DOI:
10.1039/C9RA00618D
(Paper)
RSC Adv., 2019,
9, 6011-6020
Novel chalcone derivatives containing a 1,2,4-triazine moiety: design, synthesis, antibacterial and antiviral activities†
Received
24th January 2019
, Accepted 6th February 2019
First published on 19th February 2019
Abstract
A series of novel chalcone derivatives containing the 1,2,4-triazine moiety were synthesized and their structures were confirmed by 1H NMR, 13C NMR and elemental analyses. Antiviral bioassays revealed that most of the compounds exhibited good antiviral activity against tobacco mosaic virus (TMV) at a concentration of 500 μg mL−1. The designated compound 4l was 50% effective in terms of curative and protective activities against TMV with 50% effective concentrations (EC50) of 10.9 and 79.4 μg mL−1, which were better than those of ningnanmycin (81.4 and 82.2 μg mL−1). Microscale thermophoresis (MST) also showed that the binding of compound 4l to coat protein (TMV-CP) yielded a Kd value of 0.275 ± 0.160 μmol L−1, which was better than that of ningnanmycin (0.523 ± 0.250 μmol L−1). At the same time, molecular docking studies for 4l with TMV-CP (PDB code:1EI7) showed that the compound was embedded well in the pocket between the two subunits of TMV-CP. Meanwhile, compound 4a demonstrated excellent antibacterial activities against Ralstonia solanacearum (R. solanacearum), with an EC50 value of 0.1 μg mL−1, which was better than that of thiodiazole-copper (36.1 μg mL−1) and bismerthiazol (49.5 μg mL−1). The compounds act by causing folding and deformation of the bacterial cell membrane as observed using scanning electron microscopy (SEM). The chalcone derivatives thus synthesized could become potential alternative templates for novel antiviral and antibacterial agents.
1. Introduction
Plant bacterial diseases, such as citrus canker, rice bacterial leaf blight and tobacco bacterial wilt, caused by Xanthomonas axonopodis pv. citri (Xac), Xanthomonas oryzae pv. oryzae (Xoo) and Ralstonia solanacearum (R. solanacearum), respectively, can be very serious and difficult to manage in agricultural production.1,2 Tobacco mosaic virus (TMV), a classic pathogenic virus, has been extensively studied, and being a virus causes massive loss to crops and plants. This virus has been found to commonly infect nine plant families and at least 125 individual species including tobacco, tomato, pepper, potato, and cucumber, and its infection is extremely difficult to control under field conditions.3,4 Therefore, it is necessary to protect these crops against infestation and destruction by the bacterial and viral infections, and this has been effectively achieved through the use of some agrochemicals.5,6 So far, some traditional commercial viricides and bactericides, such as ribavirin, ningnanmycin, dufulin, thiodiazole-copper and bismerthiazol, are available to be used in the control of plant viral and bacterial diseases. However, the application of these traditional viricides and bactericides are limited by their negative impacts on the environment and the rapid emergence of resistance.7–9 Thus, exploring novel lead compounds with potent agricultural bioactivities is very crucial in the agricultural chemistry.10
Recent works have emphasized that the skeleton of chalcones has structural characteristics typical of diaryl enone scaffold, and are an important class of natural and synthetic bioactive compounds, which exhibit a broad spectrum of pharmacological activities,11–15 including antifungal,16 antibacterial,17 antiviral,18 inhibitors,19 anticancer,20 antiparasitic,21 anti-Alzheimer's disease,22 antitrichomonal,23 anti-trypanosoma cruzi24 activities. Meanwhile, Verma et al. demonstrated that a series of chalcones derivatives could be used to control plant viruses.25 In our previous study, we had demonstrated the synthesis of a series of (E)-3-(3,4-dimethoxyphenyl)-1-(4-(2-(quinazolin-4-ylthio)ethoxy)phenyl)prop-2-en-1-one and (E)-3-(4-(2-(4-((E)-3-(4-fluorophenyl) acryloyl) phenoxy)ethoxy)-3-methoxy phenyl)acrylic acid derivatives (Fig. 1 1a, 1b), which exhibited excellent antiviral activities.26,27
 |
| Fig. 1 Compounds previously reported against tobacco mosaic virus (TMV). | |
1,2,4-triazine and their derivatives are an important class of heterocyclic compounds and thus have been reported in a wide spectrum of biological activities,28–31 including antithrombotic,32 anti-oxidant,33 anticancer,34 antiplatelet,35 thromboxane synthetase inhibition,36 anti-inflammatory,37 antimalarial38 and α-glucosidase inhibition.39 It has also been reported that a minor modification in the structure of 1,2,4-triazine can lead to quantitative, as well as qualitative changes in the biological activities.40 Fu et al. reported potent inhibitory effect of 1,2,4-triazine and their derivatives against three cell lines (MGC-803, EC-109 and PC-3) compared to 5-fluorouracil.34 However, there are only few studies that focus on 1,2,4-triazine moiety to be potentially utilize them as an antibacterial and antiviral scaffold. Based on these, it is of urgent importance to search for novel effective agro-chemicals that have unique mechanism of action. In this work, 5,6-disubstituted-1,2,4-triazine group was introduced into the 4-position of chalcone nucleus to build a new molecular structure and the potency of which was tested in terms of biological activity (Fig. 2). The synthesized compounds were evaluated for their antibacterial and antiviral activities.
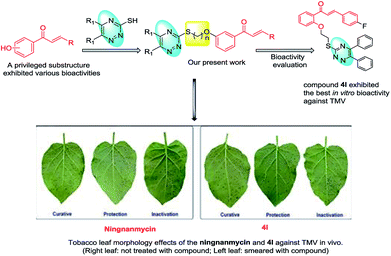 |
| Fig. 2 Design route of the title compounds 4a–4w. | |
2. Experimental
2.1. Methods and materials
Melting points of the synthesized compounds (4a–4w) were measured using XT-4 Binocular Microscope (Beijing Tech. Instrument, China) and were uncorrected. Using DMSO-d6 as the solvent and TMS as an internal standard 1H and 13C NMR spectra of the target compounds were recorded using JEOL-ECX 500 NMR spectrometer (JEOL, Japan). HRMS data were obtained using Thermo Scientific Q Exactive Hybrid Quadrupole Mass Spectrometer (Thermo Scientific Inc., St Louis, MO, USA). The microscale thermophoresis (MST) of the compounds to check the interaction with TMV CP (tobacco mosaic virus coat protein) was determined using a micro thermophoresis instrument (NanoTemper Technologies GmbH, Germany). The fluorescence spectroscopy of the compounds interacting with TMV CP was determined by FluoroMax-4 fluorescence spectrometer (HORIBA Scientific, France). The molecular docking was performed by using DS-CDocker implemented in Discovery Studio (version 4.5). All reagents and solvents were purchased from Chinese Chemical Reagent Company and were of chemically pure analytical grade reagents. The synthetic route to chalcone derivatives containing 1,2,4-triazine moiety is shown in Scheme 1. The intermediates 1, 2 and 3 were prepared according to the methods already reported in the literature.26,27,41
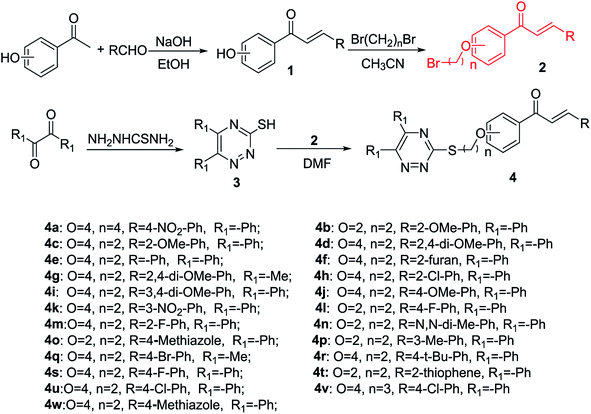 |
| Scheme 1 Synthetic route to title compounds 4a–4w. | |
2.2. General procedure for the synthesis of intermediates 1 and 2
As shown in Scheme 1, using 1-(4-hydroxyphenyl) ethan-1-one or 1-(2-hydroxyphenyl)ethan-1-one and different substituted benzaldehyde as the initial materials in ethanol under ice-bath conditions in the presence of different substituted chalcone, intermediate 2 was favourably prepared according to the previously reported procedure.26,27 The 1H NMR, 13C NMR and HMRS spectra of the intermediate 2 have been provided in the ESI.†
2.3. General procedure for the synthesis of intermediate 3
A general synthesis of 5,6-diphenyl-1,2,4-triazine-3-thiol or 5,6-dimethyl-1,2,4-triazine-3-thiol is shown in Scheme 1. Benzil (10 mmol) or biacetyl (10 mmol) with thio-semicarbazide (11 mmol) was refluxed in acetic acid (20 mL) and water (35 mL), to obtain the corresponding 5,6-diphenyl-1,2,4-triazine-3-thiol or 5,6-dimethyl-1,2,4-triazine-3-thiol (3).41
2.4. General procedure for the synthesis of title compounds 4a–4w
The synthetic route to the title compounds, (E)-1-(4-(2-((5,6-diphenyl-1,2,4-triazin-3-yl)thio)ethoxy)phenyl)-3-(2-substituted aromatic groups)prop-2-en-1-one or (E)-1-(2-(2-((5,6-diphenyl-1,2,4-triazin-3-yl)thio)ethoxy)phenyl)-3-(2-substituted aromatic groups)prop-2-en-1-one derivatives (4a–4w) are shown in Scheme 1. A mixture of intermediate 3 (10 mmol) and anhydrous potassium carbonate (30 mmol) in dimethylformamide (DMF, 40 mL) was stirred at room temperature for 1 h. Then, the intermediate 2 (12 mmol) was added to the above solution and stirred at room temperature for 6–8 h. Upon completion of reaction (indicated by TLC), the mixture was extracted, the solvent was removed under vacuum, and the crude product was then separated by column chromatography with petroleum ether/ethyl acetate (3
:
1 v/v) to obtain the designated title compounds 4a–4w at different yields. The 1H NMR, 13C NMR and HMRS spectra of the designated title compounds 4a–4w are also provided in the ESI.†
3. Results and discussion
3.1. Spectral properties
1H NMR, 13C NMR and HRMS spectra of the target compounds are provided in the ESI.† In 1H NMR spectra, multiplet signals at δ 8.71–6.33 ppm indicate the presence of protons in olefinic bonds and aromatic nuclei, and a singlet at δ 4.59–4.20 ppm and 3.80–3.40 ppm reveals the presence of –OCH2– and –SCH2– groups, respectively. The absorption signals at δ 192.94–187.20 in 13C NMR spectra confirm the presence of –C
O group, while the absorption signals at δ 67.99–55.53 and 55.52–29.31 ppm in 13C NMR spectra confirm the presence of –OCH2– and –SCH2– groups, respectively. In the HRMS spectra of the title compounds, the characteristic absorption signals of [M + H]+ ions were obtained, which agree with their molecular weights.
3.2. Antiviral activity of the title compounds against TMV
Using N. tabacun L. leaves under the same age as that of test subjects, the curative, protective and inactivation activities against TMV (in vivo) at a concentration of 500 μg mL−1 were evaluated by the half-leaf blight spot method9,26,27,42 and the obtained results are shown in Table 1. The preliminary bioassay results demonstrated that the inhibitory rates of title compounds (4a–4w) against TMV ranged from 30.6 to 65.6% in terms of their curative activities, while their protective and inactivation activities ranged from 34.3 to 62.7% and 23.3 to 86.8%, respectively. Among them, the designated compounds, 4g, 4j, 4k, 4l, 4o, 4u and 4w afforded better curative activities against TMV at 500 μg mL−1, with 54.9%, 57.1%, 55.3%, 62.7%, 61.4, 65.6% and 60.6%, respectively. These compounds were found to exhibit better antiviral activities than ningnanmycin (45.7%). In addition, 4b, 4g, 4k, 4l, 4u and 4v compounds exhibited significant protective activities against TMV at 500 μg mL−1, with 58.7%, 55.5%, 56.8%, 58.6%, 62.7% and 56.2%, respectively, which were even better than that of ningnanmycin (53.4%). The inactivation activity of the compound 4k at 500 μg mL−1 was 86.8%, which was also better than that of ningnanmycin (77.3%).
Table 1 Antiviral activities of the target compounds against TMV in vivo at 500 μg mL−1
Compd. |
Curative activitya (%) |
Protective activitya (%) |
Inactivation activitya (%) |
Average of three replicates. A commercial agricultural antiviral agent ningnanmycin was used for comparison of antiviral activities. |
4a |
49.4 ± 0.8 |
46.3 ± 7.9 |
60.9 ± 2.4 |
4b |
40.4 ± 0.8 |
58.7 ± 1.3 |
43.8 ± 4.2 |
4c |
30.6 ± 0.4 |
34.3 ± 4.0 |
23.3 ± 1.6 |
4d |
48.3 ± 1.3 |
44.6 ± 4.4 |
55.2 ± 1.8 |
4e |
56.4 ± 1.0 |
41.7 ± 4.6 |
46.3 ± 0.1 |
4f |
55.6 ± 2.3 |
38.7 ± 2.4 |
50.8 ± 1.9 |
4g |
54.9 ± 4.6 |
55.5 ± 4.9 |
64.6 ± 0.8 |
4h |
53.6 ± 2.6 |
46.9 ± 2.8 |
51.6 ± 1.7 |
4i |
54.9 ± 9.7 |
42.2 ± 0.5 |
52.1 ± 2.5 |
4j |
57.1 ± 1.1 |
51.7 ± 0.1 |
62.9 ± 0.3 |
4k |
55.3 ± 0.6 |
56.8 ± 0.9 |
86.8 ± 1.0 |
4l |
62.7 ± 0.3 |
58.6 ± 1.3 |
62.6 ± 2.0 |
4m |
45.2 ± 4.3 |
39.5 ± 3.2 |
51.1 ± 1.1 |
4n |
44.1 ± 1.2 |
51.1 ± 0.3 |
23.9 ± 9.2 |
4o |
61.4 ± 1.4 |
55.9 ± 0.3 |
23.3 ± 0.1 |
4p |
37.8 ± 1.7 |
44.4 ± 2.3 |
40.1 ± 1.7 |
4q |
44.8 ± 0.5 |
47.4 ± 4.8 |
43.2 ± 4.0 |
4r |
41.7 ± 1.8 |
20.1 ± 0.8 |
54.6 ± 2.9 |
4s |
43.1 ± 0.5 |
27.3 ± 1.0 |
51.2 ± 2.2 |
4t |
56.1 ± 0.4 |
51.3 ± 1.9 |
46.2 ± 7.6 |
4u |
65.6 ± 0.1 |
62.7 ± 0.7 |
51.6 ± 0.7 |
4v |
50.7 ± 1.8 |
56.2 ± 2.3 |
38.8 ± 2.6 |
4w |
60.6 ± 0.5 |
50.8 ± 0.1 |
49.6 ± 0.9 |
Ningnanmycinb |
45.7 ± 2.6 |
53.4 ± 2.4 |
77.3 ± 1.6 |
To compare the antiviral activity of the synthesized compounds (4a–4w), EC50 values of 4g, 4k, 4l and 4u were calculated and have been summarized in Table 2. It could be seen that compounds 4g, 4k, 4l and 4u exhibited higher curative activity than ningnanmycin (81.5 μg mL−1), with EC50 values of 17.5, 21.8, 10.9 and 72.2 μg mL−1, respectively. Compounds 4l and 4u exhibited the best protective activity against TMV, with EC50 values of 79.4 and 52.4 μg mL−1, respectively, which were better than that of ningnanmycin (82.3 μg mL−1). Notably, compound 4k was found to exhibit significant inactivation activity (2.5 μg mL−1), which was similar to ningnanmycin (1.1 μg mL−1). It can be implied from these results that most of these novel chalcone derivatives containing 1,2,4-triazine moiety could be further studied as a potential alternative template in the search for novel antiviral agents.
Table 2 EC 50 values of the 4g, 4k, 4l and 4u against TMV in vivoa
Compd. |
Against TMV |
Regression equation |
r2 |
EC50 |
Average of three replicates. A commercial agricultural antiviral agent ningnanmycin was used for comparison of antiviral activities. |
4g |
Curative activity |
y = 0.3961x + 4.5075 |
0.9707 |
17.5 ± 0.34 |
Protection activity |
y = 0.4213x + 3.9441 |
0.9534 |
320.8 ± 0.19 |
Inactivation activity |
y = 0.5973x + 4.0952 |
0.9654 |
32.7 ± 0.03 |
4k |
Curative activity |
y = 0.3432x + 4.5403 |
0.9006 |
21.8 ± 0.05 |
Protection activity |
y = 0.2912x + 4.2541 |
0.9415 |
364.3 ± 0.21 |
Inactivation activity |
y = 0.2248x + 4.9103 |
0.9939 |
2.5 ± 0.04 |
4l |
Curative activity |
y = 0.3387x + 4.6487 |
0.9347 |
10.9 ± 0.02 |
Protection activity |
y = 0.3195x + 4.3930 |
0.9078 |
79.4 ± 0.22 |
Inactivation activity |
y = 0.5142x + 4.1551 |
0.9541 |
43.9 ± 0.03 |
4u |
Curative activity |
y = 0.4456x + 4.1718 |
0.9690 |
72.2 ± 4.04 |
Protection activity |
y = 0.4467x + 4.2318 |
0.9440 |
52.4 ± 0.06 |
Inactivation activity |
y = 0.2934x + 4.4604 |
0.9156 |
68.9 ± 0.19 |
Ningnanmycinb |
Curative activity |
y = 0.4415x + 4.1563 |
0.9720 |
81.4 ± 0.28 |
Protection activity |
y = 0.4732x + 4.0939 |
0.9097 |
82.2 ± 0.05 |
Inactivation activity |
y = 0.4591x + 4.9815 |
0.9056 |
1.1 ± 0.02 |
3.3. Structure–activity relationship (SAR) of the title compounds against TMV
An analysis of Tables 1 and 2 reveals that variations in the structural features of chalcone derivatives containing 1,2,4-triazine moiety could play a role in their anti-TMV activities. First, the 4-F-Ph, 4-methylthiazole and 4-Cl-Ph groups at –R position greatly improved the curative activities of the title compounds against TMV. For instance, the curative activities of the target compounds 4l (R = 4-F-Ph), 4u (R = 4-Cl-Ph) and 4o (R = 4-methylthiazole) were 62.7%, 65.6% and 61.4%, respectively, which were better than that of other substituent groups. In addition, when –R was 2-O-Me-Ph, 2,4-di-OMe-Ph, 3-NO2-Ph, 4-F-Ph, 4-methylthiazole and 4-Cl-Ph groups, the protective activities of the corresponding compounds 4b, 4g, 4k, 4l, 4o, 4u and 4w at 500 μg mL−1 were 58.7%, 55.5%, 56.8%, 58.6%, 55.9%, 62.7% and 56.2%, respectively, which were better than that of ningnanmycin (53.4%). Furthermore, when –R was 3-NO2-Ph group, the inactivation activity of the compound 4k at 500 μg mL−1 was 86.8%, which was better than that of ningnanmycin (77.3%). Second, when R1 was substituted by a –CH3 group, the corresponding compound 4g (R1 = Me, R = 3,4-di-OMe) had better curative, protection and inactivation effect against TMV than the compound bearing a –Ph (4c) group. When R was substituted with a 3,4-di-OMe-Ph, 3-NO2-Ph, 4-F-Ph or 4-Cl-Ph group, the obtained compounds exhibited better curative, protection and inactivation activities against TMV. For instance, the curative, protection and inactivation effects of the target compounds 4g (R = 3,4-di-OMe-Ph, R1 = –Me), 4k (3-NO2-Ph, R1 = –Ph), 4l (4-F-Ph, R1 = –Ph) and 4u (4-Cl-Ph, R1 = –Ph) were 54.9%, 55.5% and 64.6%, 55.3%, 56.8% and 86.8%, 62.7%, 58.6% and 62.6%, 65.6%, 62.7% and 51.6%, respectively, which were better than that of ningnanmycin (45.7%, 53.4% and 77.3%) (Fig. 3).
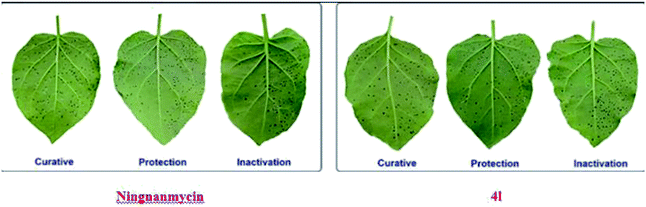 |
| Fig. 3 Tobacco leaf morphology effects of the ningnanmycin and 4l against TMV in vivo. (Right leaf: not treated with compound; left leaf: smeared with compound). | |
3.4. Binding sites of 4l, 4d, 4o and ningnanmycin to TMV-CP
To further analyze the interactions between the title compounds and TMV coat protein (TMV CP), the microscale thermophoresis (MST) analysis was used.43 The MST results as summarized in Fig. 4 and Table 3 show that the binding of compounds 4l, 4d, 4o and ningnanmycin to TMV CP protein yielded Kd values of 0.275 ± 0.160 μmol L−1, 81.744 ± 22.310 μmol L−1, 245.285 ± 63.860 μmol L−1 and 0.523 ± 0.250 μmol L−1, respectively. As indicated in MST, 4l and ningnanmycin share strong affinity, while 4d shares moderate affinity, which is contrary to 4o as it shares weak affinity. These results exhibited that the combining capacity in the following order of 4l > ningnanmycin > 4d > 4o is consistent with the trends of the screening of antiviral activities. Based on the experimental results it can be predicted that compound 4l may interact with TMV CP. As depicted in Fig. 4 and Table 3, the bioactivity was mainly determined by the electrostatic interactions, and the activity could have been enhanced by the presence of aromatic ring which is rich in electrons for absorption. For example, when R was substituted with a 4-F-Ph group, the corresponding compound (4l) exhibited a stronger combining capacity, with Kd values of 0.275 ± 0.160 μmol L−1, as compared to that of compounds 4d (81.744 ± 22.310 μmol L−1) and 4o (245.285 ± 63.860 μmol L−1), which was substituted with a 2,4-di-OMe-Ph or 4-methylthiazole.
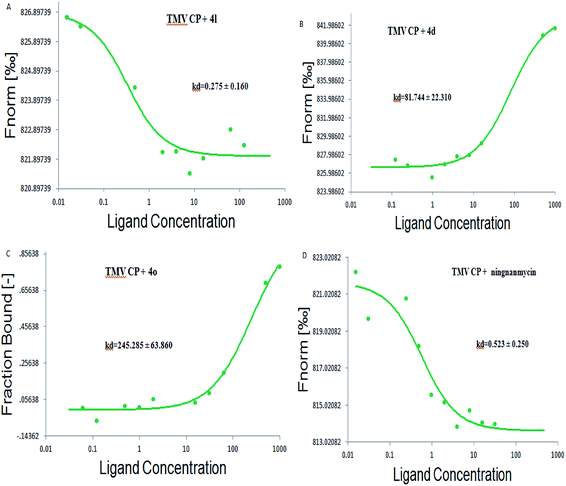 |
| Fig. 4 Microscale thermophoresis (MST) of 4l (A); 4d (B); 4o (C) and ningnanmycin (D). | |
Table 3 The dissociation constant of 4l, 4d, 4o and ningnanmycin with TMV-coat protein (TMV-CP)
Compd. |
Kd (μmol L−1) |
4l |
0.275 ± 0.16 |
4d |
81.744 ± 22.31 |
4o |
245.285 ± 63.86 |
Ningnanmycin |
0.523 ± 0.25 |
3.5. Molecular docking of 4l and TMV-CP
Molecular docking studies (Fig. 5) for 4l with TMV-CP (PDB code:1EI7)44 have shown that the compound was well-embedded between the two subunits of TMV-CP (including residues of GLU131, LYS268, GLN257, ALA74, ASN73, PHE12 and TYR139). Previous reports have demonstrated that these residues play important roles in the self-assembly of TMV particles.44,45 Some hydrophobic aromatic components and clusters such as PHE12, LYS268 and TRY139, play a key role in the continuous hydrophobic interactions within each ring of this assembly.45 In addition, there are strong interactions between GLU131, ASN73, ALA74 and GLN257 and other subunits of TMV-CP.44 Moreover, there are also notable effects of hydrogen bonding, π-sulfur, π-alkyl, and alkyl between the molecules and the above residues in TMV-CP as depicted in Fig. 5. For instance, ASN73 showed strong hydrogen bond with 4l (2.35 Å), and triazine demonstrates π-alkyl interaction with the residue VAL-74. There was also π-alkyl interaction between the phenyl group and the residue VAL-74. These interactions between molecules and TMV-CP are likely to weaken the interaction of two subunits of TMV-CP, thereby preventing the self-assembly of TMV particle, as well as the binding capability with TMV-CP. The results of molecular docking studies were consistent with the experimental results (protection and inactivation activities) and support that the chalcone derivatives may be potential lead structures for developing novel anti-TMV agents.
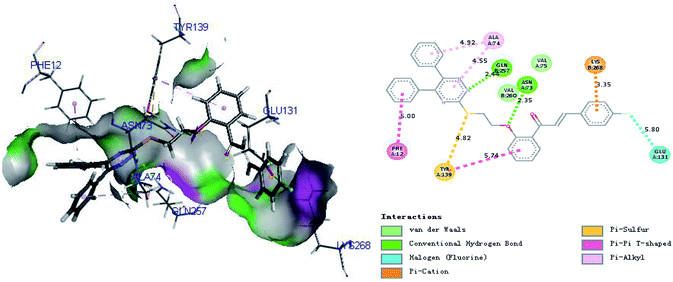 |
| Fig. 5 The binding mode of compound 4l docked with TMV-CP. | |
3.6. Antibacterial activities
The antibacterial activities of the title compounds against Xoo, R. solanacearum and Xac were evaluated at 100 μg mL−1 using a turbidimeter.2,9,46 The commercial bactericide, bismerthiazol and thiadiazole-copper were tested as a control under the same conditions, and the obtained results are summarized in Table 4. It can be observed from this table that most of the target compounds exhibited considerable antibacterial effects against Xoo, R. solanacearum and Xac. For instance, compounds 4c, 4d, 4g and 4n presented antibacterial activities against Xoo at 100 μg mL−1, with the inhibition rates of 62.9%, 60.5%, 61.8% and 69.0%, respectively, which are better than that of bismerthiazol (56.1%) and thiadiazole-copper (32.7%). The inhibition rates of compounds 4c, 4d, 4g and 4n against Xoo at 50 μg mL−1 were 44.9%, 39.9%, 44.5% and 42.3%, respectively, which were better than that of thiadiazole-copper (27.2%) and similar to bismerthiazol (49.3%). Moreover, compounds 4a, 4j, 4l, 4n, 4r, 4s and 4w presented excellent antibacterial activities against R. solanacearum at 100 μg mL−1, with the inhibition rates of 93.7%, 87.9%, 88.6%, 99.9%, 96.7%, 85.1% and 94.2%, respectively, which are higher as compared to bismerthiazol (52.1%) and thiadiazole-copper (80.2%). Compounds 4a, 4j, 4l, 4n, 4r, 4s and 4w revealed excellent antibacterial activities against R. solanacearum at 50 μg mL−1 with the inhibition rates of 74.2%, 70.0%, 82.9%, 74.8%, 71.1%, 80.3% and 87.0%, respectively. These rates were also higher compared to bismerthiazol (44.2%) and thiadiazole-copper (48.3%). In addition, compounds 4a, 4c, 4d, 4e, 4j, 4m and 4n at 100 and 50 μg mL−1, exhibited better antibacterial activities against Xac than bismerthiazol (70.5% and 33.6%) and thiodiazole-copper (75.9% and 29.5%), with the values of 81.1% and 66.5%, 81.2% and 60.9%, 83.3% and 60.6%, 93.1% and 40.0%, 96.8% and 48.6%, 88.0% and 62.7% and 87.7% and 79.4%, respectively.
Table 4 Inhibition effect of the title compounds against Xoo, R. solanacearum and Xacb
Compd. |
Inhibition (%) |
Xoo |
R. solanacearum |
Xac |
100 μg mL−1 |
50 μg mL−1 |
100 μg mL−1 |
50 μg mL−1 |
100 μg mL−1 |
50 μg mL−1 |
BT: bismerthiazol; TC: thiadiazole-copper; average of three replicates. A commercial agricultural antibacterial agent bismerthiazol and thiadiazole-copper were used for comparison of antibacterial activities. |
4a |
35.4 ± 5.4 |
32.7 ± 8.2 |
93.7 ± 4.5 |
74.2 ± 0.2 |
81.1 ± 3.5 |
66.5 ± 1.7 |
4b |
47.9 ± 2.0 |
34.7 ± 2.7 |
63.4 ± 6.2 |
62.2 ± 7.0 |
45.9 ± 7.2 |
39.9 ± 4.8 |
4c |
62.9 ± 4.8 |
44.9 ± 2.3 |
72.2 ± 3.2 |
62.7 ± 6.2 |
81.2 ± 2.7 |
60.9 ± 2.0 |
4d |
60.5 ± 2.4 |
39.9 ± 2.5 |
78.5 ± 3.0 |
77.7 ± 1.6 |
83.3 ± 5.4 |
60.6 ± 1.7 |
4e |
25.4 ± 5.4 |
22.7 ± 8.2 |
74.7 ± 0.5 |
53.8 ± 3.4 |
93.1 ± 9.0 |
40.0 ± 3.0 |
4f |
27.9 ± 2.0 |
24.7 ± 2.7 |
70.3 ± 1.6 |
57.6 ± 6.6 |
56.4 ± 2.4 |
51.3 ± 4.1 |
4g |
61.8 ± 5.7 |
44.5 ± 3.6 |
83.5 ± 4.5 |
81.8 ± 1.8 |
63.0 ± 1.2 |
49.3 ± 3.7 |
4h |
18.8 ± 3.5 |
24.0 ± 5.6 |
71.9 ± 2.1 |
70.3 ± 3.2 |
75.4 ± 3.3 |
48.4 ± 0.6 |
4i |
18.0 ± 2.6 |
11.2 ± 3.0 |
83.7 ± 2.8 |
79.8 ± 2.0 |
53.0 ± 1.0 |
33.2 ± 9.3 |
4j |
13.9 ± 3.0 |
17.5 ± 3.9 |
87.9 ± 2.2 |
79.0 ± 2.3 |
96.8 ± 4.4 |
48.6 ± 3.3 |
4k |
27.3 ± 7.1 |
11.2 ± 4.3 |
80.3 ± 4.4 |
79.7 ± 1.5 |
70.2 ± 3.3 |
40.7 ± 3.8 |
4l |
17.5 ± 3.9 |
12.5 ± 3.9 |
88.6 ± 4.5 |
82.9 ± 0.8 |
59.4 ± 2.2 |
48.0 ± 5.3 |
4m |
17.4 ± 4.3 |
15.1 ± 4.6 |
81.2 ± 7.6 |
72.6 ± 4.7 |
88.0 ± 6.2 |
62.7 ± 3.8 |
4n |
69.0 ± 4.2 |
42.3 ± 1.2 |
99.9 ± 2.6 |
74.8 ± 1.9 |
87.7 ± 4.1 |
79.4 ± 1.3 |
4o |
17.2 ± 4.6 |
15.1 ± 4.6 |
72.5 ± 2.4 |
68.7 ± 1.8 |
39.3 ± 6.0 |
27.7 ± 6.0 |
4p |
15.6 ± 4.2 |
11.1 ± 1.2 |
63.9 ± 8.3 |
58.0 ± 14.3 |
64.5 ± 1.1 |
50.2 ± 10.5 |
4q |
26.5 ± 1.0 |
25.2 ± 2.1 |
80.3 ± 0.6 |
70.9 ± 2.8 |
39.8 ± 1.8 |
29.1 ± 3.1 |
4r |
35.4 ± 3.3 |
28.8 ± 4.3 |
96.7 ± 2.7 |
71.1 ± 1.5 |
41.8 ± 2.6 |
40.9 ± 1.4 |
4s |
19.2 ± 4.6 |
13.2 ± 4.6 |
85.1 ± 3.6 |
80.3 ± 3.4 |
65.1 ± 4.1 |
45.8 ± 9.1 |
4t |
12.2 ± 0.8 |
11.6 ± 3.6 |
75.8 ± 3.0 |
61.7 ± 3.2 |
63.9 ± 1.2 |
51.7 ± 2.7 |
4w |
42.1 ± 6.2 |
31.0 ± 6.0 |
94.2 ± 2.6 |
87.0 ± 3.5 |
70.7 ± 2.1 |
65.7 ± 4.9 |
BTa |
56.1 ± 7.3 |
49.3 ± 5.4 |
52.1 ± 3.4 |
44.2 ± 3.9 |
70.5 ± 1.5 |
33.6 ± 1.7 |
TCa |
37.2 ± 0.9 |
27.2 ± 3.2 |
80.2 ± 4.0 |
48.3 ± 4.8 |
75.9 ± 1.5 |
29.5 ± 4.3 |
Based on these preliminary results of bioassays, EC50 values of some of the compounds showed excellent antibacterial activities against Xoo, Xac and R. solanacearum as indicated in Table 5. As can be seen from Table 5, compounds 4a and 4e exhibited satisfactory in vitro antibacterial activities against Xac, with the EC50 values of 53.6 and 67.0 μg mL−1, which were higher than that of bismerthiazol (153.7 μg mL−1) and thiodiazole-copper (77.8 μg mL−1) (Table 5). Also, compound 4g was found to exhibit higher antibacterial activity against Xoo (76.7 μg mL−1), than bismerthiazol (94.5 μg mL−1). On the other hand, as shown in Table 5, the title compounds 4a–4w exhibited in vitro antibacterial activities against R. solanacearum, with the EC50 values of 0.1–93.9 μg mL−1. The target compounds 4a, 4g, 4j, 4l, 4s, 4t and 4v showed excellent in vitro antibacterial activities against R. solanacearum, with the EC50 values of 0.1, 0.1, 0.3, 0.4, 0.2, 0.1 and 2.7 μg mL−1, respectively. These values were comparable with thiodiazole-copper (36.1 μg mL−1) and bismerthiazol (49.5 μg mL−1) as shown in Table 5.
Table 5 EC50 values of some title compounds against Xoo, Xac and R. solanacearumb
Tested bacteriasl |
Compd. |
Regression equation |
r2 |
EC50 (μg mL−1) |
BT: bismerthiazol; TC: thiadiazole-copper. A commercial agricultural antibacterial agent bismerthiazol and thiadiazole-copper were used for comparison of antibacterial activities. |
Xac |
4a |
y = 1.1509x + 3.0094 |
0.9084 |
53.6 ± 4.5 |
4c |
y = 0.3984x + 3.9390 |
0.9265 |
460.4 ± 2.4 |
4d |
y = 0.3020x + 4.3584 |
0.9155 |
133.2 ± 4.7 |
4e |
y = 0.5608x + 3.9757 |
0.9644 |
67.0 ± 4.9 |
BTa |
y = 0.3926x + 4.1415 |
0.9072 |
153.7 ± 5.9 |
TCa |
y = 1.7056x + 1.7748 |
0.9161 |
77.8 ± 2.3 |
Xoo |
4c |
y = 0.7929x + 3.0664 |
0.9622 |
274.5 ± 5.0 |
4g |
y = 0.9379x + 3.2319 |
0.9558 |
76.7 ± 6.2 |
4n |
y = 0.7539x + 3.4235 |
0.9573 |
123.3 ± 6.3 |
BTa |
y = 1.5696x + 1.8988 |
0.9551 |
94.5 ± 4.3 |
TCa |
y = 0.9843x + 3.3369 |
0.9475 |
48.9 ± 8.3 |
RS |
4a |
y = 0.3081x + 5.3419 |
0.9157 |
0.1 ± 3.6 |
4b |
y = 1.3629x + 3.9942 |
0.9383 |
1.0 ± 4.2 |
4c |
y = 0.6011x + 3.9125 |
0.9449 |
64.4 ± 5.6 |
4d |
y = 0.8807x + 3.5862 |
0.9583 |
42.5 ± 8.1 |
4e |
y = 0.9347x + 4.2405 |
0.9546 |
6.5 ± 5.1 |
4f |
y = 0.3225x + 4.7271 |
0.9655 |
7.02 ± 2.8 |
4g |
y = 0.4227x + 5.4036 |
0.9696 |
0.1 ± 0.4 |
4h |
y = 0.3235x + 4.3949 |
0.9113 |
74.2 ± 6.1 |
4i |
y = 2.0693x + 2.9527 |
0.9540 |
9.8 ± 2.1 |
4j |
y = 0.5553x + 5.2478 |
0.9379 |
0.3 ± 0.6 |
4k |
y = 1.5277x + 3.5958 |
0.9378 |
8.3 ± 0.9 |
4l |
y = 0.4623x + 5.1549 |
0.9939 |
0.4 ± 4.5 |
4m |
y = 1.3267x + 2.5265 |
0.9339 |
73.2 ± 6.1 |
4n |
y = 0.6065x + 3.9014 |
0.9395 |
64.8 ± 4.8 |
4o |
y = 2.1930x + 2.0260 |
0.9601 |
24.3 ± 5.2 |
4p |
y = 1.1930x + 3.0290 |
0.9301 |
22.7 ± 4.8 |
4q |
y = 0.2018x + 4.6019 |
0.9719 |
93.9 ± 7.8 |
4r |
y = 2.3888x + 1.4419 |
0.9198 |
30.8 ± 5.1 |
4s |
y = 0.4483x + 5.3221 |
0.9351 |
0.2 ± 0.2 |
4t |
y = 0.4271x + 5.3495 |
0.9695 |
0.1 ± 0.5 |
4u |
y = 0.6484x + 3.7942 |
0.9415 |
72.4 ± 5.5 |
4v |
y = 0.6851x + 4.7031 |
0.9378 |
2.7 ± 3.5 |
4w |
y = 0.9945x + 3.4690 |
0.9266 |
34.6 ± 2.8 |
BTa |
y = 1.0223x + 3.2674 |
0.9095 |
49.5 ± 8.5 |
TCa |
y = 0.7337x + 3.8570 |
0.9042 |
36.1 ± 3.9 |
3.7. Structure–activity relationships (SAR) of antibacterial activities
Table 4 reveals that the antibacterial activities of the title compounds were considerably affected by the structural variations in the chalcone derivatives that contain 1,2,4-triazine moiety. Notably, all the title compounds exhibited better anti R. solanacearum and anti Xac activities than their corresponding anti Xoo activities as could be observed from the results presented in Table 4. The antibacterial activities of the title compounds were also affected by the nature or identity of different groups attached to R. For instance, the designated compounds 4a (R = –NO2-Ph), 4j (R = 4-OMe-Ph), 4l (R = 4-F-Ph), 4n (R = N,N-di-Me-Ph), 4r (R = isopropyl-Ph) and 4w (R = 4-methylthiazole) exhibited significant anti R. solanacearum effects at 100 μg mL−1, with the inhibition rates of 93.7%, 87.9%, 88.6%, 99.9%, 96.7% and 94.2%, respectively. These compounds were found to be more active compared to other tested compounds. However, when R was substituted with 4-NO2-Ph, 2,4-di-OMe-Ph, phenyl, 4-OMe-Ph, 2-F-Ph and N,N-di-Me-Ph groups, the activities of the corresponding compounds 4a, 4d, 4e, 4j, 4m and 4n, against Xac at 100 μg mL−1 were 81.1%, 83.3%, 93.1%, 96.8%, 88.0% and 87.7%, respectively, which were higher than that of bismerthiazol (70.5%) and thiadiazole-copper (75.9%).
3.8. SEM study
In order to further explore the mechanism of antibacterial action against R. solanacearum, scanning electron microscopic (SEM) studies were carried out using the designated compound 4a and the observed SEM micrographs are shown in Fig. 6. It could be noted that the compounds act by causing the bacterial cell membranes to wrinkle. Wrinkling of the cell membranes became more obvious with an increase in the concentration of compound 4a. For instance, when the concentration was 12.5 μg mL−1, the cell membrane began to wrinkle (Fig. 6B). Whereas, a further increase in the concentration to 50 μg mL−1, it results in the development of folds in the cell membrane (Fig. 6C). In contrast, without any treatment with compound 4a, the bacterial cells were smooth and the cell membrane was intact (Fig. 6A). The results from SEM provide clear evidence that the compound 4a exhibited antibacterial effects on the cell, and this effect might eventually results in cell death.
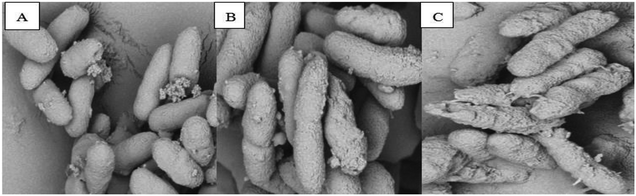 |
| Fig. 6 SEM images for R. solanacearum after incubated in different concentration of compound 4a (A) 0 mg mL−1, (B) 12.5 mg mL−1, and (C) 50 mg mL−1. Scale bar for (A, B and C) are 2 μm. | |
4. Conclusions
With the aim to develop novel, highly-efficient and environmentally benign agrochemicals, 1,2,4-triazine scaffold was introduced into chalcone structure to synthesize chalcone derivatives, and also their in vivo antibacterial and antiviral activities were evaluated. The obtained bioassay results revealed that some of the title compounds exhibited excellent antibacterial and antiviral activities and notably they were better than the commercial agents. Compound 4l exhibited significant curative and protective activities against tobacco mosaic virus (TMV), with the EC50 values of 10.9 μg mL−1 and 79.4 μg mL−1, which was better than that of ningnanmycin (81.4 μg mL−1 and 82.2 μg mL−1). Microscale thermophoresis (MST) showed that the binding of compound 4l to coat protein (TMV-CP) yielded Kd values of 0.275 ± 0.160 μmol L−1, which was better than that of ningnanmycin (0.523 ± 0.250 μmol L−1). Molecular docking studies for 4l with TMV-CP (PDB code: 1EI7) showed that the compound was embedded well in the pocket between the two subunits of TMV-CP. Compounds 4a, 4g, 4j, 4s, 4l and 4t demonstrated excellent antibacterial activities against R. solanacearum, with the EC50 values of 0.1, 0.1, 0.3, 0.46 and 0.1 μg mL−1, which were better than that of thiodiazole-copper (36.1 μg mL−1) and bismerthiazol (49.5 μg mL−1). The observed antibacterial activity could be due to folding and deformation of the bacterial cell membrane by the addition of compound as observed using SEM. All these results support that the chalcone derivatives containing 1,2,4-triazine moiety possess antibacterial effects, and thus could be further studied as potential alternative templates in the search for novel antiviral and antibacterial agents.
Conflicts of interest
The authors declare that they have no competing interests.
Acknowledgements
The authors gratefully acknowledge the financial support of the National Key Research and Development Program of China (No. 2017YFD0200506) and Science and Technology Project of Guizhou Province (No. 20185781, 20171028).
References
- L. F. Zou, L. I. Yu-Rong and G. Y. Chen, Agric. Sci. China, 2011, 10, 1139–1150 CrossRef CAS.
- P. Li, P. Tian, Y. Chen, Y. Z. Chen, S. Yang and B. A. Song, Pest Manage. Sci., 2017, 11, 106 Search PubMed.
- P. Y. Wang, W. B. Shao, H. T. Xue, B. A. Song and S. Yang, Res. Chem. Intermed., 2017, 43, 6115–6130 CrossRef CAS.
- G. Han, L. Chen and Q. Wang, J. Agric. Food Chem., 2018, 66, 780–788 CrossRef CAS PubMed.
- A. Hazra, S. Mondal and A. Maity, Eur. J. Med. Chem., 2011, 46, 2132–2140 CrossRef CAS PubMed.
- H. Khalilullah and M. J. Ahsan, Mini-Rev. Med. Chem., 2012, 12, 789 CrossRef CAS PubMed.
- P. Y. Wang, L. Zhou, J. Zhou, Z. B. Wu, W. Xue, B. A. Song and S. Yang, Bioorg. Med. Chem. Lett., 2016, 26, 1214–1217 CrossRef CAS PubMed.
- X. Wang, P. Li, Z. Li, J. Yin, M. He, W. Xue, Z. Chen and B. A. Song, J. Agric. Food Chem., 2013, 61, 9575–9582 CrossRef CAS PubMed.
- X. M. Zhong, X. B. Wang, L. J. Chen and W. Xue, Chem. Cent. J., 2017, 11, 106 CrossRef PubMed.
- Y. Lu and X. H. Gan, Molecules, 2017, 22, 658 CrossRef PubMed.
- R. Schobert, B. Biersack and A. Dietrich, J. Med. Chem., 2009, 52, 241–246 CrossRef CAS PubMed.
- S. Vogel, M. Barbic and J. Guido, Eur. J. Med. Chem., 2010, 45, 2206–2213 CrossRef CAS PubMed.
- V. Tomar and G. Bhattacharjee, Eur. J. Med. Chem., 2010, 45, 745–751 CrossRef CAS PubMed.
- J. C. Aponte, D. Castillo and Y. Estevez, Bioorg. Med. Chem. Lett., 2010, 20, 100–103 CrossRef CAS PubMed.
- B. T. Yin, C. Y. Yan and X. M. Peng, Eur. J. Med. Chem., 2014, 71, 148–159 CrossRef CAS PubMed.
- Z. Nowakowska, Eur. J. Med. Chem., 2007, 42, 125–137 CrossRef CAS PubMed.
- Y. Zuo, Y. Yu and S. Wang, Eur. J. Med. Chem., 2014, 50, 393–404 CrossRef PubMed.
- Y. J. Wang, D. G. Zhou and F. C. He, Chin. Chem. Lett., 2018, 29, 127–130 CrossRef CAS.
- S. Kuthyala, G. K. Nagaraja and S. Sheik, J. Mol. Struct., 2018, 1177, 381–390 CrossRef.
- K. Sharanabasappa, J. Megha and B. Prakash, J. Mol. Struct., 2018, 1173, 142–147 CrossRef.
- S. Gurjaspreet, A. Aanchal, K. Pooja and K. M. Indresh, Bioorg. Med. Chem., 2018, 27, 188–195 Search PubMed.
- J. E. Kang, J. K. Cho, M. J. Curtis-Long, H. W. Ryu and J. H. Kim, Molecules, 2013, 18, 140–153 CrossRef CAS PubMed.
- A. O. Oyedapo, V. O. Mankanju, C. O. Adewunmi, E. O. Iwalewa and T. K. Adenowo, Afr. J. Tradit., Complementary Altern. Med., 2004, 1, 55–62 CAS.
- J. C. Aponte, M. Verastegui, E. Malaga, M. Zimic, M. Quiliano, A. J. Vaisberg, R. H. Gilman and G. B. Hammond, J. Med. Chem., 2008, 51, 6230–6234 CrossRef CAS PubMed.
- V. S. Verma, Zentralbl. Bakteriol., Parasitenkd., Infektionskrankh. Hyg., Abt. 2, Naturwiss.: Allg., Landwirtsch. Tech. Mikrobiol., 1973, 128, 467–472 CAS.
- Z. H. Wan, D. Y. Hu and P. Li, Molecules, 2015, 20, 11861–11874 CrossRef CAS PubMed.
- X. H. Gan, D. Y. Hu and Y. Wang, J. Agric. Food Chem., 2017, 65, 4367–4377 CrossRef CAS PubMed.
- H. Irannejad, M. Amini, F. Khodagholi, N. Ansari, S. K. Tusi, M. Sharifzadeh and A. Shafiee, Bioorg. Med. Chem., 2010, 18, 4224–4230 CrossRef CAS PubMed.
- G. Wang, Z. Peng, J. Wang, X. Li and J. Li, Eur. J. Med. Chem., 2017, 125, 423–429 CrossRef CAS PubMed.
- E. Plebanek, F. Chevrier, V. Roy, T. Garenne, F. Lecaille, D. Warszycki, A. J. Bojarski, G. Lalmanach and L. A. Agrofoglio, Eur. J. Med. Chem., 2016, 121, 12–20 CrossRef CAS PubMed.
- P. Singla, V. Luxami and K. Paul, Eur. J. Med. Chem., 2016, 117, 59–69 CrossRef CAS PubMed.
- R. S. Tamboli, R. Giridhar and H. P. Gandhi, J. Enzyme Inhib. Med. Chem., 2015, 5, 1475–6366 Search PubMed.
- M. Khoshneviszadeh, O. Shahraki and M. Khoshneviszadeh, J. Enzyme Inhib. Med. Chem., 2016, 31, 1–10 Search PubMed.
- D. J. Fu, J. Song and Y. H. Hou, Eur. J. Med. Chem., 2017, 138, 1076–1088 CrossRef CAS PubMed.
- S. Konno, T. Kokubo and M. Amano, Yakugaku Zasshi, 1992, 112, 729–741 CrossRef CAS.
- A. Monge, J. Palop and C. Ramirez, Eur. J. Med. Chem., 1991, 26, 179–188 CrossRef CAS.
- M. O'Rourke and S. A. Lang-Jr, Bioorg. Med. Chem. Lett., 1977, 20, 6024–6029 Search PubMed.
- R. S. Tamboli, R. Giridhar and H. P. Gandhi, J. Enzyme Inhib. Med. Chem., 2015, 31, 704–713 Search PubMed.
- P. Li, L. Shi and M. N. Gao, Bioorg. Med. Chem. Lett., 2015, 25, 481–484 CrossRef CAS PubMed.
- Y. Jin, B. A. Song, D. Y. Hu, X. Y. Li, P. S. Bhadury, Z. C. Wang and S. Yang, Chem. Cent. J., 2011, 5, 21 CrossRef CAS PubMed.
- G. Wang and Z. Peng, Bioorg. Chem., 2018, 78, 195–200 CrossRef CAS PubMed.
- C. J. Wienken, P. Baaske and U. Rothbauer, Nat. Commun., 2011, 1, 100 CrossRef PubMed.
- B. Bhyravbhatla, S. J. Watowich and D. L. Caspar, Biophys. J., 1998, 74, 604–615 CrossRef CAS PubMed.
- Y. Y. Wang, F. Z. Xu and Y. Y. Zhan, Bioorg. Med. Chem. Lett., 2018, 28, 2979–2984 CrossRef CAS PubMed.
- A. C. Bloomer, J. N. Champness and G. Bricogne, Nature, 1978, 276, 362–368 CrossRef CAS PubMed.
- X. B. Wang, Z. J. Ren, M. Q. Wang and M. Chen, Chem. Cent. J., 2018, 12, 83 CrossRef PubMed.
Footnotes |
† Electronic supplementary information (ESI) available. See DOI: 10.1039/c9ra00618d |
‡ These authors contributed to this work equally. |
|
This journal is © The Royal Society of Chemistry 2019 |
Click here to see how this site uses Cookies. View our privacy policy here.