DOI:
10.1039/C9RA03774H
(Paper)
RSC Adv., 2019,
9, 16418-16422
Highly α-position regioselective ring-opening of epoxides catalyzed by halohydrin dehalogenase from Ilumatobacter coccineus: a biocatalytic approach to 2-azido-2-aryl-1-ols†
Received
20th May 2019
, Accepted 20th May 2019
First published on 24th May 2019
Abstract
Halohydrin dehalogenases are usually recognized as strict β-position regioselective enzymes in the nucleophile-mediated ring-opening of epoxides. Here we found the HheG from Ilumatobacter coccineus exhibited excellent α-position regioselectivity in the azide-mediated ring-opening of styrene oxide derivatives 1a–1k, producing the corresponding 2-azido-2-aryl-1-ols 2a–2k with the yields up to 96%.
Introduction
1,2-Azido alcohols are versatile precursors in organic synthesis for a wide variety of target compounds such as triazoles,1 triazole-fused dihydrooxazinones,2 2-oxazolidinones,3 1,4-oxazepines and 1,3-oxazines,4 oxazaborolidines,5 and 1,3-oxazolidines.6 More importantly, they have been widely employed for the regioselective preparation of 1,2-amino alcohols and highly oxygenated compounds such as carbohydrates and nucleosides.7
The epoxy group is a highly reactive moiety, which makes it easily react with a broad range of nucleophiles and provides a convenient access to diverse highly functionalized molecules.8 Thus among the transformations used for the preparation of 1,2-azido alcohols, the epoxide ring-opening is straightforward and effective method which has been widely studied. Though trimethylsilyl azide and other metal azides have been used to introduce the azide group,9 the sodium azide is generally employed under various reaction conditions.10
Halohydrin dehalogenases (HHDHs) are valuable enzymes from short dehydrogenases/reductases superfamily, which exhibit promiscuous catalytic functions in the synthesis and conversion of epoxides.11 HHDHs are not only able to catalyze the dehalogenation of vicinal haloalcohols to produce epoxides, but also can accept several anionic nucleophiles in epoxide ring-opening reactions with the formation of a broad range of β-functionalized alcohols, which are useful biocatalytic routes to prepare some important pharmaceuticals and biologically active compounds.12 Azide can be processed by HHDHs as one of the nucleophiles to produce β-azido alcohols. However, all the reported HHDHs have been described to transform the azide group to the epoxides at β-position (Scheme 1, red), generating the 2-azido-1-phenylethan-1-ols (2′).12a,12h,12j In this study, we explored the HheG from I. coccineus for highly α-position regioselective ring-opening of styrene oxide derivatives 1 with azide (Scheme 1, blue), which gave the 2-azido-2-aryl-1-ols (2) with the yields up to 96%.
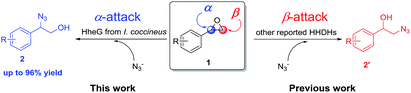 |
| Scheme 1 HHDH-catalyzed α-position and β-position regioselective ring-opening of epoxides with azide. | |
Results and discussion
Recently Schallmey and coworkers have reported that the HHDH from I. coccineus, namely HheG, exhibited significant activity in the azidolysis of cyclohexene oxide and limonene oxide.13 Analysis of the HheG's structure revealed a large open cleft harboring the active site to accept these cyclic epoxide substrates, which was in sharp contrast to other known HHDH structures. Attracted by these results, we inferred the HheG might possess a special regioselectivity in the ring-opening process.
With this conjecture in mind, we first investigated the HheG-catalyzed ring-opening of styrene oxide 1a with azide on an analytical scale. The HheC-catalyzed and non-enzymatic reactions were also carried out as controls. The HPLC analysis results were depicted in Fig. 1. In the absence of enzyme, ring-opening of 1a preferred α-position regioselectivity (Fig. 1, pink line) and the reaction rate was pH-dependent.14 Previous study has revealed HheC was a R-enantioselective and β-regioselective enzyme for ring-opening of epoxides by several nucleophiles. HheC catalyzed azide ring-opening of 1a to produced 2-azido-1-phenylethan-1-ol (2a′) with 79% β-position regioselectivity and 98% ee (R).12a Herein, the azide was excessive and we found that the HheC showed about 100% conversion of 1a with about 50% β-position regioselectivity (Fig. 1, red line). Formation of 2-azido-2-phenylethan-1-ol (2a) might be contributed by the chemical reaction via α-position ring-opening of 1a. Interestingly, the HheG showed highly α-position regioselectivity in the ring-opening process (Fig. 1, blue line) without β-position selectivity, which was different from the other reported HHDH regioselectivity.
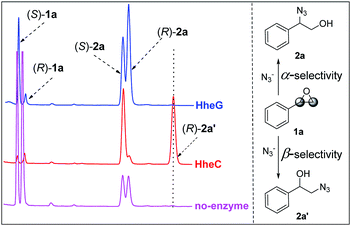 |
| Fig. 1 Ring-opening of 1a catalyzed by HheG (blue line), HheC (red line), and no enzyme (pink line). | |
Subsequently, we tested the conditions of the HheG-catalyzed azide-mediated ring-opening (ARO) reaction using epoxide 1a as the model substrate. All the reactions were performed on analytical scale, and the relative yields of 2a at different conditions were determined by HPLC analysis to screen the optimal conditions. First the reaction pH/buffer and temperature were examined and the results were summarized in Table 1. It could be found that the optimal pH/buffer was 7.0/PBS (entry 4). Reaction in 5.0/HAc–NaAc gave a very low yield (entry 1). Reaction in the higher pH/PBS caused a dramatic decrease of the yield (entries 5 and 6 vs. entry 4). A good yield was obtained in 8.0/Tris–H2SO4, which was dramatic higher than that obtained in 8.0/PBS (entry 6 vs. entry 8). Temperature study revealed the optimal reaction temperature was 30 °C (entry 4). An excellent yield was also obtained at 25 °C (entry 10). Increasing reaction temperature to 35–45 °C resulted in a sharp decrease of the yield (entries 11–13 vs. entry 4), which corresponded to the previous study of the thermostability of the HheG.15
Table 1 Investigation of the pH/buffer and temperature of ARO reaction
We further investigated the ratio of azide to substrate 1a. From the results of Table 2 (entries 1–4), we could find that the optimal ratio for the ARO reaction was 1.5
:
1 (entry 2). Increasing the ratio to 2
:
1 and 3
:
1 did not improve the yield (entries 3 and 4 vs. entry 2). The recombinant cell density was subsequent investigated at the ratio of 1.5
:
1 (entries 5–7). A higher yield was obtained by increasing cell density (entries 5–7 vs. 2). However, the yields generated by 15 and 20 g cdw L−1 of cells were almost equivalent (entry 6 vs. entry 7), and 15 g cdw L−1 of cells was selected for the ARO reaction in the following experiments.
Table 2 Investigation of the ratio of NaN3 to 1a and cell density of ARO reaction
After obtaining the optimum reaction conditions, the reaction courses at the substrate concentrations from 5 to 60 mM were investigated. The yields were analyzed by HPLC at 6, 12 and 24 h for each reaction. The highest yield was set to 100% to calculate the relative yields. The results depicted in Fig. 2 indicated the reactions at the substrate concentration of 5–40 mM were almost finished at 12 h. Prolonging the reaction time to 24 h did not caused notable increase in yields. For a higher substrate concentration of 60 mM, the yield obtained at 6 h was as same as that obtained from the lower concentrations. It should be noted that the yield didn't increase by extending reaction time, which might be caused by product inhibition at high substrate concentration. The ARO reaction was established to react on 40 mM of epoxides for 12 h.
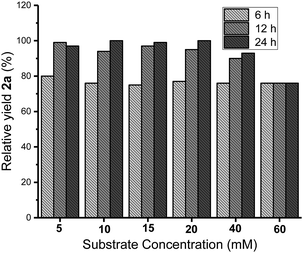 |
| Fig. 2 Investigation of ARO reaction courses at the substrate concentrations from 5 to 60 mM. | |
Finally, several structurally different aromatic epoxides 1a–1k were selected as substrates to investigate the substrate scope of the ARO reaction. All the reactions were carried out on the optimal conditions with 40 mM of epoxides 1. DMSO (1 vol%) was used as co-solvent to solubilize the epoxides in the aqueous reaction medium. From the results of Table 3, we could find that the HheG showed a broad substrate range in the ARO reaction. The 2-azido-2-aryl-1-ols 2a–2k were synthesized from the corresponding epoxides 1a–1k with the high yields up to 96%. The epoxide substrates with substituent group at p-position generated relative higher yield than that with substituent group at m-position (entries 6–9 vs. entries 2–5). The azidolysis of p-cyanophenyl-substituted epoxide 1j and p-tert-butyl-substituted epoxide 1k also gave the corresponding azidoalcohols with moderate yields of 65% and 76% respectively (entry 10 and entry 11). All the isolated yields were obtained from 40 mM of epoxides after reaction for 12 h, which pointed to a good enzyme activity in such biocatalytic ring-opening process. More importantly, the ARO reaction exhibited highly α-position regioselectivity in the ring-opening of epoxides 1a–1k, which indicated the HheG was an excellent α-position regioselective HHDH. To our knowledge, this was the first time that 2-azido-2-aryl-1-ols 2a–2k were synthesized by an enzymatic catalysis.
Table 3 Substrate scope of the ARO reaction
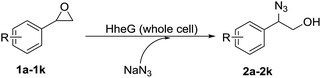
|
Entrya |
Substrate |
R |
Product |
Yieldb (%) |
Reaction condition: epoxide 1 conc. 40 mM in PBS (50 mM, pH 7.0), NaN3 conc. 60 mM, cells conc. 15 g cdw L−1, 30 °C, 12 h. Isolated yields. |
1 |
1a |
H |
2a |
96 |
2 |
1b |
m-F |
2b |
73 |
3 |
1c |
m-Cl |
2c |
69 |
4 |
1d |
m-Br |
2d |
66 |
5 |
1e |
m-CH3 |
2e |
76 |
6 |
1f |
p-F |
2f |
80 |
7 |
1g |
p-Cl |
2g |
91 |
8 |
1h |
p-Br |
2h |
76 |
9 |
1i |
p-CH3 |
2i |
95 |
10 |
1j |
p-CN |
2j |
65 |
11 |
1k |
p-C(CH3)3 |
2k |
76 |
Experimental
Strains culture and enzymes preparation
The HheC gene (GenBank accession number: AF397296.1) and HheG gene (GenBank accession number: AP012057.1, 3979394–3980182) were synthesized after codon optimization and ligated into pET-28a(+) plasmid using restricted enzymes NcoI and XhoI. The recombinant plasmid pET-28-HheC and pET-28-HheG were transformed into Escherichia coli BL21 (DE3), respectively. The kanamycin antibiotic was used in whole culture process to a final concentration of 50 μg mL−1.
A single recombinant E. coli colony was taken from the agar plate and inoculated in Luria-Bertani (LB) medium. The culture was grown overnight at 37 °C, 200 rpm, for 16 h. A large flask (1000 mL) containing 100 mL LB was inoculated with 5 mL of the overnight culture. The flask culture was grown overnight at 37 °C, 200 rpm, until the OD600 up to 0.6–0.8. Then, culture was induced with IPTG (final concentration 0.1 mM) and further shaken at 200 rpm, 28 °C, for 12–14 h. The cultures were centrifuged and harvested to obtain recombinant E. coli cells.
General procedure for the synthesis of 2a–2k
To a 20 mL suspension of 15 g cdw L−1 recombinant E. coli cells (HheG) in 50 mM PBS buffer (pH 7.0), NaN3 was added to a concentration of 60 mM. Then the epoxides 1 was added to a concentration of 40 mM using 200 μL of dimethyl sulfoxide (DMSO) as co-solvent. The mixture was stirred at 250 rpm and 30 °C. After reaction for 12 h, the mixture was extracted using ethyl acetate (2 × 20 mL). The organic phase was separated, dried over anhydrous Na2SO4, filtered, and concentrated by rotary evaporation. The residue was purified by flash column chromatography on silica gel (petroleum ether/ethyl acetate = 10
:
1–5
:
1) to afford the desired product 2.
2-Azido-2-phenylethanol (2a). Light yellow oil, 125 mg, 96% yield, 1H NMR (400 MHz, CDCl3) δ 7.44–7.31 (m, 5H), 4.68 (t, J = 6.4 Hz, 1H), 3.74 (t, J = 6.0 Hz, 2H), 2.24 (t, J = 6.1 Hz, 1H). 13C NMR (100 MHz, CDCl3) δ 136.3, 129.1, 128.8, 127.3, 68.0, 66.6.
2-Azido-2-(3-fluorophenyl)ethanol (2b). Light yellow oil, 82.9 mg, 73% yield, 1H NMR (400 MHz, CDCl3) δ 7.39–7.32 (m, 1H), 7.13–7.01 (m, 3H), 4.66 (dd, J = 7.9, 4.7 Hz, 1H), 3.81–3.66 (m, 2H), 2.57 (s, 1H); 13C NMR (100 MHz, CDCl3) δ 163.0 (d, J = 246.0 Hz), 138.9 (d, J = 7.0 Hz), 130.6 (d, J = 8.0 Hz), 122.9 (d, J = 3.0 Hz), 115.7 (d, J = 21.0 Hz), 114.2 (d, J = 22.0 Hz), 67.2 (d, J = 1.0 Hz), 66.4.
2-Azido-2-(3-chlorophenyl)ethanol (2c). Light yellow oil, 56 mg, 69% yield, 1H NMR (400 MHz, CDCl3) δ 7.33 (d, J = 4.9 Hz, 3H), 7.21–7.21 (m, 1H), 4.64 (dd, J = 7.7, 4.8 Hz, 1H), 3.74–3.70 (m, 2H), 2.30 (s, 1H); 13C NMR (100 MHz, CDCl3) δ 138.5, 134.9, 130.3, 129.0, 127.4, 125.4, 67.2, 66.5.
2-Azido-2-(3-bromophenyl)ethanol (2d). Light yellow oil, 128 mg, 66% yield, 1H NMR (400 MHz, CDCl3) δ 7.48–7.43 (m, 2H), 7.23 (dd, J = 4.4, 0.8 Hz, 2H), 4.60 (dd, J = 7.8, 4.7 Hz, 1H), 3.69 (qd, J = 11.6, 6.3 Hz, 2H), 2.68 (s, 1H); 13C NMR (100 MHz, CDCl3) δ 138.7, 131.8, 130.5, 130.2, 125.8, 123.0, 67.0, 66.3.
2-Azido-2-(3-methphenyl)ethanol (2e). Light yellow oil, 108 mg, 76%, yield, 1H NMR (400 MHz, CDCl3) δ 7.31–7.22 (m, 1H), 7.13 (m, 3H), 4.62 (t, J = 6.4 Hz, 1H), 3.72 (d, J = 6.5 Hz, 2H), 2.36 (s, 3H), 1.24 (s, 1H); 13C NMR (100 MHz, CDCl3) δ 138.9, 136.3, 129.6, 129.0, 127.9, 124.3, 68.0, 66.6, 21.6.
2-Azido-2-(4-fluorophenyl)ethanol (2f). Light yellow oil, 115 mg, 80% yield, 1H NMR (400 MHz, CDCl3) δ 7.31 (dd, J = 8.3, 5.4 Hz, 2H), 7.08 (t, J = 8.6 Hz, 2H), 4.68–4.61 (m, 1H), 3.80–3.65 (m, 2H), 2.32 (s, 1H); 13C NMR (100 MHz, CDCl3) δ 162.9 (d, J = 246.0 Hz), 132.3 (d, J = 4.0 Hz), 129.0 (d, J = 8.0 Hz), 116.0 (d, J = 22.0 Hz), 67.2, 66.5.
2-Azido-2-(4-chlorophenyl)ethanol (2g). White solid, 144 mg, 91% yield, 1H NMR (400 MHz, CDCl3) δ 7.35 (d, J = 8.4 Hz, 2H), 7.25 (d, J = 8.4 Hz, 2H), 4.62 (dd, J = 7.4, 5.1 Hz, 1H), 3.76–3.62 (m, 2H), 2.34 (s, 1H). 13C NMR (100 MHz, CDCl3) δ 134.9, 134.6, 129.2, 128.6, 67.1, 66.4.
2-Azido-2-(4-bromophenyl)ethanol (2h). Light yellow solid, 96 mg, 76% yield, 1H NMR (400 MHz, CDCl3) δ 7.53 (d, J = 8.3 Hz, 2H), 7.21 (d, J = 8.3 Hz, 2H), 4.64 (dd, J = 7.3, 5.1 Hz, 1H), 3.78–3.66 (m, 2H), 1.99 (s, 1H); 13C NMR (100 MHz, CDCl3) δ 135.5, 132.3, 129.0, 122.9, 67.3, 66.5.
2-Azido-2-(p-tolyl)ethanol (2i). Light yellow oil, 136 mg, 95% yield, 1H NMR (400 MHz, CDCl3) δ 7.28 (s, 4H), 4.70 (t, J = 6.4 Hz, 1H), 3.79 (d, J = 6.5 Hz, 2H), 2.62 (s, 1H), 2.43 (s, 3H); 13C NMR (100 MHz, CDCl3) δ 138.7, 133.7, 129.7, 127.2, 67.7, 66.47, 21.2.
2-Azido-2-(4-cyanophenyl)ethanol (2j). Light yellow oil, 90 mg, 65% yield, 1H NMR (400 MHz, CDCl3) δ 7.71–7.69 (m, 2H), 7.48–7.46 (m, 2H), 4.74 (dd, J = 7.7, 4.5 Hz, 1H), 3.77 (dq, J = 19.3, 11.5, 6.1 Hz, 2H), 1.79 (s, 1H); 13C NMR (100 MHz, CDCl3) δ 138.7, 133.7, 129.7, 127.2, 67.7, 66.4, 21.2.
2-Azido-2-(4-(tert-butyl)phenyl)ethanol (2k). Light yellow solid, 100 mg, 76% yield, 1H NMR (400 MHz, CDCl3) δ 7.41 (d, J = 8.3 Hz, 2H), 7.25 (d, J = 8.3 Hz, 2H), 4.64 (t, J = 6.4 Hz, 1H), 3.73 (d, J = 6.4 Hz, 2H), 2.56 (s, 9H), 1.32 (s, 9H); 13C NMR (100 MHz, CDCl3) δ 151.8, 133.3, 126.9, 125.9, 67.7, 66.5, 34.7, 31.4.
Conclusions
In summary, we found the HheG exhibited highly α-position regioselectivity for ring-opening of styrene oxide derivatives with azide and developed a biocatalytic approach to 2-azido-2-aryl-1-ols 2a–2k with good yields. Our results brought a complementary regioselective biotransformation for the HHDH-catalyzed ring-opening of epoxides. We expect that it would broaden the product spectrum of β-functional alcohols synthesized by HHDHs.
Conflicts of interest
There are no conflicts of interest to declare.
Acknowledgements
We are grateful for financial support from the National Natural Science Foundation of China (No. 21662050 and 31800663), Guizhou Science and Technology Department (QKHRC-2016-4029, QKHPTRC-2016-5801, QKHJC-2019-1467 and QKHZC-2019-2579), Program for Outstanding Youth of Zunyi Medical University (17zy-001), National First-Rate Construction Discipline of Guizhou Province (Pharmacy) (YLXKJS-YX-04) and the Fifth Batch of Talent Base in Guizhou Province (2016).
Notes and references
-
(a) H. Sharghi, M. H. Beyzavi, A. Safavi, M. M. Doroodmand and R. Khalifeh, Adv. Synth. Catal., 2009, 351, 2391–2410 CrossRef CAS;
(b) L. S. Campbell-Verduyn, W. Szymanski, P. C. Postema, R. A. Dierckx, P. H. Elsinga, D. B. Janssen and B. L. Feringa, Chem. Commun., 2010, 46, 898–900 RSC;
(c) C. Francois-Endelmond, T. Carlin, P. Thuery, O. Loreau and F. Taran, Org. Lett., 2010, 12, 40–42 CrossRef CAS PubMed.
- H. Sharghi, M. Hosseini-Sarvari, F. Moeini, R. Khalifeh and A. S. Beni, Helv. Chim. Acta, 2010, 93, 435–449 CrossRef CAS.
- F. De Moliner, S. Crosignani, A. Galatini, R. Riva and A. Basso, ACS Comb. Sci., 2011, 13, 453–457 CrossRef CAS PubMed.
- G. Madhusudhan, M. S. Reddy, Y. N. Reddy, V. Vijayalakshmi, M. Suribabu and V. Balraju, Indian J. Chem., Sect. B: Org. Chem. Incl. Med. Chem., 2010, 49, 978–984 Search PubMed.
- D. Blomberg, M. Hedenstroem, P. Kreye, I. Sethson, K. Brickmann and J. Kihlberg, J. Org. Chem., 2004, 69, 3500–3508 CrossRef CAS PubMed.
- M. Hedenstroem, L. Holm, Z. Q. Yuan, H. Emtenas and J. Kihhlberg, Bioorg. Med. Chem. Lett., 2002, 12, 841–844 CrossRef.
-
(a) E. F. V. Scriven and K. Turnbull, Chem. Rev., 1988, 88, 297–368 CrossRef CAS;
(b) G. A. Jacobs, J. A. Tino and R. Zahler, Tetrahedron Lett., 1989, 30, 6955–6958 CrossRef CAS;
(c) D. M. Coe, P. L. Myers, D. M. Parry, S. M. Roberts and R. Storer, J. Chem. Soc., Chem. Commun., 1990, 2, 151–153 RSC.
-
(a) C. Wang, L. Luo and H. Yamamoto, Acc. Chem. Res., 2016, 49, 193–204 CrossRef CAS PubMed;
(b) M. Lee, J. R. Lamb, M. J. Sanford, A. M. LaPointe and G. W. Coates, Chem. Commun., 2018, 54, 12998–13001 RSC;
(c) Z. Wang, Y. Kuninobu and M. Kanai, J. Am. Chem. Soc., 2015, 137, 6140–6143 CrossRef CAS PubMed.
-
(a) C. E. Song, C. R. Oh, E. J. Roh and D. J. Choo, Chem. Commun., 2000, 18, 1743–1744 RSC;
(b) V. Di Bussolo, M. Caselli, M. R. Romano, M. Pineschi and P. Crotti, J. Org. Chem., 2004, 69, 8702–8708 CrossRef CAS PubMed;
(c) J. Keilitz and R. Haag, Eur. J. Org. Chem., 2009, 19, 3272–3278 CrossRef;
(d) M. Caron, P. R. Carlier and K. B. Sharpless, J. Org. Chem., 1998, 53, 5185–5187 CrossRef.
-
(a) F. Fringuelli, F. Pizzo and L. Vaccaro, J. Org. Chem., 2001, 66, 3554–3558 CrossRef CAS PubMed;
(b) F. Fringuelli, F. Pizzo and L. Vaccaro, J. Org. Chem., 2001, 66, 4719–4722 CrossRef CAS PubMed;
(c) G. Sabitha, R. S. Babu, M. Rajkumar and J. S. Yadav, Org. Lett., 2002, 4, 343–345 CrossRef CAS PubMed;
(d) N. Iranpoor, H. Firouzabadi and M. Shekarize, Org. Biomol. Chem., 2003, 1, 724–727 RSC;
(e) J. S. Yadav, B. V. S. Reddy, B. Jyothirmai and M. S. R. Murty, Tetrahedron Lett., 2005, 46, 6559–6562 CrossRef CAS;
(f) K. Hang, H. Haiyang, V. Stepanenko, M. De Jesus, C. Torruellas, W. Correa and M. Ortiz-Marciales, J. Org. Chem., 2011, 76, 1883–1886 CrossRef PubMed.
-
(a) J. E. T. van Hylckama Vlieg, L. Tang, J. H. Lutje Spelberg, T. Smilda, G. J. Poelarends, T. Bosma, A. E. J. van Merode, M. W. Fraaije and D. B. Janssen, J. Bacteriol., 2001, 183, 5058–5066 CrossRef CAS PubMed;
(b) D. B. Janssen, M. Majerić-Elenkov, G. Hasnaoui, B. Hauer and H. L. Spelberg, Biochem. Soc. Trans., 2006, 34, 291–295 CrossRef CAS PubMed;
(c) Z.-Y. You, Z.-Q. Liu and Y.-G. Zheng, Appl. Microbiol. Biotechnol., 2013, 97, 9–21 CrossRef CAS PubMed;
(d) A. Schallmey and M. Schallmey, Appl. Microbiol. Biotechnol., 2016, 100, 7827–7839 CrossRef CAS PubMed.
-
(a) J. H. Lutje Spelberg, J. E. T. van Hylckama Vlieg, L. Tang, D. B. Janssen and R. M. Kellogg, Org. Lett., 2001, 3, 41–43 CrossRef;
(b) M. M. Elenkov, B. Hauer and D. B. Janssen, Adv. Synth. Catal., 2006, 348, 579–585 CrossRef CAS;
(c) M. M. Elenkov, H. W. Hoeffken, L. Tang, B. Hauer and D. B. Janssen, Adv. Synth. Catal., 2007, 349, 2279–2285 CrossRef CAS;
(d) M. M. Elenkov, H. W. Hoeffken, L. Tang, B. Hauer and D. B. Janssen, Adv. Synth. Catal., 2007, 349, 2279–2285 CrossRef CAS;
(e) B. Seisser, I. Lavandera, K. Faber, J. L. Spelberg and W. Kroutil, Adv. Synth. Catal., 2007, 349, 1399–1404 CrossRef CAS;
(f) G. Hasnaoui-Dijoux, M. M. Elenkov, J. H. Lutje Spelberg, B. Hauer and D. B. Janssen, ChemBioChem, 2008, 9, 1048–1051 CrossRef CAS PubMed;
(g) M. M. Elenkov, L. Tang, A. Meetsma, B. Hauer and D. B. Janssen, Org. Lett., 2008, 10, 2417–2420 CrossRef CAS PubMed;
(h) C. Molinaro, A.-A. Guilbault and B. Kosjek, Org. Lett., 2010, 12, 3772–3775 CrossRef CAS PubMed;
(i) W. Szymanski, C. P. Postema, C. Tarabiono, F. Berthiol, L. Campbell-Verduyn, S. de Wildeman, J. G. de Vries, B. L. Feringa and D. B. Janssen, Adv. Synth. Catal., 2010, 352, 2111–2115 CrossRef CAS;
(j) M. M. Elenkov, I. Primozic, T. Hrenar, A. Smolko, I. Dokli, B. Salopek-Sondi and L. Tang, Org. Biomol. Chem., 2012, 10, 5063–5072 RSC;
(k) S. Chen, C. Yang, J. Wu, G. Xu and L. Yang, Adv. Synth. Catal., 2013, 355, 3179–3190 CrossRef CAS;
(l) A. Mikleušević, Z. Hameršak, B. Salopek-Sondi, L. Tang, D. B. Janssen and M. M. Elenkov, Adv. Synth. Catal., 2015, 357, 1709–1714 CrossRef;
(m) N.-W. Wan, Z.-Q. Liu, F. Xue, Z.-Y. Shen and Y.-G. Zheng, ChemCatChem, 2015, 7, 2446–2450 CrossRef CAS.
- J. Koopmeiners, C. Diederich, J. Solarczek, H. Voß, J. Mayer, W. Blankenfeldt and A. Schallmey, ACS Catal., 2017, 7, 6877–6886 CrossRef CAS.
- F. Fringuelli, O. Piermatti, F. Pizzo and L. Vaccaro, J. Org. Chem., 1999, 64, 6094–6096 CrossRef CAS.
- J. Koopmeiners, B. Halmschlag, M. Schallmey and A. Schallmey, Appl. Microbiol. Biotechnol., 2016, 100, 7517–7527 CrossRef CAS PubMed.
Footnote |
† Electronic supplementary information (ESI) available: Experiment procedures and NMR data. See DOI: 10.1039/c9ra03774h |
|
This journal is © The Royal Society of Chemistry 2019 |