DOI:
10.1039/C9RA02267H
(Paper)
RSC Adv., 2019,
9, 16215-16222
Aerobic, metal-free synthesis of 6H-chromeno[4,3-b]quinolin-6-ones†
Received
25th March 2019
, Accepted 17th May 2019
First published on 23rd May 2019
Abstract
A Friedländer-based method for transition-metal free, aerobic synthesis of chromene-fused quinolinones is reported. The coupling of 4-hydrocoumarins and 2-aminobenzyl alcohols proceeds in the presence of acetic acid solvent and oxygen oxidant, affording 6H-chromeno[4,3-b]quinolin-6-ones in good to excellent yields. The reactions are tolerant of functionalities such as alkyl, methoxy, bromo, chloro, and N-heterocycle. Isosteric cyclic 1,3-diketones and 2-amino acetophenones also give fused quinolinones under reaction conditions. The method herein offers a rapid and benign synthesis of hitherto challenging N-heterocycles. To our best knowledge, such a convenient pathway to obtain chromene-fused quinolinones have not been known in the literature.
1. Introduction
6H-Chromeno[4,3-b]quinolin-6-ones find use as flourescent sensors and bioactive molecules.1 Some derivatives are inherent intermediates for synthesis of potent agents in biorelevant studies.1b Conventional methods to prepare 6H-chromeno[4,3-b]quinolin-6-ones rely on the Vilsmeier-Haack conditions to build up bridge quinolines. Pioneering reports by Tabakovic and Heber perhaps disclosed the earliest examples of formylation/cyclization methodology.2 Following these reports, a number of couplings have been developed to avoid the use of toxic POCl3. Notably, most of the available methods require prefunctionalized 2-halobenzaldehydes or 4-halo-3-formylcoumarin (halo = bromo or chloro).3 Complementary to atom economy, it would be much more beneficial should commercial, simple, and non-toxic reagents be used. Iodine-, Lewis acid-, and ionic liquid-catalyzed coupling of 4-hydroxycoumarins, anilines, and aldehydes to obtain 7-aryl 6H-chromeno[4,3-b]quinolin-6-ones are known.4 For synthesis of unsubstituted, chromene-fused quinolines, the group of Yao reported a seminal method for iron-mediated reductive condensation of 2-nitrobenzaldehydes and 4-hydroxycoumarins (Scheme 1, pathway A).5a Cyclic 1,3-diketones were also competent substrates. Later, Su, Xie, and co-workers described a synthesis of 6H-chromeno[4,3-b]quinolin-6-ones from specialized 4-(phenylamino)-2H-chromen-2-ones and N,N-dimethylformamide (DMF) as a methine bridge (Scheme 1, pathway B).5b Recently, Sakhuja et al. presented a similar coupling of 4-hydroxycoumarins, phenylhydrazines, and a C1 source dimethylsulfoxide (DMSO) (Scheme 1, pathway C).5c These methods, however, suffer from undisputed problems such as using excess amount of transition metal,5a the requirement of prefunctionalized starting materials,5b and the formation of toxic by-products,5c thus limiting the use in pharmaceutical industry. Consequently, development of a simple and benign method for synthesis of unsubstituted 6H-chromeno[4,3-b]quinolin-6-ones is pushed to the forefront of synthetic demands.
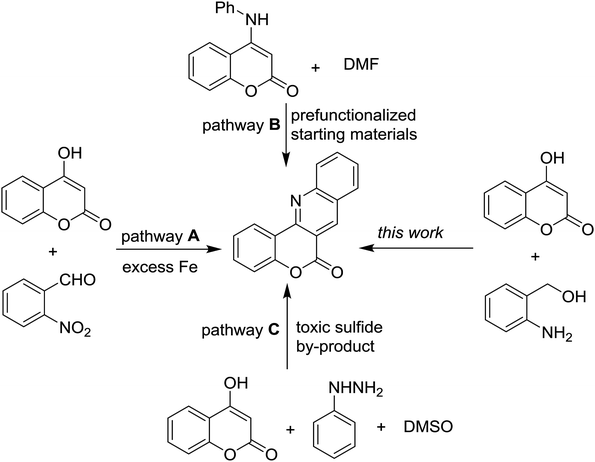 |
| Scheme 1 Synthesis of 6H-chromeno[4,3-b]quinolin-6-one. | |
In 2013, our group reported a method for synthesis of quinolines through the modified Friedländer mechanism.6,7 The targeted compounds could be obtained from condensation of 2-aminobenzaldehydes and acetophenones. The aldehydes, however, are commercially limited and commonly prone to some Friedländer conditions.8 Our conditions allowed for use of 2-aminobenzyl alcohols, which are more abundant and not decomposed during the course of Friedländer reactions. We hypothesize that if 4-hydroxycoumarins oxidatively couple with 2-aminobenzyl alcohols, 6H-chromeno[4,3-b]quinolin-6-ones would be obtained. Reported prominent examples of modified Friedländer for fused heterocycles' synthesis commonly require the use of transition metals.9 Herein we report a method for a rapid, metal-free, aerobic synthesis of 6H-chromeno[4,3-b]quinolin-6-ones and other isosteric fused quinolinones. Importantly, oxygen is used as a sole oxidant for alcohol oxidation.10 Until now there has not such an aerobic coupling of 4-hydroxycoumarins and 2-aminobenzyl alcohols reported in the literature, to our best knowledge.
2. Results and discussion
Reaction of 2-aminobenzyl alcohol and 4-hydroxycoumarin was investigated with respect to solvent, oxidant, temperature, and molar ratio of reactants (Table 1). Among acidic solvents, acetic acid gave the best yield of product 1.11 The reaction can be run in water, albeit at lower yield (entry 3). Using 1 equivalent of acetic acid in glycerol resulted in incomplete conversion of starting materials (entry 4). The reaction was cleanly progressed under oxygen oxidant. A moderate yield of 1 was obtained if the condensation was run under air atmosphere (entry 5). Although organic peroxides are common oxidants for organic reactions, using peroxides was not successful for the condensation. Di-tert-butyl peroxide was inferior to oxygen as oxidant (entry 6), while other peroxide-typed oxidants were much less reactive (entries 7–9). Use of an inorganic oxidant K2S2O8 gave the product in moderate yield (entry 10). Although we could not rationalize the mechanism at this moment, low solubility of K2S2O8 may help not oxidize the aldehyde intermediate, thus avoid decomposition. Decreasing the reaction temperature plummeted the yield of product 1 (entries 11 and 12). Almost no conversion of starting material was observed if the reaction was run at room temperature. During the reaction course, self-condensation of 2-aminobenzyl alcohol did not occur, presumably showing that reaction conditions are not extremely oxidative. Consequently, increasing the amount of 4-hydroxycoumarin with regard to 2-aminobenzyl alcohol was then considered because of the much cheaper reagent 4-hydroxycoumarin. As expected, the yield of 1 increased if excess amount of 2-aminobenzyl alcohol was used (entries 13–15). Increasing the amount of solvent helped get better yield of the reaction, since the product was somewhat insoluble (entry 16). Using more than 2 mL of acetic acid, however, did not affect the yield of 1. Kinetic of the reaction was also studied and presented in Scheme 2. Almost full conversion of 2-aminobenzyl alcohol was observed after only 2 hours. The method, thus, provides a rapid route to synthesize a complex heterocycles in a high yield.
Table 1 Optimization of reaction conditionsa
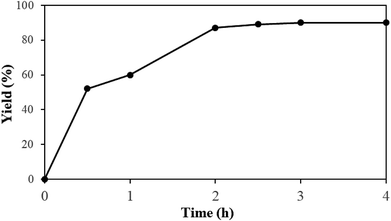 |
| Scheme 2 Kinetic profile for the reaction of 2-aminobenzyl alcohol with 4-hydroxycoumarin. Conditions: 2-aminobenzyl alcohol (0.2 mmol), 4-hydroxycoumarin (0.8 mmol), acetic acid (2 mL), in oxygen. Yields are GC yields using diphenyl ether internal standard. | |
Synthesis of 6H-chromeno[4,3-b]quinolin-6-ones is presented in Table 2. Good to excellent yields were obtained in most cases. Reactions gave clean crude mixtures without forming byproducts from decomposition of 2-aminobenzyl alcohols. We firstly observe the compatibility of functionality on 4-hydroxycoumarins in the reaction. Isolation of 6H-chromeno[4,3-b]quinolin-6-one from the coupling of 2-aminobenzyl alcohol gave 92% yield (entry 1). If 2-aminobenzyl amine was used, a same product was obtained, albeit at lower yield. To prove the practicability of the method, the reaction was run at larger scales and still afforded an excellent yield of compound 1. Recrystallization could be used to obtain the product in good yield if 50 mmol scale reaction was run. Wide tolerance of functional groups were observed in the method. Chloro (2), bromo (3), methyl (4), ethyl (5), and methoxy (7) groups gave products in good yields. Halogenated 6H-chromeno[4,3-b]quinolin-6-ones are valuable, since further modifications could be obtained through transition metal catalyzed carbon-halogen activation. Especially, the bromo derivative is firstly synthesized using a simple method. In the report of Xie and Su,5b the use of high valent copper for selective C–H activation would be competed with C–Br activation, resulting in the regioselective problem. Meanwhile, a metal-hydride condition, as described by Yao and co-workers,5a could substitute a good leaving group such as bromide by hydride. Thus, our method allows the synthesis of notorious halogenated 6H-chromeno[4,3-b]quinolin-6-ones. A dibenzonaphthyridinone 8 was obtained in good yield, showing that synthesis of N-heterocycles is affordable in the conditions. Unprotected 4-hydroxyquinolin-2(1H)-one failed to give the product. A few 2-aminobenzyl alcohols were also studied with regard to the coupling with 4-hydroxycoumarin. 9-Chloro-6H-chromeno[4,3-b]quinolin-6-one 9 was obtained in 81% yield (entry 9). A hindered 2-aminobenzyl alcohol with respect to the nucleophilic amine site still afforded the product in good yield (10). If 2-amino benzyhydrol was used, a 7-arylated 6H-chromeno[4,3-b]quinolin-6-one 11 was obtained in 86% yield (entry 11). It should be noted that this compound is not synthetically possible if the conditions of Xie or Yao are applied.5a,5b
Table 2 Scope of 6H-chromeno[4,3-b]quinolin-6-ones synthesisa
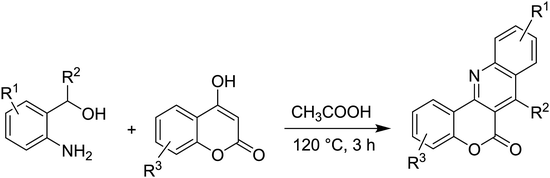
|
Entry |
Reactant 1 |
Reactant 2 |
Product |
Yield, % |
2-Aminobenzyl alcohols (0.2 mmol), 4-hydroxycoumarins (0.8 mmol), acetic acid (2 mL), 120 °C, 3 h. Yields are isolated yields. Please see the ESI for details. 2-Aminobenzyl amine (0.2 mmol) instead 2-aminobenzyl alcohol. 2-Aminobenzyl alcohol (2 mmol), 120 °C, 5 h. 2-Aminobenzyl alcohol (50 mmol), 4-hydroxycoumarin (100 mmol), acetic acid (250 mL), 120 °C, 24 h. The product was obtained after recrystallization. Purity: 93%. |
1 |
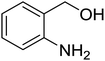 |
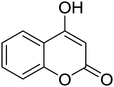 |
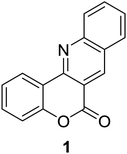 |
92, 85b, 90c, 88d |
2 |
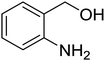 |
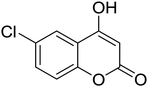 |
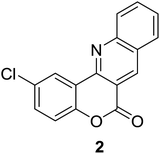 |
76 |
3 |
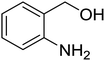 |
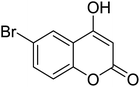 |
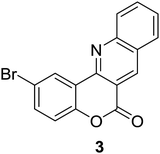 |
78 |
4 |
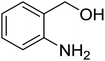 |
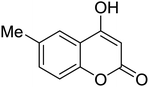 |
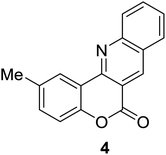 |
85e |
5 |
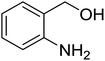 |
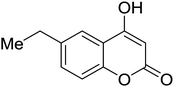 |
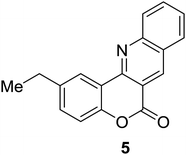 |
84 |
6 |
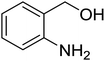 |
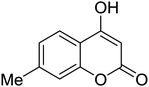 |
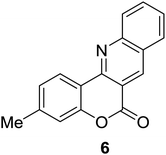 |
86 |
7 |
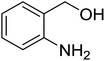 |
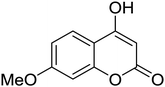 |
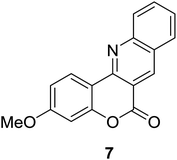 |
75 |
8 |
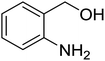 |
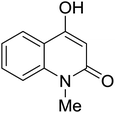 |
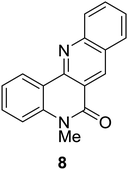 |
89 |
9 |
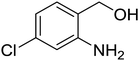 |
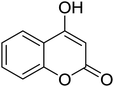 |
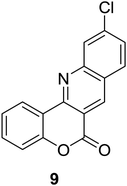 |
81 |
10 |
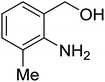 |
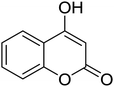 |
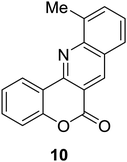 |
90 |
11 |
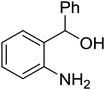 |
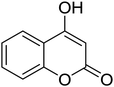 |
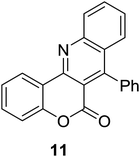 |
86 |
The condensation became much more sluggish if 2-amino carbonyl compounds are used. A 7-substituted 6H-chromeno[4,3-b]quinolin-6-one 12 was obtained in moderate yield when 4-hydroxycoumarin reacted with 2′-aminoacetophenone (Scheme 3). It should be noted that modified reaction conditions were required for this entry, since self-condensation of the 2-amino keto compound was detected. A more hindered 2-aminobenzophenone failed to couple with 4-hydroxycoumarin. Synthesis of other fused quinolinones was also attempted. Friedländer reactions of 2-aminobenzyl alcohol and cyclic ketones afforded products (13–15, Scheme 3) in moderate to good yields. It should be noted that isosteric amides (barbituric acid) or esters (meldrum's acid) failed to give the products.
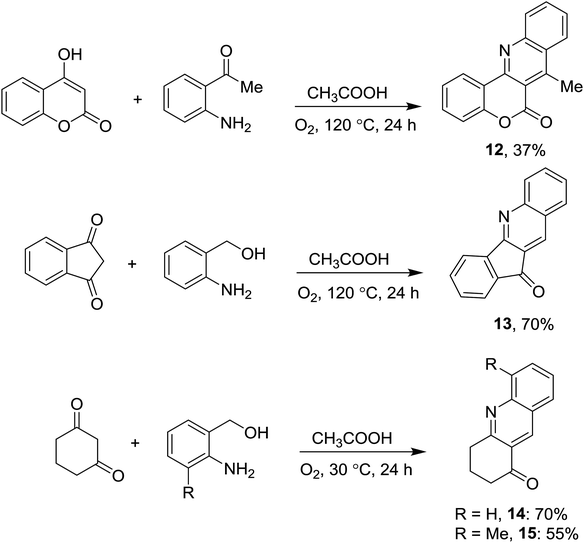 |
| Scheme 3 Synthesis of other fused quinolinones. | |
A possible mechanism is proposed as presented in Scheme 4. A tautomerism of 4-hydroxycoumarin would give a more active form A, which then affords an imine intermediate with 2-aminobenzyl alcohol. Oxidation of alcohol to aldehydes possibly happens after condensation, since 2-amino carbonyls either gave a complicated reaction mixture or failed to give product. The following enamine-aldehyde condensation (B to D) would complete the formation of quinoline ring. The presence of hydroxy-form intermediate C was slightly detected by GC-MS and efforts to isolate the compound is on going. Alternatively, formation of a benzyl cation from the intermediate B could afford D by a nucleophilic addition. Aromatisation would give the desired product. Although oxidation of the C–C bond commonly occurs in the presence of a transition metal,5b this hypothesis could not be completely ruled out at this moment.
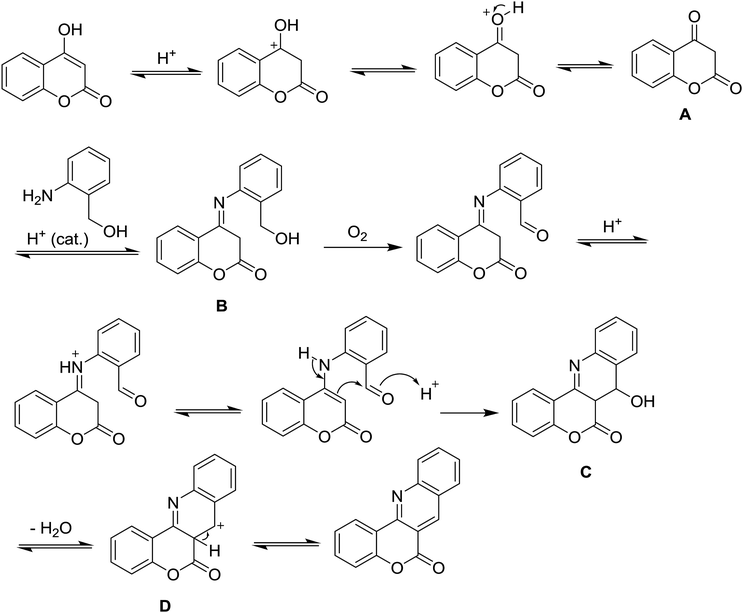 |
| Scheme 4 Plausible mechanism. | |
3. Conclusions
In conclusion, we have developed an operationally simple and general method for aerobic, metal-free synthesis of 6H-chromeno[4,3-b]quinolin-6-ones. The reaction employs acetic acid as a Brønsted acid mediator and a solvent, and oxygen oxidant. Significant development from earlier methods include excellent atom economy, ease in scale up, and general substrate scope. The reaction is tolerant of functionalities such as methyl, methoxy, ethyl, chloro, bromo, and N-heterocycle. Other fused quinolines could also be obtained in good yields without major modification of reaction conditions. The method provides a rapid and convenient pathway to synthesize complex fused N-heterocycles that, to our best knowledge, have not been reported in the literature.
Conflicts of interest
The authors declare no competing financial interest.
Acknowledgements
This research is funded by Ho Chi Minh City University of Technology – VNU-HCM under grant number T-KTHH-2018-99. We also want to thank Hanh T. H. Nguyen (University of Houston) and Tuong A. To (Ho Chi Minh City University of Technology – VNU-HCM) for giving us some valuable substrates.
References
- For review:
(a) L. J. Nunez-Vergara, J. A. Squella, P. A. Navarrete-Encina, E. Vicente-Garcia, S. Preciado and R. Lavilla, Curr. Med. Chem., 2011, 18, 4761 CrossRef CAS PubMed ; selected examples:;
(b) N. Mulakayala, D. Rambabu, M. R. Raja, M. Chaitanya, C. K. Kumar, A. M. Kalle, G. R. Krishna, C. M. Reddy, M. V. Rao and M. Pal, Bioorg. Med. Chem., 2012, 20, 759 CrossRef CAS PubMed;
(c) A. A. Patel, H. B. Lad, K. R. Pandya, C. V. Patel and D. I. Brahmbhatt, Med. Chem. Res., 2013, 22, 4745 CrossRef CAS;
(d) S. Kumari, S. M. Abdul Shakoor, S. Khullar, S. K. Mandal and R. Sakhuja, Org. Biomol. Chem., 2018, 16, 3220 RSC.
-
(a) K. Tabaković, I. Tabaković, N. Ajdini and O. Leci, Synthesis, 1987, 308 CrossRef;
(b) D. Heber, Arch. Pharm., 1987, 320, 595 CrossRef CAS;
(c) V. O. Iaroshenko, S. Ali, T. M. Babar, S. Dudkin, S. Mkrtchyan, N. H. Rama, A. Villinger and P. Langer, Tetrahedron Lett., 2011, 52, 373 CrossRef CAS.
-
(a) J. Wu and X. Wang, Org. Biomol. Chem., 2006, 4, 1348 RSC;
(b) V. O. Iaroshenko, F. Erben, S. Mkrtchyan, A. Hakobyan, M. Vilches-Herrera, S. Dudkin, A. Bunescu, A. Villinger, V. Y. Sosnovskikh and P. Langer, Tetrahedron Lett., 2011, 67, 7946 CrossRef CAS;
(c) F. Peng, J. Liu, L. Li and Z. Chen, Org. Biomol. Chem., 2018, 666 CrossRef CAS . See also ref. 1c and d.
-
(a) Md. N. Khan, S. Pal, S. Karamthulla and L. H. Choudhury, New J. Chem., 2014, 38, 4722 RSC;
(b) K. V. Sashidhara, G. R. Palnati, L. R. Singh, A. Upadhyay, S. R. Avula, A. Kumar and R. Kant, Green Chem., 2015, 17, 3766 RSC;
(c) G. Rahimzadeh, S. Bahadorikhalili, E. Kianmehr and M. Mahdavi, Mol. Diversity, 2017, 21, 597 CrossRef CAS.
-
(a) R. R. Rajawinslin, S. D. Gawande, V. Kavala, Y.-H. Huang, C.-W. Kuo, T.-S. Kuo, M.-L. Chen, C.-H. He and C.-F. Yao, RSC Adv., 2014, 4, 37806 RSC;
(b) Y. Weng, H. Zhou, C. Sun, Y. Xie and W. Su, J. Org. Chem., 2017, 82, 9047 CrossRef CAS;
(c) S. Kumari, S. M. A. Shakoor, D. Markad, S. K. Mandal and R. Sakhuja, Eur. J. Org. Chem., 2019, 705 CrossRef CAS.
- N. T. S. Phan, T. T. Nguyen, K. D. Nguyen and A. X. T. Vo, Appl. Catal., A, 2013, 464–465, 128 CrossRef CAS.
- J. Marco-Contelles, E. Pérez-Mayoral, A. Samadi, M. do Carmo Carreiras and E. Soriano, Chem. Rev., 2009, 109, 2652 CrossRef CAS.
-
(a) A. A. Cordi, P. Desos, J. C. R. Randle and J. Lepagnol, Bioorg. Med. Chem., 1995, 3, 129 CrossRef CAS;
(b) E. C. Riesgo, X. Jin and R. P. Thummel, J. Org. Chem., 1996, 61, 3017 CrossRef CAS;
(c) C. Zhang, C. K. De, R. Mal and D. Siedel, J. Am. Chem. Soc., 2008, 130, 416 CrossRef CAS PubMed.
-
(a) C. S. Cho, B. T. Kim, T.-J. Kim and S. C. Shim, Chem. Commun., 2001, 2576 RSC;
(b) M. Mastalir, M. Glatz, E. Pitternauer, G. Allmaier and K. Kirchner, J. Am. Chem. Soc., 2016, 138, 15543 CrossRef CAS PubMed;
(c) S. Parua, R. Sikari, S. Sinha, S. Das, G. Chakraborty and N. D. Paul, Org. Biomol. Chem., 2018, 16, 274 RSC;
(d) B. Pan, B. Liu, E. Yue, Q. Liu, X. Yang, Z. Wang and W.-H. Sun, ACS Catal., 2016, 6, 1247 CrossRef CAS;
(e) S. Das, D. Maiti and S. De Sarkar, J. Org. Chem., 2018, 83, 2309 CrossRef CAS PubMed.
- N. Anand, S. Koley, B. J. Ramulu and M. S. Singh, Org. Biomol. Chem., 2015, 13, 9570 RSC.
- Please see the ESI† for full studies of reaction conditions..
Footnote |
† Electronic supplementary information (ESI) available. See DOI: 10.1039/c9ra02267h |
|
This journal is © The Royal Society of Chemistry 2019 |
Click here to see how this site uses Cookies. View our privacy policy here.