DOI:
10.1039/C9PY00793H
(Perspective)
Polym. Chem., 2019,
10, 4389-4401
Photoresponsive polymers with multi-azobenzene groups
Received
30th May 2019
, Accepted 30th June 2019
First published on 2nd July 2019
Abstract
Azobenzene is a switchable compound that exhibits reversible trans–cis photoisomerization. Photoresponsive polymers have been prepared by incorporating azobenzene groups into polymers. Most of the reported azobenzene-containing polymers (azopolymers) have only one azobenzene group in the repeat unit of the polymers (mono-azopolymers). To improve photoresponsiveness, multi-azobenzene groups have been covalently or non-covalently introduced into the repeat units of azopolymers (multi-azopolymers). In this Perspective, we introduce photoresponsive multi-azopolymers. We summarize the preparation of such polymers and highlight their potential applications in photoactuation, photo-patterning, and photoinduced birefringence. The innovative potential, open questions, and remaining challenges in this field are also discussed.
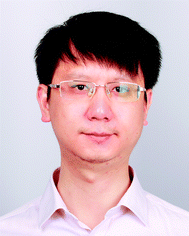 Si Wu | Si Wu is a full professor at the University of Science and Technology of China (USTC), Hefei, China. He studied polymer chemistry at the USTC and obtained a bachelor's degree in 2005. He was supported by the joint doctoral promotion programme working at the USTC and the Max Planck Institute for Polymer Research (MPIP), Mainz, Germany. After obtaining his PhD in 2010, he worked as a postdoctoral fellow at the MPIP and became a group leader in 2012. He headed a research group at the MPIP from 2012 to 2018. In 2018, he joined the USTC as a full professor. His research focuses on photoresponsive polymers. |
Introduction
Azobenzene is a photoswitchable compound.1 Light irradiation can induce reversible trans-to-cis isomerization.2,3 This property enables the use of light to control a variety of properties of azobenzene-containing materials, such as their polarity,4,5 solubility,5,6 melting points,7–9 glass transition temperature (Tg) values,10–12 viscosity,13–15 wrinkles,16–18 morphology,19–23 mechanical properties,24,25etc. In the past, the photophysical and photochemical properties of azobenzene derivatives have been extensively studied. Substituents strongly influence the spectral characteristics and photoswitching behaviors of azobenzene derivatives. Rau divided azobenzenes26,27 into three types (Fig. 1): (1) azobenzene-type compounds bearing alkyl, aryl, halide, carbonyl, amide, nitrile, ester, and carboxylic acid substituents. The π–π* and n–π* absorption bands of trans azobenzene are located in the UV range and visible range, respectively. UV irradiation induces trans-to-cis isomerization (Fig. 2a). The half-life of cis isomers is usually from hours to days. Visible light irradiation or thermal relaxation induces cis-to-trans isomerization (Fig. 2a); (2) aminoazobenzene-type compounds having at least one amino or hydroxyl group located ortho or para to the azobenzene group. The π–π* absorption band of the trans isomer is bathochromically shifted to 400–450 nm, partially overlapping with the n–π* absorption band. The half-life of cis isomers becomes shorter, usually from milliseconds to seconds; (3) pseudo-stilbene-type compounds containing a push–pull substitution para to the azobenzene group. Their π–π* absorption band and n–π* absorption band are both located in the visible range, completely overlapping with each other. These types of azobenzene compounds have a fast rate for photoisomerization. The half-lives of cis isomers are usually from milliseconds to seconds.
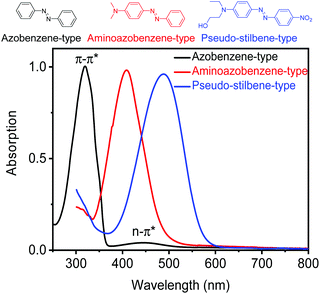 |
| Fig. 1 Typical chemical structures and UV/Vis absorption spectra of three types of azobenzenes. | |
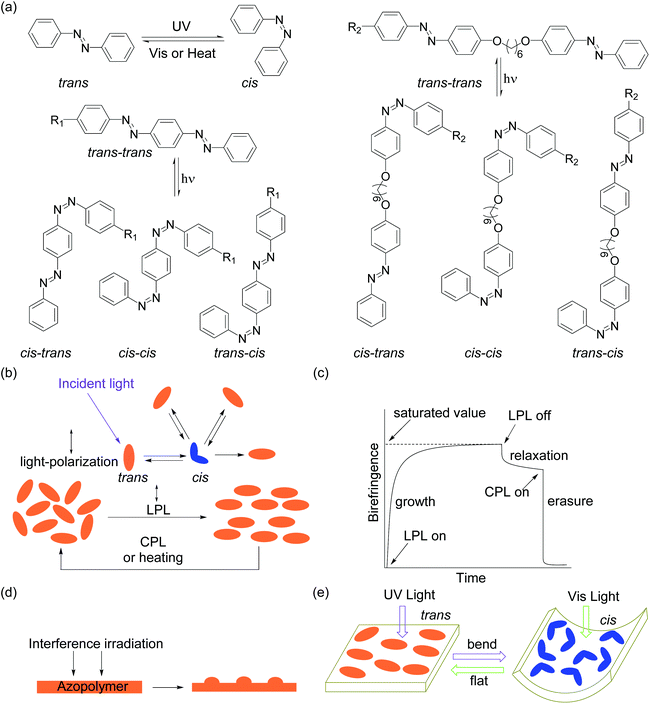 |
| Fig. 2 (a) Photoisomerization of azobenzene and bis-azobenzene derivatives. (b) Schematic illustrations of the photoinduced orientations of azobenzene chromophores in the solid state. (c) Birefringence is induced under LPL irradiation, reaches a saturated value after irradiation for a certain time, relaxes via thermal relaxation when LPL is turned off, and is erased using CPL. (d) Formation of photoinduced SRGs. (e) Schematic illustrations of photoactuation. | |
All these types of azobenzene compounds have been incorporated into polymers that have better processability than small molecules. Polymers provide azobenzene group suited matrices for photoisomerization in the solid state. In addition, besides photoisomerization,28,29 azobenzene-containing polymers (azopolymers) show other interesting photoresponsive properties such as photoinduced orientation (Fig. 2b and c),30–43 formation of surface relief gratings (SRGs) (Fig. 2d),44–48 photoactuation (Fig. 2e),49–54 and photo-controlled self-assembly behaviors.55–66 When an azopolymer film is irradiated with linearly polarized light (LPL),67,68 the azobenzene groups tend to orient perpendicular to the light polarization. Subsequent circularly polarized light (CPL) irradiation or heating can erase the orientation (Fig. 2b).30–35 Thus, birefringence can be generated and erased by light irradiation (Fig. 2c). When an azopolymer film is irradiated with interference laser beams, SRGs are induced (Fig. 2d).69–73 Another photoresponsive behavior of azopolymers is photoactuation. Light irradiation can induce macroscopic motions such as bending and contraction of azopolymers (Fig. 2e).74,75 Azopolymers can also undergo self-assembly via supramolecular interactions and light can control the physicochemical properties of supramolecular azopolymers.76–86 There are comprehensive reviews about the development of azopolymers and the investigations of photoresponsive properties mentioned above.67,87–89
Different from previous reviews, in this Perspective, we focus on azopolymers that have multi-azobenzene groups in the repeat unit. We call these types of polymers multi-azopolymers. Conventional azopolymers have only one azobenzene group in each repeat unit; such mono-azopolymers have been widely studied. The photoisomerization of multi-azobenzene groups results in more types of isomers (Fig. 2a). Multi-azobenzene groups may have a larger aspect ratio than mono-azobenzene groups, exhibiting larger birefringence when oriented. Multi-azobenzene groups also exhibit interesting (liquid) crystallinities. With more azobenzene groups in a repeat unit of polymers, multi-azopolymers show some unique photoresponsive properties.90–92 Here, we present the current status of multi-azopolymers, their potential applications, and the challenges, with the aim of highlighting the most relevant literature in this field.
Types of multi-azopolymers
We summarize a few types of multi-azopolymers in Fig. 3. There is still room for designing new multi-azopolymers based on polymer chemistry, supramolecular chemistry, and organic chemistry. From the polymer chemistry point of view, multi-azobenzene groups can be introduced into polymer side chains, backbones, or both. Because of the diversity of polymer structures, new multi-azopolymers can be designed. From the supramolecular chemistry point of view, multi-azopolymers can be prepared by non-covalently introducing multi-azobenzene groups into polymers. From the organic chemistry point of view, small-molecule multi-azobenzene groups can have different structures (see for example bis-azobenzenes with flexible and conjugated linkers in Fig. 2a). Multi-azobenzene groups can be introduced into a repeat unit of polymers either in parallel or in series. In the following, we introduce different types of multi-azopolymers according to their structures.
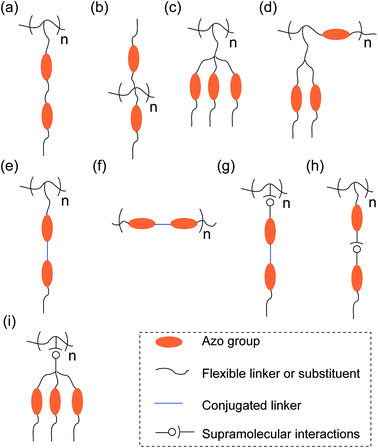 |
| Fig. 3 Schematic illustrations of multi-azopolymers with different structures: (a–d) non-conjugated multi-azopolymers, (e and f) conjugated multi-azopolymers, and (g–i) supramolecular multi-azopolymers. | |
Polymers with non-conjugated multi-azobenzene groups
Non-conjugated azobenzene groups mean that two or more azobenzene groups are connected via non-conjugated flexible linkers. Non-conjugated multi-azobenzene groups can be introduced into polymers in series. For example, Aida and co-workers studied a polymer with three azobenzene groups in series in the side chain of each repeat unit (Fig. 4).93 They synthesized a methacrylate monomer with three azobenzene groups and prepared polymer 1 through free radical polymerization (Fig. 4a, m = 3). Because of the long side chains, polymer 1 is like a brush. The polymer chains of 1 were oriented homeotropically to the substrates via one-step hot-pressing (Fig. 4b). The hot-pressed film exhibited a rapid and reversible photomechanical response under the irradiation of UV and visible light (Fig. 4c and d).74,94 In contrast, the control sample, polymer 2 (Fig. 4a, m = 1), which had only one azobenzene group in each repeat unit, did not show the alignment behavior by hot pressing. These results indicate that the multi-azopolymer forms ordered structures more easily via hot pressing than the mono-azopolymer does. On the basis of these interesting findings, the authors demonstrated a design principle for polymers that form 2D homeotropic orders.95
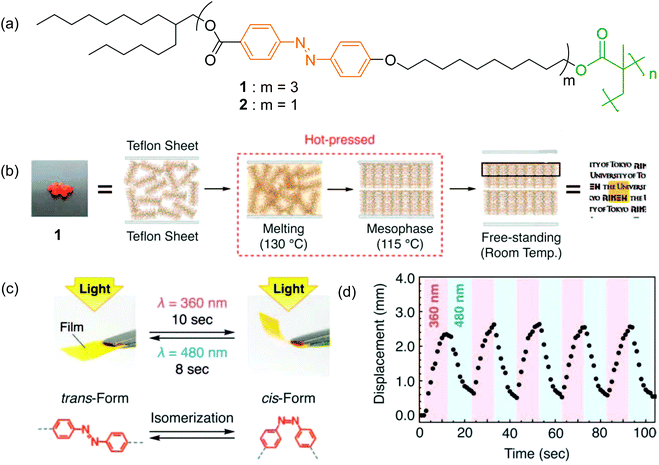 |
| Fig. 4 (a) Chemical architectures of polymers 1 and 2. (b) Schematic illustrations of the preparation of free-standing films via hot-pressing. (c) Photographs of a hot-pressed film (5 mm × 6 mm × 10 μm) prepared with parallel-arranged Teflon sheets before (left) and after (right) exposure to UV light (λ = 360 ± 2 nm). The bent film recovered the initial flat shape upon exposure to visible light (λ = 480 ± 2 nm). (d) Time-dependent change in the displacement of the free edge of a hot-pressed film prepared with parallel-arranged Teflon sheets upon alternating irradiation with UV and visible lights. Reproduced with permission from ref. 93, copyright 2010, AAAS. | |
Akiyama et al. prepared oligo-dendrimer type molecules, multiazobenzene sugar–alcohol derivatives,96–98 in which the multi azobenzene groups were connected in parallel with each other. These kinds of molecules showed photochemically reversible solid–liquid transitions and could be applied to reworkable adhesives.
In 2013, Zhu and Wang reported polymers with two and three azobenzene groups in parallel in the polymer side chains.99 These polymers showed reversible photoisomerization. Furthermore, birefringence was induced by LPL irradiation. Multi-azobenzene moieties in each repeat unit of the polymer resulted in larger birefringence.
Kim et al. also prepared polymers with azobenzene groups in parallel in the polymer side chains (Fig. 5).100 Different from Zhu and Wang's strategy, polymer 3 was synthesized via ring opening metathesis polymerization (ROMP). Free-standing films were prepared. The backbone and azobenzene side chains of polymer 3 in the film were perpendicular and parallel to the layer normal, respectively. When the film was exposed to UV light, it quickly bent away from the light source due to trans-to-cis isomerization (Fig. 5b–d). After subsequent visible light irradiation, the film recovered to its original state because of cis-to-trans back isomerization. Photoinduced bending and unbending were fully reversible (Fig. 5e). Further investigations showed that such types of multi-azopolymers exhibit photoisomerization101 and photoinduced chirality,102 and can be used as photoresponsive semiconductors with good photo-fatigue resistance.103
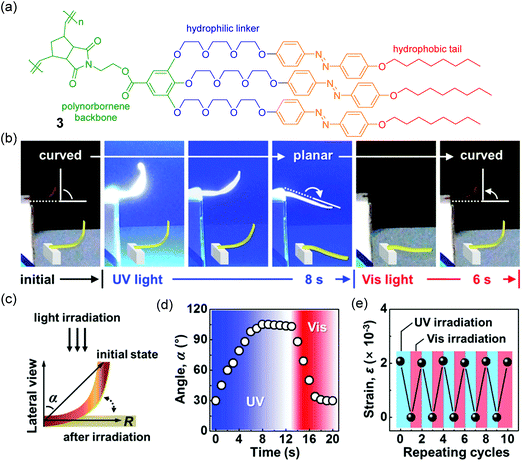 |
| Fig. 5 (a) Chemical structure of polymer 3. (b) Photo-modulation of a freestanding film of 3 upon UV and visible light irradiation. (c) Schematic illustration of the photomechanical motion. (d) Reversible flexion angle changes with respect to UV and visible irradiation. (e) Repeated experiments of the photo-stimulated bending strain. Reproduced from ref. 100. Copyright (2017) Wiley-VCH Verlag GmbH & Co. KGaA, Weinheim. | |
Polymers with conjugated multi-azobenzene groups
Some azo groups were connected via conjugated linkers. Conjugated multi-azobenzene groups have been introduced into polymer side chains (Fig. 6a). Polymer 4 was prepared by Meng and Natansohn through free radical polymerization.90 Compared with 5 that has mono-azobenzene groups (Fig. 6b),1044 showed larger photoinduced birefringence because of the intrinsic higher aspect ratio of the bis-azobenzene group (Fig. 6c and d). In addition, 4 showed a slower rate of photoinduced birefringence and a higher birefringence stability than 5, which is because of the lower mobility of the bis-azobenzene group.
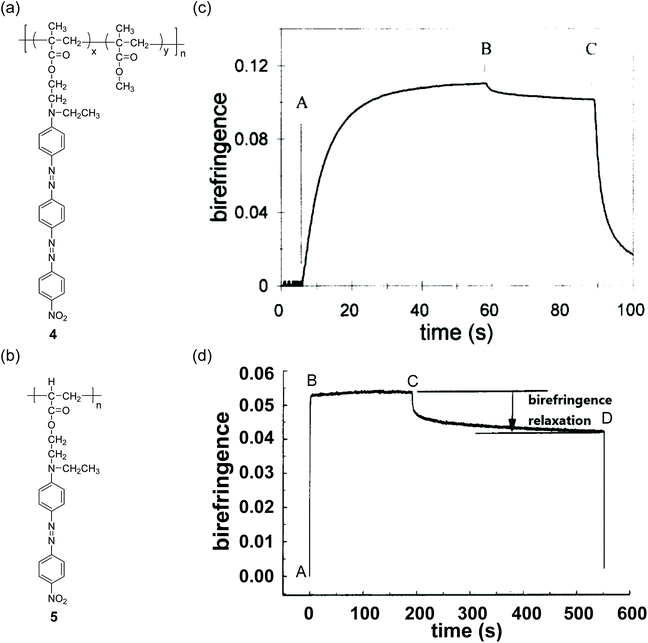 |
| Fig. 6 Chemical structures of (a) 4 and (b) 5. Writing–erasing profile of the photoinduced birefringence of films of (c) 4 and (d) 5. Panel (c) is reprinted from ref. 90. Copyright (1997) with permission from Elsevier. Panel (d) is reprinted with permission from ref. 104. Copyright (1995) American Chemical Society. | |
In addition, polymethacrylates with similar structures have been synthesized and their photoresponsive properties, such as photoisomerization,91,105–112 photo-alignment,106,107,110 photoinduced birefringence,91,105,106,108–110,113 information storage,112 and the induction of helical chirality,111,114,115 have been investigated. The rate of photoinduced birefringence for the bis-azopolymers was slower than that for mono-azopolymers,90,91 while the birefringence values for bis-azopolymers were higher and the birefringence stability for bis-azopolymers was better than those for mono-azopolymers.90,91,108,109 However, there was an exception, where both the rate and the level of photoinduced birefringence for the bis-azopolymers were better than those for mono-azopolymers.105 In addition to multi-azopolymers with a poly(meth)acrylate backbone, multi-azopolymers with other types of backbones have also been studied, such as polymethacrylamides showing photoinduced birefringence116 and polyurethane anionomers showing photoisomerization.117
Besides photoinduced birefringence, multi-azopolymers are also suited for photoinduced SRGs. In an early example, Wang et al. reported the inscription of SRGs on polymers with conjugated bis-azobenzene groups on polymer side chains.118 Recently, Zhang et al. synthesized poly(aryl ether)s 6, 7, 8, and 9 (Fig. 7) via polycondensation.119 Polymers 6, 7, 8, and 9 had high glass transition temperature (Tg) values and good thermal stability.119 These polymers also showed photoisomerization (Fig. 7b) and photoinduced SRGs under the irradiation of interference beams (Fig. 7c and d), which have potential applications in optical data storage.
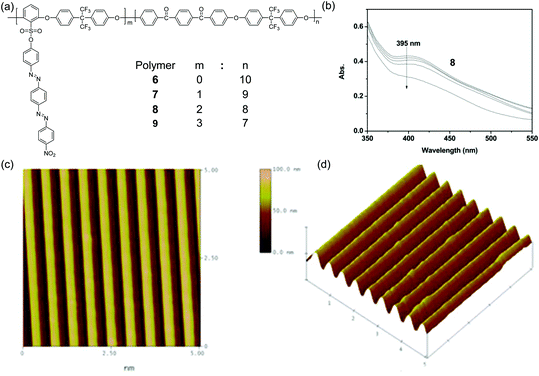 |
| Fig. 7 (a) Chemical structures of 6, 7, 8 and 9. (b) Changes in the UV-Vis absorption spectra of 8 in DMF induced by 405 nm light irradiation. (c) AFM plane view of the SRGs and (d) AFM 3-D view of the SRGs. Reprinted with permission from ref. 119. Copyright (2016) SAGE Publications. | |
Besides polymers with conjugated multi-azobenzene groups on side chains, polymers with conjugated multi-azobenzene groups on polymer backbones were also reported. Wu et al. reported a series of main-chain type bis-azopolyurethanes (10 and 11) (Fig. 8).120 The mono-azopolyurethane (12) was also studied for comparison. Polymers 10, 11, and 12 had Tg values at 182 °C, 167 °C, and 143 °C, respectively.120 All of them showed photoinduced birefringence (Fig. 8b). Polymers 10 and 11 displayed smaller birefringence than polymer 12 due to the bent V shape of the bis-azobenzene groups that did not generate large birefringence. Wu et al. quantitatively investigated the stability of birefringence with parameter En, where En is defined as the ratio of birefringence conserved for a very long time to saturated birefringence. A higher value of En meant higher stability. They found that the birefringence of 10 and 11 showed higher stability than that of 12 at high temperatures, even close to Tg (Fig. 8c), which was attributed to the following two reasons: (1) the bulky bis-azobenzene groups with lower mobility enhanced the stability of the orientation; (2) the special architectures of V-shaped bis-azobenzene groups might result in higher relative stability of the photoinduced birefringence. The SRG formed in polymer 11 could be only partly erased by heating (Fig. 8d and e), which was distinct from thermally erasable SRGs reported in other literature studies.121,122 Based on this work, the authors further investigated photoinduced birefringence and SRGs in polyurethane elastomers with bis-azobenzene groups in the hard segment.123
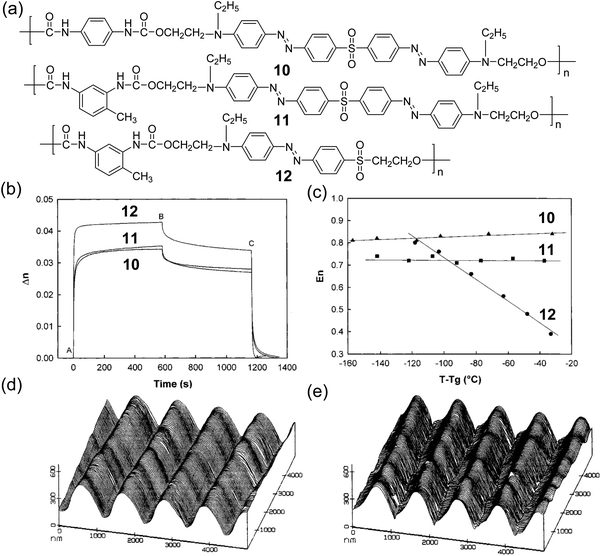 |
| Fig. 8 (a) Chemical structures of 10, 11, and 12. (b) Typical birefringence curves for 10, 11, and 12 films. LPL is turned on at point A and turned off at point B; CPL is turned on at point C. (c) Long-term stable birefringence, En, which is denoted as the ratio of birefringence conserved for a very long time to saturated birefringence, as a function of reduced temperature. The lines are only a guide to the eye. ▲, 10; ■, 11; ●, 12. AFM surface grating profile of 11 observed before (d) and after (e) thermal treatment at 182 °C for 90 min. Reprinted with permission from ref. 120. Copyright (2001) American Chemical Society. | |
Another type of main-chain conjugated multi-azopolymer, which contained azobenzene crown ether moieties, has been studied by Ardeleanu et al.124,125 This type of multi-azopolymer also showed good thermal stability and reversible photoisomerization behavior.
In addition, a novel organo-phosphonate with bis-azobenzene moieties in the main chain has been reported by Homocianu et al.126 The new structure endowed the polymers with reversible dual stimuli response, such as light and pH.
Supramolecular multi-azopolymers
In the above two sections, multi-azobenzene groups were introduced into polymers covalently. In contrast, multi-azobenzene groups can also be introduced into polymers via supramolecular interactions. Supramolecular multi-azobenzene groups can be introduced into polymers in series or in parallel.
One of us designed supramolecular bis-azopolymers by connecting a side-chain azopolymer with azobenzene small molecules via hydrogen bonding (Fig. 9a).80 Compared with the mono-azopolymer (13, Fig. 9a), the supramolecular polymers 13(14)x showed larger photoinduced birefringence and better stability when the content of 14 increased (Fig. 9b). These results are attributed to higher concentrations of azobenzene groups, a higher aspect ratio of the chromophores, and more ordered structures based on chromophore–chromophore interactions. In addition, by using a newly designed laser direct writing system (Fig. 9c), the supramolecular polymer 13(14)1.0 was used for four-dimensional optical recording by integrating the polarization and the intensity of the laser and the planar two dimensions (Fig. 9d), which had an information density 20 times that of a normal DVD. Fig. 9(e–j) show the polarized optical microscopy images of the microstructure at different angles. These types of supramolecular bis-azopolymers have better solubility and processability than the covalent bis-azopolymers.
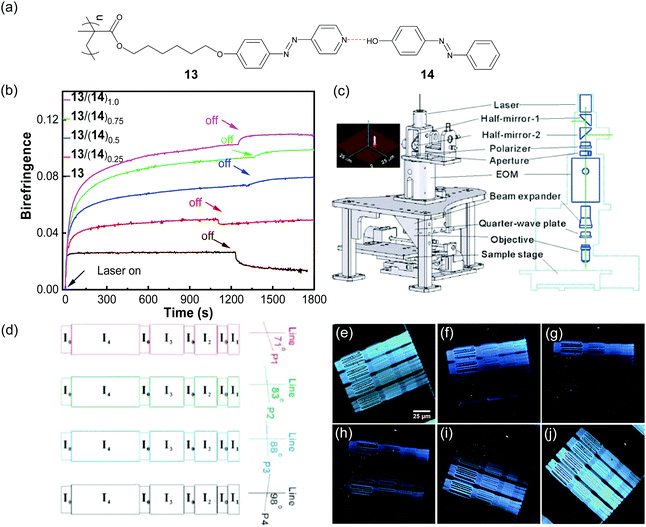 |
| Fig. 9 (a) Chemical structures of 13, 14, and the supramolecular polymer. (b) Photoinduced birefringence of the spin-casting films of 13 and 13/(14)x (x is the molar ratio of 14/13). (c) Schematic model of the laser direct writing system for optical recording. The energy distribution of the laser around the focal plane is shown in the upper left. (d) Schematic model of the four-dimensional patterns with 5 different writing powers and 4 different polarization directions. I0 = 2 mw, I1 = 4 mw, I2 = 6 mw, I3 = 8 mw and I4 = 10 mw. Polarized optical microscopy images of four-dimensional patterns with different angles: (e) 65°, (f) 80°, (g) 90°, (h) 95°, (i) 107°, and (j) 130°. Reproduced with permission from ref. 80, copyright 2010, the Royal Society of Chemistry. | |
Priimagi et al. reported another type of supramolecular bis-azopolymer by connecting poly(4-vinylpyridine) (P4VP) with bis-azobenzene small molecules via hydrogen bonding.127–129 They prepared SRGs on the supramolecular bis-azopolymers. Furthermore, Vapaavuori et al. compared the photoresponsive properties of supramolecular polymers with bis-azobenzene and mono-azobenzene groups (Fig. 10).92 Supramolecular multi-azopolymers showed better photoinduced birefringence (Fig. 10c). Supramolecular bis-azopolymers 15(17)0.5 exhibited more efficient photoinduced SRG formation (Fig. 10d). A few investigations based on similar supramolecular azopolymers were reported.129–132
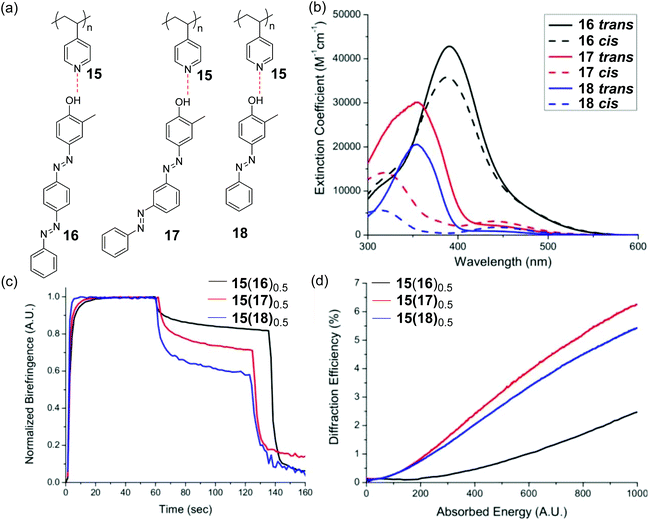 |
| Fig. 10 (a) Chemical structures of 15, 16, 17, and 18 and hydrogen-bonded supramolecular polymers constructed using these compounds. (b) UV–Vis absorption spectra of 16, 17, and 18 in THF. (c) Normalized birefringence for thin films of 15(16)0.5, 15(17)0.5, and 15(18)0.5 induced by 488 nm light, where 0.5 means the average number of azobenzene chromophores per polymer repeat unit. (d) First-order diffraction efficiencies of 15(16)0.5, 15(17)0.5 and 15(18)0.5 during SRG inscription under 488 nm light irradiation as a function of absorbed energy. Reprinted with permission from ref. 92. Copyright (2014) American Chemical Society. | |
Besides hydrogen-bonded supramolecular multi-azopolymers, supramolecular multi-azopolymers based on other supramolecular interactions such as host–guest interactions133 and ionic interactions84 have also been investigated. These polymers formed supramolecular hydrogels with multi-stimuli responsive properties133 and were used for photoinduced SRGs.84
The above-mentioned examples showed that supramolecular multi-azobenzene groups can be introduced into polymers in series, while del Barrio et al. demonstrated supramolecular polymers that had hydrogen-bonded multi-azobenzene groups in parallel (Fig. 11).134 The photoinduced orientations of these supramolecular multi-azopolymers were studied (Fig. 11a–c). The supramolecular multi-azopolymers showed large and stable photoinduced birefringence even at low contents of the azobenzene compounds (Fig. 11b–d), which were superior to the systems previously reported.135 Additionally, other studies based on hydrogen-bonded polymers with supramolecular multi-azobenzene groups in parallel have been reported. Photoinduced anisotropy,136 orientation,136,137 or birefringence136,137 and the photoinduced deformation138 of these supramolecular multi-azopolymers have been investigated.
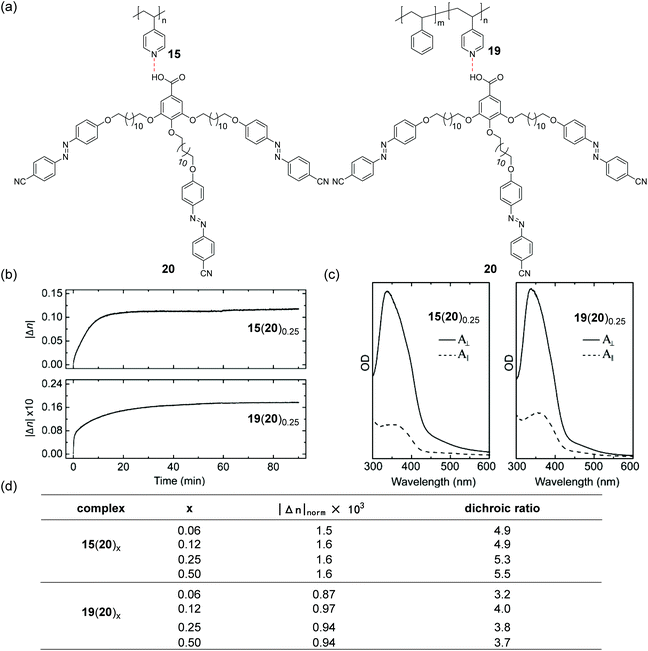 |
| Fig. 11 (a) Chemical structures of 15, 19, 20, and supramolecular polymers constructed using these compounds. (b) Evolution of the photoinduced birefringence in the thermally treated films of 15(20)0.25 and 19(20)0.25 under the irradiation of 488 nm LPL (films were irradiated between t = 0 and t = 60 min). (c) Stable polarized absorption spectra of 15(20)0.25 and 19(20)0.25 recorded at room temperature after irradiation with 488 nm LPL for 60 min and subsequent evolution in the dark for 30 min at the same temperature. (d) Values of |Δn|norm after the irradiation and relaxation periods and the equilibrium dichroic ratio. Reprinted with permission from ref. 134. Copyright (2014) American Chemical Society. | |
Conclusions and perspective
We introduced multi-azopolymers that exhibit interesting photoresponsive properties. Both side-chain and main-chain multi-azopolymers have been investigated. The photoisomerization of multi-azobenzene groups resulted in more types of isomers. Because multi-azobenzene groups usually have a larger aspect ratio than mono-azobenzene groups, they exhibit larger photoinduced birefringence. Multi-azobenzene groups can be introduced into polymers via supramolecular interactions. This supramolecular strategy may simplify the route for the preparation of multi-azopolymers.139 Moreover, the composition of supramolecular complexes can be adjusted easily by changing the ratio of azobenzene components and polymer matrices.
Although multi-azopolymers have been extensively studied, we believe that the full potential of multi-azopolymers has not been realised yet. Some key areas in photoresponsive polymers remain to profit from designing multi-azopolymers. Some challenges with regard to multi-azopolymers should be addressed to take this field forward.
First, multi-azobenzene groups, especially the conjugated ones, have poorer solubility than their mono-azobenzene counterparts, which makes the synthesis and processing of multi-azopolymers more difficult. Decoupling the conjugation of multi-azobenzene groups via supramolecular interactions may improve solubility and address the issue of processing.
Second, almost all the reported multi-azopolymers have linear architectures. A field that awaits exploration is the combination of interesting photoresponsiveness of multi-azobenzene groups with a variety of polymer topologies, such as stars, branches, and dendrimers. Such a design will result in new photoresponsive polymers.
Thirdly, hydrogen bonding, host–guest interactions133 and ionic interactions84 have been used to construct supramolecular multi-azopolymers. Supramolecular multi-azopolymers constructed using other supramolecular interactions such as halogen bonding140,141 and coordination81–83 are still awaiting exploration.
Fourth, multi-azobenzene small molecules with heterogenic azobenzene groups showed orthogonal photoswitching by light with different wavelengths.85,142,143 Such heterogenic multi-azobenzene groups can be switched between more than 2 states. Multi-azopolymers with heterogenic azobenzene groups have not been explored yet and can be controlled with light of different colors.
Conflicts of interest
There are no conflicts to declare.
Acknowledgements
S. W. acknowledges the Thousand Talents Plan and the Deutsche Forschungsgemeinschaft (DFG, WU 787/2-1 and WU 787/8-1) for financial support. Open Access funding provided by the Max Planck Society.
Notes and references
- G. S. Hartley, Nature, 1937, 140, 281 CrossRef CAS.
- Y. Wu, S. Wu, X. Tian, X. Wang, W. Wu, G. Zou and Q. Zhang, Soft Matter, 2011, 7, 716–721 RSC.
- Y. Wu, S. Wu, G. Zou and Q. Zhang, Soft Matter, 2011, 7, 9177–9183 RSC.
- X. Tong, G. Wang, A. Soldera and Y. Zhao, J. Phys. Chem. B, 2005, 109, 20281–20287 CrossRef CAS.
- G. S. Kumar and D. C. Neckers, Chem. Rev., 1989, 89, 1915–1925 CrossRef CAS.
- M. Irie and H. Tanaka, Macromolecules, 1983, 16, 210–214 CrossRef CAS.
- Y. Norikane, E. Uchida, S. Tanaka, K. Fujiwara, E. Koyama, R. Azumi, H. Akiyama, H. Kihara and M. Yoshida, Org. Lett., 2014, 16, 5012–5015 CrossRef CAS.
- Y. Norikane, S. Tanaka and E. Uchida, CrystEngComm, 2016, 18, 7225–7228 RSC.
- Y. Hao, S. Huang, Y. Guo, L. Zhou, H. Hao, C. J. Barrett and H. Yu, J. Mater. Chem. C, 2019, 7, 503–508 RSC.
- C. Appiah, G. Woltersdorf, R. A. Perez-Camargo, A. J. Muller and W. H. Binder, Eur. Polym. J., 2017, 97, 299–307 CrossRef CAS.
- H. Zhou, C. Xue, P. Weis, Y. Suzuki, S. Huang, K. Koynov, G. K. Auernhammer, R. Berger, H.-J. Butt and S. Wu, Nat. Chem., 2017, 9, 145–151 CrossRef CAS.
- W.-C. Xu, S. Sun and S. Wu, Angew. Chem., Int. Ed., 2019, 58, 9712–9740 Search PubMed.
- G. S. Kumar, P. Depra, K. Zhang and D. C. Neckers, Macromolecules, 1984, 17, 2463–2467 CrossRef CAS.
- G. S. Kumar, P. DePra and D. C. Neckers, Macromolecules, 1984, 17, 1912–1917 CrossRef CAS.
- M. Irie, Y. Hirano, S. Hashimoto and K. Hayashi, Macromolecules, 1981, 14, 262–267 CrossRef CAS.
- T. Takeshima, W.-Y. Liao, Y. Nagashima, K. Beppu, M. Hara, S. Nagano and T. Seki, Macromolecules, 2015, 48, 6378–6384 CrossRef CAS.
- C. Zong, Y. Zhao, H. Ji, X. Han, J. Xie, J. Wang, Y. Cao, S. Jiang and C. Lu, Angew. Chem., Int. Ed., 2016, 55, 3931–3935 CrossRef CAS.
- C. Zong, U. Azhar, C. Zhou, J. Wang, L. Zhang, Y. Cao, S. Zhang, S. Jiang and C. Lu, Langmuir, 2019, 35, 2601–2609 Search PubMed.
- E. Uchida, K. Sakaki, Y. Nakamura, R. Azumi, Y. Hirai, H. Akiyama, M. Yoshida and Y. Norikane, Chem. – Eur. J., 2013, 19, 17391–17397 CrossRef CAS.
- R. H. Zha, G. Vantomme, J. A. Berrocal, R. Gosens, B. de Waal, S. Meskers and E. W. Meijer, Adv. Funct.
Mater., 2018, 28, 1703952 CrossRef.
- S. Wu, F. Shi, Q. Zhang and C. Bubeck, Macromolecules, 2009, 42, 4110–4117 CrossRef CAS.
- J. Huang, S. Beckemper, S. Wu, J. Shen, Q. Zhang, K. Wang and A. Gillner, Phys. Chem. Chem. Phys., 2011, 13, 16150–16158 RSC.
- S. Wu and J. Huang, RSC Adv., 2012, 2, 12084–12087 RSC.
- Y. Yue, Y. Norikane, R. Azumi and E. Koyama, Nat. Commun., 2018, 9, 3234 CrossRef.
- E. Uchida, R. Azumi and Y. Norikane, Nat. Commun., 2015, 6, 7310 CrossRef CAS.
-
H. Rau, Photochemistry and Photophysics, CRC, Boca Raton, 1990 Search PubMed.
- H. M. D. Bandara and S. C. Burdette, Chem. Soc. Rev., 2012, 41, 1809–1825 RSC.
- P. Weis and S. Wu, Macromol. Rapid Commun., 2018, 39, 1700220 CrossRef.
- P. Weis, D. Wang and S. Wu, Macromolecules, 2016, 49, 6368–6373 CrossRef CAS.
- D. Han, X. Tong, Y. Zhao, T. Galstian and Y. Zhao, Macromolecules, 2010, 43, 3664–3671 CrossRef CAS.
- Y. Kawashima, M. Nakagawa, T. Seki and K. Ichimura, Chem. Mater., 2002, 14, 2842–2844 CrossRef CAS.
- Y. Morikawa, S. Nagano, K. Watanabe, K. Kamata, T. Iyoda and T. Seki, Adv. Mater., 2006, 18, 883–886 CrossRef CAS.
- T. Wang, X. Li, Z. Dong, S. Huang and H. Yu, ACS Appl. Mater. Interfaces, 2017, 9, 24864–24872 CrossRef CAS.
- H. Yu, T. Iyoda and T. Ikeda, J. Am. Chem. Soc., 2006, 128, 11010–11011 CrossRef CAS.
- A. Housni, Y. Zhao and Y. Zhao, Langmuir, 2010, 26, 12366–12370 CrossRef CAS.
- S. Wu, X. Yu, J. Huang, J. Shen, Q. Yan, X. Wang, W. Wu, Y. Luo, K. Wang and Q. Zhang, J. Mater. Chem., 2008, 18, 3223–3229 RSC.
- J. Shen, S. Wu, J. Huang, Q. Zhang and K. Wang, Thin Solid Films, 2010, 518, 2128–2133 CrossRef CAS.
- S. Wu, J. Shen, J. Huang, Y. Wu, Z. Zhang, Y. Hu, W. Wu, W. Huang, K. Wang and Q. Zhang, Polymer, 2010, 51, 1395–1403 CrossRef CAS.
- S. Fu and Y. Zhao, Macromolecules, 2015, 48, 5088–5098 CrossRef CAS.
- S. Nagano, Y. Koizuka, T. Murase, M. Sano, Y. Shinohara, Y. Amemiya and T. Seki, Angew. Chem., Int. Ed., 2012, 51, 5884–5888 CrossRef CAS.
- A. V. Bogdanov and A. K. Vorobiev, J. Phys. Chem. B, 2013, 117, 13936–13945 CrossRef CAS.
- M. Sailer, R. Fernández, X. Lu and C. J. Barrett, Phys. Chem. Chem. Phys., 2013, 15, 19985–19989 RSC.
- X. Wang, J. Vapaavuori, C. G. Bazuin and C. Pellerin, Macromolecules, 2018, 51, 1077–1087 CrossRef CAS.
- P. Che, Y. He and X. Wang, Front. Chem. Eng. China, 2007, 1, 360–364 CrossRef CAS.
- P. Che, Y. He and X. Wang, Chin. J. Polym. Sci., 2012, 30, 478–486 CrossRef CAS.
- H. Yu, K. Okano, A. Shishido, T. Ikeda, K. Kamata, M. Komura and T. Iyoda, Adv. Mater., 2005, 17, 2184–2188 CrossRef CAS.
- X. Xue, J. Zhu, Z. Zhang, N. Zhou, Y. Tu and X. Zhu, Macromolecules, 2010, 43, 2704–2712 CrossRef CAS.
- P. Weis, W. Tian and S. Wu, Chem. – Eur. J., 2018, 24, 6494–6505 CrossRef CAS.
- J. Hu, X. Li, Y. Ni, S. Ma and H. Yu, J. Mater. Chem. C, 2018, 6, 10815–10821 RSC.
- H. Zeng, O. M. Wani, P. Wasylczyk and A. Priimagi, Macromol. Rapid Commun., 2018, 39, 1700224 CrossRef.
- K. Kumar, C. Knie, D. Bléger, M. A. Peletier, H. Friedrich, S. Hecht, D. J. Broer, M. G. Debije and A. P. Schenning, Nat. Commun., 2016, 7, 11975 CrossRef CAS.
- T. J. White and D. J. Broer, Nat. Mater., 2015, 14, 1087–1098 CrossRef CAS.
- Y. Yu and T. Ikeda, Angew. Chem., Int. Ed., 2006, 45, 5416–5418 CrossRef CAS.
- K. M. Lee, M. L. Smith, H. Koerner, N. Tabiryan, R. A. Vaia, T. J. Bunning and T. J. White, Adv. Funct. Mater., 2011, 21, 2913–2918 CrossRef CAS.
- Y. Wang, S. Lin, M. Zang, Y. Xing, X. He, J. Lin and T. Chen, Soft Matter, 2012, 8, 3131–3138 RSC.
- Y. Xing, S. Lin, J. Lin and X. He, Chin. J. Polym. Sci., 2013, 31, 833–840 CrossRef CAS.
- X. Li, B. Li, M. He, W. Wang, T. Wang, A. Wang, J. Yu, Z. Wang, S. W. Hong, M. Byun, S. Lin, H. Yu and Z. Lin, ACS Appl. Mater. Interfaces, 2018, 10, 4961–4970 CrossRef CAS.
- W. Su, Y. Luo, Q. Yan, S. Wu, K. Han, Q. Zhang, Y. Gu and Y. Li, Macromol. Rapid Commun., 2007, 28, 1251–1256 CrossRef CAS.
- S. Wu, L. Niu, J. Shen, Q. Zhang and C. Bubeck, Macromolecules, 2009, 42, 362–367 CrossRef CAS.
- S. Wu, Q. Zhang and C. Bubeck, Macromolecules, 2010, 43, 6142–6151 CrossRef CAS.
- S. Wu, L. Wang, A. Kroeger, Y. Wu, Q. Zhang and C. Bubeck, Soft Matter, 2011, 7, 11535–11545 RSC.
- S. Wu, J. Huang, S. Beckemper, A. Gillner, K. Wang and C. Bubeck, J. Mater. Chem., 2012, 22, 4989–4995 RSC.
- D. Wang and S. Wu, Langmuir, 2016, 32, 632–636 CrossRef CAS.
- Y. Chen, S. Huang, T. Wang, Z. Dong and H. Yu, Macromolecules, 2019, 52, 1892–1898 CrossRef CAS.
- S. Huang, Y. Chen, S. Ma and H. Yu, Angew. Chem., Int. Ed., 2018, 57, 12524–12528 CrossRef CAS PubMed.
- Y. Ni, X. Li, J. Hu, S. Huang and H. Yu, Chem. Mater., 2019, 31, 3388–3394 CrossRef CAS.
- A. Natansohn and P. Rochon, Chem. Rev., 2002, 102, 4139–4176 CrossRef CAS.
- K. Anderle, R. Birenheide, M. Eich and J. H. Wendorff, Makromol. Chem., Rapid Commun., 1989, 10, 477–483 CrossRef CAS.
- C. J. Barrett, A. L. Natansohn and P. L. Rochon, J. Phys. Chem., 1996, 100, 8836–8842 CrossRef CAS.
- C. J. Barrett, P. L. Rochon and A. L. Natansohn, J. Chem. Phys., 1998, 109, 1505–1516 CrossRef CAS.
- J. Kumar, L. Li, X. L. Jiang, D.-Y. Kim, T. S. Lee and S. Tripathy, Appl. Phys. Lett., 1998, 72, 2096–2098 CrossRef CAS.
- S. Bian, J. M. Williams, D. Y. Kim, L. Li, S. Balasubramanian, J. Kumar and S. Tripathy, J. Appl. Phys., 1999, 86, 4498–4508 CrossRef CAS.
- O. M. Tanchak and C. J. Barrett, Macromolecules, 2005, 38, 10566–10570 CrossRef CAS.
- T. Ikeda, J. Mamiya and Y. Yu, Angew. Chem., Int. Ed., 2007, 46, 506–528 CrossRef CAS.
- Y. Xiong, L. Zhang, P. Weis, P. Naumov and S. Wu, J. Mater. Chem. A, 2018, 6, 3361–3366 RSC.
- S. Wu and C. Bubeck, Macromolecules, 2013, 46, 3512–3518 CrossRef CAS.
- Y. Zhou, D. Wang, S. Huang, G. Auernhammer, Y. He, H.-J. Butt and S. Wu, Chem. Commun., 2015, 51, 2725–2727 RSC.
- Y. Xiong, Z. Chen, H. Wang, L.-M. Ackermann, M. Klapper, H.-J. Butt and S. Wu, Chem. Commun., 2016, 52, 14157–14160 RSC.
- J. Huang, Y. Huang and S. Wu, Front. Chem. Sci. Eng., 2018, 12, 450–456 CrossRef CAS.
- S. Wu, S. Duan, Z. Lei, W. Su, Z. Zhang, K. Wang and Q. Zhang, J. Mater. Chem., 2010, 20, 5202–5209 RSC.
- L. Cui, S. Dahmane, X. Tong, L. Zhu and Y. Zhao, Macromolecules, 2005, 38, 2076–2084 CrossRef CAS.
- S. Dahmane, A. Lasia and Y. Zhao, Macromol. Chem. Phys., 2006, 207, 1485–1491 CrossRef CAS.
- S. Dahmane and Y. Zhao, J. Appl. Polym. Sci., 2006, 102, 744–750 CrossRef CAS.
- O. Kulikovska, L. M. Goldenberg and J. Stumpe, Chem. Mater., 2007, 19, 3343–3348 CrossRef CAS.
- D. Wang, M. Wagner, A. K. Saydjari, J. Mueller, S. Winzen, H.-J. Butt and S. Wu, Chem. – Eur. J., 2017, 23, 2628–2634 CrossRef CAS.
- D. Wang, M. Wagner, H.-J. Butt and S. Wu, Soft Matter, 2015, 11, 7656–7662 RSC.
- H. Yu and T. Ikeda, Adv. Mater., 2011, 23, 2149–2180 CrossRef CAS.
- S. Lee, H. S. Kang and J.-K. Park, Adv. Mater., 2012, 24, 2069–2103 CrossRef CAS.
- D. Wang and X. Wang, Prog. Polym. Sci., 2013, 38, 271–301 CrossRef CAS.
- X. Meng, A. Natansohn and P. Rochon, Polymer, 1997, 38, 2677–2682 CrossRef CAS.
- L. Angiolini, T. Benelli, L. Giorgini, F. Mauriello, E. Salatelli, R. Bozio, A. Daurù and D. Pedron, Eur. Polym. J., 2007, 43, 3550–3561 CrossRef CAS.
- J. Vapaavuori, A. Goulet-Hanssens, I. T. Heikkinen, C. J. Barrett and A. Priimagi, Chem. Mater., 2014, 26, 5089–5096 CrossRef CAS.
- N. Hosono, T. Kajitani, T. Fukushima, K. Ito, S. Sasaki, M. Takata and T. Aida, Science, 2010, 330, 808–811 CrossRef CAS.
- Y. Yu, M. Nakano and T. Ikeda, Nature, 2003, 425, 145 CrossRef CAS.
- Z. Chen, Y.-T. Chan, D. Miyajima, T. Kajitani, A. Kosaka, T. Fukushima, J. M. Lobez and T. Aida, Nat. Commun., 2016, 7, 13640 CrossRef.
- H. Akiyama, S. Kanazawa, M. Yoshida, H. Kihara, H. Nagai, Y. Norikane and R. Azumi, Mol. Cryst. Liq. Cryst., 2014, 604, 64–70 CrossRef CAS.
- H. Akiyama, S. Kanazawa, Y. Okuyama, M. Yoshida, H. Kihara, H. Nagai, Y. Norikane and R. Azumi, ACS Appl. Mater. Interfaces, 2014, 6, 7933–7941 CrossRef CAS.
- H. Akiyama and M. Yoshida, Adv. Mater., 2012, 24, 2353–2356 CrossRef CAS.
- Y. Zhu and X. Wang, Polym. Chem., 2013, 4, 5108–5118 RSC.
- D.-Y. Kim, S. Shin, W.-J. Yoon, Y.-J. Choi, J.-K. Hwang, J.-S. Kim, C.-R. Lee, T.-L. Choi and K.-U. Jeong, Adv. Funct. Mater., 2017, 27, 1606294 CrossRef.
- K. Wang, L. Yin, T. Miu, M. Liu, Y. Zhao, Y. Chen, N. Zhou, W. Zhang and X. Zhu, Mater. Chem. Front., 2018, 2, 1112–1118 RSC.
- J. Royes, A. Nogales, T. A. Ezquerra, L. Oriol, R. M. Tejedor and M. Piñol, Polymer, 2018, 143, 58–68 CrossRef CAS.
- J. Tian, L. Fu, Z. Liu, H. Geng, Y. Sun, G. Lin, X. Zhang, G. Zhang and D. Zhang, Adv. Funct. Mater., 2019, 29, 1807176 CrossRef.
- D. Brown, A. Natansohn and P. Rochon, Macromolecules, 1995, 28, 6116–6123 CrossRef CAS.
- J. Wang, L. Zhang, Y. Niu, Z. Liang, Y. Chen, Y. Huang, H. Wang and W. Lin, Polym. Int., 2003, 52, 1165–1168 CrossRef CAS.
- M. Jin, Q. X. Yang, R. Lu, T. H. Xu and Y. Y. Zhao, J. Polym. Sci., Part A: Polym. Chem., 2004, 42, 4237–4247 CrossRef CAS.
- C. Cojocariu and P. Rochon, Macromolecules, 2005, 38, 9526–9538 CrossRef CAS.
- K. Okano, O. Tsutsumi, A. Shishido and T. Ikeda, J. Am. Chem. Soc., 2006, 128, 15368–15369 CrossRef CAS.
-
J. Y. Kim, T. Fukuda, D. Barada, H. Ushijima and K. Yase, presented in part at Organic Holographic Materials and Applications IV, San Diego, California, United States, September, 2006 Search PubMed.
- M. Jin, R. Lu, Q. X. Yang, C. Y. Bao, R. Sheng, T. H. Xu and Y. Y. Zhao, J. Polym. Sci., Part A: Polym. Chem., 2007, 45, 3460–3472 CrossRef CAS.
- Z. Zheng, L. Wang, Z. Su, J. Xu, J. Yang and Q. Zhang, J. Photochem. Photobiol., A, 2007, 185, 338–344 CrossRef CAS.
- Z. Zhang, Y. Hu, Y. Luo, Q. Zhang, W. Huang and G. Zou, Opt. Commun., 2009, 282, 3282–3285 CrossRef CAS.
- B. L. Lachut, S. A. Maier, H. A. Atwater, M. J. A. de Dood, A. Polman, R. Hagen and S. Kostromine, Adv. Mater., 2004, 16, 1746–1750 CrossRef CAS.
- L. Angiolini, T. Benelli, L. Giorgini, F. Mauriello and E. Salatelli, Macromol. Symp., 2006, 234, 68–75 CrossRef CAS.
- L. Angiolini, T. Benelli, L. Giorgini, F. Mauriello and E. Salatelli, Macromol. Chem. Phys., 2007, 208, 207–217 CrossRef CAS.
- S. Wu, F. Zeng, S. Yao, Z. Tong, W. She and D. Luo, Macromolecules, 2003, 36, 9292–9294 CrossRef CAS.
- T. Buruiana and E. C. Buruiana, J. Polym. Sci., Part A: Polym. Chem., 2004, 42, 5463–5470 CrossRef CAS.
- X. Wang, J. Yin and X. Wang, Polymer, 2011, 52, 3344–3356 CrossRef CAS.
- J. Zhang, H. Zhang, Q. Zhang, Z. Jiang, Q. Chen and Y. Zhang, High Perform. Polym., 2016, 28, 518–524 CrossRef CAS.
- Y. Wu, A. Natansohn and P. Rochon, Macromolecules, 2001, 34, 7822–7828 CrossRef CAS.
- D. Y. Kim, S. K. Tripathy, L. Li and J. Kumar, Appl. Phys. Lett., 1995, 66, 1166–1168 CrossRef CAS.
- P. Rochon, E. Batalla and A. Natansohn, Appl. Phys. Lett., 1995, 66, 136–138 CrossRef CAS.
- Y. Wu, A. Natansohn and P. Rochon, Macromolecules, 2004, 37, 6090–6095 CrossRef CAS.
- R. Ardeleanu, A. Airinei, G. Sacarescu and L. Sacarescu, Eur. Polym. J., 2002, 38, 2265–2270 CrossRef CAS.
- R. Ardeleanu, N. Fifere, V. Barboiu, L. Sacarescu and A. Airinei, J. Mol. Liq., 2017, 229, 362–370 CrossRef CAS.
- M. Homocianu, D. Serbezeanu, I.-D. Carja, A. M. Macsim, T. Vlad-Bubulac and A. Airinei, RSC Adv., 2016, 6, 49980–49987 RSC.
- J. Vapaavuori, A. Priimagi and M. Kaivola, J. Mater. Chem., 2010, 20, 5260–5264 RSC.
- A. Priimagi, M. Kaivola, M. Virkki, F. J. Rodriguez and M. Kauranen, J. Nonlinear Opt. Phys. Mater., 2010, 19, 57–73 CrossRef CAS.
- J. E. Koskela, J. Vapaavuori, J. Hautala, A. Priimagi, C. F. J. Faul, M. Kaivola and R. H. A. Ras, J. Phys. Chem. C, 2012, 116, 2363–2370 CrossRef CAS.
- D. Hu, K. Chen, G. Zou and Q. Zhang, J. Polym. Res., 2012, 19, 9983 CrossRef.
- L. M. Saiz, P. A. Oyanguren, M. J. Galante and I. A. Zucchi, Nanotechnology, 2014, 25, 065601 CrossRef.
- L. M. Saiz, I. A. Zucchi, P. A. Oyanguren, M. J. Galante, R. C. Sanfelice, D. T. Balogh and O. N. Oliveira Jr., Opt. Mater., 2014, 37, 816–822 CrossRef CAS.
- X. Wang, J. Wang, Y. Yang, F. Yang and D. Wu, Polym. Chem., 2017, 8, 3901–3909 RSC.
- J. del Barrio, E. Blasco, C. Toprakcioglu, A. Koutsioubas, O. A. Scherman, L. Oriol and C. Sánchez-Somolinos, Macromolecules, 2014, 47, 897–906 CrossRef CAS.
- J. del Barrio, E. Blasco, L. Oriol, R. Alcalá and C. Sánchez-Somolinos, J. Polym. Sci., Part A: Polym. Chem., 2013, 51, 1716–1725 CrossRef CAS.
- A. Concellón, E. Blasco, M. Piñol, L. Oriol, I. Díez, C. Berges, C. Sánchez-Somolinos and R. Alcalá, J. Polym. Sci., Part A: Polym. Chem., 2014, 52, 3173–3184 CrossRef.
- A. Kozanecka-Szmigiel, J. Konieczkowska, K. Switkowski, J. Antonowicz, B. Trzebicka, D. Szmigiel and E. Schab-Balcerzak, J. Photochem. Photobiol., A, 2016, 318, 114–123 CrossRef CAS.
- S. Wang, N. Zhang, X. Ge, Y. Wan, X. Li, L. Yan, Y. Xia and B. Song, Soft Matter, 2014, 10, 4833–4839 RSC.
- M. L. Hallensleben and B. Weichart, Polym. Bull., 1989, 22, 553–556 CrossRef CAS.
- Y. Chen, H. Yu, L. Zhang, H. Yang and Y. Lu, Chem. Commun., 2014, 50, 9647–9649 RSC.
- F. Fernandez-Palacio, M. Poutanen, M. Saccone, A. Siiskonen, G. Terraneo, G. Resnati, O. Ikkala, P. Metrangolo and A. Priimagi, Chem. Mater., 2016, 28, 8314–8321 CrossRef CAS.
- F. Zhao, L. Grubert, S. Hecht and D. Bleger, Chem. Commun., 2017, 53, 3323–3326 RSC.
- D. Wang, F. Schellenberger, J. T. Pham, H.-J. Butt and S. Wu, Chem. Commun., 2018, 54, 3403–3406 RSC.
|
This journal is © The Royal Society of Chemistry 2019 |
Click here to see how this site uses Cookies. View our privacy policy here.