DOI:
10.1039/C9RA00113A
(Paper)
RSC Adv., 2019,
9, 6995-7003
Immunomodulatory mechanism of α-D-(1→6)-glucan isolated from banana
Received
6th January 2019
, Accepted 24th February 2019
First published on 1st March 2019
Abstract
Banana is a delicious fruit with potent immunomodulatory function. In this study, α-D-(1→6)-glucan was purified from banana pulp. It could significantly promote pinocytic activity and production of nitric oxide (NO), interleukin-6 (IL-6), and tumor necrosis factor-α (TNF-α). The mRNA expression of nitric oxide synthase (iNOS), IL-6 and TNF-α was increased in RAW264.7 macrophages. α-D-(1→6)-glucan could not only increase the expression levels of p-p65 and p-IκBα, but also induce the translocation of nuclear factor-kappa B (NF-κB) p65 into the nucleus. Moreover, mitogen-activated protein kinases (MAPKs), including p-ERK, p-JNK and p-p38, were upregulated. These results suggested that NF-κB and MAPK signaling pathways were involved in the immunomodulatory mechanisms of α-D-(1→6)-glucan. The results revealed that α-D-(1→6)-glucan might be the critical component responsible for the health benefits of banana.
Introduction
Consumption of fruits is beneficial for health maintenance and prevention of diseases, including cancer, diabetes, cardiovascular disorders, Alzheimer disease, and age-related functional decline.1,2 Banana is a fruit widely accepted by people over the world due to its pleasant flavor and great health benefits.3 A variety of bioactive compounds have been found in banana fruits. Among these compounds, polysaccharides are the predominant bioactive components.4 Starch, one of the most important polysaccharides in banana pulp, is a polymeric mixture of glucose polymers, amylose and amylopectin.5 Other polysaccharides, which included homogalacturonans, arabinogalactan, rhamnogalacturonan and mannan have been reported in banana pulp previously.6,7
Polysaccharides have attracted much attention recently due to their significant bioactivities,8,9 especially the immunomodulatory effects.10 Our previous studies found that polysaccharides from longan pulp and Citrus reticulata Blanco peel show great immunomodulatory activity.11,12 Moreover, polysaccharides from Gracilaria lemaneiformis possess immunomodulatory effect by inducing nitric oxide (NO) and cytokines secretion in macrophages.13 Macrophages play an important role in the immune system, and act as a part of the host defense system. Once the body is invaded by xenobiotics, macrophages are the first line of defense to recognize them and will be activated immediately.14 Furthermore, activated macrophages could act as the “bridge cell”, which is of great importance to the specific and non-specific immune reactions.15
Banana fruit is important component of a healthy diet with medicinal importance, including regulating immunity, lowering blood pressure and cholesterol, and improving intestinal health.16 Shiga et al. have pointed out that banana fruits may have a potential immunomodulatory activity due to the presence of arabinogalactan and mannans.6 However, no further investigations about the immunomodulatory activity of such polysaccharides from banana have been reported. The predominant neutral polysaccharide was isolated in banana pulp, and its precise structure was identified to be α-D-(1→6)-glucan in our previous work. Moreover, α-D-(1→6)-glucan has showed potent in vivo immunomodulatory activity.17 α-D-(1→6)-glucan was found to be the predominant polysaccharides in banana pulp, which may be responsible for the immunomodulatory effect of banana fruit. However, the mechanism of its immunomodulatory activity remains unknown. In the present work, the immunomodulatory activity of α-D-(1→6)-glucan was further examined using murine macrophage RAW264.7 cells, by determining the effects on the secretions of NO and cytokines, and related gene expression. The possible mechanism of its immunomodulatory effect was analyzed as well. The results could be evidence that polysaccharides from banana pulp acts as immunomodulatory agent by enhancing macrophage function.
Materials and methods
Materials
Fresh bananas at the ripe stage were collected from a local farm in Guangzhou, China. Ripe banana fruits were peeled, and were immediately subjected to polysaccharides extraction.
Chemicals
Lipopolysaccharide (LPS), neutral red, and 3-(4,5-dimethylthiazol-2-y1)-2,5-diphenyltetrazoliumbromide (MTT) were purchased from Sigma-Aldrich Chemical Co. (St. Louis, MO, USA). Dulbecco's modified Eagle's medium (DMEM), fetal bovine serum (FBS), phosphate-buffered saline (PBS), and other reagents for cell culture were purchased from Gibco Life Technologies (Grand Island, NY, USA). The primary monoclonal antibodies against NF-κB p65, phospho-IκBα, phospho-p65, phospho-ERK, phospho-SAPK/JNK, phospho-p38 MAPK and GAPDH, and horseradish peroxidase-conjugated secondary antibodies were purchased from Cell Signaling Technology, Inc., (Boston, MA, USA). Anti-TATA binding protein TBP antibody-Nuclear Loading Control was obtained from Abcam plc., (Cambridge, UK). The ECL western blot detection kit was from Bio-Rad Laboratories (Shanghai) Co., Ltd. (Shanghai, China). The specific inhibitors, including BAY11-7082, SB203580, SP600125 and PD98059 were purchased from Target Molecules Corp. (Boston, MA, USA). All the other chemicals used were of analytical grade.
Preparation of α-D-(1→6)-glucan from banana pulp
The water-soluble polysaccharides in banana pulp were prepared by hot water extraction, as described previously.7 The crude polysaccharides were treated with Sevag reagent (chloroform/butanol = 4
:
1, v/v) to remove the proteins. Then the polysaccharides were subjected to column chromatography, which was performed over DEAE Sepharose Fast Flow and Sephadex G-100, respectively. The neutral banana pulp polysaccharide (NBPP) was obtained after lyophilization. NBPP accounted for 83.2 ± 5.6% of crude polysaccharides, indicating that NBPP was the dominant fraction in banana polysaccharides. The precise structure of NBPP was identified to be α-D-(1→6)-glucan by monosaccharide composition assay and NMR assay, as described in our previous report.17 The molecular weight of NBPP was higher than 2990 kDa by high performance gel permeation chromatography.
Cell culture
Mus macrophage cell RAW264.7 (ATCC TIB-71) was purchased from the American Tissue Culture Collection (Manassas, VA, USA). The cells were cultured in a growth medium containing DMEM plus 10% FBS, and penicillin-streptomycin-neomycin (PSN) antibiotics mixture (Gibco Life Technologies, Grand Island, NY, USA). The cells were kept in a humidified incubator at 37 °C with an atmosphere containing 5% CO2.
Cytotoxicity assay
The cytotoxicity assay of α-D-(1→6)-glucan was performed by MTT staining method as previously described.18 Briefly, RAW264.7 cells (1.7 × 104 cells per well) were placed in a 96-well flat-bottom plate for 24 h. The growth medium was discarded from each well, and the cells were washed by PBS before the treatment of various concentrations of α-D-(1→6)-glucan (10–400 μg mL−1). After incubation for 24 h, 10 μL of MTT solution (5 mg mL−1) were added to each well. The cells were stained for 4 h, then media was carefully removed from each well. The formazan crystals were dissolved in DMSO before measuring the absorbance at 570 nm. The cells without α-D-(1→6)-glucan or LPS treatment was set as normal control, and LPS (200 ng mL−1) was used as positive control.
Pinocytic activity assay
The pinocytic activity of α-D-(1→6)-glucan was measured according to the method of Zhao et al.19 RAW264.7 cells were pretreated with a variety of concentrations (10, 20, 40, 80 and 120 μg mL−1) of α-D-(1→6)-glucan for 24 h as described before. Then culture media was discarded, and 0.075% neutral red (100 μL per well) was added. After incubation for 3 h, media were removed, and the cells were wash with PBS twice before adding 100 μL of cell lysate (ethanol
:
acetic acid = 1
:
1 v/v). The absorbance was measured at 520 nm, and the pinocytic activity was calculated as following:
where A1 and A0 were the absorbance of tested sample and the absorbance of the normal control, respectively.
Measurement of nitric oxide and cytokines secretion
After incubated for 24 h in a 96-well flat-bottom plate, RAW264.7 cells were treated with different concentrations (10, 20, 40, 80 and 120 μg mL−1) of α-D-(1→6)-glucan. Supernatants were collected following another 24 h incubation, nitric oxide (NO), tumor necrosis factor alpha (TNF-α) and interleukin-6 (IL-6) secretion were measured by using NO assay kit (Beyotime Biotechnology, Shanghai, China), TNF-α and IL-6 ELISA kits (Neobioscience Technology Co., Ltd. Shenzhen, China) according to the manufacturer's instructions, respectively. LPS was used as the positive control.
Gene expression analyses
RAW264.7 cells (6 × 105 cells per well) were seeded in a 6-well flat-bottom plate followed by incubation of 24 h. Then, the cells were incubated with α-D-(1→6)-glucan at different concentrations (10, 20, 40, 80 and 120 μg mL−1) for 24 h. Total RNA was isolated using the HiPure total RNA Mini kit (Magen Biological Technology Co., Ltd., Guangzhou, China). The first strand cDNA synthesis, and quantitative real-time (qRT-PCR) analysis were conducted as described by Li et al.20 The qPCR primers used were: inducible nitric oxide synthase (iNOS) (F: AAGCAGCTGGCCAATGAG, R: CCCCATAGGAAAAGACTGC), TNF-α (F: GGGAGCAAAGGTTCAGTGAT, R: CCTGGCCTCTCTACCTTGTT), IL-6 (F: CTGACAATATGAATGTTGGG, R: TCCAAGAAACCATCTGGCTAGG), interleukin-10 (IL-10) (F: GGTTGCCAAGCCTTATCGGA, R: ACCTGCTCCACTGCCTTGCT), and GAPDH (F: GTCATTGAGAGCAATGCCAG, R: GTGTTCCTACCCCCAATGTG). Data were analyzed by using the ΔΔCT method, and GADPH was applied as an endogenous control.
Western blotting analyses
RAW264.7 cells were seeded in 6-well flat-bottom plates at a concentration of 1 × 106 cells per well followed by incubation of 24 h. Then, the cells were incubated with α-D-(1→6)-glucan at different concentrations (20, 40 and 80 μg mL−1) for 30 or 90 min (for the expression of nuclear NF-κB p65). The cells receiving the same level of treatment were collected together for protein extraction and further western blot analysis was performed as previously described.2 The expressions of GAPDH and anti-TATA binding protein TBP antibody were analyzed as an internal standard for process control, and nuclear lysate loading control, respectively. The bands were finally quantified using Image J software (National Institutes of Health, USA), normalized with corresponding GAPDH or TBP antibody.
Statistical analyses
All data were expressed as the means of three replicated determinations. Results were subjected to analysis of variance and differences between means were located using Duncan or Dunnett's T3 test. p < 0.05 or p < 0.01 was regarded as significant value.
Results and discussion
The cytotoxicity activity of α-D-(1→6)-glucan
The potential cytotoxicity of α-D-(1→6)-glucan varying from 10–400 μg mL−1 on RAW264.7 cells was investigated by measuring cell viability. As shown in Fig. 1, α-D-(1→6)-glucan and LPS were nontoxic to the cells within the tested concentrations. On the contrary, α-D-(1→6)-glucan could significantly promote cell proliferation of RAW264.7 cells at a low concentration (10 μg mL−1). These results indicated that α-D-(1→6)-glucan from banana fruit pulp was a noncytotoxic polysaccharide.
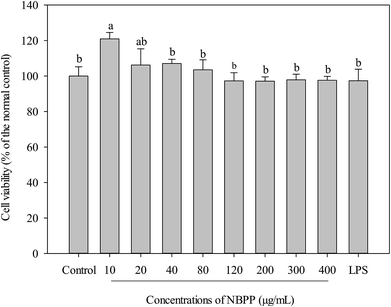 |
| Fig. 1 Effects of α-D-(1→6)-glucan and LPS on the cell viabilities of RAW264.7 macrophages. NBPP refers to α-D-(1→6)-glucan. Bars with no letters in common are significantly different (p < 0.05). | |
Effect of α-D-(1→6)-glucan on pinocytic activity
Activation of macrophages is one of the most important steps in innate immune response through initiating and propagating defensive reactions against xenobiotics.21 Therefore, phagocytosis functions play significantly important roles for macrophages to exert innate immunity. An increase in pinocytotic activity is one of the most significant feature of macrophage activation.19 As shown in Fig. 2, α-D-(1→6)-glucan significantly up-regulated the pinocytotic activity of RAW264.7 cells in a dose-dependent manner. Similarly, polysaccharide from Ficus carica and β-glucan from Durvillaea antarctica were reported to improve pinocytic capacity of RAW264.7 cells.22,23
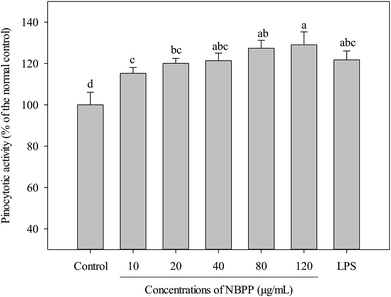 |
| Fig. 2 Effects of α-D-(1→6)-glucan and LPS on the pinocytotic activities of RAW264.7 macrophages. NBPP means α-D-(1→6)-glucan. Values with different letters show significant differences (p < 0.05). | |
Effect of α-D-(1→6)-glucan on NO and cytokines production
To further investigate whether α-D-(1→6)-glucan could activate macrophages, the productions of NO and cytokines (TNF-α and IL-6) in RAW264.7 macrophages were determined as well. Without α-D-(1→6)-glucan or LPS treatment, the concentrations of NO, TNF-α and IL-6 in RAW264.7 macrophages were 0.294 μmol L−1, 7.94 and 183.8 pg mL−1, respectively. Compared to the normal control, α-D-(1→6)-glucan could significantly alleviate the productions of NO, TNF-α and IL-6 in a dose-dependent manner (Fig. 3). The secretions of NO, TNF-α and IL-6 for α-D-(1→6)-glucan treatment at 120 μg mL−1 were significantly higher than those of LPS stimulation.
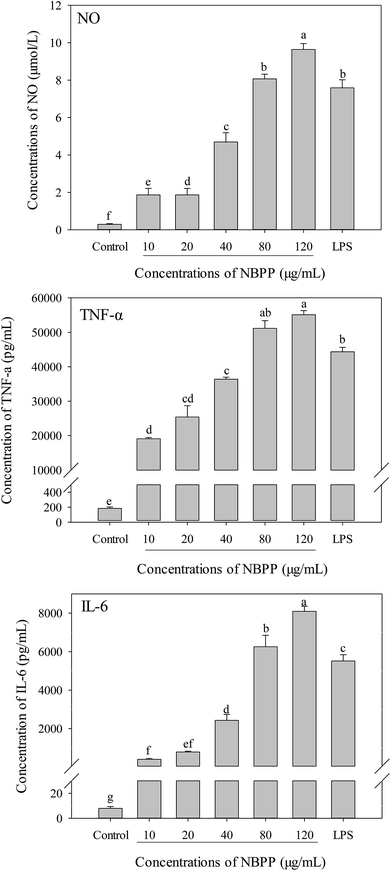 |
| Fig. 3 Effects of α-D-(1→6)-glucan and LPS on the productions of NO and cytokines in RAW264.7 macrophages. NBPP refers to α-D-(1→6)-glucan. Bars with different letters are significantly different (p < 0.05). | |
Previous report indicated that when macrophages were activated, they could promote phagocytosis, NO and cytokine production. It was consistent with our present results.13,14 Phagocytosis, which elevates the innate immune response after activation, is the first step of macrophages response to foreign bodies.24 NO is a free-radical gas that can diffuse freely through cells and is synthesized from L-arginine by NO synthases (NOS), which included neuronal NOS (nNOS), iNOS and endothelial NOS (eNOS).25,26 Bogdan has suggested that NO is one of the most important mediators in the immune system. It contributes to killing, and inhibiting microorganisms and tumor cells via activating macrophages.26 The concentration of NO in RAW264.7 cells was extremely low without α-D-(1→6)-glucan or LPS treatment, while a significant promotion in the NO synthesis was observed in the present study, implying the activation of macrophages induced by α-D-(1→6)-glucan. Additionally, NO was able to modulate the production and function of cytokines, including IL-1, IL-6, IL-8, IL-10, IFN-γ, and TNF.26 Cytokines are mainly produced by immune cells and play important roles in the activation, differentiation, and maturation of a variety of immune cells.27 Among reported cytokines, TNF-α is a product of activated macrophages, natural killer cells and T lymphocytes, involving in regulation of TNF-dependent adaptive immunity by activating monocytes and macrophages.13 IL-6, which is produced in monocytes and macrophages at sites of inflammation during acute inflammation and in T cells in chronic inflammation, is a pro-inflammatory and immunoregulatory cytokine as a results of host defense against infections and tissue injuries.28,29 Moreover, IL-6 is a key regulatory signal in the differentiation of naive T cells to Th17 cells, which can lead to autoimmunity when the level is increased.30 Compared to other pro-inflammatory cytokines, TNF-α and IL-6 have unique immunomodulatory actions in immune response.28 NO, TNF-α and IL-6 are common biomarkers to evaluate the immunomodulatory effect of polysaccharides.13,15,24 Our results indicated that α-D-(1→6)-glucan might generate immunostimulatory effects via activated macrophages, which plays an important role for the immunological activity exerted by polysaccharides.
Our results indicated that α-D-(1→6)-glucan prepared from banana showed potent immunological activity. Ferreira et al. have also reviewed α-D-(1→6)-glucan from Aconitum carmichaeli, Armillariella tabescens, Ipomoea batatas and Lepista sordida which showed immunomodulatory effect in vitro and/or in vivo.31 Therefore, α-D-(1→6)-glucan might be the key component responsible for the health benefits of banana. Furthermore, α-D-(1→6)-glucan from the cultured Armillariella tabescens mycelia could induced NO and cytokines production in macrophages from 50 to 200 μg mL−1.32 However, the immunomodulatory effect was weaker than that of α-D-(1→6)-glucan from banana. Previously, polysaccharides with relatively low molecular weight likely possess greater bioactivities.8 However, α-D-(1→6)-glucan prepared from banana showed significantly better immunological activity than other polysaccharides reported previously, such as GLP from Gracilaria lemaneiformis, and HSP from Hibiscus sabdariffa. All these polysaccharides possessed lower molecular weight than α-D-(1→6)-glucan in the present work, but significantly weaker immunomodulatory effect.13,33 Thereby, α-D-(1→6)-glucan isolated from banana could potentially be used as a potent immunomodulatory agent in functional foods.
Effects of α-D-(1→6)-glucan on mRNA expression of NO and cytokines
As shown in Fig. 4, the mRNA expression levels of iNOS, IL-6 and IL-l0 were significantly induced in a concentration-dependent manner after treating by α-D-(1→6)-glucan for 24 h. When the concentration of α-D-(1→6)-glucan was up to 120 μg mL−1, the expression levels of iNOS, IL-6 and IL-10 were higher than those of LPS. Furthermore, the TNF-α expression level of α-D-(1→6)-glucan-treated cells increased steadily within the concentrations of 20–120 μg mL−1. Significant promotion of TNF-α expression was observed within test concentration, comparing with the normal control group. Therefore, α-D-(1→6)-glucan regulated NO and cytokines secretion at the mRNA level. The endogenous control GAPDH was constitutively expressed and was unaffected by α-D-(1→6)-glucan treatment. Thus, α-D-(1→6)-glucan regulated the transcriptional stimulations of iNOS, TNF-α, IL-6 and IL-10.
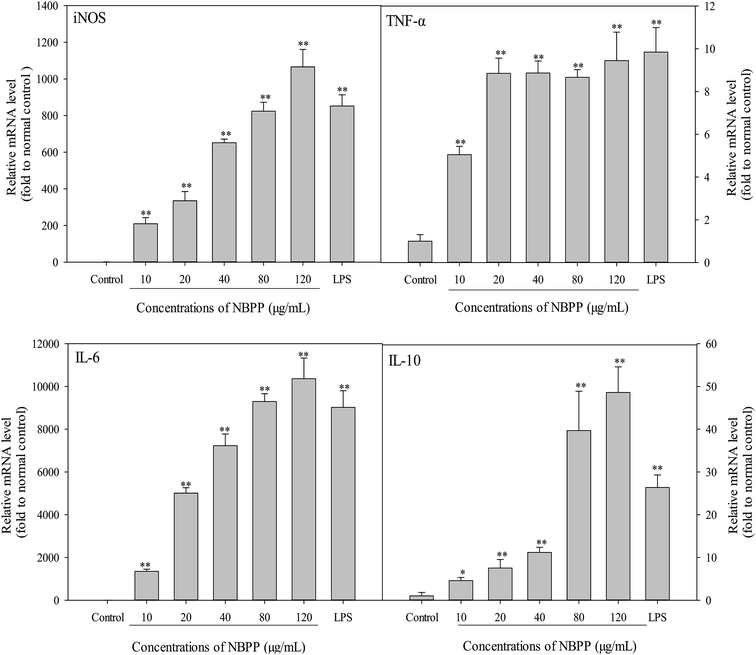 |
| Fig. 4 Effects of α-D-(1→6)-glucan and LPS on the mRNA expression of iNOS and cytokines in RAW264.7 macrophages. NBPP means α-D-(1→6)-glucan. “*” refers to a significant difference from the normal control at p < 0.05, “**” refers to a significant difference from the normal control at p < 0.01. | |
Among NOS, iNOS is responsible for most NO production in a wide array of cells.34 iNOS is helpful in preventing excessive immune reactions, protecting to some extent against autoimmunity and acting as an inter- and intra-cellular signaling molecule shaping the immune response.26 IL-10 is also an important immunoregulatory cytokine that can be produced by innate and adaptive immune cells. Generally, IL-10 is an anti-inflammatory cytokine, inhibiting the production of a number of pro-inflammatory cytokines such as TNF-α and IL-6.27 As mentioned before, pro-inflammatory mediators, including TNF-α and IL-6, are important for host survival from abnormal cells and tissues, and can be produced by the activation of immune cells. However, hypernomic production of pro-inflammatory cytokines by over-activating immune cells can lead to chronic inflammation. Therefore, anti-inflammatory cytokines like IL-10 also secrete to prevent potential harmful effects from immoderate macrophage activation.35 A balanced ratio of IL-6 to IL-10 decreases the risk of chronic inflammation.36 Previous study has indicated that IL-10 is induced to suppress the over-activation of RAW264.7 cells, thus avoiding potential occurrence of severe inflammation.35 Our results demonstrated that considerable expression of IL-10 was induced by α-D-(1→6)-glucan treatment. Moreover, a higher concentration of α-D-(1→6)-glucan triggered a drastic increase of expression levels (39.67–48.59 times to the normal control) compared with the lower concentration (4.57–11.17 times to the normal control). Thereby, the present results implied that α-D-(1→6)-glucan might be an immunostimulatory polysaccharide that activated macrophages via producing pro-inflammatory mediators while suppressing the excessive activation by releasing anti-inflammatory cytokines. Similar phenomenon has been observed in polysaccharides from Enteromorpha prolifera, suggesting that α-D-(1→6)-glucan is a good immunostimulators.35
Effects of α-D-(1→6)-glucan on nuclear factor-kappa B (NF-κB) activation and nuclear translocation
To investigate the α-D-(1→6)-glucan-mediate signal transduction pathways in the regulation of gene expression, western blotting assay was carried out to analyze the expression levels of NF-κB proteins, including phosphorylated p65 (p-p65), phosphorylated IκB-α (p-IκB-α), and NF-κB subunit p65. The results are displayed in Fig. 5. The western blotting assay demonstrated that the expression level of p-IκB-α in RAW264.7 cells stimulated with α-D-(1→6)-glucan significantly increased from 3.19 to 5.49 times comparing with the normal control, in a dose-dependent manner (Fig. 5B and D). Similarly, the level of p-p65 was significantly increased after α-D-(1→6)-glucan treatment (Fig. 5A). Moreover, α-D-(1→6)-glucan significantly elevated the nuclear levels of NF-κB subunit p65 (Fig. 5C). However, all the expression levels were lower than those of LPS treatment. To further confirm the role of NF-κB in α-D-(1→6)-glucan-induced NO production, BAY 11-7082, which could specifically inhibit NF-κB activation, was used. As shown in Fig. 5E, pretreating with BAY11-7082 significantly inhibited NO production stimulated by α-D-(1→6)-glucan and LPS, especially at 5 μM, where α-D-(1→6)-glucan-induced NO production was almost completely suppressed. All the results implied that the NO production was induced by α-D-(1→6)-glucan through a NF-κB-dependent mechanism.
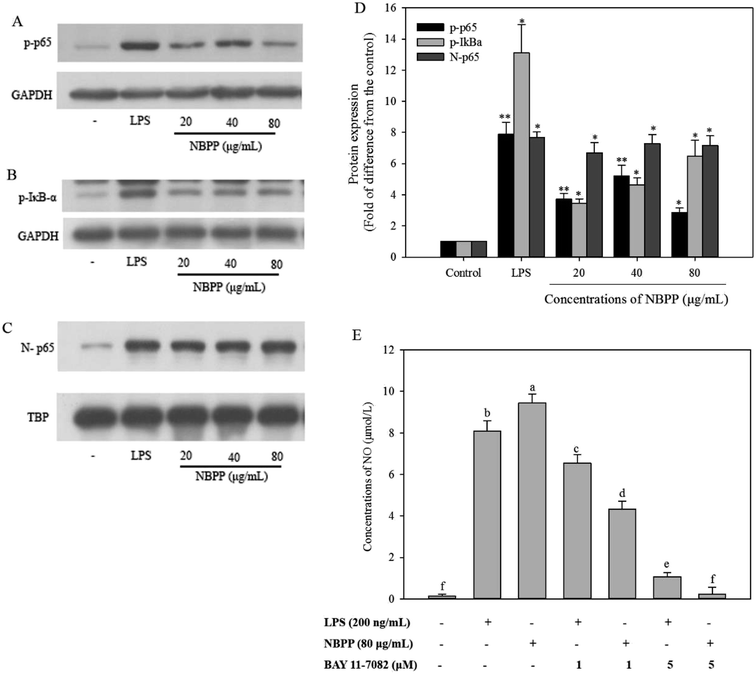 |
| Fig. 5 Effects of α-D-(1→6)-glucan and LPS on NF-κB activation and nuclear translocation in RAW264.7 macrophages. Western blotting assays show the expression of phospho-p65 (A), phospho-IκBα (B), and nuclear NF-κB p65 (N-p65) (C) after α-D-(1→6)-glucan or LPS treatment; the protein expression levels of phospho-p65, phospho-IκBα, and nuclear NF-κB p65 (N-p65) after α-D-(1→6)-glucan or LPS stimulation, comparing to the normal control (D), the asterisk “*” or “**” indicates a significant difference from the normal control at p < 0.05 or p < 0.01, respectively; effects of NF-κB activation on α-D-(1→6)-glucan and LPS induced NO production (E), RAW264.7 macrophages (1.7 × 105 cells per mL) were pretreated with BAY 11-7082 (1 or 5 μM) for 30 min, and then stimulated with α-D-(1→6)-glucan (80 μg mL−1) or LPS (200 ng mL−1) for 24 h. The level of NO production was determined by measuring concentration of NO in the culture medium, bars with no letters in common are significantly different (p < 0.05). NBPP refers to α-D-(1→6)-glucan. | |
The expression of iNOS in murine macrophages is predominantly regulated by transcription factor NF-κB. Additionally, NF-κB is also one of the most important regulators for the expression of the genes associated with immune and inflammatory responses, including macrophage-related cytokines and antioxidant enzymes.37,38 In the resting cells, NF-κB is sequestered in cytosol by interacting with IκB, which is the inhibitory protein of NF-κB. Once the cells are exposed to stimuli, the phosphorylation, ubiquitinated and subsequent degradation of IκB leads to NF-κB activation. Then free NF-κB rapidly translocates to the nucleus to induce transcription of target genes.14 In the present work, α-D-(1→6)-glucan induced IκBα and NF-κB subunit p65 phosphorylation, and nuclear translocation of NF-κB subunit p65. BAY11-7082, an IκBα kinase inhibitor, specifically inhibits the activation of NF-κB by preventing the phosphorylation of IκBα.39 α-D-(1→6)-glucan induced NO production was effectively inhibited by BAY11-7082. These findings suggested that α-D-(1→6)-glucan could induce NF-κB activation through increasing IκBα and p65 phosphorylation. Similar phenomenon has been reported in polysaccharides from Hibiscus sabdariffa Linn. and Cheonggukjang.24,33
Effects of α-D-(1→6)-glucan on mitogen-activated protein kinase (MAPK) activation
MAPKs, a family of serine/threonine-specific protein kinase, mainly consists of three members, including ERK1/2, p38MAPK, and JNK1/2. Reportedly, MAPKs play a predominant role in cellular response to extracellular stimuli, such as LPS and polysaccharides, leading to activation of macrophages to induce relevant genes transcription and cytokines secretion.23 The phosphorylation of MAPKs was reported to activate the NF-κB signaling pathway.40 In the present work, RAW264.7 cells stimulated with α-D-(1→6)-glucan concentration-dependently increased the expressions levels of phosphorylation of ERK1/2 (Fig. 6A and D) and JNK1/2 (Fig. 6C and E). The expression levels of phosphorylation of ERK1/2 and JNK1/2 in α-D-(1→6)-glucan treatment group (80 μg mL−1) were 2.37/2.57-fold, and 10.49/15.62-fold, respectively, as much as normal control, but lower than those of LPS stimulation. The expression levels of p-p38 were 1.53, 5.37, and 2.79-fold at varying concentrations of α-D-(1→6)-glucan treatment, comparing to the normal control (Fig. 6B and D). MAPK phosphorylation was found to be a precondition for NO and cytokine production in activated macrophages.41 MAPK inhibitors, such as SB 203580, SP600125 and PD98059, which are p38 MAPK, JNK, and ERK inhibitors, respectively, were used to verify the effects of MAPK activation on α-D-(1→6)-glucan-induced NO production. As shown in Fig. 6F, SB 203580, SP600125 and PD98059 significantly inhibited NO production stimulated by α-D-(1→6)-glucan to different extents.
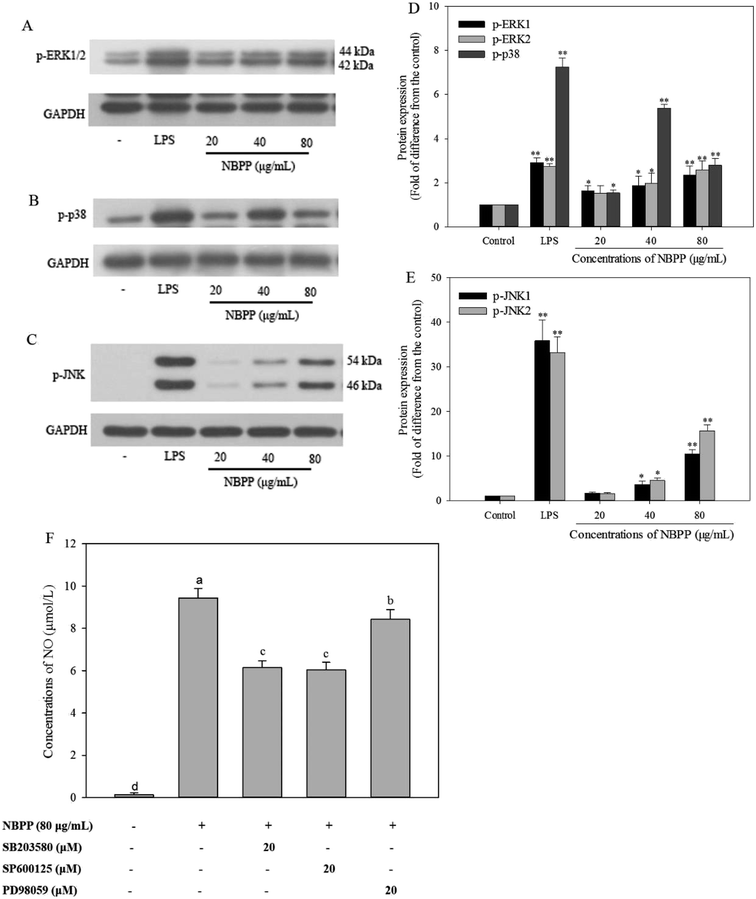 |
| Fig. 6 Effects of α-D-(1→6)-glucan and LPS on MAPK activation in RAW264.7 macrophages. Effects of α-D-(1→6)-glucan and LPS on phosphorylation of ERK1/2 (A), p38MAPK (B), and JNK (C) by western blotting analysis; the protein expression levels of phospho-ERK1/2 (D), phosphor-p38 (D), and phospho-JNK (E) induced by α-D-(1→6)-glucan or LPS, comparing to the normal control, “*” indicates a significant difference from the normal control at p < 0.05, “**” indicates a significant difference from the normal control at p < 0.01; effects of MAPK activation on α-D-(1→6)-glucan induced NO production (F), RAW264.7 macrophages (1.7 × 105 cells per mL) were pretreated with SB 203580 (20 μM), SP600125 (20 μM), PD98059 (20 μM) for 30 min, respectively, and then stimulated with α-D-(1→6)-glucan (80 μg mL−1) for 24 h. The level of NO production was determined by measuring concentration of NO in the culture medium. Values with different letters show significant differences (p < 0.05). NBPP refers to α-D-(1→6)-glucan. | |
MAPKs are essential regulators for both innate and adaptive immune responses. MAPK activity is regulated by reversible phosphorylation at threonine and tyrosine residues.42 Studies have revealed that polysaccharides from Hibiscus sabdariffa Linn., Ficus carica, and Durvillaea antarctica can promote expression of p-ERK1/2, p-p38, and p-JNK1/2 in MAPK signaling pathways of RAW264.7 macrophages.22,23,33 Consequently, phosphorylated p38, ERK1/2, and JNK1/2 were induced after α-D-(1→6)-glucan treatment. Previous report has also showed that MAPK inhibitors effectively inhibited polysaccharide-induced NO production in rIFN-γ-primed RAW264.7 macrophages, in accordance with present results.24 Taken together, α-D-(1→6)-glucan activated macrophages partly through the activation of p38, JNK, and ERK in the MAPK signaling pathway, and then promoted mediator production corresponding to immunity response.
Conclusions
In this study, the predominant polysaccharide, α-D-(1→6)-glucan was prepared from banana. It could significantly promote the pinocytic activity of RAW264.7 macrophages and increase the secretions of NO, TNF-α and IL-6. NF-κB and MAPK signaling pathways were involved in the macrophage activation induced by α-D-(1→6)-glucan. These results suggested that α-D-(1→6)-glucan could potentially be used as a potent immunomodulatory agent in functional foods and pharmaceuticals.
Conflicts of interest
The authors declare that they have no competing interests.
Acknowledgements
The authors are grateful to the financial support from National Natural Science Foundation of China (31671906 and 31871851), National Key Research and Development Program of China (2017YFD0401301), Frontier Science Key Program of Chinese Academy of Sciences (QYZDB-SSW-SMC018), National Natural Science Foundation of Guangdong Province (2018A030310387), and Postdoctoral Science Foundation (2017LH026 and 2017M622821).
References
- R. H. Liu, Adv. Nutr., 2013, 4, 384S–392S CrossRef CAS PubMed.
- L. Wen, R. Guo, L. You, A. M. Abbasi, T. Li, X. Fu and R. H. Liu, Food Chem. Toxicol., 2017, 100, 149–160 CrossRef CAS PubMed.
- N. S. Mathew and P. S. Negi, J. Ethnopharmacol., 2017, 196, 124–140 CrossRef CAS PubMed.
- J. Sun, L. Li, X. You, C. Li, E. Zhang, Z. Li, G. Chen and H. Peng, Anal. Methods, 2011, 3, 2212–2220 RSC.
- P. Zhang and B. R. Hamaker, Carbohydr. Polym., 2012, 87, 1552–1558 CrossRef CAS.
- T. M. Shiga, N. C. Carpita, F. M. Lajolo and B. R. Cordenunsi-Lysenko, Carbohydr. Polym., 2017, 164, 31–41 CrossRef CAS PubMed.
- H. Liu, Y. Jiang, H. Yang and B. Yang, Int. J. Biol. Macromol., 2017, 101, 299–303 CrossRef CAS PubMed.
- L. Wen, Y. Zhang, D. Sun-Waterhouse, L. You and X. Fu, RSC Adv., 2017, 7, 9141–9151 RSC.
- L. Wen, Q. Gao, C.-w. Ma, Y. Ge, L. You, R. H. Liu, X. Fu and D. Liu, J. Funct. Foods, 2016, 20, 400–410 CrossRef CAS.
- J. E. Ramberg, E. D. Nelson and R. A. Sinnott, Nutr. J., 2010, 9, 1–22 CrossRef PubMed.
- Q. Q. Zhu, Y. M. Jiang, S. Lin, L. R. Wen, D. Wu, M. M. Zhao, F. Chen, Y. X. Jia and B. Yang, Biomacromolecules, 2013, 14, 1999–2003 CrossRef CAS PubMed.
- J. L. Yang, L. R. Wen, Y. P. Zhao, Y. M. Jiang, M. M. Tian, H. L. Liu, J. Liu and B. Yang, Food Hydrocolloids, 2018, 84, 481–488 CrossRef CAS.
- Y. Ren, G. Zheng, L. You, L. Wen, C. Li, X. Fu and L. Zhou, J. Funct. Foods, 2017, 33, 286–296 CrossRef CAS.
- E. H. Han, J. H. Choi, Y. P. Hwang, H. J. Park, C. Y. Choi, Y. C. Chung, J. K. Seo and H. G. Jeong, Food Chem. Toxicol., 2009, 47, 62–69 CrossRef CAS PubMed.
- C. Y. Shen, L. Yang, J. G. Jiang, C. Y. Zheng and W. Zhu, Food Funct., 2017, 8, 796–807 RSC.
- K. P. S. Kumar, D. Bhowmik, S. Duraivel and M. Umadevi, J. Pharmacogn. Phytochem., 2012, 1, 57–70 Search PubMed.
- J. Yang, J. Tu, H. Liu, L. Wen, Y. Jiang and B. Yang, Food Chem., 2019, 277, 46–53 CrossRef CAS PubMed.
- L. Wen, D. Wu, Y. Jiang, K. N. Prasad, S. Lin, G. Jiang, J. He, M. Zhao, W. Luo and B. Yang, J. Funct. Foods, 2014, 6, 555–563 CrossRef CAS.
- L. Y. Zhao, Y. H. Dong, G. T. Chen and Q. H. Hu, Carbohydr. Polym., 2010, 80, 783–789 CrossRef CAS.
- T. Li, L. Gong, G. Jiang, Y. Wang, V. K. Gupta, H. Qu, X. Duan, J. Wang and Y. Jiang, Proteomics, 2017, 17, 1700070 CrossRef PubMed.
- K. P. Mishra, Y. S. Padwad, M. Jain, D. Karan, L. Ganju and R. C. Sawhney, Immunopharmacol. Immunotoxicol., 2006, 28, 201–212 CrossRef CAS PubMed.
- J. Du, J. J. Li, J. H. Zhu, C. H. Huang, S. X. Bi, L. Y. Song, X. J. Hu and R. M. Yu, Food Funct., 2018, 9, 3930–3943 RSC.
- Y. Yang, X. L. Zhao, J. Li, H. Jiang, X. D. Shan, Y. Wang, W. B. Ma, J. J. Hao and G. L. Yu, Carbohydr. Polym., 2018, 191, 255–265 CrossRef CAS PubMed.
- H.-S. Shin, J.-M. Lee, S.-Y. Park, J.-E. Yang, J.-H. Kim and T.-H. Yi, Phytother. Res., 2013, 27, 1352–1357 CrossRef PubMed.
- G. T. Lee, J. H. Hong, C. Kwak, J. Woo, V. Liu, C. Lee and I. Y. Kim, Cancer Res., 2007, 67, 6717–6724 CrossRef CAS PubMed.
- C. Bogdan, Nat. Immunol., 2001, 2, 907 CrossRef CAS PubMed.
- D. L. Su, Z. M. Lu, M. N. Shen, X. Li and L. Y. Sun, J. Biomed. Biotechnol., 2012, 2012, 347141 Search PubMed.
- W. E. Naugler and M. Karin, Trends Mol. Med., 2008, 14, 109–119 CrossRef CAS PubMed.
- T. Kishimoto and T. Tanaka, Interleukin 6, in Encyclopedia of Inflammatory Diseases, ed. M. Parnham, Springer Basel, Basel, 2015, pp. 1–8 Search PubMed.
- E. Bettelli, M. Oukka and V. K. Kuchroo, Nat. Immunol., 2007, 8, 345–350 CrossRef CAS PubMed.
- S. S. Ferreira, C. P. Passos, P. Madureira, M. Vilanova and M. A. Coimbra, Carbohydr. Polym., 2015, 132, 378–396 CrossRef CAS PubMed.
- X. Luo, X. Xu, M. Yu, Z. Yang and L. Zheng, Food Chem., 2008, 111, 357–363 CrossRef CAS PubMed.
- C.-Y. Shen, W.-L. Zhang and J.-G. Jiang, J. Funct. Foods, 2017, 34, 118–129 CrossRef CAS.
- M. Lechner, P. Lirk and J. Rieder, Semin. Cancer Biol., 2005, 15, 277–289 CrossRef CAS PubMed.
- J.-K. Kim, M. L. Cho, S. Karnjanapratum, I.-S. Shin and S. G. You, Int. J. Biol. Macromol., 2011, 49, 1051–1058 CrossRef CAS PubMed.
- P. Suabjakyong, K. Nishimura, T. Toida and L. J. Van Griensven, Food Funct., 2015, 6, 2834–2844 RSC.
- N. Listed, J. Immunol., 2001, 166, 3873–3881 CrossRef.
- J. L. Yang, L. R. Wen, Y. M. Jiang and B. Yang, Trends Endocrinol. Metab., 2019, 30, 66–76 CrossRef CAS PubMed.
- J. W. Pierce, R. Schoenleber, G. Jesmok, J. Best, S. A. Moore, T. Collins and M. E. Gerritsen, J. Biol. Chem., 1997, 272, 21096–21103 CrossRef CAS PubMed.
- C. M. Olson, M. N. Hedrick, H. Izadi, T. C. Bates, E. R. Olivera and J. Anguita, Infect. Immun., 2007, 75, 270–277 CrossRef CAS PubMed.
- J. L. Dean, M. Brook, A. R. Clark and J. Saklatvala, J. Biol. Chem., 1999, 274, 264–269 CrossRef CAS PubMed.
- Y. S. Liu, E. G. Shepherd and L. D. Nelin, Nat. Rev. Immunol., 2007, 7, 202–212 CrossRef CAS PubMed.
|
This journal is © The Royal Society of Chemistry 2019 |
Click here to see how this site uses Cookies. View our privacy policy here.