Bactericidal effects of hematoporphyrin monomethyl ether-mediated blue-light photodynamic therapy against Staphylococcus aureus
Received
26th March 2018
, Accepted 28th September 2018
First published on 3rd October 2018
Abstract
The implementation of photodynamic therapy (PDT) usually uses red light as the excitation source to obtain a deeper penetration depth. However, for some superficial infectious diseases, using red-light PDT may damage the normal tissues underneath. If we choose a shorter wavelength light, then the effect of PDT can be limited to the superficial region. This study assessed the effect of blue-light PDT against Staphylococcus aureus. The absorption of hematoporphyrin monomethyl ether (HMME) by S. aureus was investigated using fluorescence spectroscopy. The bactericidal effects of HMME, light alone, and PDT using blue light (405 nm) on S. aureus were studied. The results indicate that the HMME uptake by S. aureus rapidly reached a certain value, then steadily increased with time in the range of 0–80 min, and thenreached a plateau at 80 min before a slow decline afterward. Without light irradiation, less than 2 μg ml−1 HMME showed no bactericidal effect on S. aureus. Without HMME, blue-light at a power density of 20 mW cm−2 had no significant bactericidal effect for 0.5 min to 10 min. When 2 μg ml−1 of HMME was combined with blue-light (20 mW cm−2), the bactericidal effect showed a reduction of 3
log10 with the extension of irradiation time. These results demonstrated that bacteria have the ability to absorb HMME, and HMME-mediated blue-light PDT can effectively kill the bacteria, which laid the foundation for blue-light PDT as a non-invasive treatment for superficial infectious diseases.
Introduction
Photodynamic therapy (PDT) is an advanced optical technology used in medical applications. It utilizes a light-sensitive photosensitizer, a light source and available oxygen in the body to produce highly cytotoxic singlet oxygen and other reactive oxygen species (ROS) that cause cell death and tissue destruction. Studies have shown that PDT has a curative effect not only on cancers, but also on oral benign diseases.1–3 PDT shows many attractive advantages, such as non-toxicity, target-selectivity, repeatable applicability and no drug resistance. In recent years, due to the side effects of antibiotics and the emergence of antibiotic resistant bacteria, more and more research studies have been involved in the development of anti-microbial PDT (APDT).4,5
APDT is based on the concept of PDT. A nontoxic photosensitizer agent is excited by irradiation with relatively low intensity harmless light of a suitable wavelength in the presence of oxygen, generating ROS and free radicals, which kill target microbial cells.6 Photosensitizers, as an essential component of APDT, affect the efficacy of APDT. Hematoporphyrin monomethyl ether (HMME) is a recently developed photosensitizer. It consists of two monomer porphyrins, 3-(1-methyloxyethyl)-8-(1-hydro-xyethyl) deuteroporphyrin IX and 8-(1-methyloxyethyl)-3-(1-hydroxyethyl) IX, and shows a characteristic peak absorption at 405 nm (Fig. 1). Compared to porphyrin derivatives, HMME is more stable and has a shorter light avoiding period, lower phototoxicity to normal tissues,7 and higher production of ROS. Thus it has been widely used in PDT of tumors, nevus flammeus, etc.8–15
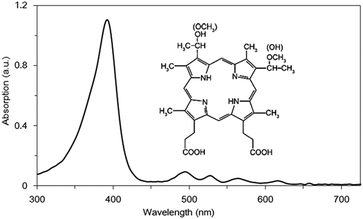 |
| Fig. 1 Absorption spectrum of HMME. | |
Many studies revealed that APDT showed promising potential as an alternative method to inactivate microbes.14,15 Commonly used photosensitizers, such as HMME,16 toluidine blue O (TBO)17 and methylene blue (MB),18 are all excited by red-light because of its strong penetrating capability. But if it was used for the treatment of superficial infection, deep normal tissues might be damaged using conventional red light PDT.19–21 Due to its shallower penetration depth, blue-light PDT could avoid damaging deep healthy tissues and would achieve good superficial treatment effects due to strong light absorption in superficial tissues.
The purpose of this study is to investigate the effect of HMME-mediated blue-light APDT on Staphylococcus aureus. We determined the incubation time by measuring the HMME concentration in S. aureus using a laser induced fluorescence spectroscopic method. We also determined the effects of HMME concentration, illumination time and light power on the bactericidal outcome for blue-light PDT. Our results provide an experimental basis for future clinical applications of HMME-mediated APDT in the treatment of bacterial superficial infectious diseases in humans.
Materials and methods
Bacteria and culture conditions
S. aureus (ATCC 6538) was cultured in Luria–Bertani (LB) broth at 37 °C for 48 h. The bacteria were washed three times with sterile saline and diluted with sterile saline to OD630 nm of 0.2 (about 108 CFU mL−1).
Photosensitizer and light sources
HMME was provided by the Laboratory for Antimalarial Drug Research of the Second Military Medical University (Shanghai, China). HMME stock solution was prepared to a concentration of 10 mg mL−1 in sterile saline and kept in darkness at −20 °C. Before use, the stock solution was appropriately diluted by sterile saline. Two diode lasers (Sanyo Electric Co, Tokyo, Japan) with continuously variable outputs of 0–500 mW were used in our study. The wavelength for the blue-light lasers is 405 nm. The laser was distributed by a fiber-optic applicator with a 0.65 mm cylindrical diffusing tip. Before irradiation, the output power was adjusted to 6.63 mW; so, the power density of the blue-light was adjusted to 20 mW cm−2. The laser output power was carefully calibrated with a power meter (PS10, Coherent Inc., Santa Clara, CA, USA) to avoid overexposure.
Measurement of incubation time
In order to measure the incubation time, a laser induced fluorescence spectroscopy method was employed. A laser (Sunlight Shenzhen Opto-Electronic Technology Co. Ltd) centered at 405 nm with a bandwidth of 20 nm was used as the light source. The fluorescence spectrum profile in the wavelength ranging from 470 nm to 750 nm was detected. The area integration of the fluorescence spectrum of HMME is directly proportional to the amount of the adsorbed HMME; thus, it was used as a measure of the amount of HMME absorbed by bacterial cells.
The specific procedure is as follows: 980 μl of bacterial cell suspension and 20 μl of HMME stock solution were mixed well to obtain the HMME concentration of 200 μg ml−1; then, 10 ml of the mixture were taken out and mixed into 40 ml sterile saline to obtain the final HMME concentration of 40 μg ml−1. The mixture was divided into 8 triplicate-groups and kept in the dark at 37 °C for 0, 5, 20, 30, 60, 80, 240 and 480 min, respectively. Then they were centrifuged (at 6000g for 3 min) and the supernatant fluids were removed. The bacteria were washed three times with sterile saline to remove unabsorbed HMME and re-suspended in sterile saline. The resultant bacterial suspension was illuminated with the selected light source to excite the fluorescence of HMME.
Toxicity of HMME on bacterial cells
The bacterial suspension and HMME stock solution were mixed well to obtain the final solutions with HMME concentrations of 0, 2, 5, 10, 20, and 40 μg ml−1, respectively. The mixtures were then kept in the dark at 37 °C for 80 min. To detect the antibacterial effects, dilution series (10−1–10−6) were made using sterile saline, and 100 μl of each dilution was plated in triplicate on BHI agar. After incubation at 37 °C for 2 days, the CFU number of surviving bacteria and log10 change in viable count for each sample were subsequently calculated.
Effects of blue-light and treatment conditions on bacterial cells
The bacterial cell suspension was incubated at 37 °C for 80 min in the dark and then randomly divided into six groups (1–6): group 1 was the control group; groups 2, 3, 4, 5, and 6 were treated with blue-light (20 mW cm−2) for 0.5, 1, 2, 5 and 10 min, respectively. Dilution series (10−1–10−6) were made using sterile saline, and 100 μl of each dilution was plated on BHI agar. Each group was prepared in triplicate. The CFU number of surviving bacteria and log10 change in viable count for each sample were subsequently calculated.
PDT treatment
The bacterial cell suspension and HMME stock solutions were mixed to obtain the final HMME concentration of 2 μg mL−1; then, the mixtures were incubated at 37 °C for 80 min in the dark. Afterwards, the mixtures were randomly divided into two groups (1 and 2): group 1 was irradiated by blue-light (20 mW cm−2) for different time periods (5, 10, 20, 30, 40, 60, 80, 100 and 120 s) and group 2 was the untreated control group. After the PDT treatment, the samples of each group were diluted into three different dilution series with sterile saline and 100 μl of each dilution was plated on BHI agar and then kept in the dark for 24 hours at 37 °C. The CFU number of surviving bacteria and log10 change in viable count for each sample were subsequently calculated. All experiments were performed in triplicate.
Statistical analysis
Statistical analysis of all data was performed using SPSS for Windows (version 13, SPSS Inc., Chicago, IL). The data were expressed as the mean ± standard deviation. The differences between each group of two were statistically analyzed by the Tukey test. Differences were considered to be significant when P < 0.05.
Results
The inset of Fig. 1 is the chemical structure of HMME. Fig. 1 shows the absorption spectrum of HMME. There are 5 absorption peaks located at 392, 494, 527, 564 and 616 nm, and the strongest absorption peak is at 392 nm. Light of 405 nm and 630 nm was used in our study. The absorptive capacity at the wavelength of 405 nm is about 32 times that of 630 nm.
After incubation with HMME for different time periods, HMME concentrations in S. aureus cells were measured by analyzing their fluorescence emission profile between 470 nm and 750 nm. Fig. 2 shows the fluorescence emission profiles of bacterial cells after incubation with 40 μg ml−1 HMME for different time periods of 5–480 min. The characteristic fluorescence emission profile is broad and there are two peaks centered at 620 nm and 680 nm. The area beneath the curve, which is directly proportional to the amount of HMME absorbed, was calculated and plotted as shown in the inset of Fig. 2. The graph displays that the amount of intracellular accumulation of HMME rapidly reached a certain value, then steadily increased with time in the range of 0–80 min, and reached a plateau at 80 min, then slowly decreased with time.
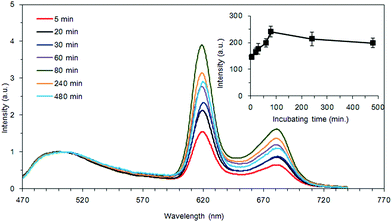 |
| Fig. 2 Fluorescence emission profiles of bacterial cells using 40 μg mL−1 HMME after 5–480 min of incubation, as determined using an OLYMPUS IX81 fluorescence microscope (A, 400). Intracellular HMME content reaches the maximum at 80 min in our study. The inset is the area under the curve indicating the dependence of fluorescence intensity of HMME adsorbed by bacteria and incubation time. Data represent mean values (n = 4), and error bars represent standard deviations. | |
The effect of HMME alone (2–40 μg mL−1) on S. aureus was determined and is shown in Fig. 3. As the HMME concentration increased, the survival rate of the bacteria in every group decreased. As shown in Fig. 3, using 2 μg mL−1 HMME alone did not show any bactericidal effect on S. aureus. When the HMME concentration reached 5 μg mL−1, the survival rate of the bacteria showed noticeable decrease (P < 0.05). It is worth noting that as the HMME concentration increased from 5 to 40 μg ml−1, the bactericidal effect enhanced.
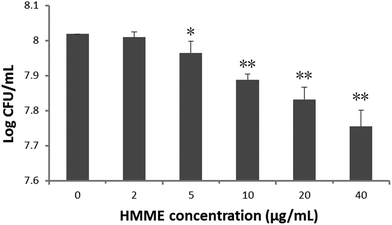 |
| Fig. 3 The dose effect of HMME on S. aureus. HMME concentrations range from 2 to 40 μg mL−1. Data were expressed as mean ± SD of the number of surviving CFUs and log10 change in viable count for different HMME concentrations, and error bars represent standard deviations. *P < 0.05; **P < 0.01 vs. controls. | |
Fig. 4 illustrates the effect of blue-light alone on S. aureus. As the irradiation time increased from 0 to 10 min, no significant bactericidal effect has been observed.
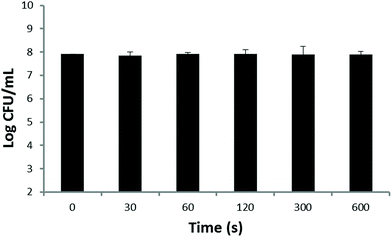 |
| Fig. 4 The effect of blue-light (20 mW cm−2) and irradiation time on S. aureus. Each value represents the mean ± SD of 10 individuals. | |
In order to obtain the effect of PDT treatment on the survival of S. aureus, the relationship between the number of surviving CFUs and log10 change and the irradiation time (t) was measured as shown in Fig. 5. It was found that as the time of illumination increased, the survival of bacteria decreased gradually. When the illumination time was longer than 30 s, there was a statistical difference between the PDT and the control group. A typical recording is shown in Fig. 5.
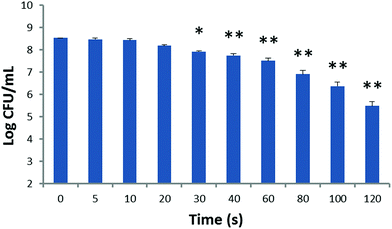 |
| Fig. 5 The relationship between the survival of S. aureus and irradiation time. PACT treatment of 2 μg mL−1 HMME, 20 mW cm−2 different blue-light and irradiation time (5, 10, 20, 30, 40, 60, 80, 100 and 120 s) was compared with control (without any treatment). The data represent mean values (n = 10), and error bars represent standard deviations. *P < 0.05; **P < 0.01 vs. controls. | |
Discussion
In our study, blue light was chosen. The reasons were as follows: at first, in PDT, the sensitizer absorbs light to produce ROS,3,22 and the amount of ROS produced is proportional to the light absorbed by the photosensitizer.23 We can see from Fig. 1 that the absorption of HMME at 405 nm is 32 times stronger than that at 630 nm, indicating that HMME has a much higher photosensitivity at 405 nm than at 630 nm. Secondly, in order to cure some clinical superficial infectious diseases, such as periodontitis, the thicknesses of plaque biofilm and gingival tissue were measured to be 27.55 ± 6.35 μm and 1.46 mm, respectively.19,20 The penetration depths of blue-light and red-light were 1 mm and 10 mm, respectively.21 If red-light PDT was chosen, due to its stronger penetrability, deeper normal tissues might be damaged. So from the perspective of killing bacteria and protecting the deeper normal tissues, blue-light PDT is certainly a better option than red-light PDT.
The effect of PDT was related to the concentration of photosensitizer attachment to bacteria. So it is necessary to observe the relationship between the concentration of the photosensitizer and time. We found from the measurement of incubation time that quite a large amount of HMME was adsorbed when the bacterial cell suspension and HMME stock solutions were mixed, as seen in the inset of Fig. 2. The HMME attachment to bacteria increased with incubation time but did not change much, which means that we could perform PDT immediately after applying the drug, and that there is no need to wait. In this study, 40 μg mL−1 HMME was selected for obtaining strong fluorescence signals and high signal-to-noise ratio.
In order to avoid the effect of photosensitizer toxicity and light on the final effect of PDT, drug toxicity and light-alone experiments were performed. Our results showed that obvious bactericidal effects by the photosensitizer were found when the HMME concentration is higher than 5 μg ml−1 (Fig. 3). This corroborates the findings of Pratten and Wilson who used higher light intensities to perform PDT (66, 132, and 264 J cm−2).24 As the HMME concentration further was increased to 5 μg ml−1, the direct bactericidal effect was enhanced for drug only. So the HMME concentration of 2 μg mL−1 was chosen to perform our PDT treatments. It can be seen from Fig. 4 that there were no obvious effects when blue-light (20 mW cm−2) alone irradiates at the chosen power intensity.
The implementation of PDT involves many factors, including photosensitizer concentration, light power, irradiation time and so on.14 In our study, in order to find the relationship between parameters of PDT and bactericidal effect, a lot of experiments had been conducted under different experimental conditions, including photosensitizer concentration, light power and irradiation time. By analyzing the experimental results, we found from Fig. 3 and 4 that no obvious effects were found in the photosensitizer (less than 2 μg/ml) alone group or light (20 mW/cm2) alone group. However, in the PDT, excellent action in killing bacteria has been seen under the same concentration of HMME and power density and irradiation time had a determinant effect on lethal photosensitization of the organisms. HMME mediated blue-light PDT therapy exhibited very good antibacterial effects in a relatively short time. The survivor bacteria decreased markedly as the irradiation time was 120 s, and a 3
log10 reduction at 2 μg ml−1 HMME combined with 20 mW cm−2 blue-light is achieved compared to control samples (Fig. 5). In addition, the CFU assay also exhibited irradiation time dependence. The study about the antibacterial effect of S. aureus by PDT had been done by many researchers. Honglue Tan's experiments show that 40 mM ALA combined with 300 J cm−2 light dose led to a 2
log10 reduction in colony count.25 Mrinalini Sharma et al. observed only 2
log10 reduction in CFU with 40 μM TBO and 100 J cm−2 light dose.26 Mariusz Grinholc et al. reported that protoporphyrin IX as well as PPArg2 exert an effective antibacterial activity at the concentration of 10 μM, illumination lasting for 60 min and a light dose of only 12 J cm−2. In their studies, the phototoxic effect resulted in 2.4 and 2.1
log10 reduction (for PPIX and PPArg2, respectively).27 Photodynamic therapy with the use of another water-soluble porphyrin sensitizer, a haematoporphyrin diarginate derivative (HpD-Arg2), was studied by Lasocki et al. When 25 μg ml−1 of HpD-Arg2 was applied, the number of viable S. aureus cells decreased 99.99% after 30 min under illumination (the light intensity was 80 klx).28 Similar results were observed by Szpakowska et al. In their experiments, the minimum bactericidal concentration (MBC) of HpD-Arg2 ranged from 1.6 to 50 μg ml−1.29 Our results suggest that HMME mediated blue-light PDT therapy showed a better antibacterial effect than that of the reported photosensitizers, and the irradiation time was relatively short.
The antibacterial effect of PDT is unquestionable, while the penetrating depth is the main problem. In order to obtain better penetration, red-light has been chosen by many researchers. But for superficial tissue infections, such as periodontal disease, blue-light PDT is a good choice. The antibacterial effect can be determined by the illumination time when the photosensitizer and light power are determined, which has great guiding significance for clinical operations. However, it must be further investigated whether the treatment is safe in animals, and many experiments will be required.
Compliance with ethical standards
This study was funded by a government research funding. This article does not contain any studies with human participants or animals performed by any of the authors. Informed consent was obtained from all individual participants included in the study.
Conflicts of interest
All authors declare that he/she has no conflict of interest.
Acknowledgements
This study was supported by the Natural Science Foundation of Heilongjiang Province (no. QC2016119) and the Technology Research and Development Project of Harbin (no. 2016RQQXJ216). We express thanks to the Department of Physics and the School of Environmental and Municipal Engineering, Harbin Institute of Technology, for their kind supply of materials used in this study.
References
- A. D. Garg, M. Bose, M. I. Ahmed, W. A. Bonass and S. R. Wood, In vitro studies on erythrosine-based photodynamic therapy of malignant and pre-malignant oral epithelial cells, PLoS One, 2012, 7, e34475 CrossRef CAS PubMed.
- M. T. Wan and J. Y. Lin, Current evidence and applications of photodynamic therapy in dermatology, Clin., Cosmet. Invest. Dermatol., 2014, 7, 145–163 Search PubMed.
- G. B. Kharkwal, S. K. Sharma, Y. Y. Huang, T. Dai and M. R. Hamblin, Photodynamic therapy for infections: clinical applications, Lasers Surg. Med., 2011, 43, 755–767 CrossRef PubMed.
- Z. Oruba, P. Labuz, W. Macyk and M. Chomyszyn-Gajewska, Antimicrobial photodynamic therapy-A discovery originating from the pre-antibiotic era in a novel periodontal therapy, Photodiagn. Photodyn. Ther., 2015, 12, 612–618 CrossRef CAS PubMed.
- A. Ichinose-Tsuno, A. Aoki, Y. Takeuchi, T. Kirikae, T. Shimbo, M. C. Lee, F. Yoshino, Y. Maruoka, T. Itoh, I. Ishikawa and Y. Izumi, Antimicrobial photodynamic therapy suppresses dental plaque formation in healthy adults: a randomized controlled clinical trial, BMC Oral Health, 2014, 14, 152–161 CrossRef PubMed.
- T. Maisch, A new strategy to destroy antibiotic resistant microorganisms: antimicrobial photodynamic treatment, Mini-Rev. Med. Chem., 2009, 9, 974–983 CrossRef CAS PubMed.
- J. Cheng, H. Liang, Q. Li, C. Peng, Z. Li, S. Shi, L. Yang, Z. Tian, Y. Tian, Z. Zhang and W. Cao, Hematoporphyrin monomethyl ether-mediated photodynamic effects on THP-1 cell-derived macrophages, J. Photochem. Photobiol., B, 2010, 101, 9–15 CrossRef CAS PubMed.
- K. Song, B. Kong, L. Li, Q. Yang, Y. Wei and X. Qu, Intraperitoneal photodynamic therapy for an ovarian cancer ascite model in Fischer 344 rat using hematoporphyrin monomethyl ether, Cancer Sci., 2007, 98, 1959–1964 CrossRef CAS PubMed.
- K. Song, J. Li, L. Li, P. Zhang, F. Geng, R. Dong, Q. Yang, X. Qu and B. Kong, Intracellular metabolism, subcellular localization and phototoxicity of HMME/HB in ovarian cancer cells, Anticancer Res., 2011, 31, 3229–3235 CAS.
- Z. Tian, X. Quan, A. W. Leung, J. Xiang and C. Xu, Hematoporphyrin monomethyl ether enhances the killing of ultrasound on osteosarcoma cells involving intracellular reactive oxygen species and calcium ion elevation, Integr. Cancer Ther., 2010, 9, 365–369 CrossRef CAS PubMed.
- M. Shams, B. Owczarczak, P. Manderscheid-Kern, D. A. Bellnier and S. O. Gollnick, Development of photodynamic therapy regimens that control primary tumor growth and inhibit secondary disease, Cancer Immunol. Immunother., 2015, 64, 287–297 CrossRef CAS PubMed.
- S. Y. Zhang, J. L. Li, X. K. Xu, M. G. Zheng, C. C. Wen and F. C. Li, HMME-based PDT restores expression and function of transporter associated with antigen processing 1 (TAP1) and surface presentation of MHC class I antigen in human glioma, J. Neurooncol., 2011, 105, 199–210 CrossRef CAS PubMed.
- P. Wang, F. Qin, L. Wang, F. Li, Y. Zheng, Y. Song, Z. Zhang and W. Cao, Luminescence and photosensitivity of gadolinium labeled hematoporphyrin monomethyl ether, Opt. Express, 2014, 22, 2414–2422 CrossRef PubMed.
- Y. Sun, D. Xing, L. Shen, M. Sun, M. Fang, L. Bi, Y. Sui, Z. Zhang and W. Cao, Bactericidal effects of hematoporphyrin monomethyl ether-mediated photosensitization against pathogenic communities from supragingival plaque, Appl. Microbiol. Biotechnol., 2013, 97, 5079–5087 CrossRef CAS PubMed.
- L. Liu, Y. Song, L. Ma, L. Zang, L. Tao, Z. Zhang and J. Han, Growth inhibition effect of HMME-mediated PDT on hepatocellular carcinoma HepG2 cells, Lasers Med. Sci., 2014, 29, 1715–1722 CrossRef PubMed.
- H. Zeng, M. Sun, C. Zhou, F. Yin, Z. Wang, Y. Hua and Z. Cai, Hematoporphyrin monomethyl ether-mediated photodynamic therapy selectively kills sarcomas by inducing apoptosis, PLoS One, 2013, 8, e77727 CrossRef CAS PubMed.
- T. Dai, V. J. Bil de Arce, G. P. Tegos and M. R. Hamblin, Blue dye and red light, a dynamic combination for prophylaxis and treatment of cutaneous Candida albicans infections in mice, Antimicrob. Agents Chemother., 2011, 55, 5710–5717 CrossRef CAS PubMed.
- T. M. Baran, B. R. Giesselman, R. Hu, M. A. Biel and T. H. Foster, Factors influencing tumor response to photodynamic therapy sensitized by intratumor administration of methylene blue, Lasers Surg. Med., 2010, 42, 728–735 CrossRef PubMed.
-
C. H. Tang and S. G. Sheng, et al., Measurement of labial soft tissue thickness in the Maxillary anterior regin with cone-beam computed tomography, in The tenth national periodontics academic conference papers in assembly, 2014 Search PubMed.
- W. L. Dong, Y. H. Zhou, C. Z. Li, H. Liu, S. H. Shang and B. Q. Pan, Establishment and application of an intact natural model of human dental plaque biofilm, Shanghai Kouqiang Yixue, 2010, 19, 196–201 Search PubMed.
- A. B. Uzdensky, The biophysical aspects of photodynamic therapy, Biophysics, 2016, 61, 461–469 CrossRef CAS.
- A. Kurek, A. M. Grudniak, A. Kraczkiewicz-Dowjat and K. I. Wolska, New antibacterial therapeutics and strategies, Pol. J. Microbiol., 2011, 60, 3–12 CAS.
- P. Wang, F. Qin, Z. Zhang and W. Cao, Quantitative monitoring of the level of singlet oxygen using luminescence spectra of phosphorescent photosensitizer, Opt. Express, 2015, 23, 22991–23003 CrossRef CAS PubMed.
- M. Wilson and J. Pratten, Lethal photosensitisation of Staphylococcus aureus, Microbios, 1994, 78, 163–168 CAS.
- X. Li, H. Guo, Q. Tian, G. Zheng, Y. Hu, Y. Fu and H. Tan, Effects of 5-aminolevulinic acid-mediated photodynamic therapy on antibiotic-resistant staphylococcal biofilm: an in vitro study, J. Surg. Res., 2013, 184, 1013–1021 CrossRef CAS PubMed.
- M. Sharma, L. Visai, F. Bragheri, I. Cristiani, P. K. Gupta and P. Spezialeet, Toluidine Blue-Mediated Photodynamic Effects on Staphylococcal Biofilms, Antimicrob. Agents Chemother., 2008, 52, 299–305 CrossRef CAS PubMed.
- M. Grinholc, B. Szramka, K. Olender and A. Graczyk, Bactericidal effect of photodynamic therapy against methicillin-resistant Staphylococcus aureus strain with the use of various porphyrin photosensitizers, Acta Biochim. Pol., 2007, 54, 665–670 CAS.
- K. Lasocki, M. Szpakowska, J. Grzybowski and A. Graczyk, Examination of antibacterial activity of the photoactivated arginine haematoporphyrin derivative, Pharmacol. Res., 1999, 39, 181–184 CrossRef CAS PubMed.
- M. Szpakowska, K. Lasocki, J. Grzybowski and A. Graczyk, Photodynamic activity of the haematoporphyrin derivative with rutin and arginine substituents (HpD-Rut(2)-Arg(2)) against Staphylococcus aureus and Pseudomonas aeruginosa, Pharmacol. Res., 2001, 44, 243–246 CrossRef CAS PubMed.
Footnote |
† These authors contributed equally to this work. |
|
This journal is © The Royal Society of Chemistry and Owner Societies 2019 |