DOI:
10.1039/C8RA09785B
(Paper)
RSC Adv., 2019,
9, 1976-1981
Solid-state AIEnh-circularly polarised luminescence of chiral perylene diimide fluorophores†
Received
28th November 2018
, Accepted 23rd December 2018
First published on 14th January 2019
Abstract
Solid-state organic fluorescent materials are important for the development of electroluminescent sensing devices. Herein, we report that N,N′-bis((R)-1-phenylethyl)perylene-3,4,9,10-tetracarboxylic diimide [(R,R)-BPP] and its antipode [(S,S)-BPP], which contain extended π-electrons through planar perylenes, emit solid-state aggregation-induced-enhanced (AIEnh) circularly polarised luminescence (CPL) in inorganic (KBr) pellets and organic-polymer-film (PMMA- and myo-IPU-film) states; this CPL is difficult to observe in solution. These chiral perylene fluorophores emit AIEnh-CPL with high dissymmetry factors (gCPL) (up to 2.4 × 10−3) and high quantum yields (ΦF, up to 0.43) in the three solid matrices.
Introduction
Because of their potential applications to electroluminescence (EL) and optical-sensing devices, solid-state organic fluorescent materials have attracted much attention.1 In particular, optically active organic and inorganic luminophores that emit circularly polarised luminescence (CPL) with high dissymmetry factors (gCPL) and high quantum yields (ΦF) have received significant attention in the field of circularly polarised organic light-emitting diodes (CP-OLEDs).2 Recently, aggregation-induced-enhanced (AIEnh) CPL has also attracted much interest. However, AIEnh-CPL is almost always emitted from highly concentrated solutions.3 Therefore, systems that emit solid-state AIEnh-CPL are highly desired. With the aim of providing a common design approach to novel chiral solid-state AIEnh organic fluorophores, in this study we prepared solid-state AIEnh circularly polarised luminophores by doping chiral N,N′-bis((R)-1-phenylethyl)perylene-3,4,9,10-tetracarboxylic diimide [(R,R)-BPP] and its antipode [(S,S)-BPP] into various types of solid matrix. The two BPPs are chiral bi-substituted perylene fluorophores composed of extended π-electron planar perylenes. We then examined the AIEnh-CPL emissions from these imbedded BPP systems as AIEnh-fluorophore models.4 KBr was used as the solid inorganic matrix, while poly(methyl methacrylate) (PMMA) and polyurethane (myo-IPU) were chosen as solid organic polymer matrices.5 Myo-IPU is derived from naturally occurring myo-inositol (a meso-hexose) and characteristically has a higher glass transition temperature (Tg ∼150 °C) than conventional PMMA (Tg ∼105 °C).
Herein, we report that (R,R)- and (S,S)-BPP emit solid-state AIEnh-CPL with high quantum efficiencies when imbedded in solid inorganic and organic polymer matrices (Fig. 1).
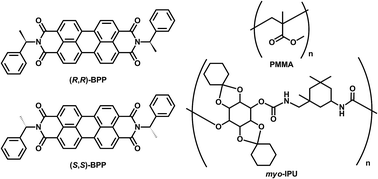 |
| Fig. 1 The chiral perylene fluorophores ((R,R)-BPP and (S,S)-BPP) and organic polymer matrices (PMMA and myo-IPU) used in this study. | |
Experimental
General
The KBr and PMMA matrices for solid pellets and films were purchased from JASCO (Tokyo, Japan) and the FUJIFILM Wako Pure Chemical Corp. (Osaka, Japan), respectively. Polyurethane (myo-IPU) was prepared by a previously reported method.5 Chloroform (CHCl3) used for the determination of solution-state optical properties was purchased from the FUJIFILM Wako Pure Chemical Corp. (Osaka, Japan).
Syntheses of N,N′-bis((R)-1-phenylethyl)perylene-3,4,9,10-tetracarboxylic diimide [(R,R)-BPP] and (S,S)-BPP
(R,R)-BPP and (S,S)-BPP were prepared with reference to a previously reported method.4 A mixture of 3,4,9,10-perylenetetracarboxylic dianhydride (430.0 mg, 1.1 mmol), (R)-1-phenylethylamine (0.3 mL, 2.4 mmol), and zinc acetate dihydrate (240.1 mg, 1.1 mmol) in quinolone (20 mL) was heated with stirring at 180 °C under nitrogen for 4 h. After cooling to room temperature, the reaction mixture was treated with 2 N HCl and stirred at room temperature overnight. The resulting dark-red precipitate was collected by filtration, washed thoroughly with water and methanol (MeOH), and dried in vacuo. The crude product was purified by silica-gel column chromatography using CHCl3/EtOAc (20
:
1, v/v) as the eluent, to give 523.2 mg (79% yield) of (R,R)-BPP as a deep-red solid. The S-enantiomer, namely (S,S)-BPP, was synthesised from (S)-1-phenylethylamine by the same procedure to provide a deep-red solid (76% yield): 1H NMR for (R,R)-BPP (CDCl3 500 MHz) δ 2.06 (d, J = 7.5 Hz, 3H), 6.60 (q, J = 7.5 Hz, 2H), 7.29 (t, J = 7.5 Hz, 2H), 7.38 (t, J = 7.5 Hz, 4H), 7.57 (d, J = 7.5 Hz, 4H), 8.58 (d, J = 8.0 Hz, 4H), 8.66 (d, J = 8.0 Hz, 4H); 13C NMR (CDCl3 125 MHz) δ 16.3, 50.5, 122.9, 123.5, 126.1, 127.2, 127.5, 128.2, 129.2, 131.3, 134.3, 140.5, 163.3.
Unpolarised photoluminescence (PL) quantum yields
Samples were dispersed in KBr pellets in the following way. Microcrystalline powdered samples (10 μg) were finely ground with 120 mg of KBr, and the mixed powder was compressed at 80 MPa cm−2 under vacuum for 25 min to provide a 10 mm-diameter, 0.7 mm-thick transparent disk. Samples dispersed in PMMA or myo-IPU films were prepared using PMMA or myo-IPU (1.0 × 10−2 M) in a spin coater at 3000 rpm (Opticoat MS-A100, Mikasa, Tokyo, Japan).
Absolute unpolarised photoluminescence (PL) quantum yields (ΦF) of the KBr-pellet-dispersed states, the PMMA- or myo-IPU-film-dispersed states, and CHCl3 solutions were determined using a Hamamatsu Photonics C9920-02 absolute quantum yield spectrometer (Hamamatsu, Japan) in air at room temperature, at excitation wavelengths of 480 nm (KBr) and 450 nm (polymer film), respectively, and at 450 nm for the CHCl3 solution. A 10 mm path length was used for solution-phase spectroscopy.
Solid-state PL and CPL spectroscopy
Solid-state PL and CPL spectra of the KBr-pelleted samples were acquired by comprehensive chiroptical spectrophotometry (CCS, JASCO, Tokyo, Japan).6 CPL spectra were recorded with a slit width of 33 mm and a 10 nm spectral bandwidth for the excitation and emission monochromators. The PL and CPL spectra of the PMMA or myo-IPU films and the CHCl3 solutions were acquired using a JASCO CPL-300 spectrofluoropolarimeter (Tokyo, Japan) at room temperature, at a scattering angle of 0° upon excitation with unpolarised, monochromated incident light with a 10 nm bandwidth. An excitation wavelength of 480 nm was used for the KBr-pelleted samples, while 450 nm was used for the PMMA or myo-IPU films, and for the CHCl3 solutions. A 10 mm path length was used for solution-phase spectroscopy.
Circular dichroism (CD) and UV-Vis absorption spectroscopy
Circular dichroism (CD) and UV-Vis absorption spectra of the KBr-pelleted samples were acquired by CCS at room temperature.6 The CD and UV-Vis absorption spectra of PMMA- or myo-IPU-film-dispersed states and CHCl3 solutions were recorded with a JASCO J-820 spectropolarimeter at room temperature. The KBr-pelleted and PMMA (or myo-IPU) films were prepared as described for the samples used for solid-state PL and CPL spectroscopy. A 1 mm path length was used for solution-phase spectroscopy.
Results and discussion
Prior to elucidating the solid-state chiroptical properties of (R,R)-BPP and (S,S)-BPP, we first investigated their PL, CPL, and CD spectral characteristics in CHCl3 solution, at room temperature. To determine whether or not the obtained results are due to artefacts introduced by (R,R)-BPP and other factors, the CPL spectrum of (S,S)-BPP was also acquired. The CPL and PL spectra of (R,R)-BPP and (S,S)-BPP are shown in Fig. 2 ((R,R)-BPP in blue; (S,S)-BPP in red). When dissolved in CHCl3 (1.0 × 10−4 M), (R,R)-BPP exhibited PL at 549, 576, 623 nm with an absolute photoluminescence quantum yield (ΦF) of 0.76. Unfortunately, meaningful CPL spectra of chiral BPP could not be acquired under these conditions.
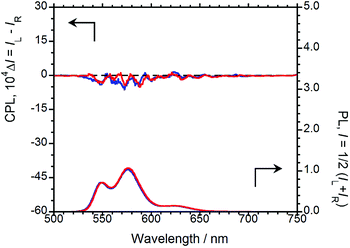 |
| Fig. 2 CPL (upper) and PL (lower) spectra of (R,R)-BPP (blue) and (S,S)-BPP (red) in CHCl3 (1.0 × 10−4 M). | |
The CD and UV-Vis absorption spectra of (R,R)-BPP and (S,S)-BPP in CHCl3 are shown in Fig. 3; as expected, the CD spectra are almost mirror images.
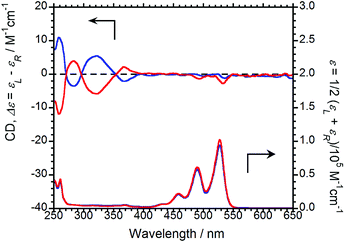 |
| Fig. 3 CD (upper) and UV-Vis absorption (lower) spectra of (R,R)-BPP (blue) and (S,S)-BPP (red) in CHCl3 (1.0 × 10−4 M). | |
Absorption bands at 459, 490, and 527 nm that originate from π–π* transitions in the perylene units are commonly observed, irrespective of the chirality of the sample. As same as CPL properties in the solution-dissolved state, these corresponding CD bands could not be mainly observed.
For the chiral BPPs to emit CPL, we turned to solid-state AIEnh-CPL spectroscopy. The solid-state PL and CPL spectra of (R,R)-BPP were acquired in the inorganic KBr matrix, the results of which are shown in Fig. 4. In contrast to the results in CHCl3 solution, solid-state CPL was observed from the KBr-pelleted sample; (R,R)-BPP in the KBr pellet exhibited a λCPL of 657 nm with a corresponding ΦF of 0.09. When the spectrum of the KBr-pellet-dispersed state is compared with that of the CHCl3 solution, quite different λPL values are observed (576 nm for CHCl3 and 632 nm for KBr). The λPL of the KBr pellet suggests that CPL from this state is AIEnh CPL derived from the aggregation of (R,R)-BPP in the solid state. Unfortunately, the value of ΦF in the KBr-dispersed state was smaller than that in CHCl3 solution, which is ascribable to fluorescence quenching under high-density conditions in the solid compared to the low-concentration conditions in solution. In addition, the external heavy-atom (Br) effect may also be partially responsible.
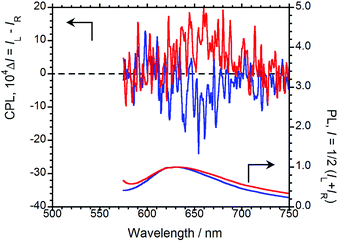 |
| Fig. 4 CPL (upper) and PL (lower) spectra of (R,R)-BPP (blue) and (S,S)-BPP (red) in KBr-pellet-dispersed states. | |
A negative (−) CPL spectrum was observed for (R,R)-BPP in the solid state. To determine whether or not these results are due to artefacts introduced by (R,R)-BPP and the KBr matrix, the CPL spectrum of (S,S)-BPP was acquired (Fig. 4). (R,R)-BPP and (S,S)-BPP in KBr matrices exhibit almost mirror-image CPL spectra. The magnitude of the circular polarisation in the excited state is defined as gCPL = ΔI/I = (IL − IR)/(IL + IR), where IL and IR are the output signals for left and right circularly polarised light under unpolarised photoexcitation conditions. The CPL efficiency, |gCPL|, of BPP in the KBr pellet is of the order of 10−3, while the |gCPL| of BPP is ∼2.0 × 10−3 in the KBr-dispersed state.
To examine molecular chirality in the ground state, the CD and UV-Vis absorption spectra of (R,R)-BPP and (S,S)-BPP in their KBr-pellet-dispersed states were acquired, the results of which are shown in Fig. 5.
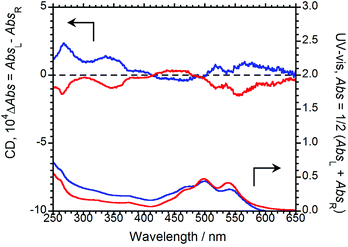 |
| Fig. 5 CD (upper) and UV-Vis (lower) absorption spectra of (R,R)-BPP (blue) and (S,S)-BPP (red) in KBr-pellet-dispersed states. | |
Several UV-Vis bands between 400 and 650 nm, which are characteristic of π–π* transitions in the perylene groups of BPP, can be observed. In this state, the Cotton CD bands of (R,R)-BPP and (S,S)-BPP, are almost mirror images and are due to π–π* transitions, as evidenced by their UV-Vis spectra. The CD spectra of (R,R)-BPP and (S,S)-BPP are almost unaffected by KBr-pellet dispersion. Interestingly, the CD spectra of the KBr-dispersed states are quite different to those of the CHCl3 solutions are similar to those of the previously reported nanoscale aggregates in the solution-dispersed state.4g We conclude that the CD spectra of the KBr-dispersed states also result from intermolecular interactions between the perylene units of multiple aggregated BPP molecules within the KBr pellets. To quantitatively evaluate the CD amplitude in the ground state, we evaluated the anisotropy factor. The magnitude of the circular polarisation in the ground state is defined as gCD = (AbsL − AbsR)/[(AbsL + AbsR)/2], where AbsL and AbsR are the absorbances of the left and right circularly polarised light, respectively. The value of |gCD| at the first Cotton CD band of BPP in KBr was determined to be ∼5.4 × 10−4 at 561 nm. The observed CD results in KBr support the CPL observations mentioned above. Interestingly, a comparison of these anisotropy factors, i.e., the |gCPL| and |gCD| values, reveals that the |gCPL| is larger than the corresponding |gCD| value, indicating that, although the perylene units are stacked and twisted in the ground state,4f the twists increase in the photoexcited state, as confirmed by the intense excimer CPL bands.
In order to emit stronger CPL, we next examined CPL from organic polymer-film states. As an organic polymer matrix, poly(methyl methacrylate) (PMMA) and polyurethane (myo-IPU) derived from naturally occurring myo-inositol were used. The CPL and PL spectra of (R,R)-BPP and (S,S)-BPP dispersed in PMMA and myo-IPU films are shown in Fig. 6(a) and (b), respectively.
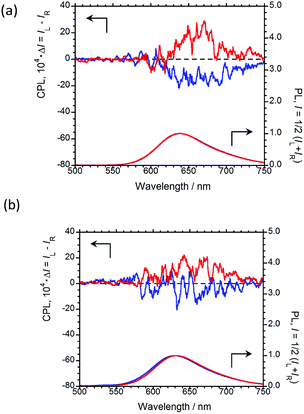 |
| Fig. 6 CPL (upper) and PL (lower) spectra of (R,R)-BPP (blue) and (S,S)-BPP (red) in (a) PMMA-film- and (b) myo-IPU-film-dispersed states. | |
(R,R)-BPP in PMMA emitted at 654 nm (λCPL) with a ΦF of 0.40, while in myo-IPU, it emitted at 637 nm (λCPL) with a ΦF of 0.43. As expected, (R,R)-BPP exhibits solid-state AIEnh-CPL in both polymers, with several regressional phonon sidebands originating from the lowest π–π* transitions between perylene groups observed. The CPL spectra of (R,R)-BPP in PMMA and myo-IPU films mostly exhibited negative (−) CPL bands (Fig. 6(a) and (b), respectively). As expected, (S,S)-BPP exhibited positive (+) CPL spectra in these polymers (Fig. 6(a) and (b)). Although the CPL spectral shapes of (R,R)-BPP and (S,S)-BPP in the KBr-pellet and PMMA- and myo-IPU-film states are nearly mirror images, subtle differences in the shapes of the CPL signals are noted. These differences may be due to the effect of humidity in the pellets and films during the preparation of the pellet and film samples. The |gCPL| values of BPP in the PMMA and myo-IPU states are ∼2.4 × 10−3 and ∼1.3 × 10−3, respectively, which are similar to that observed in the KBr state (∼2.0 × 10−3).
As expected, despite the CPL wavelengths of (R,R)-BPP in the KBr and polymer states being similar, the values of ΦF in these polymers are significantly higher than that observed for the KBr state (0.09). We assume that these approximately five-times-higher values are due to the suppression of thermal deactivation modes and effective packing arrangements because the molecular geometry of the chiral BPP is effectively and suitably fixed by the surrounding solid polymer matrix without quenching.
The CD and UV-Vis absorption spectra of (R,R)-BPP and (S,S)-BPP in the PMMA and myo-IPU films are shown in Fig. 7(a) and (b), respectively; the CD spectra in these polymers are similar in their long-wavelength tails and are similar to those of the KBr-dispersed states. These results suggest that the CD spectra of the polymer-dispersed states are essentially due to intermolecular interactions between multiple molecules rather than intramolecular interactions within individual BPP molecules. The characteristic UV peaks originating from π–π* transitions of the perylene groups are commonly observed between 400 and 600 nm. The CD spectra of (R,R)-BPP and (S,S)-BPP in both matrices are almost mirror images, as expected. The signs of the first Cotton CD bands of (R,R)-BPP, at 553 nm in PMMA and at 551 nm in myo-IPU, are both positive (+), while the CD signs of the corresponding bands for (S,S)-BPP in PMMS and myo-IPU are both negative (−). The |gCD| value at the first Cotton CD bands are ~3.1 × 10−4 and ~2.9 × 10−4 for the PMMA- and myo-IPU-dispersed states, respectively. BPP in these matrices exhibit |gCD|values of similar magnitude.
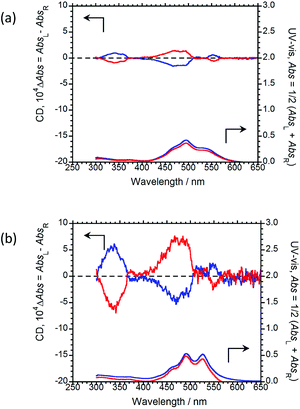 |
| Fig. 7 CD (upper) and UV-Vis absorption (lower) spectra of (R,R)-BPP (blue) and (S,S)-BPP (red) in (a) PMMA-film- and (b) myo-IPU-film-dispersed states. | |
As was observed in the KBr matrix, the |gCPL| values are higher than the corresponding |gCD| values in the polymer films. This shows that intermolecular twists between perylene units in the ground state increase upon photo-excitation, as evidenced by the intense excimer CPL bands in spectra of the polymer-matrix imbedded samples.
Conclusions
Chiral (R,R)- and (S,S)-N,N′-bis(1-phenylethyl)perylene-3,4,9,10-tetracarboxylic diimide (BPP) dispersed in solid KBr and PMMA (or myo-IPU) matrices exhibited solid-state AIEnh-CPL with high ΦF values at room temperature. The value of ΦF from the PMMA (ΦF 0.40) and myo-IPU matrices (ΦF 0.43) are significantly higher than that observed from the KBr matrix (ΦF 0.09). The present knowledge provides guidance for the design of novel solid chiral organic fluorophoric systems that emit solid-state AIEnh-CPL.
Conflicts of interest
There are no conflicts to declare.
Acknowledgements
This study was supported by a Grant-in-Aid for Scientific Research (no. 18K05094) from MEXT/Japan Society for the Promotion of Science, the MEXT-Supported Program for the Strategic Research Foundation at Private Universities (2014–2018), and a research grant from the International Polyurethane Technology Foundation.
Notes and references
-
(a) S. R. Forrest, Chem. Rev., 1997, 97, 1793–1896 CrossRef CAS;
(b) M. Glasbeek and H. Zhang, Chem. Rev., 2004, 104, 1929–1954 CrossRef CAS PubMed;
(c) A. Mishra, R. K. Behera, P. K. Behera, B. K. Mishra and G. B. Behera, Chem. Rev., 2000, 100, 1973–2011 CrossRef CAS PubMed;
(d) T. Baumgartner and R. Reau, Chem. Rev., 2006, 106, 4681–4727 CrossRef CAS PubMed;
(e) P. G. Coble, Chem. Rev., 2007, 107, 402–418 CrossRef CAS PubMed;
(f) T. P. I. Saragi, T. Spehr, A. Siebert, T. Fuhrmann-Lieker and J. Salbeck, Chem. Rev., 2007, 107, 1011–1065 CrossRef CAS PubMed;
(g) S. C. Lo and P. L. Burn, Chem. Rev., 2007, 107, 1097–1116 CrossRef CAS PubMed;
(h) K. Walzer, B. Maennig, M. Pfeiffer and K. Leo, Chem. Rev., 2007, 107, 1233–1271 CrossRef CAS PubMed;
(i) J. Grate, Chem. Rev., 2008, 108, 726–745 CrossRef CAS PubMed;
(j) F. Jakle, Chem. Rev., 2010, 110, 3985–4022 CrossRef CAS PubMed;
(k) V. K. Rai, R. Srivastava and M. N. Kamalasanan, J. Lumin., 2010, 130, 249–253 CrossRef CAS;
(l) J. Huang, B. Xu, M. K. Lam, K. W. Cheah, H. C. Chen and J. H. Su, Dyes Pigm., 2011, 89, 155–161 CrossRef CAS;
(m) V. M. Agranovich, Y. N. Gartstein and M. Litinskaya, Chem. Rev., 2011, 111, 5179–5214 CrossRef CAS PubMed;
(n) J. E. Kwon and S. Y. Park, Adv. Mater., 2011, 23, 3615–3642 CrossRef CAS PubMed;
(o) T. H. Tran-Thi, R. Dagnelie, S. Crunaireza and L. Nicole, Chem. Soc. Rev., 2011, 40, 621–639 RSC : and references cited therein.
-
(a) K. E. S. Phillips, T. J. Katz, S. Jockusch, A. J. Lovinger and N. J. Turro, J. Am. Chem. Soc., 2001, 123, 11899–11907 CrossRef CAS PubMed;
(b) J. E. Field, G. Müller, J. P. Riehl and D. Venkataraman, J. Am. Chem. Soc., 2003, 125, 11808–11809 CrossRef CAS PubMed;
(c) H. Maeda, Y. Bando, K. Shimomura, I. Yamada, M. Naito, K. Nobusawa, H. Tsumatori and T. Kawai, J. Am. Chem. Soc., 2011, 133, 9266–9269 CrossRef CAS PubMed;
(d) R. Tempelaar, A. Stradomska, J. Knoester and F. C. Spano, J. Phys. Chem. B, 2011, 115, 10592–10603 CrossRef CAS PubMed;
(e) Y. Nakano and M. Fujiki, Macromolecules, 2011, 44, 7511–7519 CrossRef CAS;
(f) N. Nishiguchi, T. Kinuta, Y. Nakano, T. Harada, N. Tajima, T. Sato, M. Fujiki, R. Kuroda, Y. Matsubara and Y. Imai, Chem.–Asian J., 2011, 6, 1092–1098 CrossRef CAS PubMed;
(g) J. Liu, H. Su, L. Meng, Y. Zhao, C. Deng, J. C. Y. Ng, P. Lu, M. Faisal, J. W. Y. Lam, X. Huang, H. Wu, K. S. Wong and B. Z. Tang, Chem. Sci., 2012, 3, 2737–2747 RSC;
(h) Y. Sawada, S. Furumi, A. Takai, M. Takeuchi, K. Noguchi and K. Tanaka, J. Am. Chem. Soc., 2012, 134, 4080–4083 CrossRef CAS PubMed;
(i) H. Oyama, K. Nakano, T. Harada, R. Kuroda, M. Naito, K. Nobusawa and K. Nozaki, Org. Lett., 2013, 15, 2104–2107 CrossRef CAS;
(j) T. Shiraki, Y. Tsuchiya, T. Noguchi, S.-I. Tamaru, N. Suzuki, M. Taguchi, M. Fujiki and S. Shinkai, Chem.–Asian J., 2014, 9, 218–222 CrossRef CAS PubMed;
(k) X. Jiang, X. Liu, Y. Jiang, Y. Quan, Y. Cheng and C. Zhu, Macromol. Chem. Phys., 2014, 215, 358–364 CrossRef CAS;
(l) Y. Morisaki, M. Gon, T. Sasamori, N. Tokitoh and Y. Chujo, J. Am. Chem. Soc., 2014, 136, 3350–3353 CrossRef CAS PubMed;
(m) S. Abbate, G. Longhi, F. Lebon, E. Castiglioni, S. Superchi, L. Pisani, F. Fontana, F. Torricelli, T. Caronna, C. Villani, R. Sabia, M. Tommasini, A. Lucotti, D. Mendola, A. Mele and D. A. Lightner, J. Phys. Chem. C, 2014, 118, 1682–1695 CrossRef CAS;
(n) K. Watanabe, Y. Koyama, N. Suzuki, M. Fujiki and T. Nakano, Polym. Chem., 2014, 5, 712–717 RSC;
(o) E. M. Sánchez-Carnerero, A. R. Agarrabeitia, F. Moreno, B. L. Maroto, G. Muller, M. J. Ortiz and S. de la Moya, Chem.–Eur. J., 2015, 21, 13488–13500 CrossRef;
(p) Y. Uchida, T. Hirose, T. Nakashima, T. Kawai and K. Matsuda, Org. Lett., 2016, 18, 2118–2121 CrossRef CAS PubMed;
(q) M. Shimada, Y. Yamanoi, T. Ohto, S. T. Pham, R. Yamada, H. Tada, K. Omoto, S. Tashiro, M. Shinoya, M. Hatton, K. Jimura, S. Hayashi, H. Koike, M. Iwamura, K. Nozaki and H. Nishihara, J. Am. Chem. Soc., 2017, 139, 11214–11221 CrossRef CAS PubMed;
(r) H. Tanaka, Y. Inoue and T. Mori, ChemphotoChem, 2018, 2, 1–18 CrossRef;
(s) K. Takaishi, R. Takehana and T. Ema, Chem. Commun., 2018, 54, 1449–1452 RSC , and references cited therein.
-
(a) H.-Z. Tang, M. Fujiki and M. Motonaga, Polymer, 2002, 43, 6213–6220 CrossRef CAS;
(b) S. Haraguchi, M. Numata, C. Li, Y. Kawagoe, M. Fujiki and Y. Nakano, New J. Chem., 2010, 34, 637–647 RSC;
(c) K. Watanabe, T. Sakamoto, M. Taguchi, M. Fujiki and T. Nakano, Chem. Commun., 2011, 47, 10996–10998 RSC;
(d) K. Okano, M. Taguchi, M. Fujiki and T. Yamashita, Angew. Chem., Int. Ed., 2011, 50, 12474–12477 CrossRef CAS PubMed;
(e) Y. Nakano, F. Ichiyanagi, M. Naito, Y. G. Yang and M. Fujiki, Chem. Commun., 2012, 48, 6636–6639 RSC;
(f) M. Fujiki, A. J. Jalilah, N. Suzuki, M. Taguchi, W. Zhang, M. M. Abdellatif and K. Nomura, RSC Adv., 2012, 2, 6663–6671 RSC;
(g) M. Fujiki, Y. Kawagoe, Y. Nakano and A. Nakao, Molecules, 2013, 18, 7035–7057 CrossRef CAS PubMed;
(h) H. Maeda and Y. Bando, Pure Appl. Chem., 2013, 85, 1967–1978 CAS;
(i) T. Shiraki, Y. Tsuchiya, T. Noguchi, S.-i. Tamaru, N. Suzuki, M. Taguchi, M. Fujiki and S. Shinkai, Chem.–Asian J., 2014, 9, 218–222 CrossRef CAS PubMed;
(j) K. Watanabe, Y. Koyama, N. Suzuki, M. Fujiki and T. Nakano, Polym. Chem., 2014, 5, 712–717 RSC;
(k) M. Fujiki, Y. Donguri, Y. Zhao, A. Nakao, N. Suzuki, K. Yoshida and W. Zhang, Polym. Chem., 2015, 6, 1627–1638 RSC;
(l) M. Fujiki and S. Yoshimoto, Mater. Chem. Front., 2017, 1, 1773–1785 RSC;
(m) C. Zhang, L. Meng, H.-Y. Lu and C.-F. Chen, RSC Adv., 2018, 8, 1014–1021 RSC.
-
(a) E. K. Todd, S. Wang, X. Wan and Z. Y. Wang, Tetrahedron Lett., 2005, 46, 587–590 CrossRef CAS;
(b) B. M. Rumyantsev, V. I. Berendyaev, A. Y. Tsegelskaya, T. S. Zhuravleva and I. V. Klimenko, Synth. Met., 2005, 152, 85–88 CrossRef CAS;
(c) S. Amiralaei, D. Uzun and H. Icil, Photochem. Photobiol. Sci., 2008, 7, 936–947 RSC;
(d) A. Pucci, F. Donati, S. Nazzi, G. U. Barretta, G. Pescitelli, L. D. Bari and G. Ruggeri, React. Funct. Polym., 2010, 70, 951–960 CrossRef CAS;
(e) B. M. Rumyantsev, V. I. Berendyaev and D. V. Pebalk, J. Phys. Chem. B, 2014, 8, 205–211 CAS;
(f) X. Shang, I. Song, Hi. Ohtsu, Y. H. Lee, T. Zhao, T. Kojima, J. H. Jung, M. Kawano and J. H. Oh, Adv. Mater., 2017, 29, 1605828 CrossRef PubMed;
(g) X. Shang, I. Song, H. Ohtsu, J. Tong, H. Zhang and J. H. Oh, Sci. Rep., 2017, 7, 5508 CrossRef PubMed.
- T. Amako, K. Nakabayashi, A. Sudo, M. Fujiki and Y. Imai, Org. Biomol. Chem., 2015, 13, 2913–2917 RSC.
- T. Harada, H. Hayakawa, M. Watanabe and M. Takamoto, Rev. Sci. Instrum., 2016, 87, 075102 CrossRef.
Footnote |
† Electronic supplementary information (ESI) available. See DOI: 10.1039/c8ra09785b |
|
This journal is © The Royal Society of Chemistry 2019 |
Click here to see how this site uses Cookies. View our privacy policy here.