DOI:
10.1039/C9RA04540F
(Paper)
RSC Adv., 2019,
9, 26768-26772
One-step construction of unsymmetrical thioureas and oxazolidinethiones from amines and carbon disulfide via a cascade reaction sequence†
Received
17th June 2019
, Accepted 17th August 2019
First published on 27th August 2019
Abstract
A concise and versatile method for the construction of unsymmetrical thioureas and oxazolidinethiones from amines and carbon disulfide has been achieved in DMSO without addition of extra reagents. The present protocol is compatible with various secondary amines and primary amines, and suitable for intermolecular and intramolecular reactions. Diverse unsymmetrical thioureas and oxazolidinethiones were efficiently obtained in good to excellent yields via a cascade reaction sequence.
Introduction
Thioureas have attracted significant interest in medicinal chemistry due to their broad spectrum of biological activities. Their derivatives and metal complexes have been demonstrated to have antimicrobial, analgesic, anticancer and anti-inflammatory activities.1 Some of them have been developed as drugs, such as hyperthyroidism drugs,1c sedative hypnotics drugs,1d to treat diseases in clinics. Thioureas are also important in pesticide chemistry, a variety of thiourea derivatives have been used as insecticides, herbicides, rodenticides and fungicides.2 In synthetic chemistry, thioureas are useful blocking buildings for the construction of many valuable compounds, such as guanidines, amides and sulfur-containing heterocycles.3 Additionally, they also play an important role in organocatalysis as auxiliaries or catalysts.4
On account of the usefulness of thioureas in different fields, methods for synthesizing this type of compounds have been widely explored.5–8 Generally, three synthetic strategies are commonly used for the preparation of substituted thioureas. One way is transforming carbonyl to thiocarbonyl employing Lawesson reagents or P2S5.6 The second way is condensation or substitution of amines with prepared isothiocyanate, 1-(methyldithiocarbonyl)imidazole, thiocarbamoylbenzotriazoles, or derivatives thereof.7 Based on these two synthetic strategies, many methods were developed for the synthesis of symmetrical and unsymmetrical thioureas. However, these protocols require extra synthetic steps for the preparation of starting materials, leading to low synthetic efficiency and a poor substrate scope. The third alternative way is the direct reaction of amines and carbon disulfide.8 In this transformation, starting materials are easy to be gained and no extra synthetic steps are required. Therefore, it is considered as a straightforward route for the preparation of substituted thioureas. In this regard, several methods have been developed on the basis of this strategy for the preparation of symmetrical and unsymmetrical thioureas. Nevertheless, the reported methods for the synthesis of unsymmetrical thioureas contain two steps, two kinds of amines need to be separately added to the reaction system under basic or microwave conditions (Scheme 1a).8e,8f In 2017, the Jiang's group reported a novel method for the preparation of unsymmetrical thioureas with amines, sulfur and chloroform in the presence of potassium tert-butoxide in mixture solvents (Scheme 1b).9 This transformation also contains two steps. A one-step reaction for the synthesis of unsymmetrical thioureas was achieved by Nguyen's group with isocyanides, amines and elemental sulfur (Scheme 1c).10 However, this protocol is only suitable for aliphatic amines. Obviously, the development of convenient and versatile methods for one-step construction of unsymmetrical thioureas is in high demand.
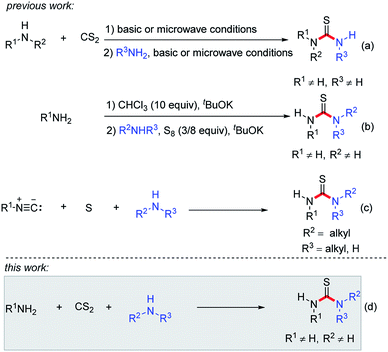 |
| Scheme 1 Straightforward methods for the synthesis of unsymmetric thioureas. | |
Cascade reactions,11 which involve multistep transformations in a one-pot fashion, are attractive in modern synthetic chemistry due to their simplicity and atom-economy. In continuation of our study on the development of efficient routes for the preparation or modification of heteroatom-containing compounds,12 we are of great interest in the exploration of new routes for the preparation of unsymmetrical thioureas. Herein, we wish to disclose a novel protocol for the synthesis of unsymmetrical thioureas via a cascade reaction sequence (Scheme 1c).
Results and discussion
Methods for the preparation of unsymmetrical thioureas using naphthylamines as substrates are rare.1f,7g,9 Our exploration started with using 2-naphthylamine (1a), carbon disulfide (2) and diethylamine (3a) as model substrates, all substrates were added into the reaction system in one step. Initially, the reaction was performed in DCE at 70 °C. Unfortunately, only a trace amount of the desired product was observed after 12 h (Table 1, entry 1). Changing DCE to toluene, the desired product was obtained in 58% isolated yield (entry 2).
Table 1 Optimization of the reaction conditionsa

|
Entry |
Solvent |
Time (h) |
Yieldb (%) |
Reaction conditions: 1a (0.2 mmol), 2 (0.4 mmol), 3a (0.4 mmol) in solvent (2 mL) at 70 °C. Yield refers to isolated products after column chromatography. Reaction was performed at 40 °C. 1.2 equiv. of 2 and 3a were used. 10 mmol of 1a were used. HFIP = hexafluoroisopropanol. |
1 |
DCE |
12 |
Trace |
2 |
Toluene |
12 |
58 |
3 |
THF |
12 |
72 |
4 |
MeOH |
12 |
78 |
5 |
DMSO |
1.5 |
89 |
6 |
MeCN |
12 |
81 |
7 |
DMF |
4 |
85 |
8 |
HFIP |
12 |
Trace |
9 |
Chlorobenzene |
12 |
69 |
10c |
DMSO |
12 |
62 |
12d |
DMSO |
1 |
95 |
14e |
DMSO |
12 |
89 |
Encouraged by this result, the reaction was examined with different solvents to improve the yield of 4a. It was found that DMSO was the best solvent for this transformation compared to THF, MeOH, MeCN, HFIP and chlorobenzene (entries 3–9); the reaction provided the expected product in 89% yield in 1.5 h (entry 5). This is probably because the solvent increased the basicity of the amines. Dropping the temperature to 40 °C, the reaction time was prolonged and the yield of the product was lowered (entry 10). To our delight, the product was obtained in 95% yield when the amounts of carbon disulfide and diethyl amine were changed to 1.2 equiv. (entry 12). Therefore, the optimal conditions for the synthesis of unsymmetrical thioureas via acascade reaction sequence were gained: carbon disulfide (1.2 equiv.), diethyl amine (1.2 equiv.), in DMSO (2 mL) at 70 °C for 1 h (entry 12). Moreover, a 10 mmol scale reaction was carried out under optimal reaction conditions, affording the desired product in 89% yield (entry 14). This result demonstrated the easy scalability of this transformation.
Having identified the optimal parameters of the model reaction, the substrate scope and generality of this transformation were evaluated. First of all, various secondary amines were tested with 2-naphthylamine under standard reaction conditions (Table 2, 4b–4m). Application of N-methylcyclohexylamine was successful, providing the desired product in 94% yield (4b). Changing dialkylamines to N-benzylethanamine or dibenzylamine, the corresponding products were afforded with 90% and 95% yields, respectively (4c, 4d). Reactions of 2-naphthylamine with cyclic amines containing different ring numbers, substituents or heteroatoms proceeded well, giving the related products in good to excellent yields (4e–4m). Subsequently, we turned our attention to explore the reaction of 2-naphthylamines with primary amines under the optimal conditions. To our delight, non-cyclic and cyclic alkylamines were suitable for this transformation, the desired products were obtained in good yields in 24 h (4n, 4o). This protocol was also applicable for benzylamines. Reactions of benzylamines with various substituents at different positions of benzene ring worked well, affording the desired products in good to excellent yields (4p–4r). Aniline and its derivatives were also examined in this transformation. Gratifyingly, when methyl or methoxyanilines were used as substrates, the expected products were obtained in good yields in 12 h (4s, 4t). However, reactions of aniline or anilines with electron-withdrawing substituents provided not only unsymmetrical thioureas but also symmetrical thioureas from the reactions of anilines with carbon disulfide, and the formed two products were inseparable due to their similar polarity. This is probably because of weak nucleophilicity of these anilines. Application of 3-aminopyridine in this transformation was successful, the desired product was obtained in 78% yield in 12 h (4u). Intriguingly, this protocol was also suitable for the reactions of 3-aminoquinoline and anilines, the desired products were gained in good yields (4v–4z).13 Symmetrical thioureas were obtained from the reactions of anilines with weak nucleophilicity, which were consistent with the results of the reactions of 2-naphthylamine and anilines. This transformation allows the preparation of unsymmetrical thioureas using diverse naphthylamines, aminoquinolines and aminoisoquinolines (4aa–4ai). Moreover, the reactions of anilines with benzyl amines were successful, the corresponding products were obtained in excellent yields (4aj, 4ak).
Table 2 Exploration of substrate scope of aminesa
Reaction conditions: 1 (0.2 mmol), 2 (0.24 mmol), 3 (0.24 mmol) in DMSO (2 mL) at 70 °C. 0.24 mmol of 1 were used, 0.2 mmol of 3 were used. |
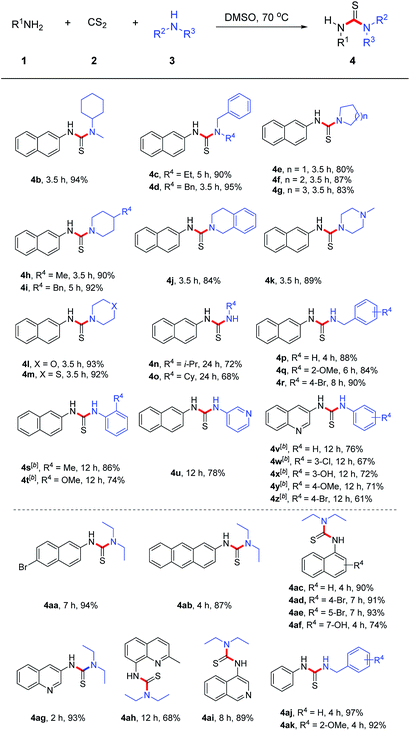 |
After successful application of this novel transformation for the preparation of unsymmetrical thioureas by intermolecular reactions of different amines with carbon disulfide, we wondered if this protocol is suitable for the intramolecular reactions.7c,8c,8d,8g,9 Reactions of naphthylamine, aniline, alkylamine and benzylamine were tested under standard reaction conditions, all of them gave the desired products in excellent yields (Table 3, 6a–6d). To our delight, this approach was also applicable for the construction of oxazolidinethiones (6e–6i), which are great of importance in organic chemistry and medicinal chemistry.9,14
Table 3 Exploration of intramolecular reactionsa

|
Reaction conditions: 5 (0.2 mmol), 2 (0.24 mmol) in DMSO (2 mL) at 70 °C. |
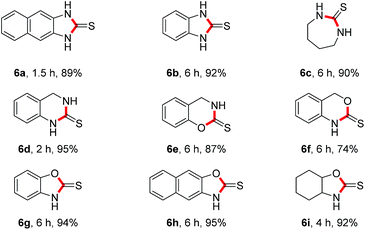 |
We also investigated the mechanism of this protocol. Radical trapping experiments were examined in the presence of 1.2 equiv. of TEMPO (2,2,6,6-tetramethyl-1-piperidinyloxy) or BHT (butylhydroxytoluene) under the standard reaction conditions (Scheme 2(a) and (b)). Almost no inhibition of the generation of the desired product 4a was observed. These results indicated that this process did not involve radicals. In order to understand the sequence of this three components reaction, the reaction of 2-naphthylamine and carbon disulfide was carried out under the standard reaction conditions. After 1 h, almost no reactions occurred (Scheme 2(c)). A new spot was observed from the reaction of diethylamine and carbon disulfide under the standard reaction conditions, it was confirmed as diethylcarbamodithioic acid (Scheme 2(d)).8d–f Subsequently, adding 2-naphthylamine to the reaction system, the desired product was obtained in 95% yield. These experiments suggested that this transformation was initiated by the reaction of diethyl amine and carbon disulfide.
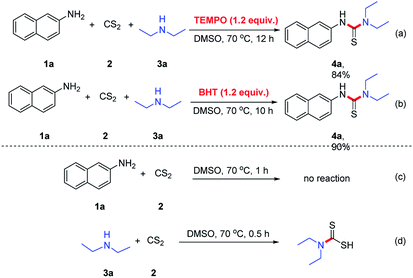 |
| Scheme 2 Mechanistic investigation. | |
On the basis of relevant reports in literatures8d–f and our experimental results, a tentative mechanism for this transformation was proposed by taking 2-naphthylamine 1a and diethylamine 3a as examples (Scheme 3).15 Initially, intermediate I is formed by nucleophilic attack of diethylamine 3a to carbon disulfide. Subsequently, 2-naphthylamine 1a attacks the intermediate I, affording the desired products 4a with concomitant release of H2S.
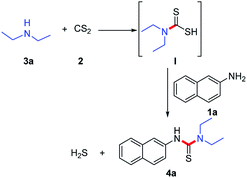 |
| Scheme 3 Proposed mechanism. | |
Conclusions
In conclusion, we have developed an efficient and versatile protocol for the preparation of thioureas and oxazolidinethiones from amines and carbon disulfide via a cascade reaction sequence. This approach features mild reaction conditions, good compatibility with different amines as well as broad substrate scopes. Further investigations on biological activity of the synthesized thioureas and oxazolidinethiones are ongoing in our laboratory.
Experimental
A mixture of amine 1 (0.2 mmol), carbon disulfide 2 (0.24 mmol), amine 3 (0.24 mmol) or amine 5 (0.2 mmol), carbon disulfide 2 (0.24 mmol) in DMSO (2 mL) was added in a 5 mL glass tube, which was stirred at 70 °C for 1–12 h. When the reaction was completed, it was mixed with water and ethyl acetate. The reaction mixture was extracted three times with ethyl acetate. The combined organic layer was dried over anhydrous magnesium sulfate and filtered. The filtrate was evaporated under vacuum and the residue was purified by flash column chromatography on silica gel (eluting with petroleum ether-ethyl acetate) to provide the desired products 4 or 6.
Conflicts of interest
There are no conflicts to declare.
Acknowledgements
This study was supported by the National Key Research Project (2017YFA0506000), National Natural Science Foundation of China (21807080, 21572166), Natural Science Foundation of Zhejiang Province (LQ18B020004).
Notes and references
-
(a) M. Weiss, R. Buhl, M. Medve and E. M. Schneider, Crit. Care Med., 1997, 25, 128 CrossRef CAS PubMed
;
(b) J. Lee, J. Lee, M. Kang, M. Shin, J. M. Kim, S. U. Kang, J. O. Lim, H. K. Choi, Y. G. Suh, H. G. Park, U. Oh, H. D. Kim, Y. H. Park, H. J. Ha, Y. H. Kim, A. Toth, Y. Wang, R. Tran, L. V. Pearce, D. J. Lundberg and P. M. Blumberg, J. Med. Chem., 2003, 46, 3116 CrossRef CAS PubMed
;
(c) E. Rodríguez-Fernández, J. L. Manzano, J. J. Benito, R. Hermosa, E. Monte and J. J. Criado, J. Inorg. Biochem., 2005, 99, 1558 CrossRef PubMed
;
(d) O. J. D'Cruz and F. M. Uckun, Curr. HIV Res., 2006, 4, 329 CrossRef
;
(e) I. V. Daele, H. Munier-Lehmann, M. Froeyen, J. Balzarini and S. V. Calenbergh, J. Med. Chem., 2007, 50, 5281 CrossRef PubMed
;
(f) M. I. C. Begum and K. M. Khan, Nat. Prod. Res., 2009, 23, 1719 CrossRef PubMed
;
(g) Y. Touati-Jallabe, E. Bojnik, B. Legrand, E. Mauchauffée, N. N. Chung, P. W. Schiller, S. Benyhe, M. C. Averlant-Petit, J. Martinez and J. F. Hernandez, J. Med. Chem., 2013, 56, 5964 CrossRef CAS PubMed
;
(h) L. Y. Ma, Y. C. Zheng, S. Q. Wang, B. Wang, Z. R. Wang, L. P. Pang, M. Zhang, J. W. Wang, L. Ding, J. Li, C. Wang, B. Hu, Y. Liu, X. D. Zhang, J. J. Wang, Z. J. Wang, W. Zhao and H. M. Liu, J. Med. Chem., 2015, 58, 1705 CrossRef CAS PubMed
. -
(a) N. G. Gratz, Bull. W. H. O., 1973, 48, 469 CAS
;
(b) G. Y. Sarkis and E. D. Faisal, J. Heterocycl. Chem., 1985, 22, 137 CrossRef CAS
;
(c) C. Walpole, S. Y. Ko, M. Brown, D. Beattie, E. Campbell, F. Dickenson, S. Ewan, G. A. Hughes, M. Lemaira, J. Lerpiniere, S. Patel and L. Urban, J. Med. Chem., 1998, 41, 3159 CrossRef CAS PubMed
;
(d) H. Kayser and P. Eilinger, Pest Manage. Sci., 2001, 57, 975 CrossRef CAS PubMed
;
(e) B. L. Wang, H. W. Zhu, Y. Ma, L. X. Xiong, Y. Q. Li, Y. Zhao, J. F. Zhang, Y. W. Chen, S. Zhou and Z. M. Li, J. Agric. Food Chem., 2013, 61, 5483 CrossRef CAS PubMed
. -
(a) D. G. Patil and M. R. Chedekel, J. Org. Chem., 1984, 49, 997 CrossRef CAS
;
(b) P. C. Kearney, M. Fernandez and J. A. Flygare, J. Org. Chem., 1998, 63, 196 CrossRef CAS PubMed
;
(c) M. Kidwai, R. Venkataramanan and B. Dave, Green Chem., 2001, 3, 278 RSC
;
(d) D. Anshu, A. Kapil and S. Meha, Synth. Commun., 2004, 34, 1141 CrossRef
;
(e) R. Yella, H. Ghosh and B. K. Patel, Green Chem., 2008, 10, 1307 RSC
;
(f) I. Cano, E. Gómez-Bengoa, A. Landa, M. Maestro, A. Mielgo, I. Olaizola, M. Oiarbide and C. Palomo, Angew. Chem., Int. Ed., 2012, 51, 10856 CrossRef CAS PubMed
;
(g) J. Zhao, H. Huang, W. Wu, H. Chen and H. Jiang, Org. Lett., 2013, 15, 2604 CrossRef CAS PubMed
. -
(a) D. J. Ager, I. Prakash and D. R. Schaad, Chem. Rev., 1996, 96, 835 CrossRef CAS PubMed
;
(b) P. R. Schreiner, Chem. Soc. Rev., 2003, 32, 289 RSC
;
(c) P. M. Pihko, Angew. Chem., Int. Ed., 2004, 43, 2062 CrossRef CAS PubMed
;
(d) Y. Hoashi, T. Okino and Y. Takemoto, Angew. Chem., Int. Ed., 2005, 44, 4032 CrossRef CAS PubMed
;
(e) T. Inokuma, Y. Hoashi and Y. Takemoto, J. Am. Chem. Soc., 2006, 128, 9413 CrossRef CAS PubMed
;
(f) Y. Zhang, Y. K. Liu, T. R. Kang, Z. K. Hu and Y. C. Chen, J. Am. Chem. Soc., 2008, 130, 2456 CrossRef CAS PubMed
;
(g) Á. Madarász, Z. Dósa, S. Varga, T. Soós, A. Csámpai and I. Pápai, ACS Catal., 2016, 6, 4379 CrossRef
. -
(a) F. Kurzer and K. Douraghi-Zadeh, Chem. Rev., 1967, 67, 107 CrossRef CAS PubMed
;
(b) B. Love, P. E. Bender, H. Bowman, A. Helt, R. Mclean and T. Jen, J. Med. Chem., 1972, 15, 1024 CrossRef PubMed
;
(c) C. Larsen, K. Steliou and D. N. Harpp, J. Org. Chem., 1978, 43, 337 CrossRef CAS
;
(d) C. R. Rasmussen, F. J. Villani Jr, L. E. Weaner, B. E. Reynolds, A. R. Hood, L. R. Hecker, S. O. Nortey, A. Hanslin, M. J. Costanzo, E. T. Powell and A. J. Molinari, Synthesis, 1988, 456 CrossRef CAS
;
(e) H. Sugimoto, I. Makino and K. Hirai, J. Org. Chem., 1988, 53, 2263 CrossRef CAS
;
(f) K. Ramadas and N. Srinivasan, Synth. Commun., 1995, 25, 3381 CrossRef CAS
;
(g) M. Xian, X. Zhu, Q. Li and J. P. Cheng, Tetrahedron Lett., 1999, 40, 1957 CrossRef CAS
;
(h) B. Yin, Z. Liu, M. Yi and J. Zhang, Tetrahedron Lett., 2008, 49, 3687 CrossRef CAS
. -
(a) T. Ozturk, E. Ertas and O. Mert, Chem. Rev., 2007, 107, 5210 CrossRef CAS PubMed
;
(b) T. Ozturk, E. Ertas and O. Mert, Chem. Rev., 2010, 110, 3419 CrossRef CAS PubMed
. -
(a) H. A. Staab, Angew. Chem., Int. Ed., 1962, 1, 351 CrossRef
;
(b) G. L'abbe and S. Leurs, Tetrahedron, 1992, 48, 7505 CrossRef
;
(c) P. K. Mohanta, S. Dhar, S. K. Samal, H. Ila and H. Junjappa, Tetrahedron, 2000, 56, 629 CrossRef CAS
;
(d) A. R. Katritzky, S. Ledoux, R. M. Witek and S. K. Nair, J. Org. Chem., 2004, 69, 2976 CrossRef CAS PubMed
;
(e) A. Ortiz and E. Sansinenea, J. Sulfur Chem., 2007, 28, 109 CrossRef CAS
;
(f) J. Li, Z. Tan, S. Tang, I. Hewlett, R. Pang, M. He, S. He, B. Tian, K. Chen and M. Yang, Bioorg. Med. Chem., 2009, 17, 3177 CrossRef CAS PubMed
;
(g) M. I. C. Begum and K. M. Khan, Nat. Prod. Res., 2009, 23, 1719 CrossRef PubMed
;
(h) M. Đud, O. V. Magdysyuk, D. Margetića and V. Štrukil, Green Chem., 2016, 18, 2666 RSC
. -
(a) J. Bernstein, H. L. Yale, K. Losee, M. Holsing, J. Martins and W. A. Lott, J. Am. Chem. Soc., 1951, 73, 906 CrossRef CAS
;
(b) J. Erickson, J. Org. Chem., 1956, 21, 483 CrossRef CAS
;
(c) M. Ballabeni, R. Ballini, F. Bigi, R. Maggi, M. Parrini, G. Predieri and G. Sartori, J. Org. Chem., 1999, 64, 1029 CrossRef CAS PubMed
;
(d) M. R. Maddani and K. R. Prabhu, J. Org. Chem., 2010, 75, 2327 CrossRef CAS PubMed
;
(e) Z. Zhang, H. H. Wu and Y. J. Tan, RSC Adv., 2013, 3, 16940 RSC
;
(f) C. M. Chau, T. J. Chuan and K. M. Liu, RSC Adv., 2014, 4, 1276 RSC
;
(g) T. Haywood, S. Kealey, S. Sánchez-Cabezas, J. J. Hall, L. Allott, G. Smith, C. Plisson and P. W. Miller, Chem.–Eur. J., 2015, 21, 9034 CrossRef CAS PubMed
. - W. Tan, J. Wei and X. Jiang, Org. Lett., 2017, 19, 2166 CrossRef CAS PubMed
. - T. B. Nguyen, L. Ermolenko and A. Al-Mourabit, Synthesis, 2014, 46, 3172 CrossRef CAS
. -
(a) B. M. Trost, Science, 1991, 254, 1471 CrossRef CAS
;
(b) M. Malacria, Chem. Rev., 1996, 96, 289 CrossRef CAS
;
(c) K. C. Nicolaou, T. Montagnon and S. A. Snyder, Chem. Commun., 2003, 34, 551 RSC
;
(d) K. C. Nicolaou, D. J. Edmonds and P. G. Bulger, Angew. Chem., Int. Ed., 2006, 45, 7134 CrossRef CAS
;
(e) C. Grondal, M. Jeanty and D. Enders, Nat. Chem., 2010, 2, 167 CrossRef CAS
;
(f) J. Xie, H. Jiang, Y. Cheng and C. Zhu, Chem. Commun., 2012, 48, 979 RSC
;
(g) H. G. Cheng, L. Q. Lu, T. Wang, Q. Q. Yang, X. P. Liu, Y. Li, Q. H. Deng, J. R. Chen and W. J. Xiao, Angew. Chem., Int. Ed., 2013, 52, 3250 CrossRef CAS PubMed
;
(h) H. Pellissier, Chem. Rev., 2013, 113, 442 CrossRef CAS PubMed
;
(i) C. M. R. Volla, I. Atodiresei and M. Rueping, Chem. Rev., 2014, 114, 2390 CrossRef CAS PubMed
. -
(a) Z. Song, Y. M. Zhao and H. Zhai, Org. Lett., 2011, 13, 6331 CrossRef CAS PubMed
;
(b) Y. Yu, Y. Zhou, Z. Song and G. Liang, Org. Biomol. Chem., 2018, 16, 4958 RSC
;
(c) C. Ding, Y. Yu, Q. Yu, Z. Xie, Y. Zhou, J. Zhou, G. Liang and Z. Song, ChemCatChem, 2018, 10, 5397 CrossRef CAS
. - For aniline, 3-chloroaniline and 4-bromoaniline, the symmetrical thioureas from the reactions of these anilines and carbon disulfide were isolated in 21%, 14% and 17% yields, respectively.
-
(a) B. Krebs and G. Henkel, Angew. Chem., Int. Ed., 1991, 30, 769 CrossRef
;
(b) F. Velazquez and H. F. Olivo, Curr. Org. Chem., 2002, 6, 303 CrossRef CAS
;
(c) A. Ortiz and E. Sansinenea, J. Sulfur Chem., 2007, 28, 109 CrossRef CAS
;
(d) S. Sahu, P. R. Sahoo, S. Patel and B. K. Mishra, J. Sulfur Chem., 2011, 32, 171 CrossRef CAS
. - For the reactions of naphthylamines, primary amines (alkylamines, benzyl amines, anilines) and carbon disulfide: dithiocarbamates were initially formed by the reactions of primary amines with carbon disulfide, subsequently they were converted to isothiocyanates (for example, J. Org. Chem., 2010, 75, 2327; RSC Adv., 2013, 3, 16940). These reactions not only generated unsymmetrical thioureas by the reactions of the formed isothiocyanates with naphthylamines, but also produced symmetrical thioureas by the reactions of the formed isothiocyanates with primary amines (like anilines) which have similar nucleophilicity with naphthylamines.
Footnote |
† Electronic supplementary information (ESI) available. See DOI: 10.1039/c9ra04540f |
|
This journal is © The Royal Society of Chemistry 2019 |