DOI:
10.1039/C9RA01638D
(Paper)
RSC Adv., 2019,
9, 11833-11841
Influence of N-acetyl cysteine (NAC) and 2-methylene-1,3-dioxepane (MDO) on the properties of polymethyl methacrylate (PMMA) bone cement
Received
4th March 2019
, Accepted 8th April 2019
First published on 16th April 2019
Abstract
The properties of polymethyl methacrylate (PMMA) bone cement make it a popular bone filling material. However, its disadvantages, such as lack of biodegradability and osteogenesis, restrict its clinical application. Studies have indicated the osteogenic properties of N-acetyl cysteine (NAC) and the biodegradability of 2-methylene-1,3-dioxepane/methyl methacrylate-based (MDO/MMA) copolymers. In this study, we developed bioactive PMMA cements through modification with fixed concentrations of NAC and different proportions of MDO. The purpose of this study was to compare the mechanical properties, morphology, NAC release, biocompatibility, degradability and mineralization capability of modified bone cements with those of conventional cement. The specific-modified specimens (NAC-p (5% MDO-co-MMA)) exhibited a lower bending modulus but had little effect on compressive strength. This material was morphologically compact and nonporous, similar to conventional PMMA bone cement. NAC could be released from NAC-p (5% MDO-co-MMA) continuously and appropriately. NAC-p (5% MDO-co-MMA) was biologically safe and showed satisfactory tissue compatibility. Ester was introduced into the polymer, which reinforced the degradation properties of NAC-p (5% MDO-co-MMA). NAC-p (5% MDO-co-MMA) enhanced the mineralization capability of osteoblastic cells.
1. Introduction
Since balloon kyphoplasty (BKP) was first developed in the 1990s, it has been the most popular method to treat osteoporotic vertebral compression fractures. The bone cement used in the operation plays a vital role in the curative effect of BKP.1 PMMA bone cement has obvious advantages, such as appropriate syringeability, solidification characteristics, mechanical strength and radiopacity. However, its application is limited because of a lack of osteoconduction, biodegradability, osteogenesis, etc. These defects may restrain the bone cement–bone interface combination, and the bone cement will not be replaced by bone tissue, which causes osteolysis, looseness and even the separation of the vertebral body.2–4
Methyl methacrylate (MMA) polymerizes to PMMA through a C–C single bond under the catalysis of benzoyl peroxide (BPO). Thus, PMMA is a kind of high-molecular compound, whose degradation performance can be affected obviously through a change in chemical construction change.5 Ester can be introduced to the C–C backbone through copolymerization, and the ester is so weak that it can be fractured easily, which makes the high-molecular compounds readily biodegradable.6–8 In this study, we produced MDO/MMA copolymers containing esters in their backbone. Fig. 1 shows the reaction equation of MDO and MMA and the degradation performance of high-molecular compounds with and without MDO.
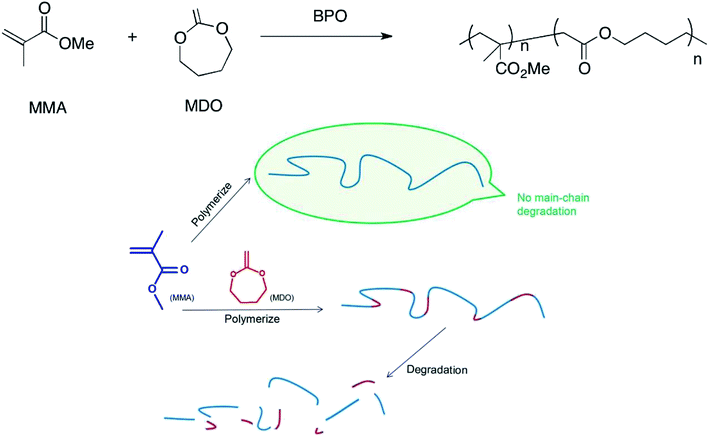 |
| Fig. 1 (Upper) Reaction equations of MDO and MMA. (Lower) Degradation performance of high-molecular compounds with and without MDO. MMA polymerizes to PMMA, which has no main-chain degradation; MMA reacts with MDO, and the product can be degraded. | |
N-Acetyl cysteine (NAC) is an amino acid derivative. NAC is widely used as expectorant in diseases of the respiratory system, and its biosafety is reliable.9–12 NAC has been reported to improve the biocompatibility of substances, enhance the expression of osteogenic genes, and activate the differentiation of osteoblasts.13–15 Naoki16 revealed that incorporating NAC improves the osteoconductivity of PMMA bone cement.
In this study, we aim to improve the degradability and mineralization property of original PMMA bone cement based on the premise that its performance is not reduced. We transformed original PMMA bone cement by using MDO and NAC, and the new bone cement was evaluated in several respects.
2. Materials and methods
2.1 Bone cement preparation
NAC powder (Aladdin, USA) was added to HEPES buffer as a stock solution (1 mol L−1) and the pH was adjusted to 7.2.
Commercial bone cement (Spineplex, USA) was used in the present research. The powder and liquid were mixed completely according to the manufacturer's recommendations as a control (C-PMMA). NAC-p (MDO-co-MMA) bone cement was prepared by mixing the powder and liquid containing NAC at concentrations of 25 mM (NAC–PMMA) and MDO at different proportions (1%, 5% or 10% in volume percentage). After mixing, the bone cement was transferred to steel molds measuring 75 × 10 × 3.3 mm (for the bending modulus test), ∅6 × 12 mm (for the compressive strength test, morphology evaluation and degradation assay), and ∅9 × 2 mm (for the NAC-release test, biological properties test and mineralization capability test) to produce different samples. In the mineralization capability assay, barium sulfate in powder was replaced by the same weight of PMMA powder.
2.2 Mechanical properties (compressive strength, bending modulus and operability)
Compressive strength and bending modulus were tested according to International Standard (ISO) 5833.17 There were 5 samples in each group.
The dough and setting time were tested in accordance with ISO 5833 (ref. 17) and ASTM C191,18 and we set four periods during solidification according to operability, including mixing, waiting, application and setting.
2.3 Degradation assay8,19
The samples were immersed in 5 mL glacial acetic acid in 15 mL tubes for 1 and 5 days. Then, 5 mL trichloromethane was added to each tube and shaken for 1 h. A 2 mL mixture was removed from each tube and transferred into new tubes after filtration. The liquid was adjusted to alkaline with caustic soda solution. A powder was obtained by drying the supernviaould-like liquid. The power was soaked in tetrahydrofuran (1 mg mL−1). Then, the number-average molecular weight (Mn) of the solute was measured by gel permeation chromatography (GPC; Waters GPC analysis system with a Waters 2414 refractive index detector, Milford, MA).
2.4 Morphology evaluation
The sample was broken off to show the section and gold was sprayed onto the section before testing. SEM (FEI-Quanta 250, USA) was used in this evaluation to observe the section at 15 kV.
2.5 NAC release test in vitro
The samples were immersed in 30 mL PBS (3 cm2 mL−1) and shaken (100 rpm) at 37 °C for a total of 64 days. Then, 0.5 mL PBS was withdrawn at 1 h, 1 day, 2 day, 4 day, 8 day, 16 day, 32 day, and 64 day. An equal volume of fresh PBS was added to the vessel after withdrawing, and a loss of NAC concentration at the next time point was taken into consideration. A standard calibration plot was used to calculate the concentration of NAC in the PBS according to a UV-vis spectrophotometer (absorbance of NAC at 205 nm). The release rate of NAC was calculated by the following expression:
Release rate/% = Ms/Mp × 100 |
where Ms was the weight of NAC in PBS and Mp was the weight of NAC in the cement samples used in this test.
2.6 Biocompatibility in vitro
2.6.1 Cell culture20. MC3T3-E1 cells (ATCC, USA) were cultured in α-MEM (HyClone, USA) with 1% penicillin/streptomycin solution (HyClone, USA) and 10% FBS (Gibco, USA). The cells were incubated with 5% CO2 at 37 °C. The cell culture medium was renewed every 3 days. The cells were detached by using 0.25% trypsin/1 mg ethylenediaminetetraacetic acid (EDTA, Beyotime, China) when 80% confluent.
2.6.2 Preparation of material extracts. According to ISO 10993,21 the samples were soaked in α-MEM (3 cm2 mL−1) at 37 °C for 48 h, and the extracts were stored at 4 °C before use.
2.6.3 Cell cytotoxicity assay. A total of 200 μL α-MEM with MC3T3-E1 cells at 1 × 104 cells per mL was cultivated on germ-free glass sheets in 48-well culture plates for 24 h. Then, the cell culture medium was replaced with 200 μL α-MEM, C-PMMA extracts and NAC-p (5% MDO-co-MMA) extracts as negative control, control and experimental groups, respectively. The medium was renewed every day. The cells were stained with hematoxylin and eosin (HE) after incubation for 1, 3, or 7 days. The cell state was evaluated using a light microscope at 40× magnification.Then 100 μL of the abovementioned cell suspension was added to the 96-well culture plates. The medium was replaced with 100 μL α-MEM, C-PMMA extracts and NAC-p (5% MDO-co-MMA) extracts after 24 h as negative control, control and experimental groups, respectively. The medium was renewed every day. Then 10 μL CCK8 reagent (Dojindo, Japan) was added to the medium at 1, 3, and 5 day, and the absorbance at 450 nm (OD450) was measured at 4 h later by using a microplate reader (Thermo Multiskan Spectrum, Thermo Scientific, USA). All assays were repeated five times.
2.6.4 Cell attachment. MC3T3-E1 cells were inoculated into the NAC-p (5% MDO-co-MMA) bone cement, C-PMMA bone cement and control tissue culture polystyrene (TCPS) in 48-well plate and incubated for 3 days. The cells were fixed with 4% paraformaldehyde solution (Liankebio, China) for 20 min and further dehydrated by using alcohol solutions at different concentrations. The materials were removed from the plate for critical point drying and then sprayed for SEM (FEI-Quanta 250, USA) observation.
2.7 Mineralization capability assay22–24
MC3T3-E1 cells were seeded onto C-PMMA bone cement, NAC-p (5% MDO-co-MMA) bone cement and polystyrene in 48-well plates at a density of 1.5 × 104 cells per cm2 as control, experimental group and negative control, respectively. The culture medium was replaced with osteogenic media (Cyagen, USA) after 24 h and renewed every 3 days.
Alizarin red staining was used to evaluate the mineralization capability of the MC3T3-E1 cells after the induction of differentiation for 14 days. The cells were fixed with 4% paraformaldehyde solution for 20 min and further incubated with alizarin red solution (Cyagen, USA) for 10 min. For quantitative analysis, cultures were washed three times with PBS and incubated in 10% cetylpyridinium chloride solution (Sigma-Aldrich Inc., St. Louis, MO, USA) with gentle shaking for 15 min. The absorbance of the solution at 562 nm (OD562) was measured by using a microplate reader.
2.8 Statistical analysis
The results are presented as the mean ± standard deviation (SD) and analyzed by using one-way analysis of variance (ANOVA) to calculate the average for multiple groups. The least significant difference (LSD) test was used for multiple comparisons between groups. Differences between two means were examined by t test. Differences between groups are considered statistically significant if p is less than 0.05.
3. Results
3.1 Compressive strength and bending modulus
The compressive strength and bending modulus of the tested samples are shown in Table 1. The test showed that the compressive strength and bending modulus decreased with increasing proportions of MDO. The difference of bending modulus between NAC-p (5% MDO-co-MMA) and C-PMMA was significant (P < 0.05). The compressive strength of NAC-p (10% MDO-co-MMA) was lower than that of C-PMMA (P < 0.05), while the difference of compressive strength between NAC-p (5% MDO-co-MMA) and C-PMMA was insignificant (P ≥ 0.05). Bone cement containing 5% MDO had the best mechanical properties: minimal bending modulus of 1.97 ± 0.08 GPa, and compressive strength meeting the international requirement according to ISO 5833 and ASTM C191 (≥70 MPa). Therefore, NAC-p (5%MDO-co-MMA) was selected for further research.
Table 1 The compressive strength and compressive modulus of bone cement with different compositions (X ± SD, n = 5)
Samples |
Compressive strength (MPa) |
Bending modulus (GPa) |
C-PMMA |
74.88 ± 2.46 |
2.67 ± 0.07 |
NAC-PMMA |
74.13 ± 3.11 |
2.54 ± 0.12 |
NAC-p (1% MDO-co-MMA) |
73.68 ± 2.90 |
2.47 ± 0.09 |
NAC-p (5% MDO-co-MMA) |
72.04 ± 4.27 |
1.97 ± 0.08 |
NAC-p (10% MDO-co-MMA) |
68.15 ± 3.28 |
1.89 ± 0.17 |
3.2 Operability
The solidification characteristics of NAC-p (5% MDO-co-MMA) are displayed in Fig. 2. The waiting period is slightly longer for NAC-p (5% MDO-co-MMA) than for C-PMMA, and the application period is approximately 1.5 min longer, which suggests that operators can obtain more sufficient time to operate the bone cement with paying little cost.
 |
| Fig. 2 Solidification characteristics of NAC-p (5% MDO-co-MMA). Measured at 23 °C with C-PMMA as a control. | |
3.3 Degradation assay
Fig. 3 presents the appearance of the bone cement soaked in glacial acetic acid for 24 h and the results of the GPC analysis. Both NAC-p (5% MDO-co-MMA) bone cement (A) and C-PMMA bone cement (B) swelled in glacial acetic acid. A long chain of NAC-p (5% MDO-co-MMA) polymer was cut off; thus, the powder could not be embedded in the polymer and became scattered in the surrounding liquid, which made the liquid turbid. The long chain of C-PMMA could not be cut off; thus, the powder became embedded in the polymer made the liquid clear. GPC analysis confirmed this phenomenon. Mn of NAC-p (5% MDO-co-MMA) declined 50.4% after soaking for 1 day, and the difference was significant (P < 0.05), while the difference between 1 day and 5 day was not significant (P ≥ 0.05). The Mn of C-PMMA did not decline significantly after soaking for 1 day (P ≥ 0.05) and 5 days (P ≥ 0.05).
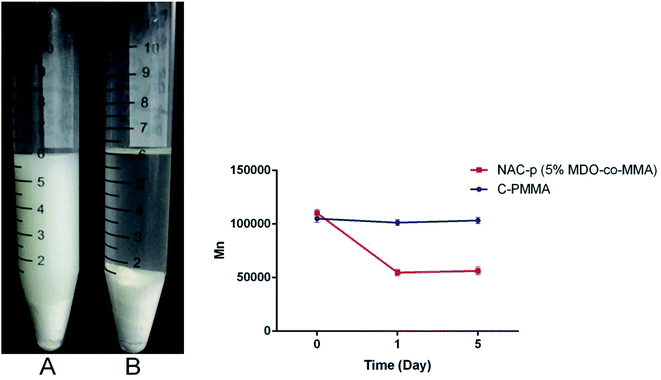 |
| Fig. 3 (Left) NAC-p (5% MDO-co-MMA) bone cement (A) and C-PMMA bone cement (B) soaked in glacial acetic acid for 24 h. (Right) GPC analysis of NAC-p (5% MDO-co-MMA) and C-PMMA. | |
3.4 Morphology evaluation
Section of NAC-p (5% MDO-co-MMA) has a pyknotic surface, and no holes can be found, which is similar to the morphology of C-PMMA (Fig. 4). This result suggests that adding NAC and MDO does not affect the morphology of PMMA bone cement.
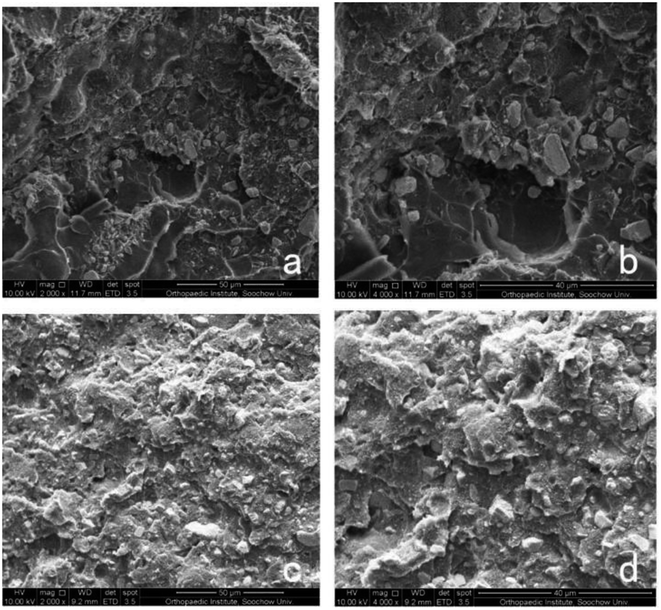 |
| Fig. 4 Morphology of bone cement. SEM of sections of C-PMMA at 2000× (a) and 4000× (b) magnification and NAC-p (5% MDO-co-MMA) at 2000× (c) and 4000× (d) magnification. | |
3.5 NAC-release test
The pattern of NAC release for 64 days in vitro was fitted to a controlled-release plot, with an initial burst effect during the first 1 h, in which 11.67 ± 1.38% NAC was released from NAC-p (5% MDO-co-MMA) bone cement into the PBS solution (Fig. 5). The release process lasted at least 2 months, and the release percentage was 47.87% ± 5.38% on day 64.
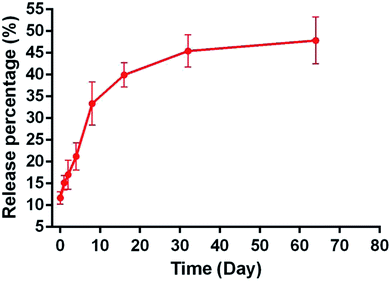 |
| Fig. 5 The cumulative release percentage of NAC in NAC-p (5% MDO-co-MMA) bone cement. | |
3.6 In vitro biocompatibility
3.6.1 Cell cytotoxicity. The left side of Fig. 5 shows the morphology of the cells cultured in different media for 1, 3, and 7 days. The cells multiplied rapidly and maintained an elongated, spindle-shaped morphology. There were no significant differences in morphology among the three groups during the culture time.The optical density (OD) of all groups changed during the culture time in the CCK8 assay (right side of Fig. 6). The OD value differences were not significant among C-PMMA, NAC-p (5%MDO-co-MMA) and control at each time point. According to ISO 10993-5,20 a reduction in cell proliferation by more than 30% is considered as cytotoxicity. Therefore, neither of the two kinds of bone cement inhibited cell proliferation.
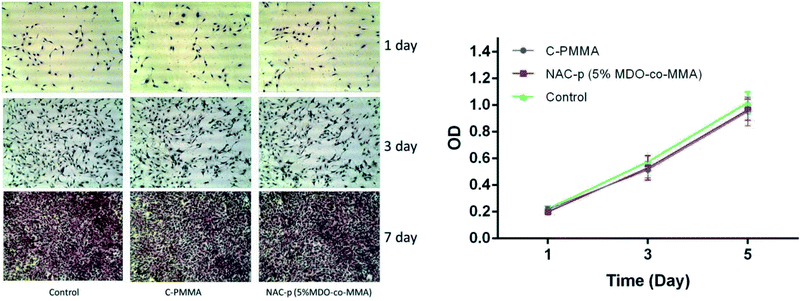 |
| Fig. 6 (Left) HE staining of MC3T3-E1 cells cultured for 1, 3, and 7 days in α-MEM (referred to as the control group) and C-PMMA and NAC-p (5% MDO-co-MMA) extracts. No significant difference could be found among the three groups. (Right) CCK8 assay of MC3T3-E1 cells cultured in α-MEM, C-PMMA and NAC-p (5% MDO-co-MMA) bone cement extracts. | |
3.6.2 Cell attachment. Fig. 7 shows SEM images of cells grown on NAC-p (5% MDO-co-MMA) bone cement (a), C-PMMA (b) and TCPS (c). The cells spread well with close contact with the surface of the two kinds of bone cements and the TCPS. Rough surface of bone cements provided more attachment points than that of TCPS. Both NAC-p (5% MDO-co-MMA) and C-PMMA bone cements had acceptable biocompatibility.
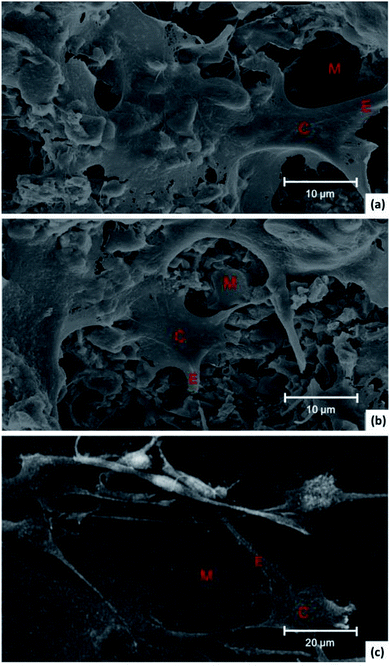 |
| Fig. 7 SEM images of MC3T3 cells grown on the surface of NAC-p (5% MDO-co-MMA) bone cement (a), C-PMMA (b) and TCPS (c). C represents the cell body of MC3T3-E1, E represents the extension and M represents the material. | |
3.7 Mineralization capability
As shown in Fig. 8, compared with polystyrene, MC3T3-E1 cells cultured on C-PMMA showed an obvious suppression of mineralization capability (P < 0.05). Compared with C-PMMA, NAC-p (5% MDO-co-MMA) bone cement was found to be mineralization-positive (P < 0.05), with values greater than those recorded for cultures on polystyrene (P < 0.05).
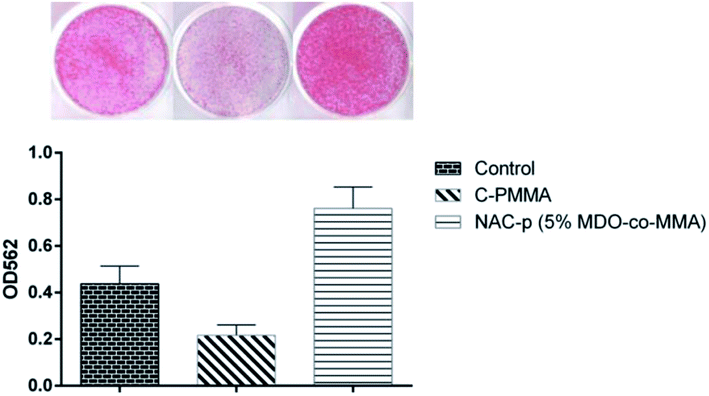 |
| Fig. 8 Improvement by NAC-p (5% MDO-co-MMA) of the mineralization capability that is suppressed by C-PMMA. | |
4. Discussion
Alkaline phosphatase activity, Von Kossa stain and in vivo experiments were used in research by Naoki16 to suggest that 25 mM is the most appropriate concentration of NAC in commercial PMMA bone cement for the best osteoconduction. We referenced the achievement of Naoki, and the concentration of NAC is 25 mM in all bone cements containing NAC.
Compressive strength, bending modulus and operability are important characteristics of bone cement. PMMA bone cement has advantages in compressive strength and operability. However, it has a high bending modulus, which may cause loosening and stress shielding in the vertebral body.25,26 Jenny8 synthesized 2-methylene-1,3-dioxepane/glycidyl methacrylate-based (MDO/GMA) copolymers with different feed ratios and indicated the changes in the physical properties of the copolymers. Since the reaction principle of MDO/GMA and MDO/MMA is the same, we found that NAC-p (5% MDO-co-MMA) showed satisfactory compressive strength and operability. In addition, the bending modulus declined from 2.67 ± 0.07 GPa to 1.97 ± 0.08 GPa, which was closer to that of spongy bone (0.05–0.5 GPa).27 Many other PMMA-based cements have been reported. In contrast, Ti-PMMA, as reported by Fukuda C, had satisfactory compressive strength but a high bending modulus.28 HEMA–PMMA, HA–chitin–PMMA and TCP–PMMA showed reductions in both compressive strength and bending modulus.29–31
Many researchers have attempted to enhance the bioactivity of PMMA-based bone cements by adding bioactive materials, such as polyhydroxyalkanoate,32 calcium phosphate,33 bioglass,34,35 and cellulose.36 However, these additives made PMMA bone cement porous and led to a reduction of its mechanical properties, making most of the bone cement unsuitable in areas of high stress. NAC-p (5% MDO-co-MMA) was compact and imporous. This characteristic was a precondition for satisfactory mechanical properties.
NAC can improve the osteoconductivity of PMMA bone cement by influencing surrounding osteoblasts.16 However, the dissolution curve of NAC release from PMMA bone cement was undefined. In this study, we put NAC-p (5% MDO-co-MMA) in a simulated human environment to discover the release rule. The test revealed that the initial burst effect of NAC-p (5% MDO-co-MMA) was slightly higher than that of calcium phosphate cements and calcium sulfate cements.37,38 With the sustained release effect, it was reasonable that the bone cement could maintain an appropriate and steady NAC concentration to act on osteoblasts.
Biocompatibility is a precondition for the application of bone cements in vivo. People have relied on the practicability and safety of PMMA-based bone cement for decades since it was first used in the human body in 1958.39 However, a small amount of MMA monomers may be released during the polymerization reaction to influence the body, even resulting in necrosis of the implanted region.40 NAC is used as expectorant, and its safety has been widely recognized. Masahiro41 revealed that NAC could inactivate MMA monomers by its antioxygenation capacity to improve the survival rates of cocultured cells. Incorporating NAC inhibited the local inflammation and systemic toxicity effects caused by the implantation of PMMA bone cement in rabbits.16 MDO is a practical synthetic source for medical material and biodegradable material. Moreover, NAC-p (5% MDO-co-MMA) is predicted to be nontoxic. In our study, NAC-p (5% MDO-co-MMA) bone cement revealed no cytotoxicity in the morphologic observation, CCK-8 assay or cell attachment assay.
Materials produced by reacting acrylic acid with MDO show obvious biodegradability. The degradation accelerates with increasing amount of MDO.42 Esters in the long-chain are easily hydrolyzed in acetic acid, and the long-chain is thus cut into pieces. Acetic acid did not make the Mn of C-PMMA decline while that of NAC-p (5% MDO-co-MMA) did decline. This result indicated that the long-chain in NAC-p (5% MDO-co-MMA) was almost completely degraded in 1 day since the difference between 1 day and 5 day was not significant.
A red compound was produced when alizarin red interacted with minerals in the cells. After the red compound dissolved in 10% cetylpyridinium chloride, the absorbance of the solution was measured to evaluate the amount of mineral manufactured by the cells. Our data suggested that the suppression of mineralization capability on the C-PMMA was pronounced. In contrast, NAC-p (5% MDO-co-MMA) enhanced the mineralization capability of osteoblastic cells. We hypothesized that NAC supplementation was responsible for the enhanced mineralization capability. Naoki reported that the incorporation of NAC detoxifies the PMMA bone cement material by eliminating free radicals and increasing the antioxidation ability of the cells and consequently adds osteoconductivity to the material.16 Our data provided certain proof to support this research and show that the modification converts the bioproperty of bone cement from a bioinert to osteoconductive level.
We succeeded in making NAC-p (5% MDO-co-MMA) compound bone cement and testing its performance in vitro. Further studies are needed to modify nondegradable solid material for complete biodegradation and to test these conditions in vivo.
5. Conclusions
NAC-p (5% MDO-co-MMA) bone cement showed superior mechanical properties, good biocompatibility, some degradability and mineralization properties, as we had expected. NAC-p (5% MDO-co-MMA) markedly lowered the bending modulus of PMMA from 2.67 GPa to 1.97 GPa and optimized solidification while having little effect on its compressive strength. NAC-p (5% MDO-co-MMA) was compact and imporous under SEM, which was a structural basis for its good mechanical properties. NAC could be released from NAC-p (5% MDO-co-MMA) continuously and appropriately. According to ISO 10993, NAC-p (5% MDO-co-MMA) was biologically safe in cytotoxicity assays with respect to cell attachment. In addition, esters were successfully introduced into the polymer, which made the degradation properties significantly better for NAC-p (5% MDO-co-MMA) than for C-PMMA in acid. A comparison of the mineralization capability between C-PMMA and NAC-p (5% MDO-co-MMA) also suggests that NAC-p (5% MDO-co-MMA) might improve the osteoconductive level. We propose that NAC-p (5% MDO-co-MMA) bone cement has potential clinical value.
Conflicts of interest
There are no conflicts of interest to declare.
Acknowledgements
This work was generously supported by the Youth Science and Technology Project of Suzhou Health and Family Planning Commission (KJXW2017059).
References
- Z. He, Q. Zhai, M. Hu, C. Cao, J. Wang, H. Yang and B. Li, Bone cements for percutaneous vertebroplasty and balloon kyphoplasty: current status and future developments, Journal of Orthopaedic Translation, 2015, 3, 1–11, DOI:10.1016/j.jot.2014.11.002.
- A. Boger, P. Heini, M. Windolf and E. Schneider, Adjacent vertebral failure after vertebroplasty: a biomechanical study of low-modulus PMMA cement, Eur. Spine J., 2007, 16, 2118–2125 CrossRef PubMed.
- U. Berlemann, C. W. Müller and C. Krettek, Percutaneous cementing techniques of the spine -- chances and limits, Der Orthopäde, 2004, 33, 6 CrossRef CAS PubMed.
- D. Granchi, E. Cenni, L. Savarino, G. Ciapetti, G. Forbicini, M. Vancini, C. Maini, N. Baldini and A. Giunti, Bone cement extracts modulate the osteoprotegerin/osteoprotegerin-ligand expression in MG63 osteoblast-like cells, Biomaterials, 2002, 23, 2359–2365 CrossRef CAS PubMed.
- F. L. Buchholz and A. T. Graham, Modern Superabsorbent Polymer Technology, Research and Markets, 1997 Search PubMed.
- V. Delplace, A. Tardy, S. Harrisson, S. Mura, D. Gigmes, Y. Guillaneuf and J. Nicolas, Degradable and comb-like PEG-based copolymers by nitroxide-mediated radical ring-opening polymerization, Biomacromolecules, 2013, 14, 3769–3779 CrossRef CAS PubMed.
- A. Seema and L. Ren, Polycaprolactone-Based Novel Degradable Ionomers by Radical Ring-Opening Polymerization of 2-Methylene-1,3-dioxepane, Macromolecules, 2009, 42, 1574–1579 CrossRef CAS.
- J. Undin, A. Finnewistrand and A. C. Albertsson, Adjustable Degradation Properties and Biocompatibility of Amorphous and Functional Poly(ester-acrylate)-Based Materials, Biomacromolecules, 2014, 15, 2800–2807 CrossRef CAS PubMed.
- A. M. Sadowska, B. Manuel-Y-Keenoy and W. A. De Backer, Antioxidant and anti-inflammatory efficacy of NAC in the treatment of COPD: discordant in vitro and in vivo dose-effects: a review, Pulm. Pharmacol. Ther., 2007, 20, 9–22 CrossRef CAS PubMed.
- M. Decramer, M. M. Rutten-Van, P. N. Dekhuijzen, T. Troosters, H. C. Van, R. Pellegrino, C. P. van Schayck, D. Olivieri, D. M. Del and B. W. De, Effects of N-acetylcysteine on outcomes in chronic obstructive pulmonary disease (Bronchitis Randomized on NAC Cost-Utility Study, BRONCUS): a randomised placebo-controlled trial, Respiratory Medicine: COPD Update, 2005, 1, 66 Search PubMed.
- F. Reichenberger and M. Tamm, N-acetylcystein in the therapy of chronic bronchitis, Pneumologie, 2002, 56, 793–797 CAS.
- S. Kuleci, I. Hanta, A. Kocabas and N. Canacankatan, The effect of different treatment modalities on oxidative stress in COPD, Adv. Ther., 2008, 25, 710 CrossRef CAS PubMed.
- M. Yamada, N. Tsukimura, T. Ikeda, Y. Sugita, W. Att, N. Kojima, K. Kubo, T. Ueno, K. Sakurai and T. Ogawa, N-acetyl cysteine as an osteogenesis-enhancing molecule for bone regeneration, Biomaterials, 2013, 34, 6147–6156, DOI:10.1016/j.biomaterials.2013.04.064.
- H. Toker, H. Ozdemir, K. Eren, H. Ozer and G. Sahin, N-acetylcysteine, a thiol antioxidant, decreases alveolar bone loss in experimental periodontitis in rats, J. Periodontol., 2009, 80, 672–678 CrossRef CAS PubMed.
- N. K. Lee, Y. G. Choi, J. Y. Baik, S. Y. Han, D. W. Jeong, Y. S. Bae, N. Kim and S. Y. Lee, A crucial role for reactive oxygen species in RANKL-induced osteoclast differentiation, Blood, 2005, 106, 852 CrossRef CAS PubMed.
- N. Tsukimura, M. Yamada, H. Aita, N. Hori, F. Yoshino, L. M. Changil, K. Kimoto, A. Jewett and T. Ogawa, N-acetyl cysteine (NAC)-mediated detoxification
and functionalization of poly(methyl methacrylate) bone cement, Biomaterials, 2009, 30, 3378–3389 CrossRef CAS PubMed.
- S. Standards, Implants For Surgery - Acrylic Resin Cements - Orthopaedic Applications, ( 1979) Search PubMed.
- ASTM C191-13, Standard Test Methods for Time of Setting of Hydraulic Cement by Vicat Needle, ASTM International, 12/01/2013 Search PubMed.
- D. Ding, X. Pan, Z. Zhang, N. Li, J. Zhu and X. Zhu, A degradable copolymer of 2-methylene-1,3-dioxepane and vinyl acetate by photo-induced cobalt-mediated radical polymerization, Polym. Chem., 2016, 7, 5258–5264, 10.1039/C6PY01061J.
- T. Ruediger, A. Berg, A. Guellmar, C. Rode, M. Schnabelrauch, A. Urbanek, K. Wagner, R. Wyrwa, R. W. Kinne and B. W. Sigusch, Cytocompatibility of polymer-based periodontal bone substitutes in gingival fibroblast and MC3T3 osteoblast cell cultures, Dent. Mater., 2012, 28, e239–e249 CrossRef CAS PubMed.
- S. Australia, Biological Evaluation of Medical Devices, ISO, 2005, vol. 16, pp. 10–15 Search PubMed.
- Z. Tang, Z. Gong and X. Sun, LncRNA DANCR involved osteolysis after total hip arthroplasty by regulating FOXO1 expression to inhibit osteoblast differentiation, J. Biomed. Sci., 2018, 25, 4, DOI:10.1186/s12929-018-0406-8.
- X. Gao, J. Ge, W. Li, W. Zhou and L. Xu, LncRNA KCNQ1OT1 promotes osteogenic differentiation to relieve osteolysis via Wnt/β-catenin activation, Cell Biosci., 2018, 8, 19, DOI:10.1186/s13578-018-0216-4.
- Q. Liu, Y. Zhou and Z. Li, PDGF-BB promotes the differentiation and proliferation of MC3T3-E1 cells through the Src/JAK2 signaling pathway, Mol. Med. Rep., 2018, 18, 3719–3726, DOI:10.3892/mmr.2018.9351.
- D. P. Beall, D. S. L. D'Souza, L. S. D'Souza, D. Gunda, J. Morelli, M. B. Johnson and N. Nabavizatleh, Percutaneous treatment of insufficiency fractures Principles, technique and review of literature, Skeletal Radiology, 2010, 39, 117–130 CrossRef PubMed.
- M. Driscoll and L. Blyum, The presence of physiological stress shielding in the degenerative cycle of musculoskeletal disorders, Journal of Bodywork and Movement Therapies, 2011, 15, 335–342 CrossRef CAS PubMed.
- T. Kokubo, H. M. Kim and M. Kawashita, Novel bioactive materials with different mechanical properties, Biomaterials, 2003, 24, 2161–2175 CrossRef CAS PubMed.
- C. Fukuda, K. Goto, M. Imamura and T. Nakamura, Bioactive bone cement with a low content of titania particles without postsilanization: effect of filler content on osteoconductivity, mechanical properties, and handling characteristics, J. Biomed. Mater. Res., Part B, 2010, 95, 407 CrossRef CAS PubMed.
- C. Wolf-Brandstetter, S. Roessler, S. Storch, U. Hempel, U. Gbureck, B. Nies, S. Bierbaum and D. Scharnweber, Physicochemical and cell biological characterization of PMMA bone cements modified with additives to increase bioactivity, J. Biomed. Mater. Res., Part B, 2013, 101, 599 CrossRef PubMed.
- S. B. Kim, Y. J. Kim, T. L. Yoon, S. A. Park, I. H. Cho, E. J. Kim, I. A. Kim and J. W. Shin, The characteristics of a hydroxyapatite-chitosan-PMMA bone cement, Biomaterials, 2004, 25, 5715–5723 CrossRef CAS PubMed.
- B. Vázquez, M. P. Ginebra, X. Gil, J. A. Planell and R. J. San, Acrylic bone cements modified with beta-TCP particles encapsulated with poly(ethylene glycol), Biomaterials, 2005, 26, 4309–4316 CrossRef PubMed.
- S. Nguyen and R. H. Marchessault, Graft copolymers of methyl methacrylate and poly([R]-3-hydroxybutyrate) macromonomers as candidates for inclusion in acrylic bone cement formulations: Compression testing, J. Biomed. Mater. Res., Part B, 2006, 77, 5–12 CrossRef PubMed.
- Y. Totani, A. Sugino, T. Miyazaki and C. Ohtsuki, Acceleration of calcium phosphate formation on bioactive PMMA-based bone cement by controlling spatial design, Mater. Sci. Eng., C, 2010, 30, 624–630 CrossRef CAS.
- S. Shinzato, T. Nakamura, T. Kokubo and Y. Kitamura, A new bioactive bone cement: effect of glass bead filler content on mechanical and biological properties, J. Biomed. Mater. Res., Part B, 2015, 54, 491–500 CrossRef.
- S. Shinzato, T. Nakamura, K. Ando, T. Kokubo and Y. Kitamura, Mechanical properties and osteoconductivity of new bioactive composites consisting of partially crystallized glass beads and poly(methyl methacrylate), J. Biomed. Mater. Res., Part A, 2002, 60, 556–563 CrossRef CAS PubMed.
- H. Qiang, H. Chen, H. Li, J. Dong, D. Guo, M. Mao, K. Liang, L. Yang, Z. Wu and L. Wei, Porous Surface Modified Bioactive Bone Cement for Enhanced Bone Bonding, PLoS One, 2012, 7, e42525 CrossRef PubMed.
- M. P. Ginebra, C. Canal, M. Espanol, D. Pastorino and E. B. Montufar, Calcium phosphate cements as drug delivery materials, Adv. Drug Delivery Rev., 2012, 64, 1090–1110 CrossRef CAS PubMed.
- M. D. Vlad, E. V. Şindilar, M. L. Mariñoso, I. Poeată, R. Torres, J. López, M. Barracó and E. Fernández, Osteogenic biphasic calcium sulphate dihydrate/iron-modified α-tricalcium phosphate bone cement for spinal applications: In vivo study, Acta Biomater., 2010, 6, 607–616 CrossRef CAS PubMed.
- J. Charnley, Anchorage of the femoral head prosthesis to the shaft of the femur, J. Bone Jt. Surg., Br. Vol., 1960, 42, 28–30 CrossRef.
- R. Feith, Side-effects of acrylic cement implanted into bone. A histologicl, (micro)angiographic, fluorescence-microscopic and autoradiographic study in the rabbit femur, Acta Orthop. Scand., Suppl., 1975, 161, 3 CAS.
- M. Yamada and T. Ogawa, Chemodynamics underlying N-acetyl cysteine-mediated bone cement monomer detoxification, Acta Biomater., 2009, 5, 2963–2973, DOI:10.1016/j.actbio.2009.04.027.
- J. W. Guo, Q. Ma, Y. H. Cui, S. Liu and J. P. Peng, Synthesis and characterization of biodegradable copolymers as non-phosphorous detergent builders, Acta Polym. Sin., 2012, 012, 958–964 CrossRef.
|
This journal is © The Royal Society of Chemistry 2019 |
Click here to see how this site uses Cookies. View our privacy policy here.