DOI:
10.1039/C9RA04864B
(Paper)
RSC Adv., 2019,
9, 27883-27887
Tandem grinding reactions involving aldol condensation and Michael addition in sequence for synthesis of 3,4,5-trisubstituted isoxazoles†
Received
27th June 2019
, Accepted 23rd August 2019
First published on 4th September 2019
Abstract
A one-pot, base-catalyzed, tandem grinding process involving carrying out aldol condensation and Michael addition in sequence to produce 3,4,5-trisubstituted isoxazoles from 3,5-dimethyl-4-nitroisoxazole, aromatic aldehydes and activated methylene compounds has been developed. In the presence of 10 mol% of pyrrolidine, aldol condensations of 3,5-dimethyl-4-nitroisoxazole with various aromatic aldehydes were performed with 3–10 minutes of grinding to provide 5-styryl-3-methyl-4-nitroisoxazoles in good to quantitative yields without further purification. Then, Michael additions between 5-styryl-3-methyl-4-nitroisoxazoles and activated methylene compounds (including ethyl 2-nitroacetate and alkyl 2-cyanoacetates) were carried out in the presence of 10 mol% of Et3N in the same mortar with 3–5 minutes of continuous grinding to produce 3,4,5-trisubstituted isoxazoles in good to excellent yields.
Introduction
Maximizing the efficiency of reactants and reducing waste generation are important contributions to atomic economy and green chemistry. Many chemists have paid increasing attention to Michael addition for C–C and C–heteroatom bond formation in recent years.1 Generally, the conventional Michael addition reactions are performed in solvent conditions, and some cases take a long reaction time (up to 7 days). In response to the requirements of green chemistry, scientists have been working on developing synthetic methods that generate little environmental pollution and display high atom economy.2 Therefore, the study of solvent-free Michael reactions involving microwave irradiation, ultrasonic irradiation and mechanochemical synthesis has also been reported extensively.3 On the one hand, reactions performed under solvent-free grinding conditions are very attractive to synthetic chemists because these reactions are easy to manipulate, cost little, and are highly efficient. The molecules in the solid state display large contact areas and high local concentration, which speeds up the reaction and increases selectivity. On the other hand, one-pot reactions4 are also highly recommended by synthetic chemists because of their high modularity and simple manipulation. In this regard, the tandem grinding reaction is regarded as a quasi-one-pot reaction because all of its reaction steps are completed in the same mortar. Ideal solutions for the synthesis of active pharmaceutical ingredients and biological products, and new strategies to improve the atomic economy of important chemical processes and valuable structures are still in demand.
Nitrogen-containing heterocyclic compounds, especially isoxazole and its derivatives, are very important heterocyclic cores with a wide range of organic and bio-activities, and are present in many natural products and medicines (Fig. 1).5–7 3-Methyl-4-nitro-5-styrylisoxazoles can be easily prepared from commercially available 3,5-dimethyl-4-nitroisoxazole and aromatic aldehydes, and they are usually used as electro-deficient Michael acceptors for asymmetric catalytic Michael reactions with various activated methylene compounds such as isocyanoacetates, α,β-unsaturated γ-butyrolactam, and anthrones.8 These Michael additions have all been performed under solvent conditions with relative long reaction times. Very recently, we reported a facile catalyst-free amination of β-nitrostyrenes and 2-aryl-3-nitro chromenes achieved by carrying out grinding.9 As part of our continuous efforts with grinding reactions, herein we demonstrated a facile synthetic route to 3,4,5-trisubstituted isoxazoles, in excellent yields, from 3,5-dimethyl-4-nitroisoxazole, aromatic aldehydes and activated methylene compounds, achieved by performing amine-catalyzed tandem grinding (Fig. 2).
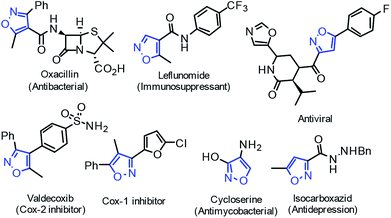 |
| Fig. 1 Representative compounds containing the biologically active isoxazole core. | |
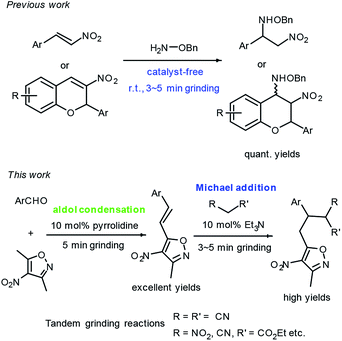 |
| Fig. 2 The syntheses of 3,4,5-trisubstituted isoxazoles achieved by performing tandem grinding. | |
Results and discussion
Pyrrolidine-catalyzed grinding reactions of aromatic aldehydes with 3,5-dimethyl-4-nitroisoxazole
Aldol condensation is one of the important C–C bond formation reactions in modern organic synthesis. Many enantioselective aldol reactions have been investigated extensively in the past two decades. Solvent-free aldol condensations have also been reported occasionally. In fact, the aldol condensation of aromatic aldehydes with 3,5-dimethyl-4-nitroisoxazole is usually reported to be carried out in a polar solvent system (e.g. EtOH) by using stoichiometric or catalytic amounts of organic secondary amines (such as diisopropyl amine, pyrrolidine, piperidine) under heating (2 h) or room-temperature stirring for 8–12 h.10 We found that this reaction can be performed under solvent-free grinding conditions, and only take 3 to 10 minutes, greatly shortening the reaction time and providing a simple strategy for synthesizing 3-methyl-4-nitro-5-styrylisoxazoles (Scheme 1). Various aromatic aldehydes (1a–q) were then used as substrates for such solvent-free grinding reactions to afford 3-methyl-4-nitro-5-styrylisoxazoles (2a–q) in good to quantitative yields. The reaction rates of aldehydes containing an electron-withdrawing group (EWG, aldehyde with Cl, Br or NO2) were found to be higher than those with an electron-donating group (EDG, aldehyde with OH or OMe). Aldehydes 1i, 1j, 1k, 1l and 1q were made to react with nitroxazole to produce corresponding aldol condensation products 2i, 2j, 2k, 2l and 2q in quantitative yields by using 30 mol% of pyrrolidine catalyst grinding at room temperature. All of the tested aldehydes provided aldol condensation products with 10 minutes of grinding, and the results are summarized in Fig. 3.
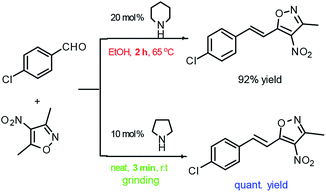 |
| Scheme 1 The preparation of 3-methyl-4-nitro-5-styrylisoxazole carried out using solvent-free grinding. | |
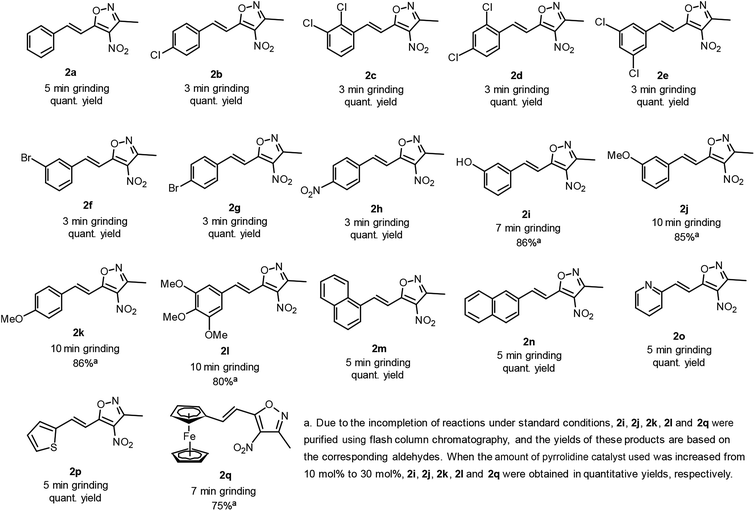 |
| Fig. 3 Pyrrolidine-catalyzed solvent-free grinding preparation of 3-methyl-4-nitro-5-styrylisoxazoles 2a–q (1.0 mmol scale). | |
Tandem grinding reactions involving aldol condensation and Michael addition in sequence for preparation of 3,4,5-trisubstituted isoxazoles
Initially, the Michael reaction of 3-methyl-4-nitro-5-styrylisoxazole 2b with ethyl 2-nitroacetate 3a was used as a model reaction to investigate the grinding Michael reaction. Various bases including Na2CO3, K2CO3, Et3N, iPr2NEt, N-methylmorpholine (NMM), 1,8-diazabicyclo[5,4,0]undec-7-ene (DBU) and 1,4-diazabicyclo[2.2.2]octane (DABCO) were each used as a catalyst for this reaction. The results are shown in Scheme 2. Of these bases, the simple tertiary amine Et3N was concluded to be the best choice for this Michael reaction because of its high efficiency, easy evaporation and convenient availability. For the first step to 3-methyl-4-nitro-5-styrylisoxazoles, pyrrolidine was used as the catalyst, and for the second step to 3,4,5-trisubstituted isoxazole, Et3N was used as the catalyst. We then considered the possibility of combining these two separate steps into one by developing a single catalyst containing both a secondary amine and tertiary amine in its structure. With this point in mind, we used A1 and A2 as catalysts, and performed a one-pot grinding reaction of 4-chlorobenzaldehyde 1b, 3,5-dimethyl-4-nitroisoxazole and ethyl 2-nitroacetate 3a. The results are shown in Scheme 3. Both A1 and A2 were found to lead to complex results.
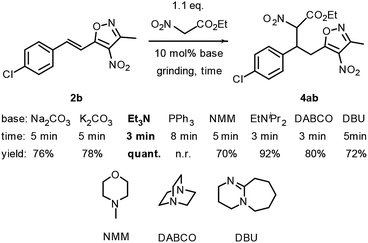 |
| Scheme 2 The screening of bases for grinding preparation of 3,4,5-trisubstituted isoxazole 4ab. | |
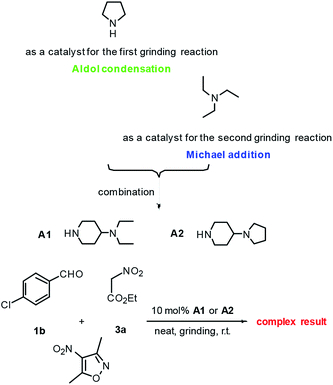 |
| Scheme 3 The screening of catalysts A1 and A2 for one-pot grinding preparation of 3,4,5-trisubstituted isoxazole 4ab. | |
Afterwards, we used pyrrolidine as a catalyst for the first grinding reaction to prepare 2b. When carrying out thin-layer chromatography (TLC) indicated that the starting materials 1b and 3,5-dimethyl-4-nitroisoxazole of this reaction were consumed, we then added ethyl 2-nitroacetate 3a and 10 mol% Et3N to the same mortar and performed another 3 minutes of grinding at room temperature. In this way, 4ab was obtained in 92% yield. We called this strategy a tandem grinding reaction. By using this strategy, we performed aldol-Michael reactions of aromatic aldehydes 1 and 3,5-dimethyl-4-nitroisoxazole and activated methylene compounds including ethyl 2-nitroacetate, alkyl 2-cyanoacetates and malononitrile to provide 3,4,5-trisubstituted isoxazoles 4 in good to excellent yields after a simple flash column chromatographic purification.
The results are shown in Fig. 4. When dialkyl malonates, ethyl acetoacetate and acetylacetone were used as Michael donors, no corresponding product was formed under standard grinding conditions even with a prolongation of reaction time. All products were determined from their 1H NMR spectra to be composed of diastereomers in a 1
:
1 ratio.
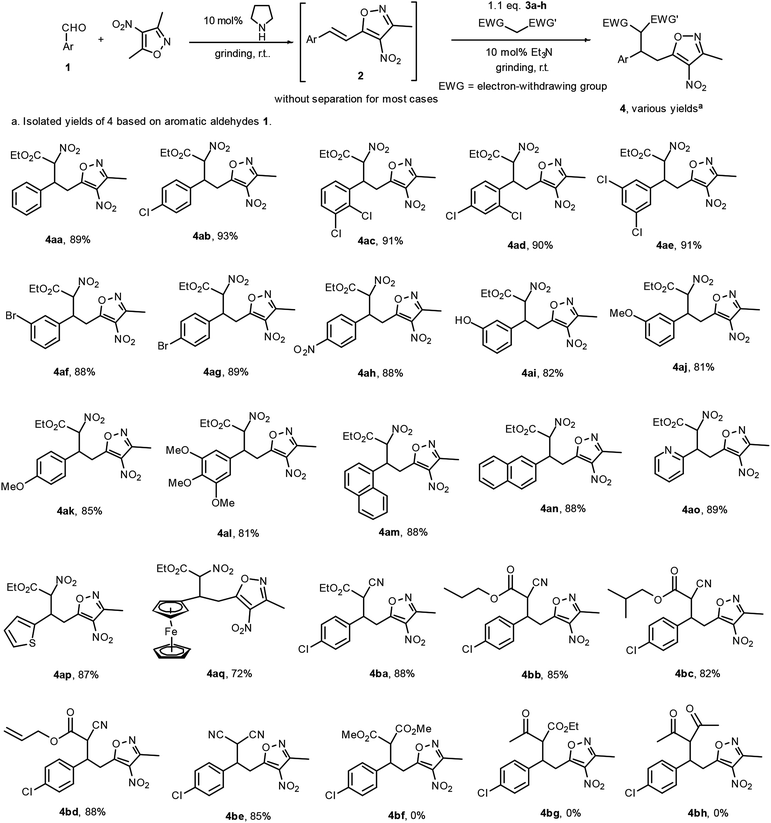 |
| Fig. 4 Tandem grinding reactions for the preparation of 3,4,5-trisubstituted isoxazoles (1.0 mmol scale). | |
Experimental
General procedure for synthesis of compound 4
To a dried agate mortar, aromatic aldehyde 1 (1 mmol), 3,5-dimethyl-4-nitroisoxazole (white crystal, 0.15 g, 1.1 mmol) and pyrrolidine (8 μL, 0.1 mmol) were added successively. The mixture was subjected to grinding at room temperature for 3–5 min, and the reaction was monitored using TLC (for most cases, the color of the reaction mixture changed obviously during the grinding process). When TLC indicated that aromatic aldehyde 1 was consumed, the activated methylene compound 3 (liquid, 1.2 mmol) and Et3N (12 μL, 0.1 mmol) were added, and grinding was carried out for another 3–5 min. (Note: aldehydes 1i, 1j, 1k, 1l and 1q were not used up under standard conditions, so the corresponding aldol condensation products 2i, 2j, 2k, 2l and 2q were separated from their respective unconsumed reactants by performing flash column chromatography.) TLC was used to check the reaction process. The crude product was diluted with DCM (20 mL) and the resulting solution was successively washed with H2O (5 mL) and brine (5 mL). The organic layers were dried over Na2SO4, filtered, and concentrated. The pure product 4 was obtained by carrying out flash column chromatography (eluted by petroleum ether/ethyl acetate = 10/1 to 5/1, v/v).
Conclusions
In conclusion, we have developed a tandem grinding strategy to prepare 3,4,5-trisubstituted isoxazoles from aromatic aldehydes, 3,5-dimethyl-4-nitroisoxazole and activated methylene compounds in the presence of catalytic amounts of pyrrolidine and Et3N in high yields and efficiency. The transformations of these 3,4,5-trisubstituted isoxazoles to complex structures for investigation of their bio-activities are underway in our laboratory.
Conflicts of interest
There are no conflicts to declare.
Acknowledgements
We thank the National Science Foundation of China (no. 21372259) for financial support of this work.
Notes and references
-
(a) S.-M. Yang, P. Karanam, M. Wang, Y.-J. Jang, Y.-S. Yeh, P.-Y. Tseng, M. R. Ganapuram, Y.-C. Liou and W.-W. Lin, Chem. Commun., 2019, 55, 1398 RSC;
(b) Y. Hayashi, T. Yamada, M. Sato, S. Watanabe, E. Kwon, K. Iwasaki and S. Umemiya, Org. Lett., 2019, 21, 5183 CrossRef CAS PubMed;
(c) Y. Hayashi, K. Nagai and S. Umemiya, Eur. J. Org. Chem., 2019, 678 CrossRef CAS;
(d) X. Zhang, T.-L. Wang, X.-J. Liu, X.-C. Wang and Z.-J. Quan, Org. Biomol. Chem., 2019, 17, 2379 RSC;
(e) M. G. Vinogradov, O. V. Turova and S. G. Zlotin, Org. Biomol. Chem., 2019, 17, 3670 RSC;
(f) T. A. Hamlin, I. Fernández and F. M. Bickelhaupt, Angew. Chem., Int. Ed., 2019, 58, 8922 CrossRef CAS PubMed;
(g) C.-K. Tang, K.-X. Feng, A.-B. Xia, C. Li, Y.-Y. Zheng, Z.-Y. Xu and D.-Q. Xu, RSC Adv., 2018, 8, 3095 RSC;
(h) S. Nayak, P. Panda, S. Bhakta, S. K. Mishra and S. Mohapatra, RSC Adv., 2016, 6, 96154 RSC;
(i) B.-L. Zhao and D.-M. Du, Chem. Commun., 2016, 52, 6162 RSC.
-
(a) D. Heijnen, M. von Zuijlen, F. Tosia and B. L. Feringa, Org. Biomol. Chem., 2019, 17, 2315 RSC;
(b) X.-T. Fang, Z.-H. Deng, W.-H. Zheng and J. C. Antilla, ACS Catal., 2019, 9, 1748 CrossRef CAS;
(c) C. H. Lam, V. Escande, K. E. Mellor, J. B. Zimmerman and P. T. Anastas, J. Chem. Educ., 2019, 96, 4761 CrossRef;
(d) T. B. Nguyen and P. Retailleau, Org. Lett., 2017, 19, 3879 CrossRef CAS PubMed;
(e) R. A. Sheldon, Green Chem., 2016, 18, 3180 RSC.
-
(a) N. A. De Simone, S. Meninno, C. Talotta, C. Gaeta, P. Neri and A. Lattanzi, J. Org. Chem., 2018, 83, 10318 CrossRef CAS PubMed;
(b) M. Bláha, O. Trhlíková, J. Podešva, S. Abbrent, M. Steinhart, J. Dybal and M. Dušková-Smrčková, Tetrahedron, 2018, 74, 58 CrossRef;
(c) C. G. Avila-Ortiz, L. Díaz-Corona, E. Jiménez-González and E. Juaristi, Molecules, 2017, 22, 1328 CrossRef PubMed;
(d) E. Drège, J. Oko, P.-E. Venot, N. Gigant and D. Joseph, RSC Adv., 2015, 5, 96720 RSC;
(e) S. Guin, D. Majee, S. Biswas and S. Samanta, Asian J. Org. Chem., 2018, 7, 1810 CrossRef CAS;
(f) A. J. Beneto, J. Sivamani, V. Ashokkumar, R. Balasaravanan, K. Duraimurugana and A. Siva, New J. Chem., 2015, 39, 3098 RSC;
(g) C. Wu, L.-H. Lu, A.-Z. Peng, G.-K. Jia, C. Peng, Z. Cao, Z.-L. Tang, W.-M. He and X.-H. Xu, Green Chem., 2018, 20, 3683 RSC;
(h) J. Kaur, A. Kumari and S. S. Chimni, Tetrahedron, 2017, 73, 802 CrossRef CAS;
(i) M. Leonardi, M. Villacampa and J. C. Menéndez, Chem. Sci., 2018, 9, 2042 RSC;
(j) T. K. r Achar, A. Bose and P. Mal, Beilstein J. Org. Chem., 2017, 13, 1907 CrossRef PubMed;
(k) M. Tavakolian, S. Vahdati-Khajeh and S. Asgari, ChemCatChem, 2019, 11, 2943 CrossRef CAS.
-
(a) T. Wang, X.-S. Qing, C.-L. Dai, Z.-J. Su and C.-D. Wang, Org. Biomol. Chem., 2018, 16, 2456 RSC;
(b) Y. Hayashi, Chem. Sci., 2016, 7, 866 RSC;
(c) S. K. Arepalli, B. Park, J.-K. Jung, K. Lee and H. Lee, Tetrahedron Lett., 2017, 58, 449 CrossRef CAS;
(d) S. Dochain, F. Vetica, R. Puttreddy, K. Rissanen and D. Enders, Angew. Chem., 2016, 128, 16387 CrossRef;
(e) B.-C. Hong, A. RajaVishal and M. Sheth, Synthesis, 2015, 47, 3257 CrossRef CAS;
(f) L. Zhen, K. Yuan, X.-Y. Li, C.-Y. Zhang, J. Yang, H. Fan and L.-Q. Jiang, Org. Lett., 2018, 20, 3109 CrossRef CAS PubMed;
(g) T. Bzeih, D. Lama, G. Frison, A. Hachem, N. Jaber, J. Bignon, P. Retailleau, M. Alami and A. Hamze, Org. Lett., 2017, 19, 4670 CrossRef PubMed.
-
(a) M. Baumann and I. R. Baxendale, Beilstein J. Org. Chem., 2013, 9, 2265 CrossRef PubMed;
(b) M. Baumann, I. R. Baxendale, S. V. Ley and N. Nikbin, Beilstein J. Org. Chem., 2011, 7, 442 CrossRef CAS PubMed.
-
(a) A. E. Garces and M. J. Stocks, J. Med. Chem., 2019, 62, 4815 CrossRef CAS PubMed;
(b) H. Zahra, R. Ali and R.-A. Nima, Curr. Org. Chem., 2018, 22, 2256 CrossRef.
-
(a) M. Börjesson, A. Tortajada and R. Martin, Chem, 2019, 5, 254 CrossRef;
(b) Q. Zhao, C. Peng, H. Huang, S.-J. Liu, Y.-J. Zhong, W. Huang, G. He and B. Han, Chem. Commun., 2018, 54, 8359 RSC.
-
(a) S. Rout, H. Joshi and V. K. Singh, Org. Lett., 2018, 20, 2199 CrossRef CAS PubMed;
(b) B. Zhu, R. Lee, Y.-L. Yin, F.-Y. Li, M. Coote and Z.-Y. Jiang, Org. Lett., 2018, 20, 429 CrossRef CAS PubMed;
(c) F. Li, W.-L. Pei, J.-J. Wang, J. Liu, J. Wang, M.-L. Zhang, Z.-M. Chen and L.-T. Liu, Org. Chem. Front., 2018, 5, 1342 RSC;
(d) M. F. A. Adamo, P. Disetti, M. Moccia, D. Salazar-Illera and S. Surisetti, Org. Biomol. Chem., 2015, 13, 10609 RSC;
(e) B. Lin, W.-H. Zhang, D.-D. Wang, Y. Gong, Q.-D. Wei, X.-L. Liu, T.-T. Feng, Y. Zhou and W.-C. Yuan, Tetrahedron, 2017, 73, 5176 CrossRef CAS;
(f) V. Sharma, J. Kaur and S. S. Chimni, Eur. J. Org. Chem., 2018, 3489 CrossRef CAS;
(g) X.-L. Liu, W.-Y. Han, X.-M. Zhang and W.-C. Yuan, Org. Lett., 2013, 15, 1246 CrossRef CAS PubMed.
- D.-X. Cui, Y.-D. Li, J.-C. Zhu, Y.-Y. Jia, A.-D. Wen and P.-A. Wang, Curr. Org. Synth., 2019, 16, 449 CrossRef CAS.
-
(a) M. F. A. Adamo, E. F. Duffy, D. Donati and P. Sarti-Fantoni, Tetrahedron, 2007, 63, 2047 CrossRef CAS;
(b) J.-L. Zhang, X.-H. Liu, X.-J. Ma and R. Wang, Chem. Commun., 2013, 49, 9329 RSC.
Footnotes |
† Electronic supplementary information (ESI) available. See DOI: 10.1039/c9ra04864b |
‡ Co-first authors. These two authors have made the same level of contribution to this work. |
|
This journal is © The Royal Society of Chemistry 2019 |