DOI:
10.1039/C9RA02741F
(Paper)
RSC Adv., 2019,
9, 21572-21577
Novel designed azo substituted semi-cyanine fluorescent probe for cytochrome P450 reductase detection and hypoxia imaging in cancer cells†
Received
11th April 2019
, Accepted 30th June 2019
First published on 11th July 2019
Abstract
A hypoxia activated fluorescent probe AZO-Cy which contains an azo group conjugated to the electron withdrawing part of the fluorescent dye was synthesized. In the presence of NADH, AZO-Cy displayed high selectivity and sensitivity to cytochrome P450 reductase (CPR) under low pO2. The probe showed high anti-interference capability in the presence of other biothiols and ions. In A549 cell imaging, the fluorescence intensity is increased about 11-fold under hypoxia compared to normoxia conditions. Further inhibitor experiments showed that CPR is not the only reductase that can take part in the process of azo bond reduction. The probe AZO-Cy displayed high oxygen sensitivity in the identified different hypoxic status of tumor cells which provides huge potential application toward in vivo hypoxia detection.
1. Introduction
Low oxygen concentration or insufficient oxygen supply which means hypoxia is a major phenomenon in various diseases. Oxygen plays an important role in the balance of pathological and physiological processes.1–6 Normally, the hypoxic area means a pO2 value ≤2.5 mm Hg which can be found in solid tumors, cardiac ischemia and inflammatory diseases. Clinical research has found that the hypoxic environment in solid tumors has a certain relationship to the tumor cells’ resistance to treatment and metastasis.7,8 Because of the inadequate supply of oxygen, there is not enough oxygen transferred to reactive oxygen species (ROS) in a hypoxic environment. In this condition, the reduction of ROS concentration means the increase in reducing ability. Groups such as aromatic nitro group, azo group and quinone group have been applied in the designing of various hypoxic activated fluorescent probes.9–12 So far, various methods have been applied in the detection of hypoxia in the clinic, including positron emission tomography/computed tomography (PET/CT), electron paramagnetic resonance imaging (EPRI) and so on.13–15 Because it is non-invasive, and has high sensitivity and high spatiotemporal resolution, fluorescence imaging is still an ideal method for hypoxia detection.16–20
As a metabolism enzyme, cytochrome P450 reductase plays a key role in the reduction of nitro functional compounds and has higher activity in cancerous cells than in normal cells, especially in a hypoxic environment. Several studies have proved that azo aromatic compounds are good substrates for cytochrome P450 reductase.18,20–23 Some fluorescent probes showed good performance under different oxygen partial pressures which meant the azo group was very effective to detect hypoxia. Based on our previous work,19 herein, we report a far-red emitting probe AZO-Cy containing an azo moiety which can be metabolized by cytochrome P450 reductase in hypoxic conditions, the probe displayed a strong fluorescence OFF–ON response at 610 nm in PBS buffer and in hypoxia imaging in tumor cells.
2. Experimental section
2.1 Instruments and chemicals
UV-visible absorption spectra were obtained using a UV-visible spectrometer (Scinco 3000 spectrophotometer). Fluorescent spectra were performed on a Hitachi F-7000 luminescence spectrophotometer in 10 × 10 mm quartz cells with the volume 3.0 mL at 37 °C. 1H and 13C spectra were measured using a Bruker ARX 400 NMR spectrometer. The molecular mass was acquired using ion trap time-of-flight mass spectrometry (MS-TOF). Fluorescence imaging experiments were performed on a confocal microscope (Olympus, IX81, JPN). A liquid chromatography system from Waters Technologies (Waters, American) was applied to all chromatography tests.
All of the chemicals and solvents were purchased from commercial suppliers and used without further purification, unless otherwise stated. Deionized water was used to prepare all aqueous solutions.
2.2 Preparation of probe AZO-Cy
Probe AZO-Cy was synthesized from the commercially available starting material 1-1 as shown in Scheme 2. The key intermediates and probe AZO-Cy were confirmed using 1H NMR, 13C NMR and HR-MS (see ESI†).
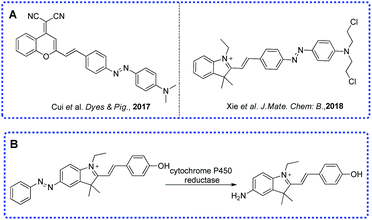 |
| Scheme 1 Previously reported probes (A) and current work (B). | |
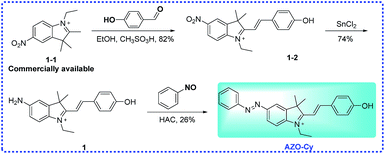 |
| Scheme 2 Synthesis of 1 and probe AZO-Cy. | |
Synthesis of AZO-Cy.
Compound 1 (300.0 mg, 1.0 mmol) and nitrosobenzene (627.0 mg, 5.9 mol) were dissolved in acetic acid (4 mL). Then the mixture was stirred at 25 °C for 5 h. 10 mL water was added and then the mixture was extracted with methylene chloride (10 mL × 3). The combined organic layers were washed with brine and dried over anhydrous Na2SO4. Then the solvent was concentrated in vacuum and the residue was purified using silica gel chromatography (DCM
:
MeOH = 20
:
1, v/v) to provide AZO-Cy (100 mg, 26%) as a bright purple-black solid. 1H NMR (400 MHz, DMSO-d6) δ 8.10 (d, J = 1.7 Hz, 1H), 8.06–7.96 (m, 2H), 7.96–7.82 (m, 4H), 7.64–7.58 (m, 2H), 7.58–7.51 (m, 2H), 6.76 (d, J = 14.4 Hz, 1H), 6.41 (d, J = 8.8 Hz, 2H), 5.76 (s, 1H), 4.29 (q, J = 6.8 Hz, 2H), 1.99 (s, 1H), 1.76 (s, 6H), 1.32 (t, J = 7.0 Hz, 3H), 1.27–1.13 (m, 3H). 13C NMR (101 MHz, DMSO-d6) δ 184.13, 168.27, 152.16, 148.29, 146.05, 145.11, 141.62, 130.85, 129.42, 126.53, 124.89, 122.46, 122.28, 114.75, 109.64, 96.79, 47.44, 37.88, 27.51, 11.98. HRMS (ESI+) calcd for C26H26N3O+ [M]+ = 396.2070, found 396.2066.
2.3 Spectroscopic materials and methods
Double distilled water was used to prepare all aqueous solutions. All spectroscopic measurements were performed in PBS buffer (1% DMSO, 0.1 mM, pH = 7.4). Samples for absorption and fluorescence measurements were contained in 1 cm × 1 cm quartz cuvettes (1.5 mL volume) by setting the slit width of 3 nm × 1.5 nm.
2.4 Cytochrome P450 reductase assay
Cytochrome P450 reductase (rabbit liver, purchased from Sigma-Aldrich, CPR) reactive activity experiments were performed in a 1 cm × 1 cm quartz cuvette with 100 μL volume. Stock solutions of probe AZO-Cy were prepared in pure DMSO (1 mM). All experiments were performed in PBS buffer (0.1 mM, pH = 7.4) in 37 °C water bath. The dosage of cytochrome P450 reductase is 1 U mL−1. Argon gas mixtures with different pO2 were bubbled into the AZO-Cy solution for 30 min to create the different hypoxic environments.
2.5 Cell culture and confocal microscope imaging
A549, purchased from American Type Culture Collection (ATCC, Manassas, VA, USA), was chosen for culturing with RPMI-1640 (Hyclone), supplemented with 10% FBS (fetal bovine serum, Gibco), 2 mM L-glutamine, 100 U mL−1 penicillin and 100 μg mL−1 streptomycin in an atmosphere of 5% CO2 and 95% air (37 °C). Cells were seeded in the 24-well plates at a density of 1 × 105 in a culture medium. For fluorescence imaging, cells were divided into two parts, one part was incubated under different hypoxic (1% pO2) conditions and the other part was under normoxic conditions (21% pO2) for 24 hours, respectively. Then, after washing with PBS buffer twice, the cells were treated with AZO-Cy in FBS-free RPMI-1640 for 30 min before fluorescence imaging, respectively. The pictures were collected with a Nikon instrument (Eclipse Ti-E2000). The fluorescence field was collected with 200 ms exposure time using a Texas Red filter and with 100 ms exposure time for bright field.
3. Results and discussion
3.1 Synthesis and structural characterization
Previously, our group have developed a series of hypoxic activated fluorescent probes which were based on different functional group, including benzoquinone, aromatic nitro group and azo group.24,25 We found that the azo bond is more sensitive than other functional group under hypoxic conditions, such as a nitro group, benzoquinone and N–O bond. Most of the probes’ functional groups were linked to the electron-rich side (phenolic hydroxyl group or aromatic amino) directly or by a linker. Here, the designed probe AZO-Cy contains an azo group which is connected to the indole group (electron-withdrawing part) directly. Probe AZO-Cy displayed a dramatic optical change as well as high sensitivity to cytochrome P450 reductase.
3.2 UV-vis and fluorescence study
The UV-vis spectra and fluorescence spectra of AZO-Cy were monitored in PBS buffer. From Fig. S1,† we can find that the absorption spectra of both compounds only showed minor differences. In contrast, the fluorescence spectra showed great differences. No fluorescence signal could be detected for the probe AZO-Cy, but an obvious signal at the wavelength of 610 nm was detected for the reduced dye 1. This means compound AZO-Cy showed great application potential in the detection of cytochrome P450 reductase with fluorescence (Table S1†).
3.3 Spectrum of probe AZO-Cy towards cytochrome P450 reductase
Cytochrome P450 reductase plays key roles in the detoxification and activation of xenobiotics and has been widely applied in the metabolism of organic molecules such as drugs, pesticides, plant toxins, and mutagens. Until now, several research studies, including from our group, have proven that the enzyme shows a strong reducing ability that could reduce azo compounds under hypoxic conditions. Here, the new designed probe has a significant difference in chemical structure, as shown in Scheme 1, to most of the reported probes, where the functional group was connected to the electron-donating end of the fluorophore molecule.9,16,19,23 Here, the azo group of the probe AZO-Cy connected to the electron-withdrawing end which is a first report.
As shown in Fig. 1, nearly no fluorescence signal at 610 nm was detected because of the vibration of the azo bond in probe AZO-Cy. After enzyme CPR was added, we monitored the fluorescence changes of AZO-Cy (10 μM in PBS, pH 7.4, λex = 450 nm) after the additions of CPR (1.0 U mL−1) and NADH (100 μM) (Fig. 1b). A significant signal emission was detected at 610 nm and enhanced about 15-fold. The detected fluorescence intensity of AZO-Cy displayed a concentration-dependent and time-dependent increase. After probe AZO-Cy, CPR and NADH reacted for 5 min, the fluorescence intensity only showed a slight increase which suggested a rapid response to enzyme CPR (Fig. 1a).
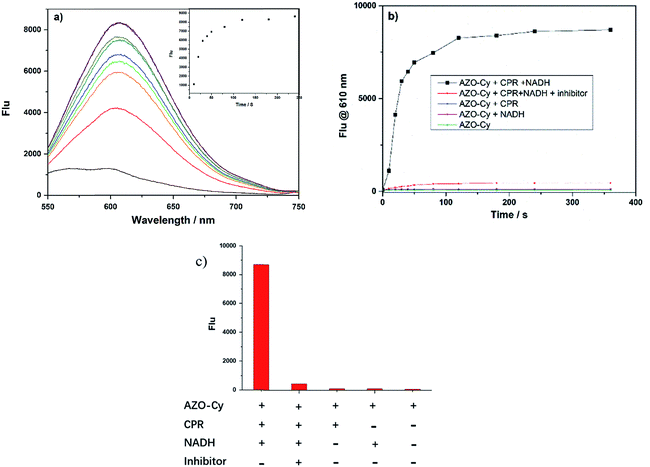 |
| Fig. 1 Activity of probe AZO-Cy towards cytochrome P450 reductase. (a) Fluorescence spectra of probe AZO-Cy (10 μM) incubated in the presence of CPR (1.0 U mL−1) and NADH (50 μM) in PBS buffer at 37 °C; (b) time dependent fluorescence changes of probe in various conditions and (c) their fluorescence responses (λex = 450 nm, 6 min). Probe AZO-Cy (10 μM) was incubated in the presence of CPR (1.0 U mL−1), NADH (50 μM), or an inhibitor (DPIC, 100 μM) at 37 °C. | |
For an inhibitor assay, diphenyliodonium chloride (DPIC) was chosen as a reported inhibitor of CPR,27 and was added to the enzyme prior to the incubation of AZO-Cy, and the inhibitory effect on CPR was investigated. The incubation of DPIC (100 μM) in a mixture of AZO-Cy, CPR and NADH led to a very weak fluorescence signal (Fig. 1b and c), the signal intensity of which was twelve times lower than AZO-Cy expressed in the presence of CPR and NADH, due to the competitive inhibition of DPIC to the enzymatic action (Fig. 1c). The experiment also proved that the coenzyme NADH is also a key factor in the enzyme-catalyzed reduction.
For accurate measurement of the real activities of CPR in various biological samples and to get the linear fluorescence response with enzyme concentration, kinetic experiments were also investigated. The kinetics of the release of 1 from AZO-Cy triggered by CPR in the presence of NADH was measured by adding a known concentration of AZO-Cy (0–100 μM) to the reaction mixture. The fluorescence change was recorded at the wavelength of 610 nm, all assays were performed in triplicate, and the results reported are the average of three experiments (Fig. 2).
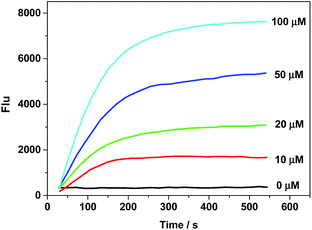 |
| Fig. 2 Fluorescence intensity changes (F610 nm) with λex = 450 nm at 37 °C over an incubation time of 10 min at varied concentrations of AZO-Cy: 0 (control), 10, 20, 50 and 100 μM. The measurements were performed in PBS buffer with 1.0 U mL−1 CPR and 100 μM of NADH. | |
Probe AZO-Cy shows high reaction activity to CPR with NADPH which is faster than reported before.18,20,26–28 Experiments show that after mixing for 2 min, the fluorescence signal can reach a maximum, which provides the basis for rapid response detection of hypoxia (Table S2†). The mechanism of the reduction of probe AZO-Cy by CPR was also confirmed by HPLC (Fig. S3†). With the passage of reaction time, the concentration of AZO-Cy (retention time was 5.68 min) decreased obviously, and it almost disappeared after about 5 min (Fig. S3†). Meanwhile, the concentration of fluorophore 1 (retention time was 4.19 min) increased gradually.
To further examine whether the probe AZO-Cy was suitable for hypoxia detection, cytochrome P450 reductase catalyzed reduction was applied in an in vitro assay under different pO2 (Fig. 3). In order to create a hypoxic environment, a mixed composition which contains argon and different concentrations of oxygen was bubbled into the AZO-Cy solution (1.0 μM, PBS buffer with 1% DMSO, pH 7.4) for 45 min, then cytochrome P450 reductase was added. Upon the addition of NADH (100 μM, as a cofactor of cytochrome P450 reductase) and CPR, a dramatic fluorescence enhancement was observed at around 610 nm. Under different hypoxia conditions, the fluorescence was produced with different intensities. We can find that when the oxygen concentration decreases, there is a significant nonlinear increase in fluorescence intensity. The experiment also demonstrated probe AZO-Cy could be used for detection of hypoxic cells.
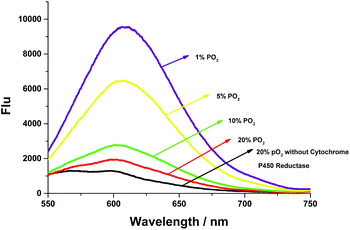 |
| Fig. 3 Fluorescence changes of AZO-Cy without (purple curve) or with (other curves) various oxygen levels. The spectra were measured in phosphate buffer (pH 7.4 with 1% DMSO, containing 0.1 mM NADH) at 37 °C. | |
3.4 Selective response of probe AZO-Cy
To further evaluate the application in living cells, the anti-interference experiment also should be considered. Probe AZO-Cy was treated with reductants (VC and glutathione), biothiols (cysteine and dithiothreitol), inorganic salts (FeCl2), glucose, NADH or amino acid (tyrosine). As shown in Fig. S2,† a noticeable fluorescence signal was observed even when the concentration of interference substances is up to 50 equiv. Therefore, it can be concluded that AZO-Cy displayed high selectivity for bio-reductase and is stable in biological systems.
3.5 Hypoxic cell imaging
Due to the excellent performance in different pO2 experiments and anti-interference capability, we carried out a cell imaging experiment to detect different hypoxic conditions in cancer cells. As shown in Fig. 4, under normoxia conditions (pO2 is 20%), the fluorescence intensity was weak. With the strengthening of the degree of hypoxia (from 10% to 1%), the fluorescence intensity was increased gradually. This experiment showed AZO-Cy was sensitive enough to the pO2. With Image J™ software (1.47v), the average fluorescence intensity value at different oxygen conditions was calculated (Fig. 4i). The mean fluorescence intensity increased from 1.573 to 17.701, nearly about an 11-fold increase.
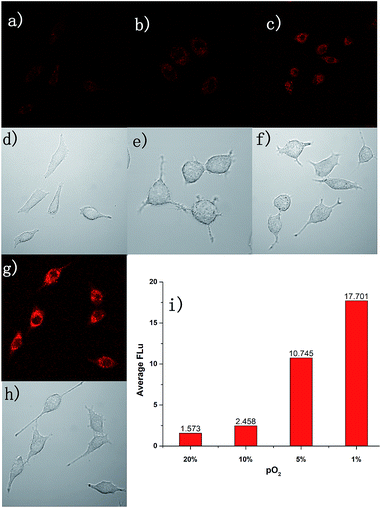 |
| Fig. 4 Fluorescence microphotographs of A549 cells incubated with 1.0 μM fluorescent probe AZO-Cy at 37 °C. All the cells were incubated at different oxygen partial pressures; (a) and (d) were taken at aerobic condition (20% pO2). The others were taken at different hypoxic conditions: (b) and (e) were at 10% pO2; (c) and (f) were at 5% pO2; (g) and (h) were at 1% pO2, respectively. (d) (e) (f) and (h) were taken with bright field illumination; (a) (b) (c) and (g) were taken in fluorescence mode (red). (i) The mean fluorescence photons and relative ratio. Image J software gave an average emission value of fluorescence photons. | |
For further evaluation of probe AZO-Cy applied in hypoxia imaging in cancer cells, A549 cell lines were also chosen for cellular fluorescence imaging in the presence of inhibitor diphenyliodonium chloride (DPIC). As shown in Fig. 5, under hypoxic conditions (1% pO2), even in the presence of low concentrations of inhibitor, a weak fluorescence signal was also observed. The fluorescence intensity is about 64% percent in the presence of the inhibitor compared to the routine experiment under hypoxic conditions. This experiment further proved that even in the presence of an inhibitor of CPR, part of the probe AZO-Cy was also reduced under hypoxic conditions.
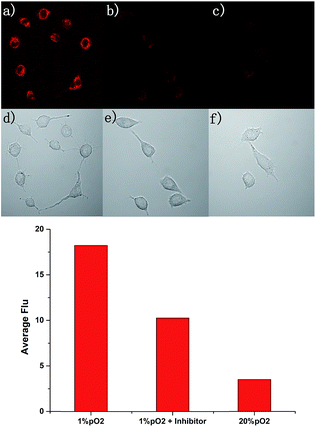 |
| Fig. 5 Fluorescence microphotographs of A549 cells incubated with 1.0 μM fluorescent probe AZO-Cy at 37 °C. All of the cells were incubated under different conditions: (a) and (d) were taken under hypoxic conditions (1% pO2); (b) and (e) were taken under hypoxic conditions (1% pO2) and the cells were incubated with inhibitor; (c) and (f) were taken under aerobic conditions (20% pO2). The first row was taken in fluorescence mode (red) (a–c); and the second row was taken with bright field illumination. | |
4 Conclusion
In summary, we present a new design concept for a hypoxia fluorescence probe in which the functional group was conjugated to the indole group (electron-deficient side). In enzyme experiments, AZO-Cy had a good ability to distinguish oxygen concentration rapidly and sensitively in the presence of NADH. The fluorescence intensity in hypoxic conditions is about 11-fold stronger than aerobic conditions. Enzyme activity inhibition experiments indicated that CPR is not the only reductase that can reduce the azo bond. The azo bond as a functional group for hypoxia detection needs further study.
Conflicts of interest
There are no conflicts to declare.
Acknowledgements
We thank the National Natural Science Foundation of China (31570360 and 31702070) for financial support. This work was also sponsored by Program of Shanghai Academic/Technology Research Leader (17XD1423000) and by Shanghai Rising-Star Program (18QB1404000).
Notes and references
- R. Choudhury, Int. J. Gen. Med., 2018, 11, 431–442 CrossRef.
- A. Schurich, I. Magalhaes and J. Mattsson, Immunotherapy, 2019, 11, 335–345 CrossRef CAS PubMed.
- V. Petrova, M. Annicchiarico-Petruzzelli, G. Melino and I. Amelio, Oncogenesis, 2018, 7, 10 CrossRef.
- H. Kim, Q. Lin, P. M. Glazer and Z. Yun, Breast Cancer Res., 2018, 20, 16 CrossRef PubMed.
- M. C. Simon, Cancer Res., 2016, 76, IA13 Search PubMed.
- C. Ward, S. P. Langdon, P. Mullen, A. L. Harris, D. J. Harrison, C. T. Supuran and I. H. Kunkler, Cancer Treat. Rev., 2013, 39, 171–179 CrossRef CAS.
- K. Teramoto, Y. Kataoka, T. Igarashi, Y. Ohshio, J. Hanaoka and Y. Daigo, Cancer Res., 2015, 75, 5080 Search PubMed.
- P. R. de Jong, A. D. Campos, S. L. Shanahan, A. Richardson and G. Powis, Cancer Res., 2016, 76, A25–A25 Search PubMed.
- Q. Cai, T. Yu, W. P. Zhu, Y. F. Xu and X. H. Qian, Chem. Commun., 2015, 51, 14739–14741 RSC.
- K. Kiyose, K. Hanaoka, D. Oushiki, T. Nakamura, M. Kajimura, M. Suematsu, H. Nishimatsu, T. Yamane, T. Terai, Y. Hirata and T. Nagano, J. Am. Chem. Soc., 2010, 132, 15846–15848 CrossRef CAS PubMed.
- K. Y. Zhang, P. L. Gao, G. L. Sun, T. W. Zhan, X. L. Li, S. J. Liu, Q. Zhao, K. K. W. Lo and W. Huang, J. Am. Chem. Soc., 2018, 140, 7827–7834 CrossRef CAS.
- S. Z. Luo, R. F. Zou, J. C. Wu and M. P. Landry, ACS Sens., 2017, 2, 1139–1145 CrossRef CAS PubMed.
- R. Stoyanova, K. Huang, K. Sandler, H. Cho, S. Carlin, P. B. Zanzonico, J. A. Koutcher and E. Ackerstaff, Transl Oncol., 2012, 5, 437–U114 CrossRef.
- T. A. M. Egeland, K. Gulliksrud, J. V. Gaustad, B. Mathiesen and E. K. Rofstad, Magn. Reson. Med., 2012, 67, 519–530 CrossRef.
- C. T. Lee, M. K. Boss and M. W. Dewhirst, Antioxid. Redox Signaling, 2014, 21, 313–337 CrossRef CAS.
- D. Yang, H. Y. Tian, T. N. Zang, M. Li, Y. Zhou and J. F. Zhang, Sci. Rep., 2017, 7, 9174 CrossRef.
- Y. Fang, W. Shi, Y. M. Hu, X. H. Li and H. M. Ma, Chem. Commun., 2018, 54, 5454–5457 RSC.
- A. Chevalier, P. Y. Renard and A. Romieu, Chem.–Asian J., 2017, 12, 2008–2028 CrossRef CAS PubMed.
- L. Cui, Y. P. Shi, S. P. Zhang, L. L. Yan, H. Zhang, Z. R. Tian, Y. Y. Gu, T. Guo and J. H. Huang, Dyes Pigm., 2017, 139, 587–592 CrossRef CAS.
- S.-Y. Na, S. Park, S.-Y. Kim and H.-J. Kim, Dyes Pigm., 2019, 161, 247–251 CrossRef CAS.
- W. Piao, S. Tsuda, Y. Tanaka, S. Maeda, F. Y. Liu, S. Takahashi, Y. Kushida, T. Komatsu, T. Ueno, T. Terai, T. Nakazawa, M. Uchiyama, K. Morokuma, T. Nagano and K. Hanaoka, Angew. Chem. Int. Ed., 2013, 52, 13028–13032 CrossRef CAS.
- X. Li, J. Cheng, Y. L. Gong, B. Yang and Y. Z. Hu, Biosens. Bioelectron., 2015, 65, 302–306 CrossRef CAS.
- Y. Zhou, M. Maiti, A. Sharma, M. Won, L. Yu, L. X. Miao, J. Shin, A. Podder, K. N. Bobba, J. Han, S. Bhuniya and J. S. Kim, J. Controlled Release, 2018, 288, 14–22 CrossRef CAS.
- T. Guo, L. Cui, J. N. Shen, W. P. Zhu, Y. F. Xu and X. H. Qian, Chem. Commun., 2013, 49, 10820–10822 RSC.
- N. Kwon, M. K. Cho, S. J. Park, D. Kim, S. J. Nam, L. Cui, H. M. Kim and J. Yoon, Chem. Commun., 2017, 53, 525–528 RSC.
- J. Zhang, H. W. Liu, X. X. Hu, J. Li, L. H. Liang, X. B. Zhang and W. Tan, Anal. Chem., 2015, 87, 11832–11839 CrossRef CAS.
- S. H. Luo, Y. C. Liu, F. Y. Wang, Q. Fei, B. Shi, J. C. An, C. C. Zhao and C. H. Tung, Analyst, 2016, 141, 2879–2882 RSC.
- P. Verwilst, J. Han, J. Lee, S. Mun, H. G. Kang and J. S. Kim, Biomaterials, 2017, 115, 104–114 CrossRef CAS PubMed.
Footnote |
† Electronic supplementary information (ESI) available. See DOI: 10.1039/c9ra02741f |
|
This journal is © The Royal Society of Chemistry 2019 |