DOI:
10.1039/C9RA05005A
(Paper)
RSC Adv., 2019,
9, 25750-25761
Synthesis of a well-dispersed CaFe2O4/g-C3N4/CNT composite towards the degradation of toxic water pollutants under visible light†
Received
2nd July 2019
, Accepted 30th July 2019
First published on 15th August 2019
Abstract
Herein, we fabricated a ternary photocatalyst composed of CaFe2O4, multiwalled carbon nanotubes (CNTs) and graphitic carbon nitride (g-C3N4) via a simple hydrothermal route. CaFe2O4 acted as a photosensitizer medium and the CNT acted as a co-catalyst, which remarkably enhanced the photocatalytic performances of g-C3N4 towards the degradation of hexavalent chromium (Cr(VI)) and the antibiotic tetracycline (TC) under visible light irradiation. To investigate the morphological and topological features of the photocatalyst, field-emission scanning electron microscopy (FE-SEM) and transmission electron microscopy (TEM) analyses were performed. The surface properties and oxidation state of the CaFe2O4/g-C3N4/CNT composite were determined by X-ray photoelectron spectroscopy (XPS). The recombination rate of the charge carriers and the band gap values of the as-synthesized catalysts were analyzed by photoluminescence spectroscopy (PL) and diffused reflectance spectroscopy (UV/Vis DRS) studies, respectively. Besides the degradation reactions, the high hydrogen production rate of 1050 μmol h−1 under visible light using the CaFe2O4/g-C3N4/CNT composite loaded with 5 wt% CNT was observed. The superior photocatalytic performances of the CaFe2O4/g-C3N4/CNT composite can be ascribed to the effective heterojunction formed between g-C3N4 and the CaFe2O4 matrix, in which the CNT act as a conducting bridge in the system, promoting the production of photoinduced charge carriers in the semiconductor system. Finally, the plausible photocatalytic mechanism towards the degradation of pollutants and hydrogen production was discussed carefully.
1. Introduction
In the past few decades, the reckless release of organic pollutants in water bodies has increased greatly, which cause severe environmental problems and health risks.1,2 Accordingly, the photocatalysis process using semiconductor photocatalysts has been proposed as a green technology to degrade the organic pollutants in water bodies, even at trace levels.3 Thus, semiconductor materials, such as TiO2, SnO2, and ZnO, have been investigated by various researchers owing to their low cost and ease of availability.4 However, their foremost drawbacks are their wide band gap energy, high recombination, and low visible light response, which limit their large-scale production in industry.
Recently, a polymeric semiconductor, g-C3N4, has emerged with visible light response, excellent quantum efficiency and photochemical stability, presenting a competitive candidate for the degradation of dyes, NOx removal, CO2 reduction, and water-splitting reactions.5 Unfortunately, the pure g-C3N4 semiconductor exhibits a sluggish performance due to its high recombination rate and poor absorption ability in the entire visible light region. Furthermore, its low quantum efficiency and turnover number (TON) are also considered a major obstacle.6 Thus, tremendous efforts have been made to resolve these problems, and a system mainly composed of well-matched semiconductors will be a good way to overcome these issues.7 The heterojunction between highly visible light active semiconductors with suitable band gap values is favorable for enhanced charge separation efficiency in g-C3N4 and dramatically enhances its light absorption ability in the entire visible region.8
Thus, calcium ferrite (CaFe2O4), as p-type visible light-active photocatalyst, has been investigated due to its low cost and toxicity with narrow band gap energy towards the degradation of pollutants in both the gaseous and liquid phase.9 Nevertheless, its major drawbacks such as inefficient hole transfer property, high recombination rate, and charge mobility result in a sluggish performance.10 Therefore, the CaFe2O4 photocatalysts suffered severely during water-splitting reactions and the degradation of pollutants. To solve these issues, Luo et al. developed a novel CaFe2O4/Bi2O3 composite via a simple wet chemical route. The photocatalytic properties of the CaFe2O4/Bi2O3 composite was evaluated through the degradation of malachite green (MG) dye under visible light irradiation.11 The higher photocatalytic efficiency can be attributed due to the improved separation of photogenerated electron–hole pairs through the incorporation of Bi2O3, which can be beneficial for a higher degradation performance. Liu et al. prepared a carbon-modified CaFe2O4 composite towards the degradation of methylene blue dye.12 The presence of carbon in CaFe2O4 greatly influenced the degradation rate of CaFe2O4 due to its excellent adsorption behavior.
Therefore, it is essential to develop simple and cost-effective methods to improve the photocatalytic ability of both CaFe2O4 and g-C3N4 without sacrificing their crystalline nature and structural stability. CNT are outstanding 1D nano-sized materials with abundant surface hydroxyl and carboxyl groups, which enable their solubility in polar solvents.13 Thus, anchoring CNT on the surface of CaFe2O4 and g-C3N4 can lead to a superior performance than that of the CaFe2O4 and g-C3N4 binary system. Besides, CNT exhibit a high specific surface area, excellent electron transfer ability, and can act as a noble metal-free co-catalyst in heterojunction systems.14 The CNT-enabled heterojunction system can afford extra active sites for the adsorption of target pollutants in degradation reactions. Inspired by this mechanism and to further improve the photo-degradation efficiency of both CaFe2O4 and g-C3N4, herein, we demonstrate a simple method for the development of a ternary photocatalyst.15 Upon the incorporation of CNT, the photocatalytic efficiency was significantly improved towards the hydrogen evolution reaction, and Cr(VI) and TC degradation to a great extent.
2. Experimental
2.1. Materials
All the chemicals used in this work were of analytical reagent (AR) grade. Dicyandiamide, melamine (C3H6N6), calcium nitrate tetrahydrate (Ca(NO3)2·4H2O), iron(III) nitrate nonahydrate (Fe(NO3)3·9H2O), potassium dichromate, tetracycline hydrochloride, glycerol, ethylene glycol, citric acid, ethanol, methanol and MWCNT were obtained from Sigma-Aldrich Corporation. Deionized (DI) was used throughout the synthesis and photocatalytic process.
2.2. Preparation of g-C3N4
g-C3N4 was prepared via a simple calcination route. In a typical synthesis, a stoichiometric amount of dicyandiamide and melamine (1
:
1 ratio) was added to a silica crucible and calcined at 550 °C for 4 h under a nitrogen atmosphere. Finally, the silica crucible was cooled naturally to obtain porous g-C3N4 sheets.
2.3. Preparation of CaFe2O4
CaFe2O4 was synthesized as follows method: a calculated amount of Ca(NO3)2·4H2O, Fe(NO3)3·9H2O, glycerol, ethylene glycol, and citric acid was mixed under ultrasound irradiation and stirred for 1 h at 80 °C. The obtained red color fluffy product was further calcined at 850 °C for 3 h under an air atmosphere to obtain the pure-phase CaFe2O4.
2.4. CaFe2O4/g-C3N4/CNTs composite
A simple hydrothermal route was adopted to synthesize the CaFe2O4/g-C3N4/CNT composite. Typically, the CNT and as-synthesized g-C3N4 were dispersed in 20 mL of DI water with the help of ultrasonication for 2 h. Then, a calculated weight percentage of CaFe2O4 powder was mixed with the above solution and transferred to a Teflon-lined autoclave, which was heated at 120 °C for 12 h. The final brown-colored precipitate was washed with DI water repeatedly and vacuum dried at 70 °C overnight.
2.5. Characterization
The CaFe2O4/g-C3N4/CNT composite was analyzed via X-ray diffraction (XRD) on a PANalytical X-ray diffractometer using CuKα radiation (λ = 1.5418 Å) in the 2θ range of 10–70°. The elemental purity and surface properties of the CaFe2O4/g-C3N4/CNT composite was examined via X-ray photoelectron spectroscopy (XPS) on an ESCALAB X-ray photoelectron spectrometer using MgKα radiation. The morphology and microstructures of the prepared samples were recorded via TEM (Tecnai G2 F20 transmission electron microscope), and field-emission scanning electron microscopy using an EM, Quanta FEG 250. UV-Vis diffuse reflectance spectra (DRS) of the samples were analyzed an a UV/Vis spectrophotometer (Jasco-V750 Japan) in the wavelength range of 200–900 nm using BaSO4 as an internal standard. Photoluminescence (PL) spectra were measured using a fluorescence spectrophotometer (F-7000, Japan).
2.6. Photocatalytic degradation reactions
The aqueous phase degradation of Cr(VI) and TC was performed in a quartz reactor under visible light irradiation (λ ≥ 420 nm) generated by a 300 W Xe lamp. The distance between the test solution and the lamp was fixed at 15 cm. In the degradation process, 0.1 g of powdered photocatalyst was mixed in 100 mL of Cr(VI) and TC with a concentration of 10 ppm. Before visible light irradiation, the suspension was kept in the dark with constant stirring for 20 min to attain equilibrium between the pollutants and photocatalyst. Subsequently, the degraded suspension was collected at given time periods and the absorbance maxima were analyzed by a UV-vis spectrophotometer.
2.7. Photocatalytic H2 evolution reactions
To measure the hydrogen production rate, the following procedure was adopted. Exactly 50 mg of CaFe2O4/g-C3N4/CNT composite was dispersed in 75 mL of 15 vol% lactic acid solution, and then purged with N2 for 30 min to remove the oxygen in the reactor vessel. A 4 W LED was applied as the visible-light source and continuous stirring was maintained to keep the photocatalyst suspended in the medium. The amount of hydrogen produced was measured by a gas chromatograph with a TCD detector. The AQY of the CaFe2O4/g-C3N4/CNTs was calculated using the following equation:
3. Results and discussion
Fig. 1 shows the XRD patterns of g-C3N4, CaFe2O4, and CaFe2O4/g-C3N4/CNT composite. Two apparent distinguishing peaks of g-C3N4 appeared at the 2θ values of 13.1° and 27.8°, which can be perfectly matched with the JCPDS card number 87-1526.16 All the peaks of CaFe2O4 correspond to the orthorhombic crystal phase and matched the standard card (JCPDS 32-0168).17 The strong interference peak of g-C3N4 at 27.5 was observed in the CaFe2O4/g-C3N4/CNT composite, confirming the successful formation of the heterojunction. Furthermore, the characteristic peaks of the CNT were not observed in the system, which can be attributed to their low concentration and poor crystallinity. Thus, the optical properties of the as-synthesized catalyst samples were recorded by UV-vis DRS analysis. Fig. 2a presents the typical UV-vis DRS spectrum of g-C3N4, CaFe2O4 and CaFe2O4/g-C3N4/CNT composite. It can be observed that the absorption maximum of CaFe2O4 is about 466 nm, while the pure g-C3N4 exhibits poor visible light absorption about 470 nm. From the Tauc plot, the band gap energies of CaFe2O4 and g-C3N4 were calculated to be 1.55 eV and 2.57 eV, respectively. Comparing the absorption maxima of g-C3N4, g-C3N4/CNT and g-C3N4/CaFe2O4 nanocomposites, the CaFe2O4/g-C3N4/CNT composite exhibited a significant red shift with the characteristic absorption edge at 585 nm and band gap energy of 2.02 eV, as shown in Fig. 2b. This may be attributed to the successful formation of an active heterojunction between the g-C3N4 and CaFe2O4 interface and strong electron conducting nature of CNT.18 Moreover, the enhanced visible light absorption confirmed that the CaFe2O4/g-C3N4/CNT composite can absorb the visible light effectively, which is favorable for the production of hydrogen and degradation of pollutants.19
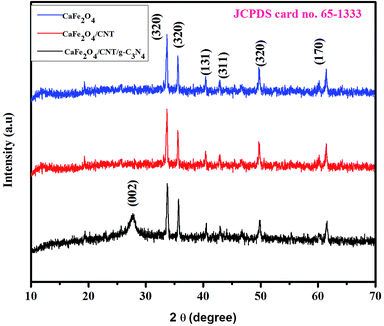 |
| Fig. 1 XRD patterns of the as-prepared CaFe2O4 and CaFe2O4/g-C3N4/CNT composite. | |
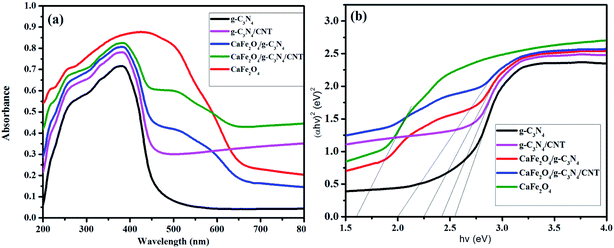 |
| Fig. 2 (a) UV-vis diffuse reflectance spectra of CaFe2O4, g-C3N4, g-C3N4/CNTs, and CaFe2O4/g-C3N4/CNT composite. (b) Optical band gap energy, Eg, of CaFe2O4, g-C3N4, g-C3N4/CNTs, and CaFe2O4/g-C3N4/CNT composite. | |
The morphologies of the as-synthesized composites were analyzed by FE-SEM and TEM analysis. The SEM images in Fig. 3a–d show the morphology and structure of CaFe2O4 and the CaFe2O4/g-C3N4/CNT composite. Fig. 3a shows that the CaFe2O4 particles are well-dispersed with an average size of 200–300 nm.20 Fig. 3b–d show the FE-SEM images of the CaFe2O4/g-C3N4/CNT composite, which contains CaFe2O4, CNT and g-C3N4 nanosheets, respectively. Furthermore, the FE-SEM images reveal that g-C3N4 exhibited a porous structure with few aggregations. The tube-like CNT were interlinked effectively on the surface of the g-C3N4 and CaFe2O4 matrices.21 The existence of this heterojunction was further confirmed by the representative elemental mapping images (EDS) in Fig. 4, which show an even distribution of carbon (C), nitrogen (N), calcium (a), oxygen (O) and iron (Fe).
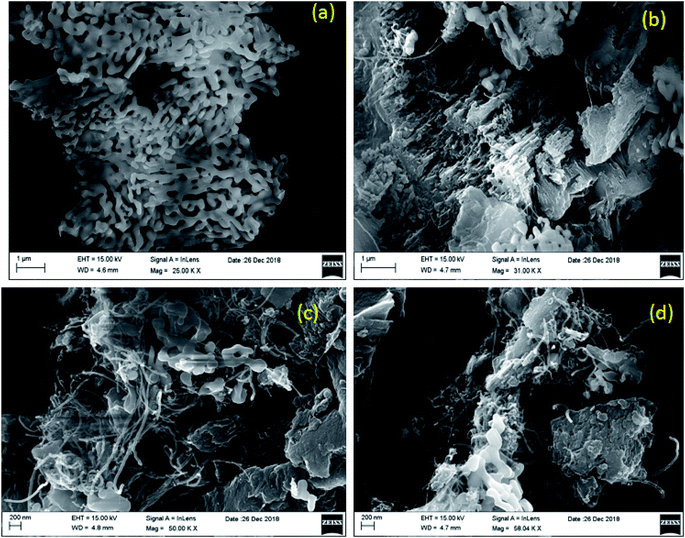 |
| Fig. 3 (a–d) FE-SEM images of CaFe2O4 and CaFe2O4/g-C3N4/CNT composite. | |
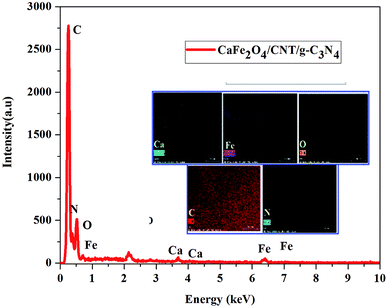 |
| Fig. 4 EDS and elemental mapping analysis of the CaFe2O4/g-C3N4/CNT composite. | |
Further, to investigate the microstructure of the obtained samples, TEM characterization was performed. Fig. 5a–d present the typical TEM images of CaFe2O4, g-C3N4, and the CaFe2O4/g-C3N4/CNT composite. For the composite, grey part can be assigned to the sheet-like g-C3N4, and the dark particles can be assigned to CaFe2O4 nanoparticles, which were dispersed effectively on the exterior surface of g-C3N4.22 Furthermore, the TEM images display the existence of a heterojunction stuck between g-C3N4 and CaFe2O4, and the tubular CNT acting as a connecting bridge between these materials. In the SAED patterns, the lattice fringes have a spacing of 0.231 nm, which is consistent with the (131) planes of CaFe2O4 (JCPDS 32-0168). Thus, these results are in accordance with the XRD analysis. Upon further analysis of the HRTEM images, as shown in Fig. 6, close contact interfaces between CaFe2O4, g-C3N4 and CNT were observed. From the TEM results, it can be concluded that an apparent interface was formed between the g-C3N4, CNT, and CaFe2O4 matrices. Therefore this ternary CaFe2O4/g-C3N4/CNT composite is promising for the transport of charge carriers towards the degradation of Cr(VI) and TC.23
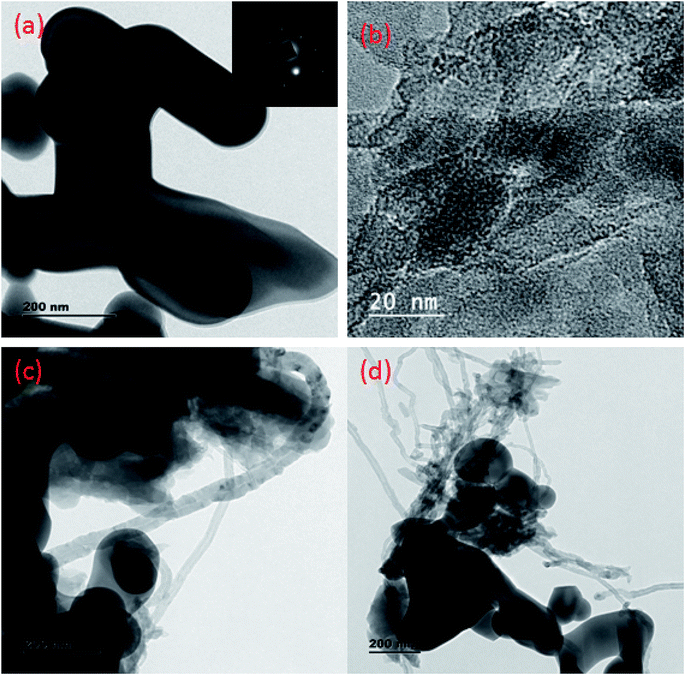 |
| Fig. 5 (a) TEM images of CaFe2O4 (b) g-C3N4 and (c and d) CaFe2O4/g-C3N4/CNT composite. | |
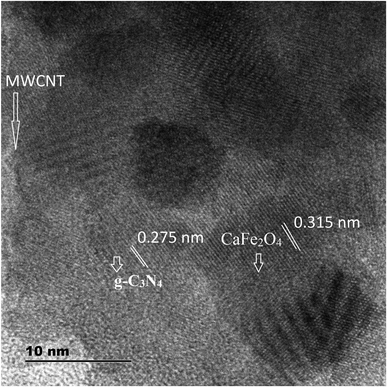 |
| Fig. 6 HRTEM images of the CaFe2O4/g-C3N4/CNT composite. | |
The chemical binding energies and oxidation states of the CaFe2O4/g-C3N4/CNT composite were confirmed by XPS (Fig. 7), which was also used to study the valence state properties of the semiconductors. Fig. 7a displays the XPS survey spectrum of the CaFe2O4/g-C3N4/CNT composite. The major peaks of the Ca, Fe, O, C and N elements appeared in the XPS spectra. Fig. 7b displays the elemental scan of the Ca 2p element, where the major peaks at 346.4 eV and 350.2 eV correspond to the Ca 2p3/2 and Ca 2p1/2 spins, respectively.24 The elemental XPS spectrum of Fe 2p in Fig. 7c mainly shows peaks at 710.8 eV and 724.8 eV, representing the Fe 2p3/2 and Fe 2p1/2 spins, which indicate the oxidation of Fe was 3+ in CaFe2O4.25 The O 1s spectrum in Fig. 7d was deconvoluted into two main peaks at 529.7 eV and 531.8 eV, corresponding to the lattice oxygen in ferrites and surface-adsorbed OH groups, respectively.26 The C 1s peaks of the composite were located at 288.6 eV and 284.5 eV, which correspond to the C–N and C–C hybridization in the carbon matrix, respectively (Fig. 7e). The peaks observed at 398.7 eV and 398.5 eV are attributed to the sp2-hybridized nitrogen atoms in the triazine rings. Furthermore, the sharp peak observed at 401.4 eV corresponds to the strong binding of nitrogen with carbon atoms such as N–(C)3 (Fig. 7f).27 Thus, the structural analysis with XRD, FE-SEM, TEM, UV-vis DRS, and XPS suggests that the CaFe2O4/g-C3N4/CNT composite was successfully synthesized.
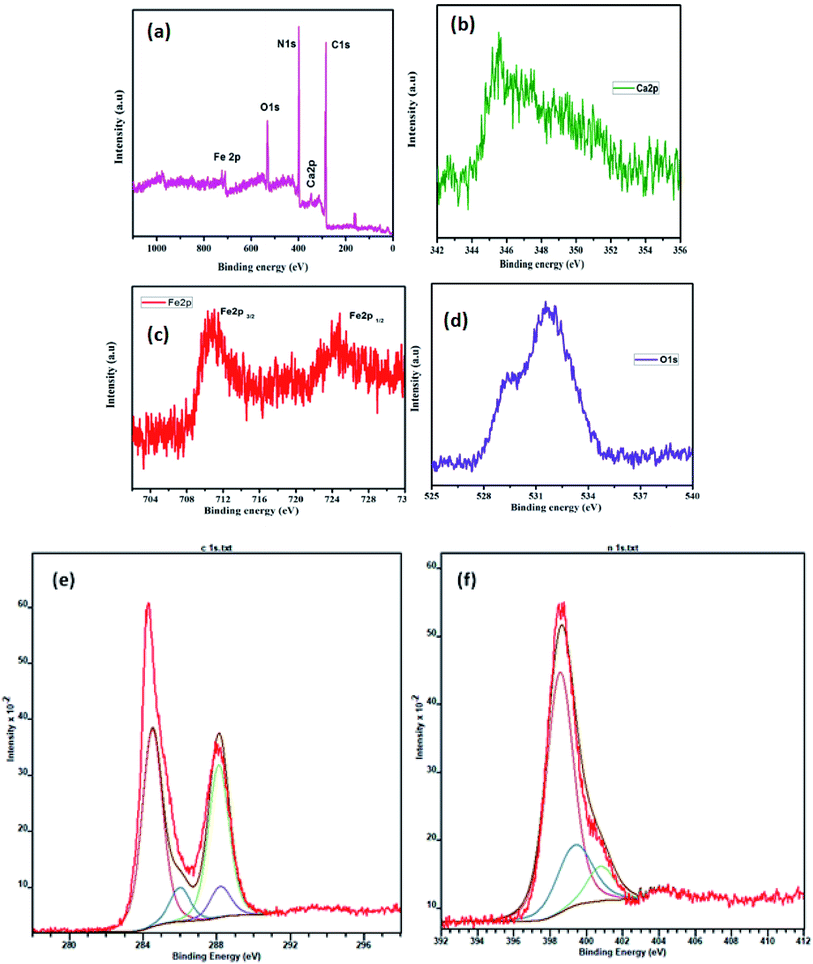 |
| Fig. 7 XPS spectra of the as-prepared CaFe2O4/g-C3N4/CNT composite: (a) survey scan, (b) Ca 2p, (c) Fe 2p, (d) O 1s, (e) C 1s, and (f) N 1s. | |
It is worth mentioning that highly proficient visible light-active photocatalytic reactions are mainly a result of fast separation efficiency, good redox reactions and high visible light absorption.28 The electron–hole separation and charge transfer properties of g-C3N4 and the CaFe2O4/g-C3N4/CNT composite was confirmed by PL analysis, and the data is shown in Fig. 8. g-C3N4 showed the highest PL peak intensity, which confirms that it has a very high recombination rate. However, the PL intensity of the CaFe2O4/g-C3N4/CNT composite was remarkably compared with that of g-C3N4. The lower recombination rate in the photocatalytic system can be beneficial for the complete degradation of pollutions within a minimum time.29
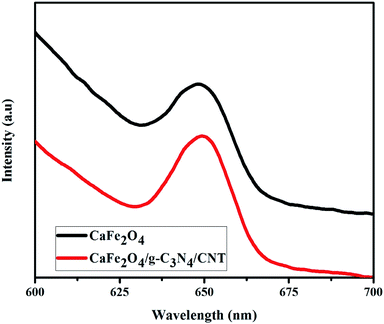 |
| Fig. 8 PL analysis of CaFe2O4 and CaFe2O4/g-C3N4/CNTs composite. | |
The BET surface area and average pore size of CaFe2O4, g-C3N4, CaFe2O4/CNTs, and the CaFe2O4/g-C3N4/CNT nanocomposite were obtained from N2 adsorption–desorption studies, as shown in Fig. 9. The N2 adsorption–desorption curves of the prepared samples exhibit type IV isotherms, indicating the pore size of the samples is mesoporous in nature using the BJH method. It was evident that the CaFe2O4/g-C3N4/CNTs photocatalyst exhibited the highest specific surface area, which is beneficial for higher photocatalytic activity.
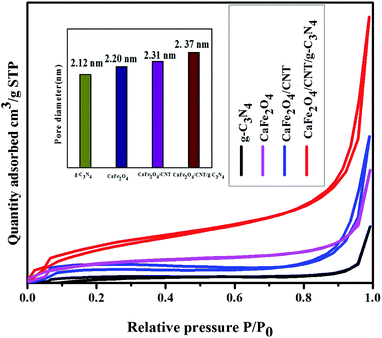 |
| Fig. 9 BET analysis of CaFe2O4, g-C3N4, CaFe2O4//CNTs, and CaFe2O4/g-C3N4/CNT composite. | |
The photogenerated charge carrier capability and separation nature of the composite were investigated by EIS measurements. Both CaFe2O4 and the CaFe2O4/g-C3N4/CNT composite exhibited semicircular Nyquist plots, but the diameter of the CaFe2O4/g-C3N4/CNT composite plot was smaller (Fig. 10), further confirming the faster interfacial charge transfer rate and outstanding separation rate of photogenerated charge carriers between g-C3N4 and CaFe2O4 due to the incorporation of CNT.
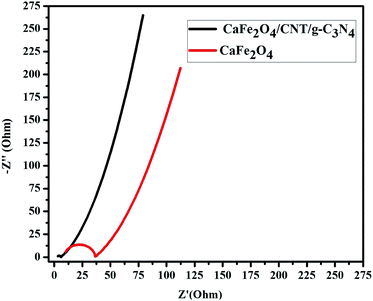 |
| Fig. 10 EIS spectra CaFe2O4 and CaFe2O4/g-C3N4/CNT composite. | |
The spin-trapping ESR measurements were used to confirm the presence of active oxidation species produced over the CaFe2O4/g-C3N4/CNT composite during the photocatalytic process, and the results are shown in Fig. 11. Under visible light irradiation, the characteristic peaks of DMPO–·O2− and DMPO–·OH were clearly observed, and the peak intensities were obvious when the irradiation time was increased up to 3 min. The ESR results confirmed that the ·O2− and ·OH radicals were mainly generated during the photocatalytic process.
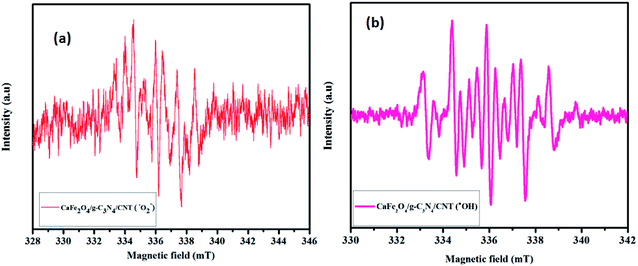 |
| Fig. 11 EPR spectrum of CaFe2O4/g-C3N4/CNT composite using DMPO. | |
3.1. Photocatalytic activities and stability tests
The photocatalytic activity of g-C3N4, CaFe2O4, and CaFe2O4/g-C3N4/CNT composite for the visible light degradation of Cr(VI) and TC is shown in Fig. 12a. The photolysis of Cr(VI) and TC under visible light without photocatalysts was negligible. Using the pure g-C3N4 and CaFe2O4, the degradation rate was not appreciable due to their high recombination rate of charge carriers. Furthermore, using the binary systems of g-C3N4/CNT and CaFe2O4/CNT, the degradation rate of Cr(VI) and TC moderately increased. We found that the introduction of CNT in the g-C3N4/CaFe2O4 heterojunction enhanced the degradation rate for Cr and TC, and the best degradation efficiency was achieved upon the incorporation of CNT in the system, which confirms the importance of CNT in the ternary structure. The CaFe2O4/g-C3N4/CNT composite exhibited a photocatalytic efficiency of 97% (at 120 min) for the degradation of Cr(VI) and 98% (at 60 min) for TC under visible light (Fig. 13a). As show in Fig. 13b, the photodegradation rate of the CaFe2O4/g-C3N4/CNT composite decreased slightly after five reaction cycles, indicating the excellent reusability of the photocatalytic material. The role of pH strongly interferes with the photocatalytic removal of Cr(VI); thus, the effect of pH was also studied in the pH range of 3–9 with the CaFe2O4/g-C3N4/CNT composite. At a higher pH, the photocatalytic reduction rate for Cr(VI) was significantly reduced (Fig. 12b) due to the formation of Cr2O72−. At a lower pH value, Cr(VI) was easily removed due to the formation of HCrO4− and the protonated surface of the catalyst, which is more favorable the Cr(VI) removal.30 Moreover, the reaction rate constant of the CaFe2O4/g-C3N4/CNTs ternary photocatalyst, as shown in Fig. 14, for the removal of Cr(VI) is 65 × 10−4 min−1 and removal of TC is 73 × 10−4 min−1.
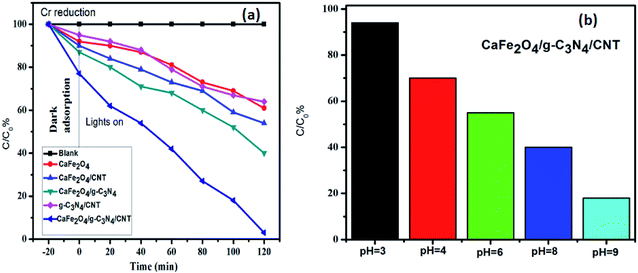 |
| Fig. 12 (a) Adsorption and visible light photocatalytic performance of the samples for the degradation of Cr(VI) and (b) effect of pH on the removal of Cr(VI) using the CaFe2O4/g-C3N4/CNTs composite. | |
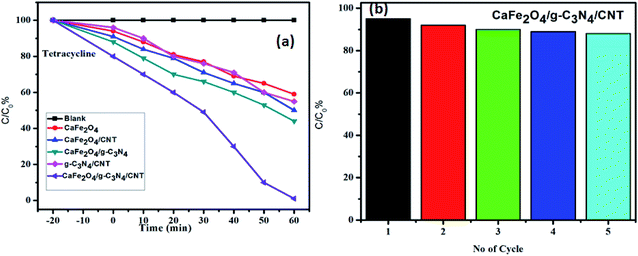 |
| Fig. 13 (a) Adsorption and visible light photocatalytic performance of the samples in the degradation of TC and (b) stability test of the CaFe2O4/g-C3N4/CNT composite in recycling degradation of TC. | |
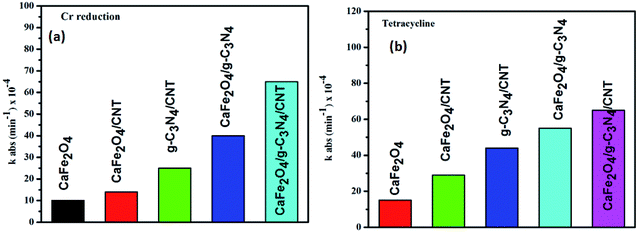 |
| Fig. 14 Photodegradation kinetics of Cr and TC over the CaFe2O4/g-C3N4/CNT composite under visible light. | |
3.2. Photocatalytic hydrogen production tests
In this work, the feasibility of using the CaFe2O4/g-C3N4/CNT composite as a versatile photocatalyst for the production of hydrogen was studied. As shown in Fig. 15, the loading of CNT on g-C3N4 and CaFe2O4 resulted in a significant enhancement in the photocatalytic hydrogen production rate. Initially, with an increase in the loading of g-C3N4 in CaFe2O4, the hydrogen generation rate showed a remarkable improvement. In particular, the hydrogen generation rate of the CaFe2O4/g-C3N4/CNT composite sample increased to 1085 μmol h−1, which is about nearly 3 times higher than that of bare CaFe2O4 (417 μmol h−1). The AQY of CaFe2O4/g-C3N4/CNTs was calculated to be 7.2%. These results indicate that the CNT act as an effective co-catalyst for g-C3N4/CaFe2O4 and heterojunctions, and the loading ratio of CNT needs to be controlled within an appropriate range. Therefore, as a noble metal-free catalyst, CaFe2O4/g-C3N4/CNT composite can potentially be used as an economically feasible catalyst to replace precious metals such as Pt and Pd industrially to produce hydrogen. As shown in Fig. 16, to study the stability of the CaFe2O4/g-C3N4/CNT composite, cyclic runs for photocatalytic hydrogen production using the CaFe2O4/g-C3N4/CNT composite sample were performed. A slight decrease in the hydrogen production rate of the CaFe2O4/g-C3N4/CNT composite was observed in the last cycle, which confirms that the CaFe2O4/g-C3N4/CNT composite has excellent stability during the photocatalytic production of hydrogen besides the degradation reactions. Furthermore, the sample was collected after the cycle test and then characterized by XRD analysis. The XRD patterns of the reused catalyst exhibited no significant changes in the crystal structure after five consecutive cycles. Thus, the CaFe2O4/g-C3N4/CNT composite was determined to display good cyclic stability during the photocatalytic production of hydrogen.31 Using all the characterization results, the plausible photocatalytic degradation mechanism with the CaFe2O4/g-C3N4/CNT composite for a high degradation rate and hydrogen production was proposed. Also, a comparison of the present work with previous reported catalysts is presented in Table 1.
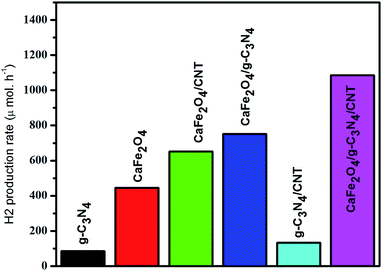 |
| Fig. 15 Time-dependent photocatalytic hydrogen production rate of the CaFe2O4/g-C3N4/CNT composite under visible light. | |
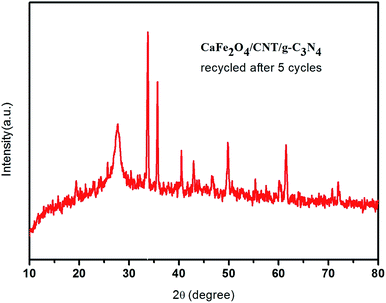 |
| Fig. 16 XRD spectrum of the reused CaFe2O4/g-C3N4/CNT composite towards hydrogen production after three cycles. | |
Table 1 Catalytic, photocatalytic and post-illumination activities of different catalysts
Photocatalyst |
Target pollutant |
Irradiation time (min) |
Decomposition (%) |
Ref. |
Biochar-coupled g-C3N4 |
Cr(VI) |
300 |
100 |
41 |
TiO2/g-C3N4@diatomite hybrid photocatalyst |
Cr(VI) |
300 |
100 |
42 |
N2-g-C3N4 photocatalyst |
Bisphenol-A |
120 |
79 |
43 |
g-C3N4/Na-bentonite composites |
Cr(VI) and RhB |
120 |
88.6 |
44 |
0D/2D bismuth molybdate homojunction |
Cr(VI) |
80 |
100 |
45 |
AgI/BiVO4 p–n junction photocatalyst |
Tetracycline and Cr(VI) |
100 |
70 |
46 |
CaFe2O4/g-C3N4/CNT composite![[thin space (1/6-em)]](https://www.rsc.org/images/entities/char_2009.gif) |
Tetracycline and Cr(VI) |
120 |
98 |
This study |
3.3. Photocatalytic mechanisms
Under visible light illumination, both CaFe2O4 and g-C3N4 can be quickly excited to generate the corresponding photoinduced electrons and holes due to their moderate band gaps of 1.55 eV and 1.55 eV, respectively. Since the ECB value of g-C3N4 (−1.15 eV)32 is highly negative compared to that of CaFe2O4 (+1.57 eV), the ejected electrons towards the CB of g-C3N4 can be simply transferred to the CB of CaFe2O4. Meanwhile, the generated holes on the VB of CaFe2O4 may be transferred to the VB of g-C3N4 because the EVB of CaFe2O4 (+1.98 eV) was more positive than that of g-C3N4 (+0.89 eV).33 Furthermore, the additional impact of the CNT results in the transfer of more electron–hole pairs through the effective linkage, leading to an improvement in the lifetime of the carriers. In this mode, the charge separation of photogenerated electron–hole pairs can be drastically enhanced and the recombination rate can be minimized, thus improving the photocatalytic ability of the CaFe2O4/g-C3N4/CNT composite.34–40 The possible photocatalytic mechanism for the photodegradation of Cr(VI) and TC as well as hydrogen generation using the ternary CaFe2O4/g-C3N4/CNT composite under visible light irradiation is proposed in Fig. S1.†
4. Conclusion
In conclusion, we demonstrated a simple hydrothermal strategy for the fabrication of a ternary CaFe2O4/g-C3N4/CNT composite. The addition of CNT in the system outstandingly promoted the separation of photogenerated charge carriers. The as-synthesized CaFe2O4/g-C3N4/CNT composite exhibited improved light-harvesting capability, more proficient charge transfer capability and improved hydrogen production performance. The hydrogen production rate of the optimal CaFe2O4/g-C3N4/CNT composite was superior to that of pure CaFe2O4 and previous works. Furthermore, the optimal CaFe2O4/g-C3N4/CNT composite photocatalyst exhibited excellent performances for the photodegradation of Cr(VI) and TC with high stability. This work may inspire the development of rare earth- and noble metal-free catalysts for the degradation of pollutants and production of hydrogen in the near future.
Conflicts of interest
There are no conflicts of interest in this paper.
Acknowledgements
This work was sponsored by Scientific Research Starting Fund from Shengli College China University of Petroleum (KQ2019-007), Shandong Postdoctoral Innovation Talent Support Program, Project of Shandong Province Higher Educational Science and Technology Program, Training Program of Innovation for Undergraduates (2019001).
References
- F. Chang, Y. Xie, C. Li, J. Chen, J. Luo, X. Hu and J. Shen, A facile modification of g-C3N4 with enhanced photocatalytic activity for degradation of methylene blue, Appl. Surf. Sci., 2013, 280, 967–974 CrossRef CAS.
- Y. Xia, Q. Li, X. Wu, K. Lv, D. Tang and M. Li, Facile synthesis of CNTs/CaIn2S4 composites with enhanced visible-light photocatalytic performance, Appl. Surf. Sci., 2017, 391, 565–571 CrossRef CAS.
- Y. Choi, M. S. Koo, A. D. Bokare, D. H. Kim, D. W. Bahnemann and W. Choi, Sequential process combination of photocatalytic oxidation and dark reduction for the removal of organic pollutants and Cr(VI) using Ag/TiO2, Environ. Sci. Technol., 2017, 51, 3973–3981 CrossRef CAS PubMed.
- A. Y. Meng, B. C. Zhu, B. Zhong, L. Y. Zhang and B. Cheng, Direct Z-scheme TiO2/CdS hierarchical photocatalyst for enhanced photocatalytic H2-production activity, Appl. Surf. Sci., 2017, 422, 518–527 CrossRef CAS.
- X. Zhang, S. Cao, Z. Wu, S. Zhao and L. Piao, Enhanced photocatalytic activity towards degradation and H2 evolution over one dimensional TiO2@MWCNTs heterojunction, Appl. Surf. Sci., 2017, 402, 360–368 CrossRef CAS.
- G. Liu, G. Zhao, W. Zhou, Y. Liu, H. Pang, H. Zhang, D. Hao, X. Meng, P. Li, T. Kako and J. Ye, In situ bond modulation of graphitic carbon nitride to construct p-n homojunctions for enhanced photocatalytic hydrogen production, Adv. Funct. Mater., 2016, 26, 6822–6829 CrossRef CAS.
- S. Chidambaram, A. Vijay, G. Mohan Kumar, M. Alagiri, J. Thiruvadigal and M. Rathinam, Three-dimensional (3D) flower-like nanoarchitectures of ZnO-Au on MWCNTs for visible light photocatalytic applications, Appl. Surf. Sci., 2018, 449, 631–637 CrossRef CAS.
- A. Payan, M. Fattahi, S. Jorfib, B. Roozbehani and S. Payan, Synthesis and characterization of titanate nanotube/single-walled carbon nanotube (TNT/SWCNT) porous nanocomposite and its photocatalytic activity on 4-chlorophenol degradation under UV and solar irradiation, Appl. Surf. Sci., 2018, 434, 336–350 CrossRef CAS.
- J. Wang, X. Xu, F. Cao, Y. Wang, S. Li and G. Qin, In situ fabrication of α-Fe2O3/CaFe2O4 p-n heterojunction with enhanced VOCs photodegradation activity, Adv. Powder Technol., 2019, 30, 590–595 CrossRef CAS.
- M. Li, H. Y. Bai, Z. L. Da, X. Yan, C. Chen, J. H. Jiang, W. Q. Fan and W. D. Shi, Electrospinning synthesis and photocatalytic property of CaFe2O4/MgFe2O4 heterostructure for degradation of tetracycline, Cryst. Res. Technol., 2015, 50, 244–249 CrossRef CAS.
- Y. K. Luo, Synthesis and characterization of novel CaFe2O4/Bi2O3 composite photocatalysts, Mater. Lett., 2018, 225, 17–20 CrossRef.
- X. Liu, Y. H. Zhang, Y. S. Jia, J. Z. Jiang, Y. B. Wang, X. S. Chen and T. Gui, Visible light-responsive carbon-decorated p-type semiconductor CaFe2O4 nanorod photocatalyst for efficient remediation of organic pollutants, Chin. J. Catal., 2017, 38, 1770 CrossRef CAS.
- R. C. Pawar, S. Kang, S. H. Ahn and C. S. Lee, Gold nanoparticle modified graphitic carbon nitride/multi-walled carbon nanotube (g-C3N4/CNT/Au) hybrid photocatalysts for effective water splitting and degradation, RSC Adv., 2015, 5, 24281–24292 RSC.
- D. Chaudhary, V. D. Vankar and N. Khare, Noble metal free g-C3N4/TiO2/CNT ternary nanocomposite with enhanced photocatalytic performance under visible light irradiation with multi-step charge transfer process, Sol. Energy, 2017, 158, 132–139 CrossRef CAS.
- Y. Bao and K. Chen, Novel Z-scheme BiOBr/reduced graphene oxide/protonated g-C3N4 photocatalyst: synthesis, characterization,
visible light photocatalytic activity and mechanism, Appl. Surf. Sci., 2018, 437, 51–61 CrossRef CAS.
- G. Mamba and A. K. Mishra, Graphitic carbon nitride (g-C3N4) nanocomposites: a new and exciting generation of visible light driven photocatalysts for environmental pollution remediation, Appl. Catal., B, 2016, 198, 347–377 CrossRef CAS.
- S. Vadivel, D. Maruthamani, A. Habibi-Yangjeh, B. Paul, S. S. Dhar and K. Selvam, Facile synthesis of novel CaFe2O4/g-C3N4 nanocomposites for degradation of methylene blue under visible-light irradiation, J. Colloid Interface Sci., 2016, 480, 126–136 CrossRef CAS PubMed.
- Y. K. Kim and H. Park, Light-harvesting multi-walled carbon nanotubes and CdS hybrids: application to photocatalytic hydrogen production from water, Energy Environ. Sci., 2011, 4, 685–694 RSC.
- Y. X. Zhang, D. Ma, J. Wu, Q. Z. Zhang, Y. J. Xin and N. Bao, One-step preparation of CNTs/InVO4 hollow nanofibers by electrospinning and its photocatalytic performance under visible light, Appl. Surf. Sci., 2015, 353, 1260–1268 CrossRef CAS.
- A. Doi, M. Nomura, Y. Obukuro, R. Maeda and K. Obata, Characterization of Ti doped CaFe2O4 prepared from a malic acid complex, J. Ceram. Soc. Jpn., 2014, 122, 175–178 CrossRef.
- H. Q. Wang, J. Z. Li, M. J. Zhou, Q. F. Guan, Z. Y. Lu, P. W. Huo and Y. S. Yan, Preparation and characterization of Ag2O/SWNTs photocatalysts and its photodegradation on tetracycline, J. Ind. Eng. Chem., 2015, 30, 64–70 CrossRef CAS.
- X. J. She, J. Wu, H. Xu, J. Zhong, Y. Wang, Y. H. Song, K. Nie, Y. Liu, Y. Yang, M.-T. F. Rodrigues, R. Vajtai, J. Lou, D. Du, H. Li and P. M. Ajayan, High efficiency photocatalytic water splitting using 2D α-Fe2O3/g-C3N4 Z-scheme catalysts, Adv. Energy Mater., 2017, 7, 1700025 CrossRef.
- X. W. Zhu, J. Y. Liu, Z. Z. Zhao, J. Yan, Y. G. Xu, Y. H. Song, H. Y. Ji, H. Xu and H. M. Li, Hydrothermal synthesis of mpg-C3N4 and Bi2WO6 nest-like structure nanohybrids with enhanced visible light photocatalytic activities, RSC Adv., 2017, 7, 38682–38690 RSC.
- N. K. Veldurthi, N. K. Eswar, S. A. Singh and G. Madras, Cooperative effect between BaTiO3 and CaFe2O4 in a cocatalyst-free heterojunction composite for improved photochemical H2 generation, Int. J. Hydrogen Energy, 2018, 43, 22929–22941 CrossRef CAS.
- T. Yamashita and P. Hayes, Analysis of XPS spectra of Fe2p and Fe3p ions in oxide materials, Appl. Surf. Sci., 2008, 254, 2441–2449 CrossRef CAS.
- X. Yang, D. Li, Z. H. Ren, R. G. Zeng, S. Y. Gong and D. K. Zhou, Colossal dielectric performance of pure barium titanate ceramics consolidated by spark plasma sintering, RSC Adv., 2016, 6, 75422–75429 RSC.
- J. H. Liu, T. K. Zhang, Z. C. Wang, G. Dawson and W. Chen, Simple pyrolysis of urea into graphitic carbon nitride with recyclable adsorption and photocatalytic activity, J. Mater. Chem., 2011, 21, 14398–14401 RSC.
- W. J. Shan, Y. Hu, Z. G. Bai, M. M. Zheng and C. H. Wei, In situ preparation of g-C3N4/bismuth-based oxide nanocomposites with enhanced photocatalytic activity, Appl. Catal., B, 2016, 188, 1–12 CrossRef CAS.
- L. Liu, Y. H. Qi, J. R. Lu, S. L. Lin, W. J. An, Y. H. Liang and W. Q. Cui, A stable Ag3PO4@gC3N4 hybrid core@shell composite with enhanced visible light photocatalytic degradation, Appl. Catal., B, 2016, 183, 133–141 CrossRef CAS.
- D. Xiao, K. Dai, Y. Qu, Y. Yin and H. Chen, Hydrothermal synthesis of α-Fe2O3/g-C3N4 composite and its efficient photocatalytic reduction of Cr(VI) under visible light, Appl. Surf. Sci., 2015, 358, 181–187 CrossRef CAS.
- L. Ge, C. Han and J. Liu, Novel visible light-induced g-C3N4/Bi2WO6 composite photocatalysts for efficient degradation of methyl orange, Appl. Catal., B, 2011, 108–109, 100–107 CrossRef CAS.
- C. Han, Novel visible light induced Co3O4/g-C3N4 heterojunction photocatalysts for efficient degradation of methyl orange, Appl. Catal., B, 2014, 147, 546–553 CrossRef CAS.
- C. Shifu, Z. Wei, L. Wei, Z. Huaye and Y. Xiaoling, Preparation, characterization and activity evaluation of p–n junction photocatalyst p-CaFe2O4/n-ZnO, Chem. Eng. J., 2009, 155, 466–473 CrossRef.
- D. K. Padhi and K. Parida, Facile fabrication of, α-FeOOH, nanorod/RGO, composite: a robust photocatalyst for reduction of Cr(VI), J. Mater. Chem. A, 2014, 2, 10300–10312 RSC.
- X. Li, J. Yu, M. Jaroniec and X. Chen, Co catalysts for Selective Photoreduction of CO2 into Solar Fuels, Chem. Rev., 2019, 119(6), 3962–4179 CrossRef CAS PubMed.
- X. Li, J. Xie, C. Jiang, J. Yu and P. Zhang, Review on design and evaluation of environmental photocatalysts, Front. Environ. Sci. Eng., 2018, 12, 14 CrossRef.
- J. Wen, J. Xie, X. Chen and X. Li, A review on g-C3N4-based photocatalysts, Appl. Surf. Sci., 2017, 391, 72–123 CrossRef CAS.
- R. Shen, J. Xie, Y. Ding, S. Liu, A. Adamski, X. Chen and X. Li, Carbon Nanotube-Supported Cu3P as High-Efficiency and Low-Cost Cocatalysts for Exceptional Semiconductor-Free Photocatalytic H2 Evolution, ACS Sustainable Chem. Eng., 2019, 7(3), 3243–3250 CrossRef CAS.
- R. Shen, J. Xie, Q. Xiang, X. Chen, J. Jiang and X. Li, Ni-based photocatalytic H2-production cocatalysts, Chinese, J. Catal., 2019, 40(3), 240–288 CrossRef CAS.
- Z. Li, Y. Ma, X. Hu and E. L. J. Fan, Enhanced photocatalytic H2 production over dual-cocatalyst-modified g-C3N4 heterojunctions, Chinese, J. Catal., 2019, 40, 434–445 CrossRef CAS.
- K. Li, Z. Huang, S. Zhu, S. Luo, L. Yan, Y. Dai, Y. Guo and Y. Yang, Removal of Cr(VI) from water by a biochar-coupled g-C3N4 nanosheets composite and performance of a recycled photocatalyst in single and combined pollution systems, Appl. Catal., B, 2019, 243, 386–396 CrossRef CAS.
- Q. Sun, X. Hu, S. Zheng, J. Zhang and J. Sheng, Effect of calcination on structure and photocatalytic property of N-TiO2/g-C3N4@diatomite hybrid photocatalyst for improving reduction of Cr(VI), Environ. Pollut., 2019, 245, 53–62 CrossRef CAS PubMed.
- H. Wang, Q. Li, S. Zhang, Z. Chen, W. Wang, G. Zhao, L. Zhuang, B. Hu and X. Wang, Visible-light-driven N2-g-C3N4 as a highly stable and efficient photocatalyst for bisphenol A and Cr(VI) removal in binary systems, Catal. Today, 2019, 335, 110–116 CrossRef CAS.
- Y. Guo, C. Li, Y. Guo, X. Wang and X. Li, Ultrasonic-assisted synthesis of mesoporous g-C3N4/Na-bentonite composites and its application for efficient photocatalytic simultaneous removal of Cr(VI) and RhB, Colloids Surf., A, 2019, 578, 12362 CrossRef.
- J. Xua, J. Yue, J. Niu and M. Chen, Synergistic removal of Cr(VI) and dye contaminants by 0D/2D bismuth molybdate homojunction photocatalyst under visible light, Appl. Surf. Sci., 2019, 484, 1080–1088 CrossRef.
- W. Zhao, J. Li, B. Dai, Z. Cheng, J. Xu, K. Ma, L. Zhanga, N. Shenge, G. Mao, H. Wud, K. Wei and D. Y. C. Leung, Simultaneous removal of tetracycline and Cr(VI) by a novel three dimensional AgI/BiVO4 p-n junction photocatalyst and insight into the photocatalytic mechanism, Chem. Eng. J., 2019, 369, 716–725 CrossRef CAS.
Footnote |
† Electronic supplementary information (ESI) available. See DOI: 10.1039/c9ra05005a |
|
This journal is © The Royal Society of Chemistry 2019 |
Click here to see how this site uses Cookies. View our privacy policy here.