track detection of Fe3+ using test strips
Assuring purity of drinking water and consumable food materials in remote areas is a challenging task where laboratory facilities are not available. Hence portable test strips were prepared for quick on-filed detection of Fe3+ ions. For this purpose test strips were soaked in MeCN solution of RG5NC and then dried in air before they were used for detection of Fe3+ in water. A distinct color change was observed immediately upon dipping the test strips in Fe3+ ion solution (Fig. 6). Presence of Fe3+ selectively changed the test-strips color to orangish red. This newly developed sensor system provide an alternative method to confirm the nature as well as the extent of metal ion induced toxicity in natural water sources.
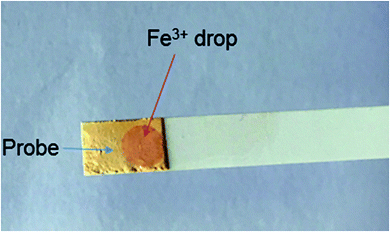 |
| Fig. 6 Photograph of the test strip of this probe RG5NC during the detection of Fe3+. | |
A comparison of the applicability and analytical section of this probe with some of the previous reports in terms of their solubility and detection limit32 is shown in Table 3.
Table 3 Comparison of our probe performance with various other reported probes
Probe |
Detection limit |
Reference |
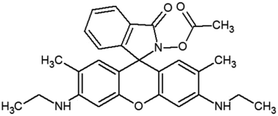 |
1.42 × 10−6 M |
32a |
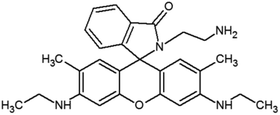 |
0.03 × 10−6 M |
32b |
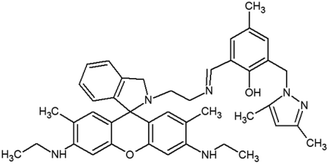 |
0.29 × 10−6 M |
32c |
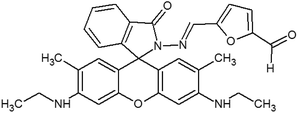 |
17 × 10−9 M |
32d |
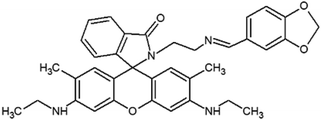 |
1.18 × 10−8 M |
32e |
Present work |
8.2 × 10−9 M |
— |
6. Conclusion
The synthesized probe showed an outstanding sensitivity and selectivity for Fe3+ in human blood serum samples and excellent uptake by cells for imaging applications. The detection limit was about 8.2 nM. Spectral changes were observed to be reversible with respect to spirolactam ring opening. Confocal laser scanning microscopy experiments showed that RG5NC could be used to detect Fe3+ in live cells. The probe sensitivity of metal ion recognition was investigated in human blood serum. The detection as well as the discrimination of this toxic metal ion was also achieved in real samples at nano molar level. In addition we have also demonstrated this detection of Fe3+ ions using portable test strips. The significance of this study lies in applying such fluorescent probes for rapid on field detection of Fe3+ in human blood serum and live cells.
Conflicts of interest
There are no conflicts to declare.
Acknowledgements
The authors acknowledge Dr Vijayalakshmi S, Scientist, IGCAR, Kalpakkam, Tamilnadu, India for constant support and timely help. The BRNS, DAE, India for financial assistance (Project Sanction No. 37(2)14/06/2014-BRNS) and the Institute of Excellence, Vijnana Bhavana, University of Mysore, India for providing the Nuclear Magnetic Resonance (NMR) and Liquid Crystal Mass Spectrometry (LC-MS) facility also TUV India Private Limited, Bengaluru, India for providing ICP-OES instrumentation facility.
References
- Y. Liu, R. Shen, J. Ru, X. Yao, Y. Yang, H. Liu, X. Tanga, D. Bai, G. Zhang and W. Liu, A reversible rhodamine 6G-based fluorescence turn-on probe for Fe3+ in water and its application in living cells imaging, RSC Adv., 2016, 6(113), 111754–111759 RSC.
- C. Wang, Y. Huang, K. Jiang, M. G. Humphrey and C. Zhang, Dual-emitting quantum dots/carbon nanodots-based nanoprobe for selective and sensitive detection of Fe3+ in cells, Analyst, 2016,(2013), 1–3 Search PubMed.
- S. Hu, S. Zhang, C. Gao, C. Xu and Q. Gao, A new selective fluorescent sensor for Fe3+ based on a pyrazoline derivative, Spectrochim. Acta, Part A, 2013, 113(2013), 325–331 CrossRef CAS PubMed.
-
(a) X. Qu, Q. Liu, X. Ji, H. Chen, Z. Zhou and Z. Shen, Enhancing the Stokes' shift of BODIPY dyes via through-bond energy transfer and its application for Fe3+-detection in live cell imaging, Chem. Commun., 2012, 48(2012), 4600–4602 RSC;
(b) Y. Guo, F. Cao and Y. Li, Solid phase synthesis of nitrogen and phosphor co-doped carbon quantum dots for sensing Fe3+ and the enhanced photocatalytic degradation of dyes, Sens. Actuators, B, 2017, 1105–1111 Search PubMed.
- Q. Yang, W. Lin, X. Zheng and L. Xiao, Single Particle Dynamic Imaging and Fe3+ Sensing with Bright Carbon Dots Derived from Bovine Serum Albumin Proteins, Sci. Rep., 2015, 5, 17727 CrossRef CAS PubMed.
-
(a) H. R. Chandan and B. R. Geetha, Study on precipitation efficiency of solvents in postpreparative treatment of nanocrystals, J. Mater. Res., 2013, 28(21), 3003–3009 CrossRef CAS;
(b) H. R. Chandan, M. Venkataramana, M. D. Kurkuri and R. G. Balakrishna, Simple quantum dot bioprobe/label for sensitive detection of Staphylococcus aureus TNase, Sens. Actuators, B, 2016, 222, 1201–1208 CrossRef CAS.
- H. Chandan, V. Saravanan, R. K. Pai and R. G. Balakrishna, Synergistic effect of binary ligands on nucleation and growth/size effect of nanocrystals: Studies on reusability of the solvent, J. Mater. Res., 2014, 29(14), 1556–1564 CrossRef CAS.
- L. P. D'Souza, V. Amoli, H. R. Chandan, A. K. Sinha, R. Krishna Pai and G. R. Balakrishna, Atomic force microscopic study of nanoscale interaction between N719 dye and CdSe quantum dot in hybrid solar cells and their enhanced open circuit potential, Sol. Energy, 2015, 116, 25–36 CrossRef.
- R. M. Renuka, J. Achuth, H. R. Chandan, M. Venkataramana and K. Kadirvelu, A fluorescent dual aptasensor for the rapid and sensitive onsite detection of E. coli O157:H7 and its validation in various food matrices, New J. Chem., 2018, 42(13), 10807–10817 RSC.
- H. R. Chandan, J. D. Schiffman and R. G. Balakrishna, Quantum dots as fluorescent probes: Synthesis, surface chemistry, energy transfer mechanisms, and applications, Sens. Actuators, B, 2018, 258, 1191–1214 CrossRef CAS.
- C. Hunsur Ravikumar, M. Ira Gowda and R. G. Balakrishna, An “OFF–ON” quantum dot–graphene oxide bioprobe for sensitive detection of micrococcal nuclease of Staphylococcus aureus, Analyst, 2019, 3999–4005 RSC.
- X. Pu, B. Hu, Z. Jiang and C. Huang, Speciation of dissolved iron(II) and iron(III) in environmental water samples by gallic acid-modified nanometer-sized alumina micro-column separation and ICP-MS determination, Analyst, 2005, 130(8), 1175–1181 RSC.
- N. Mir, P. Karimi, C. E. Castano, N. Norouzi, J. V. Rojas and R. M., Functionalizing Fe3O4@SiO2 with a novel mercaptobenzothiazole derivative: Application to trace fluorometric and colorimetric detection of Fe3+ in water, Appl. Surf. Sci., 2019, 876–888 CrossRef CAS.
- Z. Zuo, X. Song, D. Guo, Z. Guo and Q. Niu, A dual responsive colorimetric/fluorescent turn-on sensor for highly selective, sensitive and fast detection of Fe3+ ions and its applications, J. Photochem. Photobiol., B, 2019, 111876 CrossRef CAS.
- H. Y. Lee, D. R. Bae, J. C. Park, H. Song, W. S. Han and J. H. Jung, A Selective Fluoroionophore Based on BODIPY-functionalized Magnetic Silica Nanoparticles: Removal of Pb2+ from Human Blood, Angew. Chem., Int. Ed., 2009, 48(2009), 1239–1243 CrossRef CAS PubMed.
- M. Park, S. Seo, S. J. Lee and J. H. Jung, Functionalized Ni@SiO2 core/shell magnetic nanoparticles as a chemosensor and adsorbent for Cu2+ ion in drinking water and human blood, Analyst, 2010, 135(2010), 2802–2805 RSC.
- L. Liu, X. Dong, Y. Xiao, W. Lian and Z. Liu, Two-photon excited fluorescent chemosensor for homogeneous determination of copper(II) in aqueous media and complicated biological matrix, Analyst, 2011, 136(2011), 2139–2145 RSC.
- J. Hatai, S. Pal and S. Bandyopadhyay, An inorganic phosphate (Pi) sensor triggers ‘turn-on’ fluorescence response by removal of a Cu2+ ion from a Cu2+-ligand sensor: determination of Pi in biological samples, Tetrahedron Lett., 2012, 53(2012), 4357–4360 CrossRef CAS.
- V. V. Halali, M. Saxena, H. R. Chandan, A. A. Ojha and R. G. Balakrishna, Paper based field deployable sensor for naked eye monitoring of copper(II) ions; elucidation of binding mechanism by DFT studies, Spectrochim. Acta, Part A, 2019,(2019), 117291 Search PubMed.
- J. Li, Q. Wang, Z. Guo, H. Ma, Y. Zhang, B. Wang, D. Bin and Q. Wei, Highly selective fluorescent chemosensor for detection of Fe3+ based on Fe3O4@ZnO, Sci. Rep., 2016, 6, 23558 CrossRef CAS PubMed.
-
(a) L. Yang, W. Zhu, M. Fang, Q. Zhang and C. Li, A new carbazole-based Schiff-base as fluorescent chemosensor for selective detection of Fe3+ and Cu2+, Spectrochim. Acta, Part A, 2013, 109(2013), 186–192 CrossRef CAS PubMed;
(b) V. K. Gupta, N. Mergu and L. K. Kumawat, A new multifunctional rhodamine-derived probe for colorimetric sensing of Cu(II) and Al(III) and fluorometric sensing of Fe(III) in aqueous media, Sens. Actuators, B, 2016, 223(2016), 101–113 CrossRef CAS.
-
(a) S. Ma, Z. Yang, M. She, W. Sun, B. Yin, P. Liu, S. Zhang and J. Li, Design and synthesis of functionalized rhodamine based probes for specific intracellular fluorescence imaging of Fe3+, Dyes Pigm., 2015, 115(2015), 120–126 CrossRef CAS;
(b) F. Yan, T. Zheng, D. Shi, Y. Zou, Y. Wang, M. Fu, L. Chen and W. Fu, Rhodamine-aminopyridine based fluorescent sensors for Fe3+ in water: Synthesis, quantum chemical interpretation and living cell application, Sens. Actuators, B, 2015, 215(2015), 598–606 CrossRef CAS;
(c) M. S. Moorthy, H.-B. Kim, A.-R. Sung, J.-H. Bae, S.-H. Kim and C.-S. Ha, Fluorescent mesoporous organosilicas for selective monitoring of Hg2+ and Fe3+ ions in water and living cells, Microporous Mesoporous Mater., 2014, 194(2014), 219–228 CrossRef CAS;
(d) Y.-H. He, J.-P. Lai, S. Hui, Z.-M. Chen and S. Lan, A fast, sensitive and stable fluorescent fiber-optic chemosensor for quantitative detection of Fe3+ in real water and HepG2 living cells, Sens. Actuators, B, 2015, 225(2016), 405–412 Search PubMed;
(e) C.-Y. Li, C.-X. Zou, Y.-F. Li, J.-L. Tang and C. Weng, A new rhodamine-based fluorescent chemosensor for Fe3+ and its application in living cell imaging, Dyes Pigm., 2014, 104(2014), 110–115 CrossRef CAS;
(f) S. Chan, Q. Li, H. Tse, A. W. M. Lee, N. K. Mak, H. L. Lung and W.-H. Chan, Rhodamine-based “off–on” fluorescent chemosensor for selective detection of Fe3+ in aqueous media and its application in bioimaging, RSC Adv., 2016, 74389–74393 RSC;
(g) X. Jin, S. Wang, W. Yin, T. Xu, J. Yang, L. Qi, X. Xia and J. Liu, A highly sensitive and selective fluorescence chemosensor for Fe3+ based on rhodamine and its application in vivo imaging, Sens. Actuators, B, 2017, 247(2017), 461–468 CrossRef CAS;
(h) F. Zhou, T.-H. Leng, Y.-J. Liu, C.-Y. Wang, P. Shi and W.-H. Zhu, Water-soluble rhodamine-based chemosensor for Fe3+ with high sensitivity, selectivity and anti-interference capacity and its imaging application in living cells, Dyes Pigm., 2017, 429–436 CrossRef CAS;
(i) S. Adhikari, A. Ghosh, M. Ghosh and D. D. Subhajit Guria, Ratiometric sensing of Fe3+ through PET-CHEF-FRET processes: live cell imaging, speciation and DFT studies, Sens. Actuators, B, 2017, 942–950 CrossRef CAS;
(j) S. K. Dwivedi, R. C. Gupta, R. Ali, S. S. Razi, S. K. H., P. P. Manna and A. Misra, Smart PET based organic scaffold exhibiting bright “Turn-On” green fluorescence to detect Fe3+ ion: Live cell imaging and logic implication, J. Photochem. Photobiol., B, 2018, 157–166 CrossRef CAS;
(k) B. Lim, B. Baek, K. Jang, N. K. Lee, J. H. Lee, Y. Lee, J. Kim, S. W. Kang, J. Park, S. Kim, N.-W. Kang, S. Hong, D.-D. Kim, I. Kim, H. Hwang and J. Lee, Novel turn-on fluorescent biosensors for selective detection of cellular Fe3+ in lysosomes: Thiophene as a selectivity-tuning handle for Fe3+ sensors, Dyes Pigm., 2019, 51–59 CrossRef CAS;
(l) W. Wang, M. Wu, H. Liu, Q. Liu, Y. Gao and B. Zhao, A novel on–off–on fluorescent chemosensor for relay detection of Fe3+ and PPi in aqueous solution and living cells, Tetrahedron Lett., 2019, 1631–1635 CrossRef CAS;
(m) X. Gong, H. Zhang, N. Jiang, L. Wang and G. Wang, Oxadiazole-based ‘on–off’ fluorescence chemosensor for rapid recognition and detection of Fe2+ and Fe3+ in aqueous solution and in living cells, Microchem. J., 2019, 145(2019), 435–443 CrossRef CAS.
-
(a) D. T. Quang and J. S. Kim, Fluoro- and Chromogenic Chemodosimeters for Heavy Metal Ion Detection in Solution and Biospecimens, Chem. Rev., 2010, 110(10), 6280–6301 CrossRef CAS PubMed;
(b) Y. Yang, Q. Zhao, W. Feng and F. Li, Luminescent Chemodosimeters for Bioimaging, Chem. Rev., 2013, 113(1), 192–270 CrossRef CAS PubMed.
-
(a) V. K. G. Kumar, N. Mergu and A. Singh, Rhodamine-derived highly sensitive and selective colorimetric and off–on optical chemosensors for Cr3+, Sens. Actuators, B, 2015, 220(2015), 420–432 Search PubMed;
(b) S. Sun, B. Qiao, N. Jiang, J. Wang, S. Zhang and X. Peng, Naphthylamine–Rhodamine-Based Ratiometric Fluorescent Probe for the Determination of Pd2+ Ions, Org. Lett., 2013, 1132–1135 Search PubMed;
(c) S.-K. Ko, Y.-K. Yang, J. Tae and I. Shin, In Vivo Monitoring of Mercury Ions Using a Rhodamine-Based Molecular Probe, J. Am. Chem. Soc., 2006, 128(2006), 14150–14155 CrossRef CAS PubMed;
(d) F. Ge, H. Ye, H. Zhang and B.-X. Zhao, A novel ratiometric probe based on rhodamine B and coumarin for selective recognition of Fe(III) in aqueous solution, Dyes Pigm., 2013, 99, 661–665 CrossRef CAS;
(e) X. Li, Y. Yin, J. Deng, H. Zhong, J. Tang, Z. Chen, L. Yang and L.-J. Ma, A solvent-dependent fluorescent detection method for Fe3+ and Hg2+ based on a rhodamine B derivative, Talanta, 2016, 154, 329–334 CrossRef CAS PubMed;
(f) W. Yin, H. Cui, Z. Yang, C. Li, M. She, B. Yin, J. Li, G. Zhao and Z. Shi, Facile synthesis and characterization of rhodamine-based colorimetric and “off–on” fluorescent chemosensor for Fe3+, Sens. Actuators, B, 2011, 157(2), 675–680 CrossRef CAS;
(g) M. Chai, M. Li, D. Zhang, C.-c. Wang, Y. Ye and Y. Zhao, Three colorimetric and off–on fluorescent chemosensors for Fe3+ in aqueous media, Luminescence, 2013, 28(4), 557–561 CrossRef CAS PubMed;
(h) X. Han, D.-E. Wang, S. Chen, L. Zhang, Y. Guo and J. Wang, A new rhodamine-based chemosensor for turn-on fluorescent detection of Fe3+, Anal. Methods, 2015, 7(10), 4231–4236 RSC.
-
(a) B. Rathinam, C.-C. Chien, B.-C. Chen and J.-H. Liu, Fluorogenic and chromogenic detection of Cu2+ and Fe3+ species in aqueous media by rhodamine–triazole conjugate, Tetrahedron, 2013, 69(1), 235–241 CrossRef CAS;
(b) T. Geng, R. Huang and D. Wu, Turn-on fluorogenic and chromogenic detection of Fe3+ and Cr3+ in a completely water medium with polyacrylamide covalently bonding to rhodamine B using diethylenetriamine as a linker, RSC Adv., 2014, 4(86), 46332–46339 RSC;
(c) L. K. Kumawat, N. Mergu, M. Asif and V. K. Gupta, Novel synthesized antipyrine derivative based “Naked eye” colorimetric chemosensors for Al3+ and Cr3+, Sens. Actuators, B, 2016, 231, 847–859 CrossRef.
- B. Rathinam, C.C. Chien, B.-C. Chen and J.-H. Liu, Fluorogenic and chromogenic detection of Cu2+ and Fe3+ species in aqueous media by rhodamineetriazole conjugate, Tetrahedron, 2013, 69(2013), 235–241 CrossRef CAS.
-
(a) N. R. Chereddy, K. Suman, P. S. Korrapati, S. Thennarasu and A. B. Mandal, Design and synthesis of rhodamine based chemosensors for the detection of Fe3+ ions, Dyes Pigm., 2012, 95(3), 606–613 CrossRef CAS;
(b) C.-Y. Li, C.-X. Zou, Y.-F. Li, J.-L. Tang and C. Weng, A new rhodamine-based fluorescent chemosensor for Fe3+ and its application in living cell imaging, Dyes Pigm., 2014, 104, 110–115 CrossRef CAS.
-
(a) T. R. Mahalingam, S. Vijayalakshmi, R. K. Prabhu, A. Thiruvengadasami, C. K. Mathews and K. R. Shanmugasundaram, Studies on some trace and minor elements in blood. A survey of the Kalpakkam (India) population. Part I: Standardization of analytical methods using ICP-MS and AAS, Biol. Trace Elem. Res., 1997, 57(3), 191–206 CrossRef CAS;
(b) N. B. Ivanenko, A. A. Ganeev, N. D. Solovyev and L. N. Moskvin, Determination of Trace Elements in Biological Fluids, J. Anal. Chem., 2011, 900–915 Search PubMed;
(c) T. R. Mahalingam, S. Vijayalakshmi, R. Krishna Prabhu, A. Thiruvengadasami, A. Wilber, C. K. Mathews and K. R. Shanmugasundaram, Studies on Some Trace and Minor Elements in Blood, Biol. Trace Elem. Res., 1996 Search PubMed.
- K.-P. Wang, J.-P. Chen, S.-J. Zhang, Y. Lei, H. Zhong, S. Chen, X.-H. Zhou and Z.-Q. Hu, Thiophene-based rhodamine as selective fluorescence probe for Fe(III) and Al(III) in living cells, Anal. Bioanal. Chem., 2017, 409(23), 5547–5554 CrossRef CAS PubMed.
- H. L. Yan Gao, Q. Liu and W. Wang, A novel colorimetric and OFF–ON fluorescent chemosensor based on fluorescein derivative for the detection of Fe3+ in aqueous solution and living cells, Tetrahedron Lett., 2016, 1852–1855 Search PubMed.
- Y. Liu, R. Shen, J. Ru, X. Yao, Y. Yang, H. Liu, X. Tang, D. Bai, G. Zhang and W. Liu, A reversible rhodamine 6G-based fluorescence turn-on probe for Fe3+ in water and its application in living cell imaging, RSC Adv., 2016, 111754–111759 RSC.
-
(a) Z.-Q. Hu, Y.-Y. Gu, W.-Z. Hu, L.-L. Sun, J.-H. Zhu and Y. Jiang, A Highly Selective and Sensitive Turn-On Fluorescent Chemosensor Based on Rhodamine 6G for Iron(III), ChemistryOpen, 2014, 3(6), 264–268 CrossRef CAS;
(b) B. Wang, J. Hai, Z. Liu, Q. Wang, Z. Yang and S. Sun, Selective Detection of Iron(III) by Rhodamine-Modified Fe3O4 Nanoparticles, Angew. Chem., Int. Ed., 2010, 4576–4579 CrossRef CAS PubMed;
(c) R. Alam, R. Bhowmick, A. S. M. Islam, A. katarkar, K. Chaudhuri and M. Ali, A rhodamine based fluorescent trivalent sensor (Fe3+,Al3+,Cr3+) with potential applications for a live cell imaging and combinatorial logic circuit and memory device, New J. Chem., 2017, 41, 8359–8369 RSC;
(d) A. Kumar, C. Kumari, D. Sain, S. K. Hira, P. P. Manna and S. Dey, Synthesis of Rhodamine-Based Chemosensor for Fe3+ Selective Detection with off–on Mechanism and its Biological Application in DL-Tumor Cells, ChemistrySelect, 2017, 2(2017), 2969–2974 CrossRef CAS;
(e) W. Sun, X. Cao, F. Zhang, Y. Bai and X. Ding, A Highly Selective “Turn-on” Fluorescent Probe for Detection of Fe3+ in Cells, J. Fluoresc., 2019, 425–434 Search PubMed.
Footnote |
† Electronic supplementary information (ESI) available. See DOI: 10.1039/c9ra05256a |
|
This journal is © The Royal Society of Chemistry 2019 |
Click here to see how this site uses Cookies. View our privacy policy here.