DOI:
10.1039/C9RA01405E
(Paper)
RSC Adv., 2019,
9, 17432-17439
A highly efficient vortex-assisted liquid–liquid microextraction based on natural deep eutectic solvent for the determination of Sudan I in food samples
Received
24th February 2019
, Accepted 18th May 2019
First published on 4th June 2019
Abstract
A natural deep eutectic solvent (NADES) composed of choline chloride (ChCl) and sesamol was successfully employed in the vortex-assisted liquid–liquid microextraction (VALLME) of food toxicant Sudan I (1-phenylazo-2-naphthalenol) in food samples for HPLC-UV analysis. Sesamol-based NADESs exhibited better Sudan I extraction abilities than other deep eutectic solvents and conventional organic solvents. 1H NMR and 2D NOESY spectra were used to characterize the sesamol-based NADESs, indicating that hydrogen bonds were formed between ChCl and sesamol. The developed VALLME method showed a high extraction efficiency (near 100%) within 60 s at room temperature. Under the optimized extraction conditions, this established method showed good linearity (r2 = 1.000) and a low limit of detection (LOD) of 0.02 mg kg−1. The recoveries were in the range of 93–118%, and the intra-day and inter-day precisions were less than 4.5%. The developed method was successfully applied to the determination of Sudan I in various food samples, including chili oil, chili sauce, and duck egg yolk. This method gave a higher recovery than that of the EU recommended method when applied to sample analysis.
1. Introduction
Sudan I is among the most widely used synthetic lipophilic azo dyes in the chemical industry.1 As Sudan I and other Sudan dyes have toxic effects on human organs, leading to bladder and liver cancers,2 their use in food is banned in most countries, including the United States, European Union, China, Japan, and Australia.3 Owing to the red-orange color and low cost of Sudan I, it is illegally added to some food products, particularly chili and ketchup products (such as chili oil). Therefore, effective and reliable methods for the determination of Sudan I in foodstuffs are highly significant.
Several analytical techniques have been established for Sudan I determination, such as high-performance liquid chromatography (HPLC),4–7 electrochemical methods,8–10 and an immunoanalysis method,11 among which HPLC is the most accessible and reliable method. For example, HPLC was selected for the EU recommended method for Sudan analysis.12 For complicated sample matrixes, different pretreatment processes have been employed before HPLC analysis of Sudan I, such as conventional liquid–liquid extraction,12 solid-phase microextraction, and liquid–liquid microextraction.13
In recent years, liquid–liquid microextraction techniques based on deep eutectic solvents have attracted wide attention, such as ultrasonic-assisted liquid–liquid microextraction technique (UALLME),14–16 air-assisted liquid–liquid microextraction (AALLME),17 and vortex-assisted liquid–liquid microextraction (VALLME).18 Deep eutectic solvents are new green solvents that are readily available owing to their simple preparation.19 They show unique properties, such as high purity and environmental friendliness.20,21 The application of deep eutectic solvents in the extraction and separation of target analytes from various samples has attracted wide interest.22 Target analytes have included inorganic heavy metal ions (such as Cd, Pd, and Cr),23 polar organic compounds (such as phenolic compounds, phenolic acids, and flavonoids),24–26 and non-polar or medium-polar organic compounds (such as PAHs).27–29 Different deep eutectic solvents have been employed in extraction processes based on the properties of the target analytes. For example, for the extraction of PAHs from water, a deep eutectic solvent composed of choline chloride (ChCl) and phenol was used owing to π–π interactions between ChCl–phenol and benzene rings in the PAHs.27
Natural deep eutectic solvents (NADESs), which are directly composed of natural products, such as organic acids, amino acids, sugars, choline, and urea, have received increasing attention recently due to being composed of primary metabolites in living cells.20 In this work, the extraction and analysis of weakly polar compound Sudan I from food samples (such as chili oil) was performed with novel and green phenolic NADESs composed of ChCl and sesamol (considered a non-toxic and natural antioxidant) using the VALLME technique. The sesamol-based NADESs exhibited excellent Sudan I extraction abilities compared with other deep eutectic solvents and conventional organic solvents tested. A VALLME-based HPLC-UV method was developed for the determination of Sudan I in various food samples, and characterization of the analytical method was also performed.
2. Material and methods
2.1 Chemicals and reagents
Choline chloride (ChCl) was obtained from Sinopharm Chemical Reagent Co., Ltd (Shanghai, China). Sesamol (4-hydroxy-1,2-methylenedioxybenzene) was purchased from Sigma-Aldrich (Shanghai, China). Sudan I (1-phenylazo-2-naphthalenol) was purchased from Macklin Co., Ltd (Shanghai, China). Acetic acid, methanol, and acetonitrile were of HPLC grade, while other chemicals were of analytical reagent grade. Redistilled water was used in all experiments. All samples were filtered before HPLC analysis. Sudan I standard stock solutions were prepared in methanol with a concentration of 1.0 mg mL−1 and stored in a refrigerator at −18 °C for up to three months. A calibration solution of Sudan I was prepared with methanol. Soybean oil, chili oil, chili sauce, and duck egg yolk samples were purchased from local markets.
2.2 Instruments
A Waters e2695 HPLC system equipped with a Waters 2489 UV detector was used for HPLC analysis. Chromatographic separation was carried out on a C18 reversed-phase column (Waters SymmetryShield RP18, 5 μm, 4.6 × 250 mm). The mobile phases were methanol (A) and 0.5% (v/v) acetic acid aqueous solution (B). The isocratic elution solvent system was methanol–H2O (92
:
8, v/v) containing 0.5% AcOH with a flow rate of 1.0 mL min−1. The injection volume was 10 μL and the column temperature was set at 30 °C. The detected compound was quantified at a wavelength of 478 nm. A vortex apparatus (VORTEX 1, IKA, Guangzhou, China) was used in the extraction procedure.
2.3 Preparation of deep eutectic solvents
Deep eutectic solvents were synthesized by heating different hydrogen bond donors (HBD) and ChCl at 70–80 °C with constant stirring until a homogeneous liquid was formed. Abbreviations for the deep eutectic solvents prepared in this study are listed in Table 1. The as-prepared deep eutectic solvents were stored in glass bottles at room temperature until use.
Table 1 Abbreviations of deep eutectic solvents studied
Species of deep eutectic solvent |
Salt |
Hydrogen bond donor (HBD) |
Salt/HBD (mol mol−1) |
1 |
ChCl |
Lactate |
1 : 2 |
2 |
ChCl |
Acetic acid |
1 : 2 |
3 |
ChCl |
Ethylene glycol |
1 : 2 |
4 |
ChCl |
Butanediol |
1 : 2 |
5 |
ChCl |
Glycerol |
1 : 2 |
6 |
ChCl |
Phenol |
1 : 2 |
7 |
ChCl |
o-Cresol |
1 : 2 |
8 |
ChCl |
p-Cresol |
1 : 2 |
9 |
ChCl |
Sesamol |
1 : 3 |
10 |
ChCl |
Catechol |
1 : 2 |
2.4 NMR spectroscopy
1H NMR spectra and 2D NOESY spectra were recorded at 25 °C on a Bruker 500 MHz NMR spectrometer (proton frequency, 500.00 MHz) equipped with a BBI liquid probe and Z-gradient system. Pyridine-d5 in an external glass capillary was used as lock solvent. For 1D-1H NMR spectra, pulse program “zg” was used with a delay time of 10 s. 2D NOESY spectra was acquired with pulse program “noesygpphpp” with a relaxation delay of 2.0 s. The mixing time was 300 ms. A data matrix of 2048 × 256 points was recorded with eight scans for each increment. Data were zero-filled to 2048 × 2048 points prior to States-TPPI-type 2D Fourier transform.
2.5 Extraction of Sudan I from food samples using VALLME
For chili oil samples, certain amounts of chili oil and the deep eutectic solvent were added to a centrifuge tube. The mixture was oscillated in a vortex apparatus for 60 s at room temperature and then centrifuged at 6000 rpm for 3 min. After centrifugation, two clear phases (oil phase/deep eutectic solvent-rich phase) were observed, and the DESs-rich phase at the bottom was withdrawn using a microsyringe. The deep eutectic solvent-rich phase was then injected into the HPLC system for analysis.
For solid food samples (chili sauce and duck egg yolk), a certain amount of n-hexane was used to extract 0.20 g of sample. n-Hexane was then mixed with a certain amount of deep eutectic solvent. The mixture was then oscillated in vortex apparatus for 60 s at room temperature and then centrifuged at 6000 rpm for 3 min.
To optimize the extraction conditions, a simulated oil sample was prepared by adding a certain amount of Sudan I (10 mg kg−1 oil) to soybean oil.
2.6 Extraction of Sudan I from food samples using EU recommended method
Following the EU recommended method,12 food samples (1.0 g) were extracted with acetonitrile (10 mL) for 1 h under vortex oscillation and then centrifuged at 6000 rpm for 3 min. After centrifugation, the final acetonitrile solution was injected into the HPLC system for analysis.
3. Results and discussion
3.1 Characterization of deep eutectic solvents
Phenol-based deep eutectic solvents prepared in this work were characterized using 1H NMR and 2D NMR techniques. The NADES composed of choline chloride (ChCl) and sesamol (1
:
3 molar ratio) was selected as an example, and the chemical structure was confirmed by 1H NMR spectroscopy (Fig. 1). The 1H NMR integration results shown in Fig. 1 confirmed that the molar ratio of ChCl and sesamol in the NADES was 1
:
3. To further identify the interaction between ChCl and sesamol in the natural deep eutectic mixture, nuclear Overhauser effect (NOE) NMR analysis was also conducted (Fig. 2). The NOESY spectrum of NADES (ChCl/sesamol) indicated a strong interaction between protons in the hydroxyl groups (–OH) of ChCl and sesamol, which indicated that hydrogen bonds were formed, as shown in Fig. 3a.
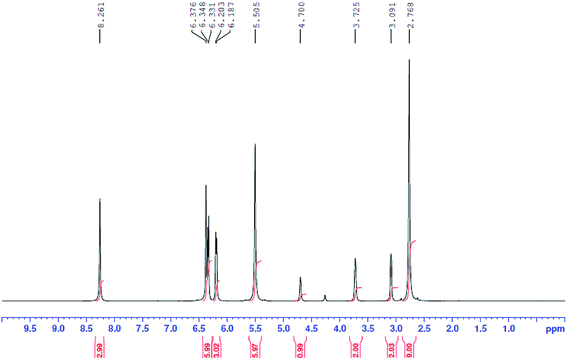 |
| Fig. 1 ChCl–sesamol NADES 1H NMR proton profiling. | |
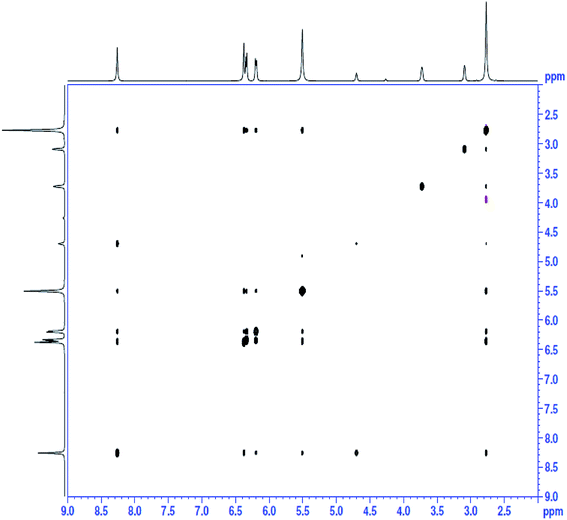 |
| Fig. 2 ChCl–sesamol NADES 2D NMR proton profiling. | |
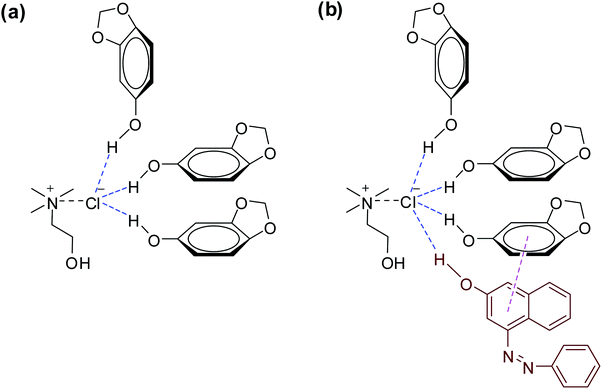 |
| Fig. 3 (a) ChCl–sesamol NADES; (b) π–π and hydrogen bond interactions between NADES and Sudan I. | |
3.2 Effect of deep eutectic solvents on the extraction process
The components of deep eutectic solvents significantly influence their physicochemical properties, such as polarity, viscosity, and dissolving capacity, which directly affects the extraction efficiency toward target compounds (Sudan I). In this study, the extraction efficiency was expressed as the value of the determined amount of Sudan I divided by the added amount.
In this study, various ChCl-based deep eutectic solvents were synthesized by combining ChCl with different HBDs (Table 1), which are considered non-toxic or low-toxicity compounds in food or chemical industry. The prepared ten deep eutectic solvents (ChCl–lactate, ChCl–acetic acid, ChCl–ethylene glycol, ChCl–butanediol, ChCl–glycerol, ChCl–phenol, ChCl–p-cresol, ChCl–o-cresol, ChCl–sesamol, and ChCl–catechol) were evaluated in the extraction of Sudan I from the simulated oil sample (Fig. 4).
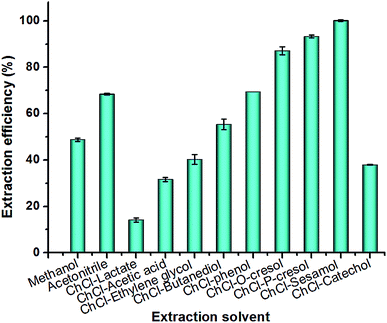 |
| Fig. 4 Effect of solvent type on Sudan I extraction efficiency. Extraction conditions: Vortex extraction at room temperature for 60 s, oil (0.20 g), extraction solvent (800 μL), three replicate tests. | |
For polyol-based deep eutectic solvents (ChCl–ethylene glycol and ChCl–butanediol), the Sudan I extraction efficiencies were lower than 60%. The deep eutectic solvent composed of ChCl and glycerol exhibited no extraction efficiency in the separation of Sudan I from the oil sample owing to the relatively high polarity of ChCl–glycerol and low polarity of Sudan I. The polyol-based deep eutectic solvents were more suitable for extracting polar targeted compounds owing to their high polarity.24,25,30,31
To our delight, most phenolic deep eutectic solvents (ChCl–phenol, ChCl–p-cresol, ChCl–o-cresol, and ChCl–sesamol) showed much higher Sudan I extraction efficiencies than those of polyol-based deep eutectic solvents. Among all tested phenol-based deep eutectic solvents, ChCl–sesamol showed the highest Sudan I extraction efficiency (near 100%) owing to hydrogen bonding between Sudan I and ChCl–sesamol, and high π–π interaction between sesamol and Sudan I (Fig. 3b).27 However, the organic functional group on the phenol ring also influenced the extraction capacity of deep eutectic solvents. For example, ortho-substituted phenols (catechol) showed decreased extraction capacities among phenol-based deep eutectic solvents owing to the decreased π–π interactions resulting from steric hindrance.
Importantly, control experiments indicated that commonly used organic solvents, such as methanol (CH3OH) and acetonitrile (CH3CN), all showed lower Sudan I extraction efficiencies (<70%) under the same extraction conditions. Therefore, the NADES composed of ChCl and sesamol was selected as the best solvent for Sudan I extraction.
3.3 Optimization of extraction conditions
To obtain a higher extraction efficiency, the extraction conditions, including the molar ratio of the NADES (ChCl/sesamol), extraction time, and the amounts of NADES and simulated oil sample, were investigated, as shown in Fig. 5.
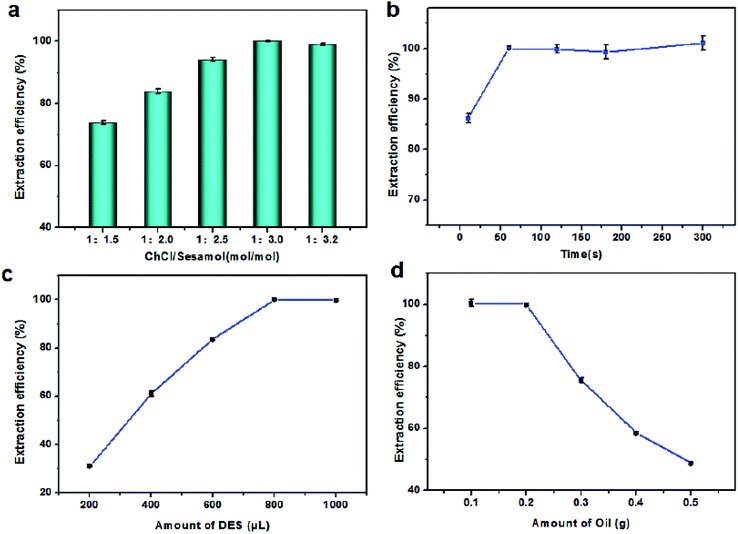 |
| Fig. 5 Effect of different conditions on Sudan I extraction efficiency from simulated oil sample using VALLME based on NADES in three replicate tests: (a) molar ratio of ChCl and sesamol; (b) extraction time; (c) NADES amount; (d) oil sample amount. Extraction conditions: Vortex extraction at room temperature for 60 s, oil (0.20 g), extraction solvent (800 μL; ChCl/sesamol molar ratio = 1 : 3), three replicate tests. | |
In this work, sesamol-based NADESs were synthesized by combining ChCl with sesamol in different molar ratios (1
:
1.5 to 1
:
3.2). As shown in Fig. 5a, as the ChCl/sesamol molar ratio increased from 1
:
1.5 to 1
:
3.0, the Sudan I extraction efficiency increased from 73% to 100%. Further increasing the molar ratio to 1
:
3.2 led to a slight decrease in the Sudan I extraction efficiency (98%), which clearly showed that the NADES extraction capacity was affected by the molar ratio of components. Therefore, the sesamol-based NADES with a ChCl/sesamol molar ratio of 1
:
3 was selected as the best extraction solvent for Sudan I.
The extraction time was also investigated, with the results shown in Fig. 5b. Notably, only extraction for 10 s afforded an extraction efficiency of about 86%. As the extraction time was increased from 10 s to 60 s, the extraction efficiency increased, reaching 100%. However, increasing the extraction time from 60 s to 300 s had no effect on the extraction efficiency. The above results indicated that VALLME using NADES was a highly efficient extraction method. In contrast, UALLME based on deep eutectic solvents requires a higher temperature (60 °C) and longer extraction time (30 min), as reported previously.31 Owing to the high viscosity of ChCl/sesamol NADES, VALLME was clearly more efficiency than UALLME due to the shorter extraction time (60 s) and milder conditions (room temperature) needed. Therefore, VALLME for 60 s at room temperature was selected for use in subsequent experiments.
The effects of amounts of NADES and oil sample were also evaluated, with the results summarized in Fig. 5(c) and (d). Increasing the amount of NADES from 200 to 800 μL enhanced the extraction efficiency of Sudan I from 31 to 100%. When the amount of oil was increased from 0.1 g to 0.2 g, the extraction efficiency showed almost no change (100%). Introducing larger amounts of oil sample (0.3–0.5 g), decreased the Sudan I extraction efficiency, probably due to that increased solubility of Sudan I in the oil sample compared with the NADES. Therefore, 800 μL of NADES and 0.2 g of oil sample were used in the extraction process.
3.4 Evaluation of analytical method
Sudan I determination was performed using an HPLC system, with the HPLC-UV chromatogram of an oil sample shown in Fig. 6. Quantitative analysis of Sudan I with a retention time of 8.3 min was achieved. Furthermore, the NADES-rich phase could be directly injected into the reversed-phase HPLC system for analysis without needing phase inversion.
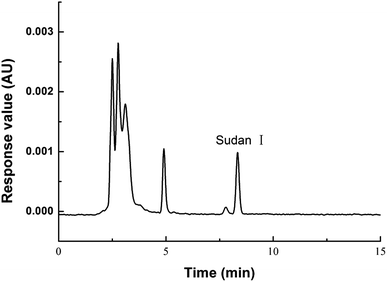 |
| Fig. 6 HPLC-UV chromatogram of a typical soybean oil sample. Detected wavelength is 478 nm. | |
To evaluate the proposed extraction method, a series of experiments were designed to evaluate the linearity, recovery, precision, limit of detection (LOD), and limit of quantification (LOQ). A seven-point calibration curve of Sudan I in the range of 0.2–100 mg kg−1 was conducted using the external standard method, as analyzed by HPLC-UV. The linear regression equation was y = 37
621.5x − 528.5, where y is the analyte peak area and x is the analyte concentration (mg kg−1). Sudan I showed good linearity with correlation coefficients (R2) of 1.000. Based on a signal-to-noise ratio of 3 and 10, the LOD and LOQ were 0.02 mg kg−1 and 0.067 mg kg−1, respectively. To assess the recovery, a soybean oil sample spiked with the standard solution of Sudan I at three different concentration levels was analyzed using three replicate measurements. As shown in Table 2, higher recoveries in the range of 93–118% were obtained. The intra-day and inter-day precision were also investigated. For intra-day precision, a typical oil sample was determined six times on the same day, and the RSD was 3.2%. For inter-day precision, the oil sample was determined on five different days, and the RSD was 4.5%. Therefore, the developed method clearly showed high recovery and precision.
Table 2 Recovery of Sudan I
Concentration in sample (mg kg−1) |
Spiked concentration (mg kg−1) |
Determined concentration (mg kg−1) |
Recovery |
Mean ± SD (n = 3). |
9.78 ± 0.29a |
5.00 |
15.50 ± 0.49 |
99.1–118% |
10.00 |
21.18 ± 0.61 |
105–117% |
15.00 |
29.63 ± 0.97 |
93.0–103% |
3.5 Sample analysis
To study the applicability of the proposed method to real sample analysis, different food samples, such as chili oil, chili sauce, and duck egg yolk, were determined. However, Sudan I was not detected in all samples tested. Therefore, these samples were spiked with Sudan I at a concentration level of 10 mg kg−1 and subjected to determination again. For comparison, these samples were also analyzed using the EU recommended method,12 with the results shown in Table 3. Notably, the recovery of this developed method was higher than that of the EU recommended method. Furthermore, the pretreatment used in our developed method was faster than that used in the EU recommended method (60 s and 1 h, respectively). Obviously, this VALLME technique based on sesamol-NADESs was highly efficient and environmentally friendly. The designed phenolic NADES composed of ChCl and sesamol was suitable for extracting weakly polar aromatic compounds through π–π interactions.
Sample |
Sudan I amount (mg kg−1) |
Spiked levels (mg kg−1) |
Detected amount (mg kg−1) |
Recovery (%) |
Method of this work |
EU recommended method |
Method of this work |
EU recommended |
ND: not detected. |
Chili oil (1) |
ND |
10 |
9.93 ± 0.35 |
9.22 ± 0.06 |
96.8–101.7 |
91.7–92.6 |
Chili oil (2) |
— |
10 |
8.95 ± 0.20 |
8.71 ± 0.06 |
87.7–91.7 |
86.7–87.5 |
Chili sauce (1) |
— |
10 |
9.00 ± 0.12 |
8.75 ± 0.21 |
89.0–91.4 |
86.0–90.0 |
Chili sauce (2) |
— |
10 |
9.12 ± 0.42 |
8.75 ± 0.11 |
86.4–93.6 |
86.7–88.2 |
Duck egg yolk (1) |
— |
10 |
9.79 ± 0.40 |
8.70 ± 0.04 |
93.3–100.5 |
86.8–87.3 |
Duck egg yolk (2) |
— |
10 |
9.45 ± 0.39 |
8.88 ± 0.08 |
90.3–98.2 |
88.2–89.4 |
4. Conclusions
In the present study, a fast, highly efficient, and green VALLME technique at room temperature based on sesamol-based NADES was successfully employed for the extraction of Sudan I from food samples, using HPLC-UV method for analysis. The NADES composed of ChCl and sesamol (1
:
3 molar ratio) showed a high extraction efficiency (near 100%) for Sudan I as a weakly polar aromatic compound under the optimized conditions. This developed method required a short extraction time (60 s) and mild temperature (room temperature). Sample analysis was conducted using different food samples, affording a higher recovery than the EU recommended method.
Conflicts of interest
The authors have no conflicts of interest to declare.
Acknowledgements
This work was supported by the China Postdoctoral Science Foundation (No. 2018T110730) and Grain & Corn Engineering Technology Research Center, State Administration of Grain (No. GA2017007).
References
- G. J. Nohynek, R. Fautz and F. Benech-Kieffer, Toxicity and human health risk of hair dyes, Food Chem. Toxicol., 2004, 42, 517–543 CrossRef CAS PubMed.
- M. Stiborová, V. Martínek and H. H. Schmeiser, Modulation of CYP1A1-mediated oxidation of carcinogenic azo dye Sudan I and its binding to DNA by cytochrome b5, Neuroendocrinol. Lett., 2006, 27, 35–38 Search PubMed.
- X. Ye, J. Zhang, H. Chen, X. Wang and F. Huang, Fluorescent nanomicelles for selective detection of Sudan dye in pluronic F127 aqueous media, ACS Appl. Mater. Interfaces, 2014, 6, 5113–5121 CrossRef CAS PubMed.
- M. Bazregar, M. Rajabi and Y. Yamini, Centrifugeless dispersive liquid-liquid microextraction based on salting-out phenomenon followed by high performance liquid chromatography for determination of Sudan dyes in different species, Food Chem., 2017, 244, 1–6 CrossRef PubMed.
- Y. Zhang, Z. Zhang and Y. Sun, Development and optimization of an analytical method for the determination of Sudan dyes in hot chilli pepper by high-performance liquid chromatography with on-line electrogenerated BrO-luminol chemiluminescence detection, J. Chromatogr. A, 2006, 1129, 34–40 CrossRef CAS PubMed.
- B. Chen and Y. Huang, Dispersive liquid-phase microextraction with solidification of floating organic droplet coupled with high-performance liquid chromatography for the determination of Sudan dyes in foodstuffs and water samples, J. Agric. Food Chem., 2014, 62, 5818–5826 CrossRef CAS PubMed.
- E. Ertaş, H. Özer and C. Alasalvar, A rapid HPLC method for determination of Sudan dyes and Para Red in red chilli pepper, Food Chem., 2007, 105, 756–760 CrossRef.
- M. Elyasi, M. A. Khalilzadeh and H. Karimi-Maleh, High sensitive voltammetric sensor based on Pt/CNTs nanocomposite modified ionic liquid carbon paste electrode for determination of Sudan I in food samples, Food Chem., 2013, 141, 4311–4317 CrossRef CAS PubMed.
- Z. Mo, Y. Zhang, F. Zhao, F. Xiao, G. Guo and B. Zeng, Sensitive voltammetric determination of Sudan I in food samples by using gemini surfactant-ionic liquid-multiwalled carbon nanotube composite film modified glassy carbon electrodes, Food Chem., 2010, 121, 233–237 CrossRef CAS.
- H. Lin, L. Gang and K. Wu, Electrochemical determination of Sudan I using montmorillonite calcium modified carbon paste electrode, Food Chem., 2008, 107, 531–536 CrossRef CAS.
- Y. Z. Wang, D. P. Wei and Y. Hong, Development of a highly sensitive and specific monoclonal antibody-based enzyme-linked immunosorbent assay (ELISA) for detection of Sudan I in food samples, Talanta, 2009, 77, 1783–1789 CrossRef CAS PubMed.
- X. Li, X. Qi and L. Zhang, 3D hierarchical magnetic hollow sphere-like CuFe2O4 combined with HPLC for the simultaneous determination of Sudan I-IV dyes in preserved bean curd, Food Chem., 2018, 241, 268–274 CrossRef PubMed.
- K. Yamjala, M. S. Nainar and N. R. Ramisetti, Methods for the analysis of azo dyes employed in food industry – A review, Food Chem., 2016, 192, 813–824 CrossRef CAS PubMed.
- P. Reboredo-Rodriguez, L. Rey-Salgueiro, J. Regueiro, C. Gonzalez-Barreiro, B. Cancho-Grande and J. Simal-Gandara, Ultrasound-assisted emulsification-microextraction for the determination of phenolic compounds in olive oils, Food Chem., 2014, 150, 128–136 CrossRef CAS PubMed.
- W. Liu, K. Zhang, J. Chen and J. Yu, Ascorbic acid and choline chloride: a new natural deep eutectic solvent for extracting tert-butylhydroquinone antioxidant, J. Mol. Liq., 2018, 260, 173–179 CrossRef CAS.
- W. Liu, B. Zong, J. Yu and Y. Bi, Ultrasonic-assisted liquid-Liquid microextraction based on natural deep eutectic solvent for the HPLC-UV determination of tert-butylhydroquinone from soybean oils, Food Anal. Methods, 2018, 11, 1797–1803 CrossRef.
- N. Lamei, M. Ezoddin and K. Abdi, Air assisted emulsification liquid-liquid microextraction based on deep eutectic solvent for preconcentration of methadone in water and biological samples, Talanta, 2017, 165, 176–181 CrossRef CAS PubMed.
- F. Aydin, E. Yilmaz and M. Soylak, Vortex assisted deep eutectic solvent (DES)-emulsification liquid-liquid microextraction of trace curcumin in food and herbal tea samples, Food Chem., 2018, 243, 442–447 CrossRef CAS PubMed.
- E. L. Smith, A. P. Abbott and K. S. Ryder, Deep eutectic solvents (DESs) and their applications, Chem. Rev., 2014, 114, 11060–11082 CrossRef CAS PubMed.
- A. Paiva, R. Craveiro, I. Aroso, M. Martins, R. L. Reis and A. R. C. Duarte, Natural deep eutectic solvents-solvents for the 21st century, ACS Sustainable Chem. Eng., 2014, 2, 1063–1071 CrossRef CAS.
- Q. Zhang, K. D. O. Vigier and S. Royer, Deep eutectic solvents: syntheses, properties and applications, Chem. Soc. Rev., 2012, 41, 7108–7146 RSC.
- S. C. Cunha and J. O. Fernandes, Extraction techniques with deep eutectic solvents, TrAC, Trends Anal. Chem., 2018, 105, 225–239 CrossRef CAS.
- R. A. Zounr, M. Tuzen, N. Deligonul and M. Y. Khuhawa, A highly selective and sensitive ultrasonic assisted dispersive liquid phase microextraction based on deep eutectic solvent for determination of cadmium in food and water samples prior to electrothermal atomic absorption spectrometry, Food Chem., 2018, 253, 277–283 CrossRef CAS PubMed.
- A. García, E. Rodríguez-Juan, G. Rodríguez-Gutiérrez, J. J. Rios and J. Fernández-Bolaños, Extraction of phenolic compounds from virgin olive oil by deep eutectic solvents (DESs), Food Chem., 2016, 197, 554–561 CrossRef PubMed.
- W. Bi, M. Tian and K. H. Row, Evaluation of alcohol-based deep eutectic solvent in extraction and determination of flavonoids with response surface methodology optimization, J. Chromatogr. A, 2013, 1285, 22–30 CrossRef CAS PubMed.
- M. W. Nam, J. Zhao, M. S. Lee, J. H. Jeong and J. Lee, Enhanced extraction of bioactive natural products using tailor-made deep eutectic solvents: Application to flavonoid extraction from Flos sophorae, Green Chem., 2015, 17, 1718–1727 RSC.
- T. Khezeli, A. Daneshfar and R. Sahraei, Emulsification liquid–liquid microextraction based on deep eutectic solvent: an extraction method for the determination of benzene, toluene, ethylbenzene and seven polycyclic aromatic hydrocarbons from water samples, J. Chromatogr. A, 2015, 1425, 25–33 CrossRef CAS PubMed.
- W. Liu, B. Zong, J. Yu and Y. Bi, Ultrasonic-assisted liquid-liquid microextraction based on natural deep eutectic solvent for the HPLC-UV determination of tert-Butylhydroquinone from soybean oils, Food Anal. Methods, 2018, 11, 1797–1803 CrossRef.
- W. Liu, K. Zhang, G. Yang and J. Yu, A highly efficient microextraction technique based on deep eutectic solvent formed by choline chloride and p-cresol for simultaneous determination of lignans in sesame oils, Food Chem., 2019, 281, 140–146 CrossRef CAS PubMed.
- T. Khezeli, A. Daneshfar and R. Sahraei, A green ultrasonic-assisted liquid–liquid microextraction based on deep eutectic solvent for the HPLC-UV determination of ferulic, caffeic and cinnamic acid from olive, almond, sesame and cinnamon oil, Talanta, 2016, 150, 577–585 CrossRef CAS PubMed.
- W. Liu, K. Zhang, J. Yu and Y. Bi, A green ultrasonic-assisted liquid-liquid microextraction based on deep eutectic solvent for the HPLC-UV determination of TBHQ in edible oils, Food Anal. Methods, 2017, 10, 3209–3215 CrossRef.
|
This journal is © The Royal Society of Chemistry 2019 |
Click here to see how this site uses Cookies. View our privacy policy here.