DOI:
10.1039/C9RA01683J
(Paper)
RSC Adv., 2019,
9, 25022-25033
Retracted Article: Salidroside enhances the anti-cancerous effect of imatinib on human acute monocytic leukemia via the induction of autophagy-related apoptosis through AMPK activation
Received
6th March 2019
, Accepted 3rd June 2019
First published on 12th August 2019
Abstract
As the typical tyrosine kinase inhibitor, imatinib has been the first-line antineoplastic agent for both chronic myeloid leukemia and acute lymphoblastic leukemia. However, a large number of patients are still resistant to the benefits of imatinib, and they have a dissatisfactory prognosis. Salidroside, a compound that is extracted from natural plants, has been reported to have an excellent anticancer effect and few side effects. In the present study, we have developed a new combination therapy strategy of salidroside and imatinib for combating the growth of acute lymphoblastic leukemia. As demonstrated by the anti-proliferation assay, salidroside exhibited excellent cytotoxicity against myeloid leukemia cells. Moreover, cells treated by the combination therapy of salidroside and imatinib displayed a clear lower growth rate than cells only treated by imatinib, indicating that salidroside has a positive effect on enhancing the cytotoxicity of imatinib against leukemia cells. Subsequently, the underlying mechanisms were investigated. The results revealed that autophagy marker proteins in leukemia cells, including LC3, p62, and Beclin1, displayed a significant expression change after treating them with salidroside plus imatinib, with the levels of LC3 and Beclin1 dramatically increasing while the expression of p62 was significantly decreased. Moreover, an obvious down-regulation of p-PI3K, p-AKT and p-mTOR expression levels in leukemia cells after treatment with salidroside plus imatinib suggested that the PI3K/mTOR pathway plays an important role in the process of cell apoptosis induced by salidroside or imatinib. Further studies showed that pre-incubating the cells with an autophagy inhibitor dramatically inhibited the ability of imatinib to induce autophagy, but did not inhibit the ability of salidroside. The underlying causes were subsequently explored and the results showed that silencing AMPKα1, the most important regulator of autophagy, dramatically attenuates the ability of salidroside to induce cell apoptosis. These results together indicated that salidroside enhances the cytotoxicity of imatinib on acute monocytic leukemia via the induction of autophagy-related apoptosis through AMPK activation. The unique advantages of combination therapy were further confirmed by in vivo experiments, with the tumor-bearing cells treated with salidroside plus imatinib achieving the best anti-tumor effect.
Introduction
Acute myeloid leukemia (AML) is the hematological malignancy derived from the rapid and asexual growth of the myeloid lineage of blood cells.1 Although treatments of AML have made great progress in recent years, about 14
000 adults still suffer with AML and among them about 10
400 patients have died to date.1 Therapies for AML have been dramatically impaired by the serious resistance to most chemotherapeutics. Therefore, the selection of an appropriate antineoplastic agent and the improvement of the sensitivity of cancer cells to the chemotherapeutic drugs are critical to enhancing the treatment effect.
Imatinib is a typical tyrosine kinase inhibitor that selectively inhibits the tyrosine kinase activity of BCR/ABL.2 It has been developed as the first-line antineoplastic agent for both chronic myeloid leukemia and acute lymphoblastic leukemia.3 By selectively blocking the autophosphorylation and substrate phosphorylation of tyrosine kinase, imatinib has achieved excellent curative effects in clinical practice and has saved the lives of many patients who were suffering from the hematological malignancy.4,5 Unfortunately, with the increasing application of imatinib in clinics, the numbers of patients who are resistant to the therapy of imatinib are also upregulated, resulting in a dissatisfactory prognosis.6 The search for a novel treatment strategy is therefore necessary to address such an increasing serious issue.
To date, the exploration for novel drugs from natural plants has attracted increasing attention from researchers for their high biological activity and low toxicity.7 In addition, utilizing natural drugs to combat malignant diseases, especially cancer, is supposed to be economic and efficient.8 Salidroside, or p-hydroxyphenethyl-β-D-glucoside, is the main active ingredient of Rhodiola rosea L.8 A large number of studies have demonstrated that salidroside possesses a lot of pharmacological activity, such as anti-inflammatory, anti-aging, immune regulatory, neuroprotective protection, cardiovascular protection, and anti-tumor properties.9,10 Moreover, previous studies have pointed out that salidroside has a pivotal effect on the regulation of many signal paths that are extremely important to cell proliferation, apoptosis, and to the cell-cycle.11–13 Studies of the underlying mechanisms revealed that salidroside induced cell apoptosis might occur through the activation of AMP-activated protein kinase (AMPK)-based autophagy.14,15 As a conserved heterotrimeric protein kinase, AMPK plays an important role in the regulation of many cellular processes, including proliferation, apoptosis and differentiation.16–18 However, whether salidroside can be used to inhibit the growth and progress of acute myeloid leukemia is still unclear.
In the present study, we first explored the effects of salidroside on the proliferation and apoptosis of AML, to verify the anti-cancerous effect of such a natural drug. Subsequently, we developed a novel combination therapy strategy of salidroside and imatinib to enhance the anti-cancerous effect of imatinib on human acute monocytic leukemia. We speculated that the introduction of salidroside could significantly increase the sensitivity of leukemia cells to imatinib and result in stronger cytotoxicity of imatinib. For verification, the role of salidroside in combination with imatinib on the anti-tumor effect of AML was investigated. In addition, the potential mechanisms were further explored to clearly illustrate the effect of salidroside on enhancing the anti-tumor effect of imatinib.
Materials and methods
Cell culture and transfection
The human leukemia monocytic cell line (THP-1) and human histiocyte lymphoma cells (U937) were obtained from the American Type Culture Collection (ATCC, Manassas, VA, USA). All cell lines tested were mycoplasma-negative and were cultured in RPMI-1640, supplemented with 10% fetal bovine serum (FBS, Gibco, Grand Island, NY, USA) and 1% penicillin–streptomycin, at 37 °C in a humidified incubator with 5% CO2. Both cell lines were passaged 2–3 times a week once they reached ∼90% confluency.
The negative control genes (siNC) and AMPKα1 siRNAs (si-AMPKα1) were obtained from GenePharma Co., Ltd. (Shanghai, China), and the sequences of siNC and si-AMPKα1 are provided in Table 1. For cell transfection, the THP-1 cells were transplanted into a 6-well plate at a density of 5 × 105 cells per well. Then, the cells were respectively transfected with 50 nM siNC and 50 nM si-AMPKα1 using Lipofectamine 2000 (Invitrogen) under the guidelines of the manufacturer's instructions.
Table 1 Sequences of the primers in qRT-PCR assay
Primer |
Direction |
Sequence |
Bcl-2 |
F |
GCTCAGCCCTGTGCCACCTG |
R |
CAGAGGTCGCATGCTGGGGC |
Bax |
F |
ACTTCAACTGGGGCCGCGTC |
R |
GAGGCCTTCCCAGCCACCCT |
LC3 |
F |
GGTGGTCATATGCCGTCCGAGAAGACC |
R |
GGTGGTTGCTCTTCCGCAAGCCAGTGCTGTCCC |
P62 |
F |
TGTGTAGCGTCTGCGAGGGAAA |
R |
AGTGTCCGTGTTTCACCTTCCG |
Beclin 1 |
F |
CGGAATTCTATGGAAGGGTCTAAGACGTCC |
R |
CGGGATCCTCATTTGTTATAAAATTGTGAGGACA |
AMPKα1 |
F |
CAGATGGTGAATTTTTAAGAGATAGTTGTGGCTCACCCAACTATGC |
R |
GCATAGTTGGGTGAGCCACAACTATCTCTTAAAAATTCACCATCTG |
PI3K |
F |
CATCACTTCCTCCTGCTCTAT |
R |
CAGTTGTTGGCAATCTTCTTC |
AKT |
F |
GGACAACCGCCATCCAGACT |
R |
GCCAGGGACACCTCCATCTC |
mTOR |
F |
CGCTGTCATCCCTTTATCG |
R |
ATGCTCAAACACCTCCACC |
GAPDH |
F |
CGGAGTCAACGGATTTGGTCGTAT |
R |
AGCCTTCTCCATGGTGGTGAAGAC |
Ethical statement
Normal nude mice (male, 8–12 weeks) were obtained from Shanghai Sino-British Sippr/BK Lab Animal Ltd. (Shanghai, China). All of the experiments were performed in accordance with the rules of the animal ethics committee, and all animal procedures were approved by the Institutional Animal Care and Use Committee of Jiangsu Food and Pharmaceutical Science College.
Cytotoxicity of salidroside towards cancer cells
Cell viability of THP-1 and U937 cells after the treatment of salidroside was respectively determined using the previously reported MTT assay.19 In brief, THP-1 and U937 cells were respectively seeded into 96-well plates, with three replicates, and the cell density was 5 × 103 cells per well. After an overnight incubation period, the old medium was replaced with fresh and FBS-free RPMI-1640 containing salidroside. The concentrations of salidroside were 0.05, 0.1, 0.2, 0.5, 1, 2, 5, 10, 20, 50, 100, and 200 mM, respectively. Then the cells were left to incubate with salidroside for 48 h before the addition of 20 μL MTT (5 mg mL−1). For detection, 150 μL dimethyl sulfoxide was added into each well, followed by observation using a microplate reader at a wavelength of 570 nm. Subsequently, the cell viability of the THP-1 and U937 cells was calculated, and cells treated by a drug-free medium acted as a control. Importantly, all experiments were performed independently in triplicate.
Cell proliferation analysis
Proliferations of THP-1 and U937 cells were investigated using the MTT assay as above. After the cells were seeded into 96-well plates, as above, and incubated overnight, the old RPMI-1640 media was removed and supplemented with an equivalent volume of FBS-free medium containing 2 mM salidroside, 20 μM imatinib, and 2 mM salidroside + 20 μM imatinib, respectively. The cells treated by the drug-free medium were used as the control. After 48 h of incubation, 20 μL MTT was added into each well and subsequently incubated with the cells for 4 h. Finally, the plates were subject to cell viability detection using a Thermo Multiskan EX plate reader (Thermo Fisher, United Kingdom) at a wavelength of 450 nm.
Cell apoptosis assay
Both the THP-1 and U937 cells were respectively seeded into a 24-well plate at a density of 5 × 104 cells per well, followed by growth for 24 h. Then 1 mL of fresh and FBS-free RPMI-1640 containing 2 mM salidroside, 20 μM imatinib, and 2 mM salidroside + 20 μM imatinib, respectively, was added and incubated for 48 h. Subsequently, the medium containing the different drugs was removed and washed three times with PBS, followed by fixation with 4% formaldehyde for 15 min. Before the qualitative observation of the nuclear morphology under fluorescence microscopy, both cells were subjected to staining with Hoechst 33342 (Invitrogen) for 10 min.
Western blot assay
After treatment using various strategies as described above, the THP-1 cells were lysed using RIPA lysis buffer (100–200 μL lysate) for 5 min in an ice bath. Then the mixture was subjected to centrifugation for 30 min at 14
000 rpm and at 4 °C. The supernatant was subsequently collected and the concentration of the protein sample was quantitated by the BCA protein quantitative kit (Pierce, Rockford, IL, USA). After that, 30 μg of the protein sample was separated using 10% SDS-polyacrylamide gel electrophoresis (SDS-PAGE, 150 V, 90 min) and was transferred into a polyvinylidene difluoride membrane (PVDF, Millipore, Billerica, MA). Then the membranes were collected and blocked with 5% non-fat dried milk for 1 h at room temperature, followed by incubation with various primary antibodies at 4 °C. The primary antibodies included were rabbit-anti-GAPDH (1
:
2000; Abcam, ab8245), rabbit-anti-AMPKα1 (1
:
1000; Abcam, ab3759), rabbit-anti-t-PI3K (1
:
1000; Abcam, ab191606), rabbit-anti-p-PI3K (1
:
1000; Abcam, ab182651), rabbit-anti-t-AKT (1
:
1500; Abcam, ab179823), rabbit-anti-p-AKT (1
:
1000; Abcam, ab38449), rabbit-anti-t-mTOR (1
:
1500; Abcam, ab2732) and rabbit-anti-pmTOR (1
:
1000; Abcam, ab84400). After an overnight period of incubation, the membranes were washed three times with PBS and subsequently incubated with horseradish peroxidase labeled goat-anti-rabbit IgG (1
:
1000, LK2003L, Sungene Biotech Co., Ltd, China) under room temperature for 1 h. For detection of the protein levels, the enhanced chemiluminescence (ECL) substrate kit (Thermo scientific Pierce) was adopted and the Bio-Rad microscopic imaging system (Bio-Rad, Hercules, CA) was used to achieve the images. Most importantly, the detection assay was repeatedly performed three times.
Reverse transcription quantitative polymerase chain reaction (RT-qPCR)
All of the RNA of the cells and tumor tissues was collected using the RNeasy Mini RNA kit (Qiagen GmbH, Hilden, Germany) in accordance with the manufacturer's protocol. The concentration of the extracted RNA was determined using the BCA protein quantitative kit. Subsequently, the RNA was reversed transcribed into cDNA (Takara Biotechnology Co., Ltd., Dalian, China) through the oligo-dT method. For RT-qPCR, the SYBR green method was applied to the reaction system including 10 μL of 2 × SYBR Green Mix, 1.0 μL cDNA, 0.5 μL of the forward primer (10 μM), and 0.5 μL of the reverse primer (10 μM). Then the experiments were carried out under the conditions of a total of 40 cycles of 95 °C for 3 min, 95 °C for 15 s, 60 °C for 30 s, and finally 72 °C for 5 min. Of great importance, a dissolution curve was adopted to examine the reliability of the results and the relative expression of the target genes (2−ΔΔCt) was finally calculated by the relative quantitative method.
Immunofluorescence (IF) assay
The treated cells were fixed with 4% paraformaldehyde for 40 min at 4 °C and washed with 0.1% Tween 20 (Sigma). The fixed cells were blocked with 5% bovine serum albumin (BSA) for 15 min and incubated with anti-LC3 (1 μg mL−1, Abcam) at 4 °C overnight. After washing, the cells were incubated with donkey anti-goat IgG Alexa Fluor 488 (Invitrogen). The cell nuclei were stained with 4′,6-diamidino-2-phenylindole (DAPI, Fisher Scientific). A fluorescence microscope (Imager Z1) was used to obtain the results.
The anti-tumor effect in vivo
THP-1 tumor-bearing mice were established by an intraperitoneal injection with THP-1 cells, as previously reported.20 After 2 weeks, the tumor-bearing mice were randomly divided into four groups (n = 9) and respectively treated with saline, salidroside, imatinib, and salidroside plus imatinib. After a standard therapy procedure where a treatment of drugs was performed every two days in a week, the volumes of the tumors were carefully monitored and calculated with the following formula: volume = length × width2/2. After 21 days of observation, all the tumor tissues were collected and photographed. Subsequently, the tumor tissues were preserved for further investigations.
Haematoxylin and eosin (H&E) staining
After the animal experiments, the main organs (heart, liver, lung and kidney) of the mice were collected and embedded in paraffin, followed by preparation of slices with a thickness of 4 μm. Then the slices were fixed at 60 °C for 4 h and subsequently de-waxed with xylene and then dehydrated with gradient alcohol. Thereafter, hematoxylin (ZSGB-BIO) was adopted to stain the slices. After incubation for 2 min, the excess hematoxylin was rinsed using running water, followed by incubation with 1% hydrochloric acid for 5 s and treatment with 1% ammonia. Subsequently, the slices were stained with 0.1% eosin (Surgipath) for 30 min and dehydrated by gradient alcohol (80%, 90%, and 100%). Finally, the slices were transparentized by xylene and sealed in neutral gum for observation of the pathological changes under a light microscope.
Terminal deoxynucleotidyl transferase-mediated dUTP nick-end labeling (TUNEL) assay
After receiving various treatments, the THP-1 cancer-bearing mice were sacrificed and all tumor tissues were collected. Then the Situ Cell Death Detection Kit (R&D Systems, USA) was used to determine cell apoptosis. According to the manufacturer's instructions, the obtained tumor tissues were developed into 10 μm paraffin sections, followed by incubation with a proteinase K working solution for 30 min at 37 °C. Thereafter, the sections were washed with PBS three times before permeabilization with 0.1% Triton X-100. Finally, fluorescein-12-dUTP was adopted to visualize the apoptotic cells and the results were obtained using fluorescence microscopy (Zeiss Axiovert 100 M, Carl Zeiss, Germany).
Immunohistochemistry (IHC) assay
The obtained tumor tissues were fixed in 4% formalin for 1 h followed by embedding in paraffin. Then all the tumor tissues were cut into 4 μm thick sections. After dewaxing and rehydrating, all sections were incubated with 10 mM citrate buffer for 5 min at 100 °C and blocked with 10% FBS for 2 h at room temperature. Subsequently, the sections were incubated with anti-PCNA (1
:
25, Abcam, ab18197) and anti-Ki67 (1
:
25, Abcam, ab833) overnight at 4 °C. After washing with PBS, the sections were incubated with an anti-rabbit secondary antibody (Abcam, ab150077) for 2 h. Finally, the results were observed and photographed using an optical microscope.
Statistical analysis
All data are expressed as a mean ± standard deviation of three repeated experiments. All results were calculated using SPSS 13.0 (IBM, Armonk, NY, USA) with the Student's t test. P < 0.05 indicated a statistically significant result.
Results
Determination of the ratio of salidroside and imatinib in a combination therapy strategy
To explore an appropriate treatment dose of salidroside and imatinib, the THP-1 and U937 cells were respectively exposed to different concentrations of salidroside and imatinib. After 48 h of treatment, the cell viability was determined with a CCK8 kit. As shown in Fig. 1B, when the concentration of salidroside was above 2 mM, the cell viabilities of the THP-1 cells and the U937 cells were significantly down-regulated and exhibited a dose-dependent manner. In contrast, the cell viabilities of both cells did not decrease in a clear manner when the concentration of salidroside was below 2 mM. In this case, the concentration of salidroside was set at 2 mM.
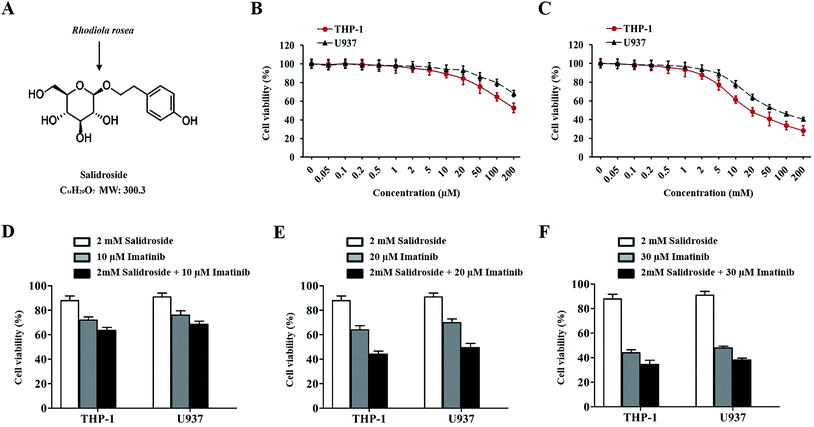 |
| Fig. 1 Cell viabilities of THP-1 and U937 cells after receiving therapy with salidroside, imatinib, or the combination therapy strategy. (A) Salidroside exists in Rhodiola rosea, and the molecular structure is shown. THP-1 and U937 cells were treated with different concentrations of imatinib (B) and salidroside (C) for 48 h, and the cell viability was detected by a CCK-8 assay. For further determination of the ratio of salidroside and imatinib in the combination therapy strategy, both THP-1 and U937 cells were treated by various combination therapy strategies: 2 mM salidroside + 10 μM imatinib (D), 2 mM salidroside + 20 μM imatinib (E), and 2 mM salidroside + 30 μM imatinib (F). | |
For imatinib, only the THP-1 cells or the U937 cells treated with a concentration of 10 μM or above of imatinib exhibited a significant down-regulation of the cell viabilities (Fig. 1C). For further determination of the ratio of salidroside and imatinib in the combination therapy strategy, both THP-1 and U937 cells were treated with various combination therapy strategies: 2 mM salidroside + 10 μM imatinib, 2 mM salidroside + 20 μM imatinib, and 2 mM salidroside + 30 μM imatinib. As the results show in Fig. 1D and E, only the cells treated by 2 mM salidroside + 20 μM imatinib exhibited a significant advantage compared with the monotherapy of salidroside or imatinib for the down-regulation of cell viabilities of the THP-1 cells and U937 cells (Fig. 1D and E). Taking these results together, the treatment dose of PMS and SOR for cell therapy was set at 2 mM and the dose of imatinib was set at 20 μM.
Salidroside significantly enhances the cytotoxicity of imatinib towards THP-1 cells and U937 cells
As confirmed by the results shown in Fig. 2A, when compared with salidroside and control groups, imatinib exhibited an obvious superior inhibition effect on the growth of leukemia cells (THP-1). However, after the THP-1 cells received the combination therapy of salidroside and imatinib, the inhibition effect on the proliferation of leukemia cells was further dramatically enhanced. The excellent effect of the combination therapy of salidroside and imatinib was also demonstrated on the U937 cells, where the proliferation ability of the cells co-incubated with salidroside and imatinib was markedly decreased compared with those cells undergoing the individual therapy (P < 0.05). For a cell apoptosis evaluation, the morphologies of the cell nuclei were determined after receiving the different treatments. As shown in Fig. 2B and C, the cells treated by salidroside or imatinib exhibited more fragments than those cells in the control group. Additionally, the nuclei of both the THP-1 cells and the U937 cells that were treated by salidroside + imatinib displayed the most nuclei fragments with unsharp borders.
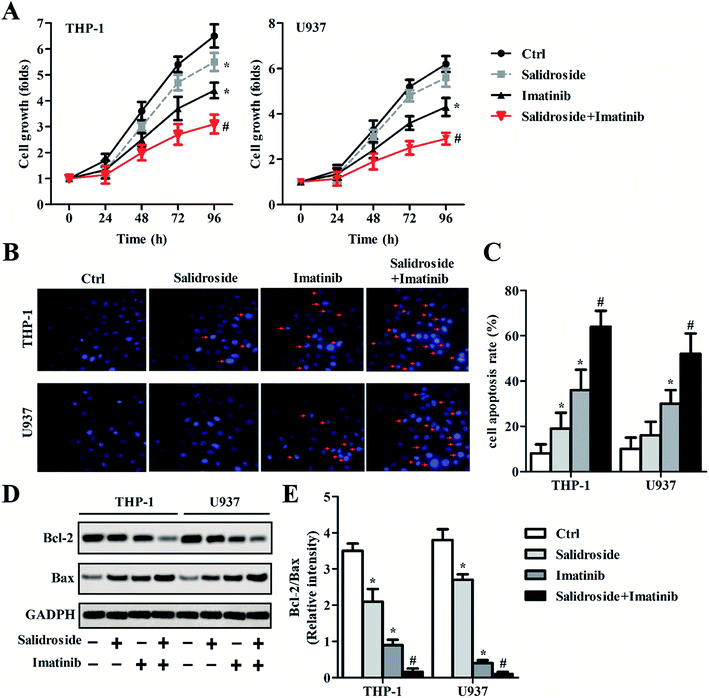 |
| Fig. 2 Salidroside combined with imatinib inhibits proliferation and promotes apoptosis in AML cells. THP-1 and U937 cells were treated with PBS (control), 2 mM salidroside, 20 μM imatinib, and 2 mM salidroside + 20 μM imatinib, respectively. (A) CCK-8 assays were performed to measure the proliferation abilities of THP-1 and U937 cells (*P < 0.05 vs. control group; #P < 0.05 vs. salidroside group). (B and C) Cell apoptosis was examined by Hoechst staining, and the cell apoptosis rate was quantitatively analyzed (*P < 0.05 vs. control group; #P < 0.05 vs. salidroside group). (D) Bcl-2 and Bax expressions were examined by Western blot assay, and the protein quantification was analyzed according to the results of an RT-qPCR assay (E). GAPDH was used as the internal reference (*P < 0.05 vs. control group; #P < 0.05 vs. salidroside group). | |
We subsequently explored whether the salidroside or imatinib inhibited cell growth through regulating the balance between Bcl-2 and Bax expression, which plays a significant role in regulating apoptosis of cells. The results from the Western blot assay (Fig. 2D) showed that the levels of Bc1-2, an apoptotic inhibitor, were significantly down-regulated in the cells that were treated by salidroside or imatinib compared with the control cells. However, in contrast to the Bc1-2 expression, the levels of Bax, a pro-apoptotic factor, were clearly higher in both THP-1 and U937 cells that were incubated with salidroside or imatinib than in the cells of the control group. Moreover, when the cells were treated with a combination therapy of salidroside and imatinib, the expression of Bax in both THP-1 and U937 cells was further dramatically elevated, while the expression of Bcl-2 decreased to its lowest levels. These results were further confirmed by qRT-PCR experiments, and the ratio of Bcl-2 to Bax in the cells was also calculated. As shown in Fig. 2E, the cells receiving the combination therapy exhibited the lowest value of Bcl-2/Bax compared with that of the cells of other groups, indicating that the pro-apoptotic factor Bax has higher expression than the apoptotic inhibitor Bcl-2. Taking the above results together, we can draw the conclusion that the salidroside or the imatinib induces cell apoptosis by regulating the expression balance between Bcl-2 and Bax.
Salidroside induces cell apoptosis by improving the activity of the autophagy system
To verify whether the autophagy system was involved during the process of cell apoptosis induced by salidroside or imatinib, related genes were detected through a Western blot assay and RT-qPCR experiments. As shown in Fig. 3A and B, the data demonstrated that p62 expression was significantly down-regulated, while the levels of Beclin1 and LC3II were significantly elevated in the cells treated by salidroside or imatinib. Additionally, when compared with those cells treated by monotherapy of salidroside or imatinib, the cells co-incubated with salidroside plus imatinib expressed lower levels of p62 and higher levels of Beclin1 and LC3II. We subsequently investigated the role of the AMPK-mTOR pathway in the activation of the drug-induced autophagy system. As demonstrated in Fig. 3C and D, significant up-regulation of AMPKα1 levels in the salidroside- or imatinib-treated cells resulted in clear lower expression of p-PI3K, p-AKT and p-mTOR than in the control cells. Moreover, similar to the above, the highest levels of AMPKα1 were observed in the cells treated with the combination therapy, while the lowest expression levels of p-PI3K, p-AKT and p-mTOR were simultaneously detected. By combining these results, it was confirmed that cell apoptosis induced by salidroside or imatinib was through activation of the autophagy system.
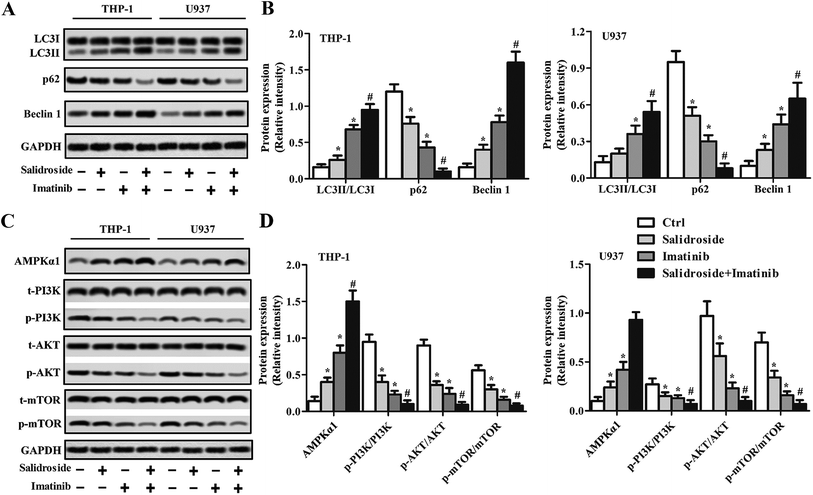 |
| Fig. 3 Salidroside combined with imatinib induces autophagy in AML in vitro. THP-1 and U937 cells were treated with PBS (control), 2 mM salidroside, 20 μM imatinib, and 2 mM salidroside + 20 μM imatinib, respectively. (A) The protein expression levels of LC3, P62 and Beclin 1 were analyzed by Western blot assay in THP-1 and U937 cells, and the expression levels were quantitatively analyzed by RT-qPCR experiments (B) (*P < 0.05 vs. control group; #P < 0.05 vs. salidroside group). (C) The protein expression levels of AMPKα1, t-PI3K, p-PI3K, t-AKT, p-AKT, t-mTOR, and p-mTOR were analyzed by Western blot assay in the THP-1 and U937 cells, and the expression levels were quantitatively analyzed by RT-qPCR experiments (D) (*P < 0.05 vs. control group; #P < 0.05 vs. salidroside group). | |
An autophagy inhibitor significantly decreases the cytotoxicity of drugs against cancer cells
As preliminarily demonstrated above, the AMPK-mTOR pathway-mediate autophagy plays a key role in the regulation of salidroside- or imatinib-induced apoptosis of both THP-1 cells and U937 cells. For further confirmation, the THP-1 cells were adopted for subsequent experiments and were co-treated with an autophagy inhibitor, 3-MA. As shown in Fig. 4A and B, in the cells incubated with salidroside or imatinib and without treatment with 3-MA, the expression of p62 was significantly down-regulated, while the levels of Beclin1 and LC3II were clearly elevated. However, when these cells were co-incubated with 3-MA, the expression levels of LC3II, p62, and Beclin1 all returned to the expression levels similar to the control group. More importantly, when compared with the cells treated by imatinib plus 3-MA, the cells treated with the combination therapy of salidroside + imatinib + 3-MA expressed dramatically higher levels of LC3II and lower levels of p62 and Beclin1, suggesting that the existence of salidroside markedly sealed the autophagy inhibition effect of 3-MA. Further immunofluorescence tests revealed that the cells treated with salidroside or imatinib facilitated the activation of autophagy with a higher level of LC3II expression compared with the untreated cells. Similar to the above, the autophagy inhibition effect of 3-MA could be opposed by salidroside as a stronger fluorescence intensity was observed when compared with the cells treated by 3-MA plus imatinib (Fig. 4C and D).
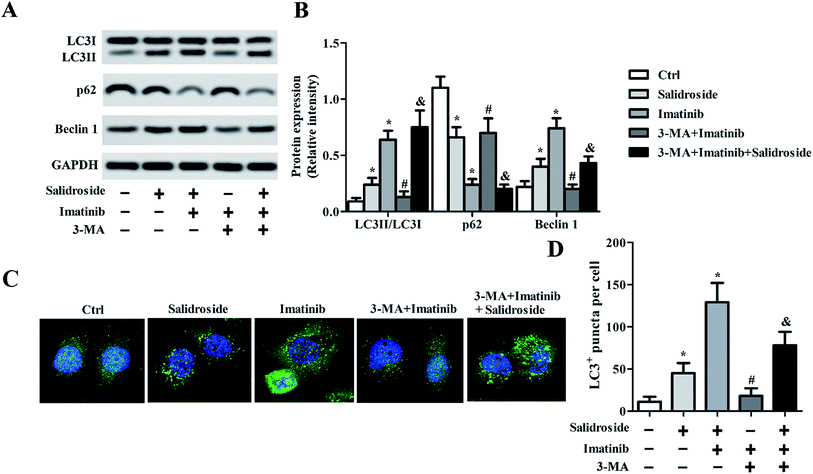 |
| Fig. 4 3-MA relieves the induction of salidroside in combination with imatinib on AML autophagy in vitro. THP-1 cells were treated with PBS (control), 2 mM salidroside, 2 mM salidroside + 20 μM imatinib, 3-MA + 20 μM imatinib, and 3-MA + 20 μM imatinib + 2 mM salidroside, respectively. (A) LC3, P62 and Beclin 1 expressions were assessed by Western blot assay in THP-1 and U937 cells, and the expression levels were quantitatively analyzed by RT-qPCR experiments (B) (*P < 0.05 vs. control group; #P < 0.05 vs. salidroside group, & vs. 3-MA + imatinib group). (C and D) LC3 expression was detected by an immunofluorescence (IF) assay (*P < 0.05 vs. control group; #P < 0.05 vs. imatinib group, and vs. 3-MA + imatinib group). | |
Silence of AMPKα1 dramatically attenuates cell apoptosis induced by salidroside
To clearly illustrate the role of AMPKα1 in regulating salidroside-induced cell apoptosis, part of the THP-1 cells was transfected with si-AMPKα1 for AMPKα1 silencing. The gene silence efficiency was first determined by Western blot assay and RT-qPCR experiments. As demonstrated in Fig. 5A and B, after the THP-1 cells were transfected with si-AMPKα1, the expression of AMPKα1 was dramatically down-regulated to an extremely low level. Then the AMPKα1-silenced cells were treated with various treatment strategies, followed by detection of the autophagy marker protein LC3II. As revealed in Fig. 5C and D, in the cells transfected with si-Ctrl, a similar expression level of LC3II was observed to the above. Nevertheless, when the cells were treated with si-AMPKα1, salidroside was not superior to the control group and the co-incubated cells with salidroside plus imatinib showed a similar expression level of LC3II to the cells treated only with imatinib. Subsequently, we adopted the AMPKα1-silenced cells to evaluate the anti-tumor effect of salidroside and imatinib. As shown in Fig. 5E and F, si-Ctrl transfected cells treated with salidroside plus imatinib displayed the lowest cell survival rate and highest cell apoptosis rate. Of great importance, the silencing of AMPKα1 significantly decreased the capacity of salidroside induced apoptosis and anti-growth of the THP-1 cells.
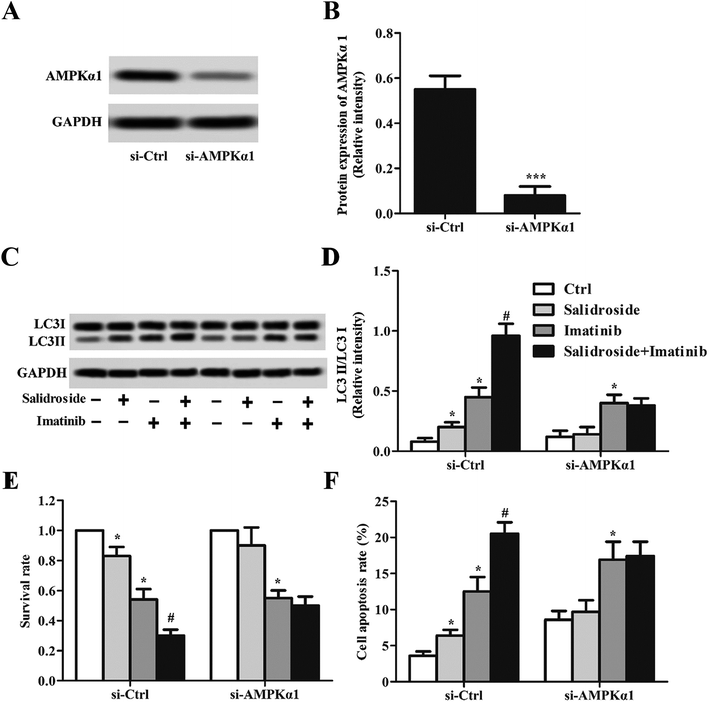 |
| Fig. 5 Silencing of AMPKα1 attenuates the induction of salidroside in combination with imatinib on AML autophagy in vitro. THP-1 cells were transfected with a control (si-Ctrl) and with AMPKα1 siRNAs (si-AMPKα1), respectively. AMPKα1 expression was evaluated by Western blot assay (A), and the relative expression level was recorded by RT-qPCR experiments (B) (***P < 0.001). THP-1 cells were treated with PBS (control), 2 mM salidroside, 20 μM imatinib, and 2 mM salidroside + 20 μM imatinib, respectively. After treatment, the cells were transfected with a control (si-Ctrl) and with AMPKα1 siRNAs (si-AMPKα1), respectively. AMPKα1 expression was assessed by Western blot assay (C), and the relative intensity of LC3II/LC3I was recorded by RT-qPCR experiments (D) (*P < 0.05 vs. control group; #P < 0.05 vs. salidroside group). (E) Cell proliferation was measured by the CCK-8 assay in THP-1 cells treated as in B (*P < 0.05 vs. control group; #P < 0.05 vs. salidroside group). (F) Cell apoptosis was detected by Hoechst staining in THP-1 cells treated as in B (*P < 0.05 vs. control group; #P < 0.05 vs. salidroside group). | |
Combination therapy of salidroside and imatinib inhibits the growth of THP-1 tumors
As shown in Fig. 6A, the photographs exhibited that the mice treated with imatinib but not salidroside exhibited significant tumor growth inhibition. However, the tumors of the mice co-injected with salidroside and imatinib had the smallest volumes when compared with the other groups. Such results were further confirmed by the tumor growth curves, with the mice that received the combination therapy of salidroside and imatinib exhibiting the slowest tumor growth rate at almost all of the time points (Fig. 6B). Subsequently, the toxicity of various treatment strategies against normal organs was studied. As shown in Fig. 6A, the results of H&E staining displayed that no obvious pathological changes could be detected for the main organs after receiving the various treatments, compared with the control groups. These results indicated that the adopted combination therapy of salidroside and imatinib did not change the pathological features of the main organs and is safe enough for further clinical applications.
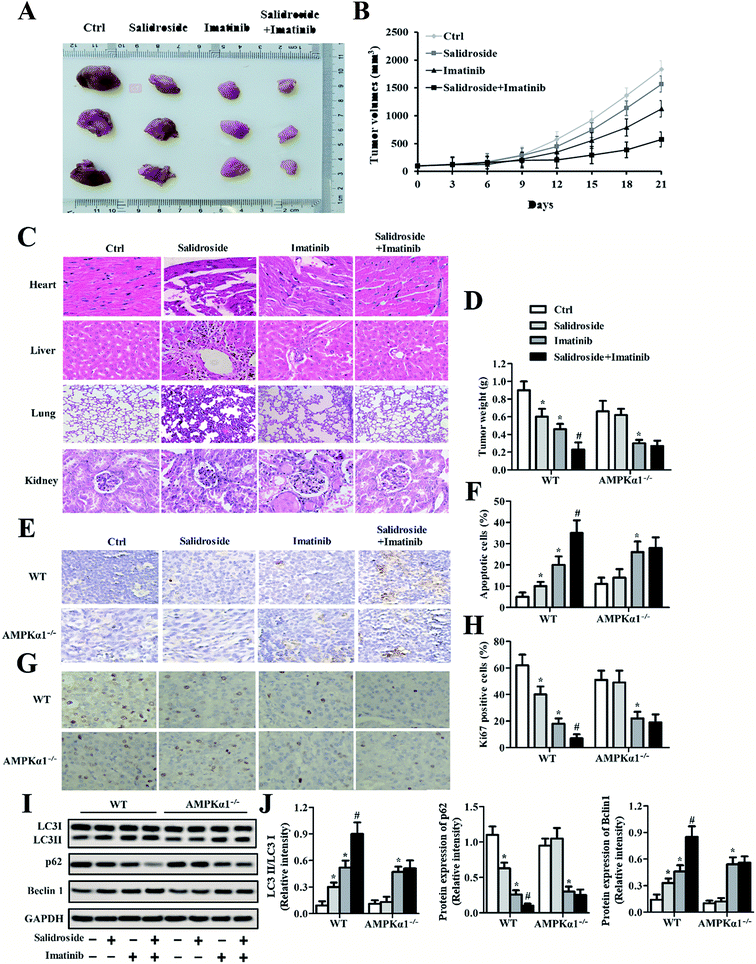 |
| Fig. 6 Salidroside in combination with imatinib promotes the anti-tumor effect of AML in vivo. (A) Photographs of tumor and (B) tumor growth curves after treatment with various strategies. (C) The heart, liver, lung and kidney sections of the treated mice were used in an HE-staining assay. The magnification was 400× for heart, 400× for liver, 400× for lung and 200× for kidney. (D) The tumor weight, (E and F) the number of apoptotic cells (TUNEL assay), and (G and H) Ki67 expression (IHC assay) were measured in wild type mice and in AMPKα1 knockout mice (*P < 0.05 vs. control group; #P < 0.05 vs. salidroside group). (I) LC3, P62 and Beclin 1 expression levels were analyzed by Western blot assay and RT-qPCR experiments (J) in WT mice and in AMPKα1 knockout mice, and the levels were also quantitatively analyzed (*P < 0.05 vs. control group; #P < 0.05 vs. salidroside group, and vs. 3-MA + imatinib group). | |
We further investigated whether the AMPKα1-dependent autophagy was significant to the salidroside-induced tumor growth inhibition. After treatment as above, all the tumor tissues were collected, followed by an evaluation of their weight. As shown in Fig. 6D, the mice that received the combination therapy exhibited the lowest tumor weights when compared with the mice treated by monotherapy of salidroside or imatinib. Importantly, all the tumor weights of the mice injected with drugs were significantly lower than that of the mice treated by saline. However, for the AMPKα1-silenced tumor-bearing mice, the anti-tumor effect of salidroside was dramatically inhibited, indicating that AMPKα1 plays a pivotal role in the process of salidroside-induced cell apoptosis.
Salidroside heightens the anti-tumor effect of imatinib by the activation of autophagy
The apoptosis of tumor cells was measured by the TUNEL assay. As shown in Fig. 6E and F, qualitative analysis revealed that salidroside notably enhanced the cytotoxicity of imatinib towards tumor tissues and such an effect could be markedly eliminated by AMPKα1 silencing. Moreover, in the salidroside or imatinib treated groups, expression of the cell proliferation-related gene Ki67 was dramatically down-regulated compared with the expression in the control groups. For the tissues treated by salidroside plus imatinib, similar results were obtained with the improvement in the inhibition effect of salidroside being impaired by AMPKα1 silencing (Fig. 6G and H). To confirm the role of autophagy in the process of cell apoptosis induced by salidroside, the autophagy-related proteins (LC3II, Beclin 1, and p62) in the tumor tissues were further determined. As demonstrated in Fig. 6I and J, the positive regulation genes (LC3II and Beclin 1) clearly showed higher expression in the tumors treated by salidroside plus imatinib. In contrast, for the negative regulation gene p62, its levels in the tumor tissues were notably decreased post treatment with the various drugs. Of great importance, the silencing of AMPKα1 exerted an extremely adverse influence on the anti-tumor effect of salidroside.
Discussion
Leukemia is the most commonly diagnosed type of cancer in children aged 0–14 years and accounts for about 35% of the total number of cancers.21,22 However, treating leukemia is still seriously hindered by the lack of appropriate antineoplastic agents and the low sensitivity of leukemia cells to chemotherapeutical drugs.23 Imatinib, a small molecule Bcr-Abl tyrosine kinase inhibitor, has been recommended as the criterion drug for leukemia treatment.4 However, there are still a large number of leukemia patients that are resistant to imatinib therapy due to the low sensitivity of cancer cells to the drug.6 In this case, novel treatment strategies are urgently needed to address the issue.
Salidroside represents a typical natural drug that is derived from natural plants and is widely used in the fields of anti-inflammation, immune regulation, neuroprotective and cardiovascular protection, and anti-tumor treatment.24 Many studies have stated that salidroside inhibits proliferation and induces apoptosis of cancer cells through activation of the AMPK-based signal pathway.25–27 However, few reports have indicated that salidroside could inhibit the growth and progress of leukemia. In the present study, we have demonstrated that salidroside holds great potential in the anti-growth of leukemia since it exhibited satisfactory cytotoxicity against leukemia cells (both THP-1 cells and U937 cells).
Based on the ideal cytotoxicity of salidroside, we further speculated that the co-incubation of leukemia cells with salidroside could enhance the anti-cancerous effect of imatinib. For verification, a novel combination therapy strategy of salidroside and imatinib was developed and subjected to evaluation of cell proliferation inhibition. By comparison between the cells treated with different strategies, we found that the cells that received the combination therapy of salidroside and imatinib displayed a clear lower rate of cell growth than the cells treated by monotherapy of salidroside or imatinib. Moreover, the cells co-incubated with salidroside plus imatinib exhibited a number of nuclei fragments through significant down-regulation of the levels of Bc1-2 (apoptotic inhibitor) and an increase in the levels of Bax (pro-apoptotic factor).
Although the effect of salidroside on enhancing the anti-leukemia effect of imatinib has been demonstrated here, the underlying mechanisms are still unclear. As a mechanism of intracellular defense and stress regulation, autophagy is an important material metabolism pathway that plays a significant role in regulation of proliferation, apoptosis, and differentiation of many kinds of cancer cells.28–30 The activation of autophagy is regulated by several genes and key protein complexes, such as the mammalian target of rapamycin (mTOR), phosphatidylinositol 3 kinase (PI3K), Atg8 (LC3) and Atg6 (Beclin-1).31,32 As confirmed in our study, when compared with the leukemia cells only treated by imatinib, the cells co-incubated with salidroside plus imatinib displayed a significant decrease in p62 expression, while showing a dramatic elevation in LC3II and Beclin-1 expression levels. Additionally, pre-incubation of cells with an autophagy inhibitor (3-MA) could notably reverse the expressions of p62, LC3II, and Beclin-1, regulated by imatinib. However, the addition of 3-MA did not affect the changes in the levels of the autophagy-related genes in cells treated by the combination therapy of salidroside and imatinib. Based on this, we can preliminarily conclude that salidroside inhibited leukemia cell growth by activation of autophagy and through a different mechanism than that used by imatinib.
Multiple signaling pathways have been demonstrated to play a significant role in the regulation of autophagy and they can be divided into two categories: mTOR-based and mTOR-independent pathways.33,34 Among these pathways, the PI3K-Akt-mTOR pathway is the only inhibitor of the autophagy process, while the AMPK-mTOR signaling pathway is the initiator.35 Activation and phosphorylation of AMPK can cause the related-mTOR site to be phosphorylated and the biological activity of mTOR is then significantly decreased.36 Finally, the phosphorylations of p70S6K and 4E-BP1, which are important to the autophagy inhibition, are dramatically decreased.36
In our study, we have proved that salidroside alone or imatinib alone significantly down-regulate p62, p-PI3K, p-AKT and p-mTOR expressions, and significantly up-regulate Beclin1, LC3II and AMPKα1 expressions, and that there is also cooperation between salidroside and imatinib on the regulations of autophagy associated proteins. Therefore, we have suggested that salidroside combined with imatinib induces AML autophagy in vitro. In addition, we also proved that salidroside or imatinib induces AML cell autophagy, and the autophagy inhibitor 3-MA or the silencing of AMPKα1 attenuates the effect of autophagy mediated by imatinib, suggesting that 3-MA or silencing AMPKα1 relieves the induction of salidroside or imatinib on AML autophagy in vitro. Furthermore, we also demonstrated that salidroside or imatinib significantly up-regulates LC3 and Beclin 1 expressions, and down-regulates P62 expression, and that there is cooperation between salidroside and imatinib, suggesting that salidroside enhances the anti-cancerous effect of imatinib on AML via the induction of autophagy-related apoptosis in vivo.
In summary, we have indicated that salidroside or imatinib inhibits AML cell proliferation, and promotes apoptosis; there is also cooperation between salidroside and imatinib in AML proliferation and apoptosis in vitro and in vivo. In addition, we proved that salidroside combined with imatinib induces AML autophagy in vitro and in vivo. Therefore, we have suggested that salidroside enhances the anti-cancerous effect of imatinib on AML via the induction of autophagy-related apoptosis through AMPK activation.
Conflicts of interest
The authors declare no competing financial interests.
Abbreviations
AML | Acute myeloid leukemia |
THP-1 | Human leukemia monocytic cell line |
U937 | Human histiocyte lymphoma cells |
AMPK | AMP-activated protein kinase |
IHC | Immunohistochemistry |
TUNEL | Terminal deoxynucleotidyl transferase-mediated dUTP nick-end labelling |
H&E | Haematoxylin and eosin |
RT-qPCR | Reverse transcription quantitative polymerase chain reaction |
Acknowledgements
The present research is supported by the subjects of the Major University Science Research Project of Jiangsu Province (18KJA36002). This study was also supported by the Huai’an Science and Technology Poverty Alleviation Project (HAN201801).
References
- T. J. Ley, E. R. Mardis, L. Ding, B. Fulton, M. D. McLellan and K. Chen, et al., DNA sequencing of a cytogenetically normal acute myeloid leukaemia genome, Nature, 2008, 456, 66–72 CrossRef CAS PubMed.
- C. Gambacorti-Passerini and R. Piazza, Imatinib--A New Tyrosine Kinase Inhibitor for First-Line Treatment of Chronic Myeloid Leukemia in 2015, JAMA Oncol., 2015, 1, 143–144 CrossRef PubMed.
- P. Ganesan, R. Rajendranath, V. Kandakumar and T. G. Sagar, Treatment of chronic phase chronic myeloid leukemia with imatinib, Indian J. Pediatr., 2015, 82, 235–239 CrossRef PubMed.
- T. P. Hughes, G. Saglio, A. Quintás-Cardama, M. J. Mauro, D. W. Kim and J. Lipton, et al., BCR-ABL1 mutation development during first-line treatment with dasatinib or imatinib for chronic myeloid leukemia in chronic phase, Leukemia, 2015, 29, 1832–1838 CrossRef CAS PubMed.
- M. Miura, Therapeutic drug monitoring of imatinib, nilotinib, and dasatinib for patients with chronic myeloid leukemia, Biol. Pharm. Bull., 2015, 38, 645–654 CrossRef CAS PubMed.
- F. Castagnetti, G. Gugliotta, M. Breccia, F. Stagno, A. Iurlo and F. Albano, et al., Long-term outcome of chronic myeloid leukemia patients treated frontline with imatinib, Leukemia, 2015, 29, 1823–1831 CrossRef CAS PubMed.
- S. Guan, J. He, W. Guo, J. Wei, J. Lu and X. Deng, Adjuvant effects of salidroside from Rhodiola rosea L. on the immune responses to ovalbumin in mice, Immunopharmacol. Immunotoxicol., 2011, 33, 738–743 CrossRef CAS PubMed.
- F. Titomanlio, M. Perfumi and L. Mattioli, Rhodiola rosea L. extract and its active compound salidroside antagonized both induction and reinstatement of nicotine place preference in mice, Psychopharmacology, 2014, 23, 2077–2086 CrossRef PubMed.
- Z. Q. Qu, Y. Zhou, Y. S. Zeng, Y. K. Lin, Y. Li and Z. Q. Zhong, et al., Protective effects of a Rhodiola crenulata extract and salidroside on hippocampal neurogenesis against streptozotocin-induced neural injury in the rat, PLoS One, 2012, 7, e29641 CrossRef CAS PubMed.
- C. B. Tan, M. Gao, W. R. Xu, X. Y. Yang, X. M. Zhu and G. H. Du, et al., Protective effects of salidroside on endothelial cell apoptosis induced by cobalt chloride, Biol. Pharm. Bull., 2009, 32, 1359–1363 CrossRef CAS PubMed.
- D. Gui, Z. Cui, L. Zhang, C. Yu, D. Yao and M. Xu, et al., Salidroside attenuates hypoxia-induced pulmonary arterial smooth muscle cell proliferation and apoptosis resistance by upregulating autophagy through the AMPK-mTOR-ULK1 pathway, BMC Pulm. Med., 2017, 17, 191 CrossRef PubMed.
- X. Zhuang, A. Maimaitijiang, Y. Li, H. Shi and and X. Jiang, Salidroside inhibits high-glucose induced proliferation of vascular smooth muscle cells via inhibiting mitochondrial fission and oxidative stress, Exp. Ther. Med., 2017, 14, 515–524 CrossRef CAS PubMed.
- X. Chen, Y. Wu, T. Yang, M. Wei, Y. Wang and X. Deng, et al., Salidroside alleviates cachexia symptoms in mouse models of cancer cachexia via activating mTOR signaling, J. Cachexia Sarcopenia Muscle, 2016, 7, 225–232 CrossRef PubMed.
- H. Li and C. Chen, Inhibition of autophagy enhances synergistic effects of salidroside and anti-tumor agents against colorectal cancer, BMC Complementary Altern. Med., 2017, 17, 538 CrossRef PubMed.
- L. Ju, X. Wen, C. Wang, Y. Wei, Y. Peng, Y. Ding, L. Feng and L. Shu, et al., Salidroside, A Natural Antioxidant, Improves β-Cell Survival and Function via Activating AMPK Pathway, Front. Pharmacol., 2017, 8, 749 CrossRef PubMed.
- D. Grahame Hardie, AMP-activated protein kinase: a key regulator of energy balance with many roles in human disease, J. Intern. Med., 2014, 276, 543–559 CrossRef CAS PubMed.
- D. S. Novikova, A. V. Garabadzhiu, G. Melino, N. A. Barlev and V. G. Tribulovich, AMP-activated protein kinase:
structure, function, and role in pathological processes, Biochemistry, 2015, 80, 127–144 CAS.
- E. Sarnowska, A. Balcerak, M. Olszyna-Serementa, D. Kotlarek, T. J. Sarnowski and J. A. Siedlecki, et al., AMP-activated protein kinase (AMPK) as therapeutic target, Postepy Hig. Med. Dosw., 2013, 67, 750–760 CrossRef.
- X. Feng, J. Yao, X. Gao, Y. Jing, T. Kang and D. Jiang, et al., Multi-targeting Peptide-Functionalized Nanoparticles Recognized Vasculogenic Mimicry, Tumor Neovasculature, and Glioma Cells for Enhanced Anti-glioma Therapy, ACS Appl. Mater. Interfaces, 2015, 7, 27885–27899 CrossRef CAS PubMed.
- R. Deng R, F. Y. Fan, H. Yi, F. Liu, G. C. He and H. P. Sun, et al., PD-1 blockade potentially enhances adoptive cytotoxic T cell potency in a human acute myeloid leukaemia animal model, Hematology, 2018, 23, 740–746 CrossRef PubMed.
- A. Schalhorn, Leukemia, MMW Fortschr. Med., 2013, 155, 48–52 CrossRef PubMed.
- G. Juliusson and R. Hough, Leukemia, Prog. Tumor Res., 2016, 43, 87–100 Search PubMed.
- S. P. Zhang, Y. N. Niu, N. Yuan, A. H. Zhang, D. Chao and Q. P. Xu, et al., Role of autophagy in acute myeloid leukemia therapy, Chin. J. Cancer, 2013, 32, 130–135 CrossRef PubMed.
- X. W. Wu, Y. S. Peng and R. F. Wang, Research progress of alternative production approaches of salidroside, Zhongguo Zhongyao Zazhi, 2013, 38, 3656–3660 CAS.
- D. S. Novikova, A. V. Garabadzhiu, G. Melino, N. A. Barlev and V. G. Tribulovich, et al., AMP-activated protein kinase: structure, function, and role in pathological processes, Biochemistry, 2015, 80, 127–144 CAS.
- E. Sarnowska, A. Balcerak, M. Olszyna-Serementa, D. Kotlarek, T. J. Sarnowski and J. A. Siedlecki, et al., AMP-activated protein kinase (AMPK) as therapeutic target, Postepy Hig. Med. Dosw., 2013, 67, 750–760 CrossRef.
- B. Faubert B, E. E. Vincent, M. C. Poffenberger and R. G. Jones, The AMP-activated protein kinase (AMPK) and cancer: many faces of a metabolic regulator, Cancer Lett., 2015, 356, 165–170 CrossRef PubMed.
- E. White, J. M. Mehnert and C. S. Chan, Autophagy, Metabolism, and Cancer, Clin. Cancer Res., 2015, 21, 5037–5046 CrossRef CAS PubMed.
- E. White, The role for autophagy in cancer, J. Clin. Invest., 2015, 125, 42–46 CrossRef PubMed.
- N. Hasima and B. Ozpolat, Regulation of autophagy by polyphenolic compounds as a potential therapeutic strategy for cancer, Cell Death Dis., 2014, 5, e1509 CrossRef CAS PubMed.
- M. ZoU, N. Lu, C. Hu, W. Liu, Y. Sun and X. Wang, et al., Beclin 1-mediated autophagy in hepatocellular carcinoma cells: implication in anticancer efficiency of oroxylin A via inhibition of mTOR signaling, Cell. Signalling, 2012, 24, 1722–1732 CrossRef CAS PubMed.
- X. Ou, M. R. Lee, X. Huang, S. Messina-Graham and H. E. Broxmeyer, et al., SIRT1 positively regulates autophagy and mitochondria function in embryonic stem cells under oxidative stress, Stem Cells, 2014, 32, 1183–1194 CrossRef CAS PubMed.
- L. Chang, P. H. Graham, J. Hao, J. Ni, J. Bucci and P. J. Cozzi, et al., PI3K/Akt/mTOR pathway inhibitors enhance radiosensitivity in radioresistant prostate cancer cells through inducing apoptosis, reducing autophagy, suppressing NHEJ and HR repair pathways, Cell Death Dis., 2014, 5, 1437 CrossRef PubMed.
- E. A. Dunlop and A. R. Tee, mTOR and autophagy: a dynamic relationship governed by nutrients and energy, Semin. Cell Dev. Biol., 2014, 36, 121–129 CrossRef CAS PubMed.
- D. Heras-Sandoval, J. M. Pérez-Rojas, J. Hernández-Damián and J. Pedraza-Chaverri, et al., The role of PI3K/AKT/mTOR pathway in the modulation of autophagy and the clearance of protein aggregates in neurodegeneration, Cell. Signalling, 2014, 26, 2694–2701 CrossRef CAS PubMed.
- X. Fan, J. Wang, J. Hou, C. Lin, A. Bensoussan and D. Chang, et al., Berberine alleviates ox-LDL induced inflammatory factors by up-regulation of autophagy via AMPK/mTOR signaling pathway, J. Transl. Med., 2015, 13, 92 CrossRef PubMed.
|
This journal is © The Royal Society of Chemistry 2019 |