DOI:
10.1039/C9RA04220B
(Paper)
RSC Adv., 2019,
9, 22891-22899
Solubility of hydrocarbon oils in alcohols (≤C6) and synthesis of difusel carbonate for degreasing†
Received
4th June 2019
, Accepted 4th July 2019
First published on 24th July 2019
Abstract
The empirical solubility of hydrocarbon fluids, polyalphaolefin (PAO) and mineral oil, in thirteen small molecular weight alcohols (C1–C6) was determined. Butanols, pentanols, and 1-hexanol could dissolve up to PAO-10 and mineral oil. tert-Pentanol and 1-hexanol could also dissolve high-viscosity PAO-150. The dialkyl carbonate of fusel oil (DFC) was synthesized from dimethyl carbonate in 69% yield. DFC had excellent non-polar solubility and could dissolve PAO-150 and several common industrial lubricants. The flash point of DFC was 93 °C, more than twice that of isoamyl alcohol. DFC had net heating value of 30.47 MJ kg−1, nearly double that of dimethyl carbonate. However, its derived cetane number of 22.8 indicates DFC could not be used directly as diesel fuel.
Introduction
Lubricants are vital materials which function to decrease friction and dissipate heat where moving parts such as bearings make contact.1–3 Bearings are made from steels machined to precise tolerances that require lubrication to extend their service lifetime.4 Lubricants are generally manufactured from non-polar base oils containing an ‘additives package' specific to the application (antiwear, rust inhibitors, etc.). Base oils are typically fractions from petroleum refining such as mineral oils (paraffinic and naphthenic) or they can be synthetic products such as polyalphaolefin (PAO). PAO are liquid hydrocarbon polymers made by the oligomerization of terminal alkene (e.g. 1-decene) followed by hydrogenation of residual unsaturation.5 PAO are superior base oils as compared to mineral oils in nearly all key features including pour point, viscosity index, volatility and both thermal and oxidative stability.6 Owing to these excellent properties and consistent quality, and despite their higher cost, PAO base oils have gained widespread use in the manufacture of both liquid lubricants and semi-solid greases7 for a variety of applications including crankcase oils, gas turbine oils, hydraulic fluids and roller bearings.
All such lubricated machinery must undergo periodic disassembly (e.g. teardown) and inspection as part of a service schedule which requires removal of the lubricant in a process commonly referred to as degreasing. Legacy halogenated solvents have often been employed for degreasing such as trichloroethylene (TCE), perchloroethylene (1,1,2,2-tetrachloroethene, PERC) and n-propyl bromide (1-bromopropane, nPB). These solvents dissolve non-polar soils very well and are inherently non-flammable, however they are carcinogens and pose great health risks. Paraffinic petroleum distillates (e.g. mineral spirits) are also used for degreasing with flash points ranges >61 °C for safety purposes. Past use of these solvents and their spills, leaks and otherwise improper disposal has led to contamination of soil and groundwater at many military and industrial sites.8 These halocarbons remain vital products for metal cleaning, typically for vapor degreasing, and only in 2017 has a ban of TCE been proposed in the U.S.9
There is new legislation in the U.S. which now requires federal agencies, including the military, to purchase cleaning products that are biobased or otherwise derived from renewable materials.10,11 Ethyl lactate (ethyl(S)-2-hydroxypropionate) may be one such solvent touted as an effective cleaner for many kinds of soils that can be produced from natural products and is readily biodegradable.12,13 Clearly new solvents that can clean well and that are safer for technicians and the environment, and possibly sustainable, are needed. There have been several studies attempting to employ mathematical comparisons of various physicochemical properties to identify new degreasing solvents.14,15 While these models may one day be fruitful, an empirical study to determine the solubility of two different fluids (e.g. cleaning solvent and soil) may be the most concise method.16
There is a rich history in the use of alcohols as solvents in the U.S. though primarily for removal or polar soils.17 As part of a project to find new degreasing solvents for the triservices that are equally effective to current technologies but have less burden on the environment, the solubility of hydrocarbon base oils in simple aliphatic alcohols was investigated. We hypothesized that longer chain alcohols could have sufficient non-polar character to become an interesting degreasing solution with low toxicity and ready biodegradability.18–21 In this brief report, the qualitative solubility of hydrocarbon base oil, PAO and mineral oil, in some common, low molecular weight alcohols (≤C6) will be shown. We then synthesized the dialkyl carbonate from fusel oil, a by-product of higher alcohols from ethanol production, by transesterification of dimethyl carbonate.22,23 Difusel carbonate (DFC) had wider hydrocarbon solubility than its parent alcohols as well as much higher flash point for increased safety. Basic fuel properties of DFC were collected as well, including derived cetane number, calorific value, density and viscosity.
Experimental
Materials
Polyalphaolefin (PAO) with kinematic viscosities (100 °C) of 2, 5, 10, 65 and 150 mm2 s−1 (Spectrasyn™ and Spectrasyn Elite™) was obtained from the ExxonMobil Chemical Company. The various industrial lubricant products were obtained from commercial sources. Mineral oil USP was obtained from a local retail pharmacy. The aliphatic alcohols were obtained from Sigma-Aldrich in 99% purity and used as received. Crude fusel oil was obtained from Archer Daniels Midland Co. Titanium tetraisopropoxide (Ti(OiPr)4), dimethyl carbonate (DMC), ethyl lactate and all other reagents were obtained from Sigma-Aldrich and used as received.
Solubility experiments
The solubility experiments were all conducted at room temperature. A clear glass test tube was charged with PAO or mineral oil (50 mg) and then alcohol or dialkyl carbonate (250 mg) was added, a 1
:
5 weight ratio respectively. A 10× magnification hand loupe was helpful in noting changes. Upon gentle mixing Schlieren lines,24 caused by changes in density and the refraction of light, were briefly observed for those hydrocarbons that dissolved in alcohol or dialkyl carbonate. Soluble mixtures were a single phase upon complete dissolution. Insoluble mixtures, however, appeared as an opaque emulsion during mixture returning to two phases shortly afterwards. All of the experiments were carried out similarly except for tert-butanol which was first melted by warming in the hand, and then the hydrocarbon added dropwise into the alcohol.
Drying of crude fusel oil
Crude fusel oil (244 g) was placed in a separatory funnel along with saturated brine (1 L). The mixture was vigorously shaken for 20 min and then allowed to settle over 1 h. The lower brine layer was then removed from the upper fusel oil layer. The fusel oil was transferred to an Erlenmeyer flask equipped with magnetic stirring bar and mixed with anhydrous MgSO4 (15 g, 6 wt% loading) on a stirplate at rt for 1 h. Afterwards, the mixture was filtered on a medium porosity glass filter frit to remove the drying agent and obtain the dry fusel oil which was taken onto the next step.
Synthesis of difusel carbonate (DFC)
A round-bottomed flask (500 mL) equipped with magnetic stirring bar and reflux condenser was filled with previously dried fusel oil (150 mL, 121.5 g), DMC (57.6 mL, 61.6 g, 0.68 mol) and Ti(OiPr)4 (408 μL, 390 mg, 1.3 mmol, 0.2 wt%). The mixture was gently refluxed for 12 h. The mixture was cooled to rt and then a Vigreux column (22 cm) and distillation head were equipped. The mixture was distilled at atmospheric pressure (Variac 30%). A fraction containing a mixture of MeOH and DMC (62–57 °C) was slowly collected over 4 h. Next the mixture was distilled at moderately reduced pressure (60 Torr, Variac 25%) to obtain a second fraction (70–80 °C) which was primarily isoamyl alcohol. Finally, the mixture was distilled at further reduced pressure (11 Torr, Variac 30%) to collect the DFC (100–122 °C). Total yield of the colorless product was 93.97 g, and 68% yield assuming the crude fusel oil as 100% isoamyl alcohol (1.37 mol). Elemental analysis calculated for C11H22O3: C, 65.31; H, 10.96. Found: C, 65.59; H, 11.13.
Results and discussion
Hydrocarbon base oil solubility in alcohol
Determining miscibility of alcohol and hydrocarbon base oil, forming a solution when mixed in any proportion, was not necessary because in the typical degreasing operation, the soiled part is brought into contact with an excess of solvent either by manual washing and/or partial or total submersion.25 Thus, our empirical solubility experiment was simply a visual determination whether or not the hydrocarbon fluid was soluble forming a single-phased solution in excess alcohol, 1
:
5 weight ratio, respectively. A variety of PAO weights (2–150 mm2 s−1 @ 100 °C) and mineral oil were tested since they have uses in different lubricant applications. The chosen alcohols and their ability to dissolve the base oils are collected in Table 1. It should be pointed out that alcohols which are described as insoluble may have in fact dissolved some of the lighter components of the oil, but such a detailed analysis was beyond the scope of this preliminary study. Methanol was unable to dissolve any of the hydrocarbon oils tested. Ethanol could only dissolve PAO-2, which is essentially the dimer of 1-decene (C20H46). The next higher homologs, 1-propanol and isopropanol, could not surpass ethanol. The isomers of butanol were interesting and a step toward greater non-polar character. 1-Butanol was able to dissolve both PAO-2 and PAO-5 yet 2-butanol could only dissolve PAO-2. For the branched isomers, isobutanol could dissolve both PAO-2 and PAO-5 while tert-butanol dissolved PAO-2, PAO-5 and PAO-10 as well as mineral oil. The latter result is remarkable since tert-butanol is completely miscible with water and yet has greater non-polar character than its isomers.26 Continuing on, 1-pentanol, 2-pentanol and the branched isomers isoamyl and 2-methyl-1-butyl alcohols showed solubility equivalent to that of tert-butanol. These data corroborate an early report on the use of amyl alcohols to dissolve paraffinic petroleum oils for dewaxing at low temperatures.27 Similar to tert-butanol, a great leap in solubility occurred with tert-pentanol which could dissolve all the hydrocarbons tested. Finally, 1-hexanol appeared to represent the gateway to very non-polar character of primary alcohols solubilizing all the base oils tested. This was remarkable when one considers that PAO-150 is poly(1-decene)n with an weight average molecular weight (Mw) of ∼9000 (n ≅ 63).28
Table 1 Empirical solubility of hydrocarbon base oil in alcohols;a and flash points and structures of the alcohols tested
Alcohol |
Structure |
Flash pointb (°C) |
2 |
5 |
PAOc 10 |
65 |
150 |
Min. oild |
s = soluble; i = insoluble. Safety data sheets. mm2 s−1 @ 100 °C. Mineral oil. |
Methanol |
 |
9 |
i |
i |
i |
i |
i |
i |
Ethanol |
 |
14 |
s |
i |
i |
i |
i |
i |
1-Propanol |
 |
22 |
s |
i |
i |
i |
i |
i |
2-Propanol |
 |
12 |
s |
i |
i |
i |
i |
i |
1-Butanol |
 |
35 |
s |
s |
i |
i |
i |
i |
2-Butanol |
 |
27 |
s |
i |
i |
i |
i |
i |
Isobutanol |
 |
28 |
s |
s |
i |
i |
i |
i |
tert-Butanol |
 |
11 |
s |
s |
s |
i |
i |
s |
1-Pentanol |
 |
49 |
s |
s |
s |
i |
i |
s |
2-Pentanol |
 |
34 |
s |
s |
s |
i |
i |
s |
Isoamyl alcohol |
 |
43 |
s |
s |
s |
i |
i |
s |
2-Methyl-1-butanol |
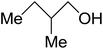 |
43 |
s |
s |
s |
i |
i |
s |
tert-Pentylalcohol |
 |
20 |
s |
s |
s |
s |
s |
s |
1-Hexanol |
 |
60 |
s |
s |
s |
s |
s |
s |
With respect to base oil solubility, alcohols ≤C4 would have limited use for degreasing as most bearing greases (NLGI2) are manufactured from PAO-10 or mineral oil range viscosities.29 Although tert-butanol can dissolve hydrocarbon at this level, tert-butanol and tert-pentanol have much greater risk of fire, since their flash points (<20 °C) are less than half of their normal isomers. The data show a trend in that alcohols with tertiary structure provide best hydrocarbon solubility among isomers but that branching also tends to decrease flash point. Clearly, 1-hexanol has the highest flash point of all the alcohols tested owing to unbranched structure and molecular weight and that its branched isomers were not necessary to achieve powerful hydrocarbon solubility. Unfortunately, none of the alcohols could be used in unblended form for degreasing under military regulations because of their low flash points (<61 °C).30
We noted that isoamyl alcohol had modest non-polar character to dissolve up to PAO-10 and mineral oil. It was presumed that its carbonate ester would have even better hydrocarbon oil solubility by masking the polar alcohol group and increasing aliphatic content.31,32 Furthermore, flash point would be increased by roughly doubling molecular weight. Most isoamyl alcohol is manufactured commercially by chlorination of isopentane followed by hydrolysis.33,34 However, isoamyl alcohol is also a natural product and is the major constituent (∼75%) of fusel oil, which are higher boiling alcohols (≤C5) remaining after distillation of ethanol from fermented vegetable matter.35–37 Carbonate esters of isoamyl alcohol have been previously prepared such as its reaction in supercritical carbon dioxide with n-butyl bromide and 1,8-diazabicycloundecene as base to form isoamyl n-butyl carbonate.38 However, dimethyl carbonate (DMC) has become a widely available solvent/reagent that can serve as a synthetic equivalent of carbon dioxide.39,40 The mixed isoamyl methyl carbonate, for nail polish removal,41 was made by potassium carbonate catalyzed reaction of isoamyl alcohol with excess DMC.42 Dialkyl carbonates have also been made by transesterification of DMC using di-n-butyltin oxide, however we wanted to avoid this toxic catalyst which oftentimes contaminates products.43,44
Synthesis of difusel carbonate
Fusel oil contains water (∼15 wt%) that would likely complicate further organic reactions, especially esterifications, when using it as a starting material. Webb et al. reported an involved fusel oil drying process with multiple charges of anhydrous magnesium sulfate over several weeks.45 Instead, the crude fusel oil could be dried sufficiently and immediately by first washing with brine followed by a single treatment with anhydrous magnesium sulfate (6 wt% loading). The proton NMR spectrum of the dry fusel oil, which dissolved completely in deuterochloroform, had a perfect 2
:
1 integral ratio of the OC
2 peaks (3.69–3.33 ppm) compared to the alcohol peak (2.2 ppm), respectively. Afterwards, the DFC was easily prepared in 69% yield by transesterification of DMC with dry fusel oil in a molar ratio of 1
:
2, respectively. The reaction was catalyzed by the Lewis acid tetraisopropyl orthotitanate (0.2 wt% loading) based on a method reported in the patent literature, Scheme 1.46,47 Careful distillation to remove the low boiling, by-product methanol steered the equilibrium by Le Châtelier's principle in favor of the higher carbonate.48 Some unreacted isoamyl alcohol was next distilled away and finally the DFC was collected without any fractionation. When attempting the reaction on fusel oil as received, there was an immediate precipitate upon addition of the catalyst, presumably from hydrolysis of the latter.
 |
| Scheme 1 Synthesis of difusel carbonate (DFC) from dimethyl carbonate. | |
The proton NMR spectrum of DFC was very similar to that of fusel oil although the OC
2 resonances were shifted downfield by ∼0.5 ppm and the alcohol peak was no longer present, Fig. 1. Integration of the OC
2 resonances showed that DFC was >85% diisoamyl carbonate. The complex multiplet centered at 3.95 ppm was the characteristic splitting pattern from esters of the chiral 2-methylbutanol, the so-called active amyl alcohol and second most abundant alcohol in fusel oil. The balance of the DFC was most likely the statistically favored isoamyl 2-methylbutyl carbonate and perhaps di(2-methylbutyl) carbonate. The carbon-13 NMR spectrum confirmed the presence of two carbonates (155.46 and 155.35 ppm) and the four isoamyl ester carbons signals (66.37, 37.28, 24.70, and 22.31 ppm) appeared with much greater intensity than the five 2-methylbutyl ester carbon signals (72.39, 34.13, 25.74, 16.09 and 11.05 ppm), Fig. 1. There are several reports indicating the challenging nature of gas chromatographic (GC) separation of mixtures of isoamyl and 2-methylbutyl alcohols that it was no surprise that DFC appeared to be essentially a single component with traces of butyl pentyl carbonate isomers by GC-mass spectrometry (GC/MS) analysis.49 Thus, the quantification of the dipentyl carbonate isomers by GC/MS would require extensive method development and/or specialized columns. The dipentyl carbonate would not survive electron impact ionization, but with chemical ionization the protonated molecular ion ([M + H]+ = 203) was observed. Additional concerns were that tetrafusel orthotitanates, formed by transesterification of the catalyst, may have co-distilled with the DFC product.50,51 However, DFC made by the above method had total titanium ion less than the limits of measurement by inductively coupled plasma atomic emission spectrometry (ICP AES)52 and furthermore, elemental microanalyses (C, H) were within accepted margins of error. To eliminate this potential contamination issue entirely, DFC was prepared equally well by a procedure similar to the above only switching to potassium hydroxide (0.2 wt% loading) as catalyst instead.53,54
 |
| Fig. 1 1H (top) and 13C (bottom) NMR spectra of difusel carbonate in deuterochloroform at room temperature. | |
Solubility of hydrocarbon base oils and lubricants in difusel carbonate (DFC)
The empirical solubility of the hydrocarbon base oils in DFC as well as three related dialkyl carbonate solvents (DMC, diethyl carbonate (DEC) and 1,2-propylene carbonate (PC)) were determined, Table 2. It was anticipated that DMC would be have a little more non-polar character, but like its parent methanol was not capable of dissolving any of the hydrocarbons oils tested. PC is a widely used carbonate solvent with a high dielectric constant and not surprisingly had no ability to dissolve any of the hydrocarbon oils. DEC showed a modest increase in non-polar character dissolving PAO-2, PAO-5 and mineral oil, which was a significant improvement over ethanol that could only dissolve PAO-2. DFC was able to dissolve all of the hydrocarbons tested in contrast to isoamyl alcohol and 2-methyl-1-butanol which could dissolve up to PAO-10 and mineral oil. Regardless of their hydrocarbon solubilities, DMC and DEC would have limited use in neat forms for degreasing purposes because of their low flash points (<35 °C) and risk for fire, Table 2. DFC had a flash point of 93 °C which was more than twice as high as the parent isoamyl alcohol (43 °C). Under the new regulations, the flash point for DFC places it right at the upper cutoff for a category 4 flammable liquids (flash point 60–93 °C), which permits double the storage capacity in metal lockers as compared to category 3 flammable liquids (flash point 23–60 °C) such as isoamyl alcohol.55
Table 2 Solubility of hydrocarbon base oil in dialkyl carbonates;a flash points of the carbonate solvents
Solventb |
Flash pointc (°C) |
2 |
5 |
PAOd 10 |
65 |
150 |
Mineral oil |
s = soluble, i = insoluble. Abbreviations: PC = 1,2-propylene carbonate, DMC = dimethylcarbonate, DEC = diethyl carbonate and DFC = difusel carbonate. Safety data sheets. mm2 s−1 @ 100 °C. This work. |
PC |
132 |
i |
i |
i |
i |
i |
i |
DMC |
17 |
i |
i |
i |
i |
i |
i |
DEC |
33 |
s |
s |
i |
i |
i |
s |
DFC |
93e |
s |
s |
s |
s |
s |
s |
Some further solubility experiments were made with DFC and isoamyl alcohol against a selection of typical lubricant classes common to the triservices, Table 3. Isoamyl alcohol had surprisingly good solubility for nearly all of these lubricants with base oil viscosities ranging from 5–18.5 mm2 s−1 (100 °C). However, isoamyl alcohol was unable to dissolve the higher viscosity gear oil (35 mm2 s−1 @ 100 °C). In contrast, DFC was able to dissolve all of the lubricants evaluated. As mentioned previously, ethyl lactate has received a great deal of attention as a renewable cleaning solvent meeting many of the tenants of green chemistry.56 However, ethyl lactate has a relatively low flash point (46 °C) and although we found ethyl lactate could dissolve hexanes, it could not dissolve any of the hydrocarbon base oils, nor any of the lubricants evaluated in this study.
Table 3 Solubilitya of general machine shop lubricants in isoamyl alcohol and difusel carbonate
Solvent |
Diesel engine oil (Rotella T®) |
10 W 40 motor oil |
ATFb |
Gear oil |
Multipurpose grease (Nyco GN 22 grease®) |
Metal working fluid (Tonna S220®) |
s = soluble; i = insoluble. Automatic transmission fluid. |
Isoamyl alcohol |
s |
s |
s |
i |
s |
s |
Difusel carbonate |
s |
s |
s |
s |
s |
s |
Isoamyl alcohol has a strong odor that most might find objectionable and fusel oil is still worse in its odor characteristics. In fact the word fusel is German and roughly translates as ‘bad liquor’ owing to the odor imparted to spirits by the higher alcohols. The off-putting odor is likely due to traces of organoamine and organosulfur compounds which become concentrated in the fusel oil after ethanol distillation. Unfortunately, short-path distillation in the purification of DFC did not remove this smell which was similar to crude fusel oil. Flammability aside, using either fusel oil or DFC directly in metal cleaning operations would certainly not be permissible on account of these odor properties. However, diisoamyl carbonate made by the described procedure starting with high purity, isoamyl alcohol (99%) had a very weak odor which was pleasant and fruity.57
Diesel fuel properties of difusel carbonate (DFC)
Oxygenated fuels have been studied as motor fuel components in both spark58 and compression ignition engines.59 DMC and other dialkyl carbonates have been shown to decrease particle matter (PM) in diesel exhaust.60,61 Identifying multiple uses for a degreasing solvent could be important to keep overall costs down and because the military is a major consumer of diesel fuels, the basic fuel properties of DFC were analyzed such as melting point, viscosity, density, net heating value and derived cetane number.62 These data were compared with literature values of these properties for DMC and diesel #2, Table 4.63–65
Table 4 Basic diesel fuel properties of dimethyl and difusel carbonates and diesel #2
Fuel |
Density @ 15 °C (g mL−1) |
Kinematic viscosity @ 40 °C (mm2 s−1) |
Net heating value (MJ kg−1) |
Cetane number |
Melting point (°C) |
Ref. 63. Not determined. Ref. 64. Ref. 65. Data from safety data sheet. Ref. 62. |
Diesel #2 |
0.85a |
1.9–4.1a |
42.64a |
≥40a |
Ndb |
Dimethyl carbonate |
1.07 (20 °C)c |
0.63 (20 °C)c |
15.78c |
35d |
2e |
Difusel carbonate |
0.91 |
2.02 |
30.46 |
22.8f |
<−80 |
The melting/freezing point of DFC must be lower than −80 °C since no phase changes were observed by differential scanning calorimetry down to or up from this temperature. Although melting point is not usually measured for diesel fuel, the low melting point of DFC indicates it would likely have good cold flow properties, obviously much better than DMC which solidifies at just 2 °C. The kinematic viscosity of DFC (2.02 m2 s−1) was within the specified range of diesel #2 (1.9–4.1 m2 s−1), and the density of DFC (0.91 g mL−1) was only slightly higher than diesel #2 (0.85 g mL−1). With all of the extra hydrocarbon content, the net heating value of DFC (30.47 MJ kg−1) was about twice that of DMC (15.78 MJ kg−1) but was 30% less than the cutoff for diesel #2 (42.64 MJ kg−1). The interest in DMC as a diesel fuel stems in part from its cetane number of 35 which is very close to the U.S. minimum of 40 for diesel #2.65,66 Unfortunately, combustion of DFC under compression ignition conditions62 gave a derived cetane number of only 22.8. The ignition delay for DFC was very long (10.9 ms) so obvious knocking and poor performance of a diesel motor would occur if DFC were used as a single-component fuel.67 The two branching points in DFC could be a contributing factor of poor combustion by increasing ignition delay as Hellier et al. described in diesel alkanes.68 We found that DFC was easily soluble in diesel #2, 1
:
5 part ratio and vice versa, which implies that DFC would be perfectly soluble in diesel #2 at the small percentage blends (10 wt%) at which DMC was shown to decrease PM emissions by 35%.69 DFC was also soluble in gasoline but further study of DFC as an additive in gasoline and diesel fuels will be reported in a future communication.
Conclusions
Often thought of as polar molecules and solvents, alcohols have non-polar character depending on their hydrocarbon content and structure. The non-polar character of simple alcohols (≤C6) could be empirically graded by their solvency of a range of PAO viscosities. Branching tended to increase hydrocarbon solubility among the alcohols but also increased flammability. 1-Hexanol was the threshold for strong, non-polar character among primary alcohols dissolving high viscosity PAO. The bioderived isoamyl alcohol and 2-methylbutanol from fusel oil had modest non-polar character and could dissolve up to PAO (10 mm2 s−1 @ 100 °C).
Fusel oil was easy to dry for the successful preparation of difusel carbonate (DFC) by transesterification of dimethyl carbonate. DFC was an excellent solvent for non-polar substances and could be a suitable degreasing solvent since it easily cleaned PAO-150, mineral oil and a variety of industrial lubricants. The flash point of DFC (93 °C) was over twice as high as isoamyl alcohol for a wider safety margin. The low cetane number of 22.8 means DFC cannot be used as diesel fuel without blending into petro- or bio-diesel. The study showed that fusel oil and its carbonate ester could be useful and sustainable degreasing solvents in certain applications.
Conflicts of interest
There are no conflicts to declare.
Acknowledgements
This work was supported in part by a grant (WP-2523) from the Strategic and Environmental Research and Development Program (SERDP). Additional funding came from the In-house Laboratory Independent Research program of the Office of Naval Research. Thanks to Jose Leboriero Hernandez and Sean Miller of the Archer Daniels Midland Company for helpful discussions about fusel oil and a generous sample. Thanks to Lawrence Baldwin (China Lake) for helpful advice about the NMR analyses. Thanks to Michelle Wade and Tom Czibovich (China Lake) for their assistance obtaining the analyses, reagents and supplies. Thanks to the staff of our Technical Library (China Lake) who collected several literature citations. Thanks to ExxonMobil Chemical Company for providing the polyalphaolefins. Mention of trade names or commercial products in this publication is solely for the purpose of providing specific information and does not imply recommendation or endorsement by the U.S. Department of the Navy.
References
- C. F. Mabery, Lubrication and lubricants, Ind. Eng. Chem., 1910, 2, 115–123, DOI:10.1021/ie50016a001.
- Chemistry and Technology of Lubricants, ed. R. M. Mortier, M. F. Fox, and S. T. Orszulik, Springer, New York, 3rd edn, 2010, DOI:10.1007/978-1-4020-8662-5.
- L. Archbutt, and R. M. Deeley, Lubrication and Lubricants, A Treatise on the Theory and Practice of Lubrication and on the Nature, Properties, and Testing of Lubricants, ed. J. B. Lippincott, Philadelphia, 2nd edn, 1907, ch. 1, pp. 1–14 Search PubMed.
- H. K. D. H. Bhadeshia, Steels for bearings, Prog. Mater. Sci., 2012, 57, 268–435, DOI:10.1016/j.pmatsci.2011.06.002.
- R. Benda, J. Bullen and A. Plomer, Synthetics basics: polyalphaolefins — base fluids for high-performance lubricants, J. Synth. Lubr., 1996, 13, 41–56, DOI:10.1002/jsl.3000130105.
- L. R. Rudnick, in Synthetics, Mineral Oils and Bio-Based Fluids, ed. L. R. Rudnick, Marcel Dekker, New York, 2005, ch. 1 Search PubMed.
- C. J. Donahue, Lubricating grease: a chemical primer, J. Chem. Educ., 2006, 83, 862–869, DOI:10.1021/ed083p862.
- D. J. Press, M. McKinley, D. Deapen, C. A. Clark and S. L. Gomez, Residential cancer cluster investigation nearby a Superfund Study Area with trichloroethylene contamination, Cancer Causes Control, 2016, 27, 607–613, DOI:10.1007/s10552-016-0734-5.
- Regulation of Certain Uses under Toxic Substances Control Act: Trichloroethylene; Vapor Degreasing, 40 CFR Part 751, Jan 19, 2017.
- United States Department of Agriculture, Biopreferred® program, https://www.biopreferred.gov/BioPreferred/faces/pages/AboutBioPreferred.xhtml, accessed Jan 31, 2019 Search PubMed.
- The Agricultural Act of 2014, H. R. 2642, Pub. L. 113–79, Jan 3, 2014.
- Presidential Green Chemistry Challenge Award Recipients 1996–2016, Green Chemistry Challenge, United States Environmental Protection Agency, p.100, https://www.epa.gov/sites/production/files/2016-10/documents/award_recipients_1996_2016.pdf, accessed 22 July 2019 Search PubMed.
- C. S. M. Pereira, V. M. T. M. Silva and A. E. Rodrigues, Ethyl lactate as a solvent: properties, applications and production processes–a review, Green Chem., 2011, 13, 2658–2671, 10.1039/C1GC15523G.
- R. Zhao and H. Cabezas, Molecular thermodynamics in the design of substitute solvents, Ind. Eng. Chem. Res., 1998, 37, 3268–3280, DOI:10.1021/ie970861p.
- C. Trevizo, D. Daniel and N. Nirmalakhandan, Screening alternative degreasing solvents using multivariate analysis, Environ. Sci. Technol., 2000, 34, 2587–2595, DOI:10.1021/es9912832.
- M. W. Jackson and J. S. Drury, Miscibility of organic solvent pairs, Ind. Eng. Chem., 1959, 51, 1491–1493, DOI:10.1021/ie50600a039.
- H. C. Holden and A. K. Doolittle, Solvents, Ind. Eng. Chem., 1935, 27, 525–530, DOI:10.1021/ie50305a008.
- C. Cowan-Ellsberry, S. Belanger, P. Dorn, S. Dyer, D. McAvoy, H. Sanderson, D. Versteeg, D. Ferrer and K. Stanton, Environmental safety of the use of major surfactant classes in North America, Crit. Rev. Environ. Sci. Technol., 2014, 44, 1893–1993, DOI:10.1080/10739149.2013.803777.
- SIDS Initial Assessment Report for SIAM 22 for Long-Chain Alcohol (Tome 1 and 2), the Organization for Economic Co-Operation and Development (OECD) 2006, retrieved from http://webnet.oecd.org, accessed Feb 2, 2019.
- D. D. Vaishnav, R. S. Boethling and L. Babeu, Quantitative structure–biodegradability relationships for alcohols, ketones and alicyclic compounds, Chemosphere, 1987, 16, 695–703, DOI:10.1016/0045-6535(87)90005-1.
- European Chemical Agency (ECHA), Diethyl Carbonate, https://echa.europa.eu/registration-dossier/-/registered-dossier/7106/5/3/2, accessed Feb 6, 2019 Search PubMed.
- Y. Hirose, M. Ogawa and Y. Kusuda, Constituents of fusel oils obtained through the fermentation of corn, barley and sweet molasses, Agric. Biol. Chem., 1962, 26, 526–531, DOI:10.1080/00021369.1962.10858005.
- M. C. Ferreira, A. J. A. Meirelles and E. A. C. Batista, Study of the fusel oil distillation process, Ind. Eng. Chem. Res., 2013, 52, 2336–2351, DOI:10.1021/ie300665z.
- G. S. Settles, Schlieren and Shadowgraph Techniques: Visualization Phenomenoa in Transparent Media, Springer-Verlag, Heidelberg, 1st edn, 2001, DOI:10.1007/978-3-642-56640-0.
- V. Rupp, and K. Surprenant, in ASM Handbook, Surface Engineering, ed. C. M. Cotell, J. A. Sprague, and F. A. Smidt Jr, ASM International, 1994, vol. 5, pp. 21–32, DOI:10.31399/asm.hb.
- Solubility Data Series, Alcohols with water, International Union of Pure and Applied Chemistry, ed. A. F. M. Barton, Pergamon Press, New York, 1984, vol. 15, p. 29, DOI:10.1016/C2013-0-03309-9.
- B. Y. McCarty, and W. E. Skelton, Removal of wax from hydrocarbon oil, US Pat., 1998398, The Texas Company, 1935.
- J. T. Carey, A. S. Galiano-Roth, and M. Wu, High viscosity metallocene catalyst PAO novel base stock lubricant blends, US Pat., 8535514, ExxonMobil Research and Engineering Company, 2013.
- E. Casserly, T. Langlais, S. P. Springer, A. Kumar, and B. Mallory, The effect of base oils on thickening and physical properties of lubricating greases, Lube Magazine, no. 144, April 2018, pp. 32–37 Search PubMed.
- MIL-PRF-680C, Performance Specification Degreasing Solvent, 25 March 2010 Search PubMed.
- A. A. G. Shaikh and S. Sivaram, Organic carbonates, Chem. Rev., 1996, 96, 951–976, DOI:10.1021/cr950067i.
- B. Schäffner, F. Schäffner, S. P. Verevkin and A. Börner, Organic carbonates as solvents in synthesis and catalysis, Chem. Rev., 2010, 110, 4554–4581, DOI:10.1021/cr900393d.
- H. Essex, H. Hibbert and B. T. Brooks, The hydrolysis of chloropentanes as affected by high pressure: synthetic fusel oil, J. Am. Chem. Soc., 1916, 38, 1368–1374, DOI:10.1021/ja02264a016.
- M. M. Wilson and F. J. Worster, Place of synthetic amyl products among lacquer solvents, Ind. Eng. Chem., 1929, 21, 592–594, DOI:10.1021/ie50234a021.
- R. C. Schupphaus, On the alcohols of fusel oil, J. Am. Chem. Soc., 1892, 14, 45–60, DOI:10.1021/ja02123a005.
- G. G. Stewart, The production of secondary metabolites with flavour potential during brewing and distilling wort fermentation, Fermentation, 2017, 3, 63, DOI:10.3390/fermentation3040063.
- L. A. Hazelwood, J. M. Daran, A. J. A. van Maris, J. T. Pronk and J. R. Dickinson, The Ehrlich pathway for fusel alcohol production: a century of a century of research on Saccharomyces cerevisiae metabolism, Appl. Environ. Microbiol., 2008, 74, 2259–2266, DOI:10.1128/AEM.02625-07.
- F. S. Pereira, L. J. Pereira, D. F. A. Crédito, L. H. V. Girão, A. H. S. Idehara and E. R. P. González, Cycling of waste fusel alcohols from sugar cane industries using supercritical carbon dioxide, RSC Adv., 2015, 5, 81515–81522, 10.1039/c5ra16346c.
- P. Tundo, M. Musolino and F. Aricò, The reactions of dimethyl carbonate and its derivatives, Green Chem., 2018, 20, 28–85, 10.1039/c7gc01764b.
- M. A. Pacheco and C. L. Marshall, Review of dimethyl carbonate (DMC) manufacture and its characteristics as a fuel additive, Energy Fuels, 1997, 11, 2–29, DOI:10.1021/ef9600974.
- A. Deswartvaegher, B. Forestier, S. Miard, J. P. Senet, S. Thiebaud-Roux, D. Cristea, P. de Caro, G. Giacinti, and M. Bandres, Varnish composition based on a solvent exclusively of vegetable origin, IPN WO 2006056558, Durlin France, 2006.
- M. Bandres, P. de Caro, S. Thiebaud-Roux and M. E. Borredon, Green synthesis of biobased solvents, Compt. Rendus Chem., 2011, 14, 636–646, DOI:10.1016/j.crci.2010.07.008.
- J. A. Kenar, G. Knothe and A. L. Copes, Synthesis and characterization of dialkyl carbonates prepared from mid-, long-chain, and Guerbet alcohols, J. Am. Oil Chem. Soc., 2004, 81, 285–291, DOI:10.1007/s11746-004-0897-4.
- A. A. Shaikh and S. Sivaram, Dialkyl and diaryl carbonates by carbonate interchange reaction with dimethyl carbonate, Ind. Eng. Chem. Res., 1992, 31, 1167–1170, DOI:10.1021/ie00004a028.
- A. D. Webb, R. E. Kepner and R. M. Ikeda, Composition of typical grape brandy fusel oil, Anal. Chem., 1952, 24, 1944–1949, DOI:10.1021/ac60072a020.
- H. Urabe, and F. Sato, in Lewis Acids in Organic Synthesis, ed. H. Yamamoto, Wiley-VHC, Weinheim, 2000, vol. 2, ch. 15, p. 653, DOI:10.1002/9783527618309.ch15.
- Decarboxylation des carbonates d’alcoyle en ethers alcoyliques, Be. Pat., 1006039 (A3), Enichem Synthesis S.P.A, 1994.
- R. S. Treptow, Le Châtelier’s principle: a reexamination and method of graphic illustration, J. Chem. Educ., 1980, 57, 417, DOI:10.1021/ed057p417.
- P. J. Porcaro and V. D. Johnston, Primary amyl alcohols determined by gas chromatography, Anal. Chem., 1961, 33, 361–362, DOI:10.1021/ac60171a015.
- R. J. Speer and D. R. Carmody, Organic compounds of titanium. Tetraalkyl orthotitanates, new waterproofing agents, Ind. Eng. Chem., 1950, 42, 251–253, DOI:10.1021/ie50482a017.
- D. C. Bradley, R. C. Mehrotra and W. Wardlaw, Structural chemistry of alkoxides. I. Amyloxides of silicon, titanium, and zirconium, J. Chem. Soc., 1952, 2027–2032, 10.1039/jr9520002027.
- E. J. Dos Santos, M. P. Dos Santos, A. B. Hermann and R. E. Sturgeon, Rapid determination of Ti in TiO2 by ICP OES, Anal. Methods, 2016, 8, 6463–6467, 10.1039/c6ay01842d.
- J. G. Kim, J. Y. Jeon, J. Chun, C. S. Kim, P. C. Lee and B. Y. Lee, Efficient synthesis of organic carbonates and poly(1,4-butylene carbonate-co-terephthalate)s, J. Appl. Polym. Sci., 2017, 134, 44951, DOI:10.1002/app.44951.
- G. Bellussi, M. Notari, E. M. Ebesco, M. C. Sevarese, P. Scorletti, D. Collia, and L. P. Serbolisca, Gas oil composition comprising dialkyl carbonate from bioalcohol, IPN WO 2011/045657 A1, Eni S.P.A, 2011.
- Flammable Liquids, 29 CFR 1910.106, Occupational Safety and Health Standards, United States Department of Labor, 28 December 2018.
- J. Van, Scientists hope to shuck old solvents, Chicago Tribune, 25 March 1998, http://www.chicagotribune.com/news/ct-xpm-1998-03-25-9803250036-story.html, accessed Feb 5, 2019 Search PubMed.
- T. Uehara, H. Ishihara, and S. Tanaka, Alkyl cycloheptylmethyl carbonates and perfume compositions, Eur. Pat., 1698684 A1, Kao Corp, 2005.
- O. I. Awad, R. Mamat, T. K. Ibrahim, A. T. Hammid, I. M. Yusri, M. A. Hamidi, A. M. Humada and A. F. Yusop, Overview of the oxygenated fuels in spark ignition engine: environmental and performance, Renewable Sustainable Energy Rev., 2018, 91, 394–408, DOI:10.1016/j.rser.2018.03.107.
- E. Kurtz, D. Kuhel, J. E. Anderson and S. A. A. Mueller, A comparison of combustion and emissions of diesel fuels and oxygenated fuels in a modern DI diesel engine, SAE Int. J. Fuels Lubr., 2012, 5, 1199–1215, DOI:10.4271/2012-01-1695.
- P. Rounce, A. Tsolakis, P. Leung and A. P. E. York, A comparison of diesel and biodiesel emissions using dimethyl carbonate as an oxygenated additive, Energy Fuels, 2010, 24, 4812–4819, DOI:10.1021/ef100103z.
- A. Arteconi, A. Mazzarini and G. Di Nicola, Emissions from ethers and organic carbonate fuel additives: a review, Water, Air, Soil Pollut., 2011, 221, 405–423, DOI:10.1007/s11270-011-0804-y.
- ASTM D6890-16e2, Standard test method for determination of ignition delay and derived cetane number (DCN) of diesel fuel oils by combustion in a constant chamber, ASTM International, West Conshohocken, Pennsylvania, 2018 Search PubMed.
- J. Bacha, J. Freel, A. Gibbs, L. Gibbs, G. Hemighaus, K. Hoekman, A. Gibbs, M. Ingham, L. Jossens, D. Kohler, D. Lesnini, J. McGeehan, M. Nikanjam, E. Olsen, R. Organ, B. Scott, M. Sztenderowicz, A. Tiedemann, C. Walker, J. Lind, J. Jones, D. Scott, and J. Mills, Diesel fuels technical review, Chevron Corporation, 2007, https://www.chevron.com/-/media/chevron/operations/documents/diesel-fuel-tech-review.pdf, accessed Aug 16, 2018 Search PubMed.
- A. O. G. Abdalla and D. Liu, Dimethyl carbonate as a promising oxygenated fuel for combustion: a review, Energies, 2018, 11, 1552, DOI:10.3390/en11061552.
- T. D. Durbin, G. Karavalakis, K. C. Johnson, D. R. Cocker, J. Yang, Y. Jiang, and S. Kumar, Evaluating the viability of dimethyl carbonate as an alternative fuel for the transportation sector; final report to the National Center for Sustainable Transportation, no. NCST-201706, 2017 Search PubMed.
- ASTM D613-18 Standard test method for cetane number of diesel fuel oil, ASTM International, West Conshohocken, Pennsylvania, 2018 Search PubMed.
- P. Hellier, N. Ladommatos, R. Allan, M. Payne and J. Rogerson, Influence of carbonate ester molecular structure on compression ignition combustion and emissions, Energy Fuels, 2013, 27, 5222–5245, DOI:10.1021/ef401415j.
- P. Hellier, N. Ladommatos, R. Allan, M. Payne and J. Rogerson, The impact of saturated and unsaturated fuel molecules on diesel combustion and exhaust emissions, SAE Int. J. Fuels Lubr., 2011, 5, 106–122, DOI:10.4271/2011-01-1922.
- T. Murayama, M. Zheng, T. Chikahisa, Y. Oh, S. Fujiwara, S. Tosaka, M. Yamashita and H. Yoshitake, Simultaneous Reductions of Smoke and NOx from a DI Diesel Engine with EGR and Dimethyl Carbonate, SAE Tech. Pap. Ser., 1995 DOI:10.4271/952518.
Footnote |
† Electronic supplementary information (ESI) available. See DOI: 10.1039/c9ra04220b |
|
This journal is © The Royal Society of Chemistry 2019 |
Click here to see how this site uses Cookies. View our privacy policy here.