DOI:
10.1039/C8NJ04689A
(Paper)
New J. Chem., 2019,
43, 111-114
Preparation of a novel polyfunctional and high adsorption capacity adsorbent by laccase-catalyzed self-polymerization of GAL and cross-linking with PEI
Received
15th September 2018
, Accepted 1st November 2018
First published on 6th November 2018
Abstract
A novel polyfunctional and high adsorption capacity adsorbent was prepared by laccase-catalyzed self-polymerization of GAL and cross-linking with PEI. When the mass ratio of GAL and PEI is 2
:
1, the self-polymerization temperature, pH and time are 50 °C, 4.5 and 24 h respectively, and the cross-linking temperature, pH and time are 70 °C, 8–9 and 24 h respectively, the PGAL–PEI has an excellent adsorption capacity for metal ions. The absorbed amounts reached 159 mg g−1, 88.23 mg g−1, 320.8 mg g−1, 511.36 mg g−1, 234.2 mg g−1 and 174.8 mg g−1 for Zn(II), Fe(III), Cu(II), Pb(II), Cd(II) and Cr(III) respectively at 25 °C and pH = 5. The stability experiment showed that PGAL–PEI has good stability under acidic conditions. These findings suggest that PGAL–PEI has considerable potential for application in metal ion removal from waste water.
1. Introduction
As a kind of conventional water pollutant, heavy metal ions are characterized by their high toxicity and inability to degrade, and have become a serious hazard in water pollutants. With the development of industry, pollution by heavy metals has become increasingly prominent, and adsorption methods have been developed. The interaction mechanisms between heavy metal ions and adsorbents are mainly surface complexation, π–π stacking and electrostatic interactions, and through these bonds, bind together to remove the pollutants.1–4 The adsorption method has the advantages of cheap materials, easy operation, and good heavy metal treatment, and has been paid much attention by researchers.
Polyethyleneimine (PEI) has attracted particular attention as an adsorbent in recent years. PEI molecules have a large amount of primary, secondary and tertiary amine groups and have higher adsorption capacity and adsorption selectivity for metal ions,5–9 and amino groups have good reactivity, can be easily functionalized and modified,10,11 and have a wide range of applications. In addition, PEI is biocompatible and belongs to a new type of environmentally friendly adsorbent. As an adsorbent, due to the PEI molecule being able to dissolve in the aqueous phase, there are many drawbacks, such as difficulty in operation, difficulty in separation and recovery and easy loss. This restricts the application of PEI as an adsorbent material in the environment. The solution to this problem is to mount PEI on matrix materials,12–15 or to modify it by grafting or cross-linking16–18 to form a morphology and material more suitable for the treatment of environmental pollution.
Gallic acid (GAL) is a natural polyphenol material, originating from gallic tannin hydrolysis. Poly(gallic acid) (PGAL) was successfully synthesized by Trametes versicolor laccase-mediated oxidative polymerization of the naturally abundant GAL.19 The molecule of PGAL contains a large number of phenolic hydroxyl groups and carboxyl groups. It can also be used as a metal ion adsorbent. However, it can also dissolve in the aqueous phase. During the synthesis, some of the phenolic groups are oxidized to form benzoquinone, and the carbonyl groups can react with primary amines via Schiff base reactions under alkaline conditions to form a water-insoluble and three-dimensional network structure.20
Considering the large number of amino groups in the structure of PEI, the large number of benzene hydroxyl and carboxyl groups in the structure of PGAL and this group’s adsorption ability of PEI and PGAL, a novel and facile process to prepare PGAL–PEI via the laccase-catalyzed self-polymerization of GAL and cross-linking with PEI is proposed. The prepared PGAL–PEI powders were used as adsorbents for the adsorption of Zn(II), Fe(III), Cu(II), Pb(II), Cd(II) and Cr(III). Then, the stability of PGAL–PEI was tested.
2. Experimental
2.1 Synthesis of PGAL–PEI
Materials.
All chemicals were of analytical grade and used as received without further purification. The possible reaction mechanism for the synthesis of PGAL–PEI is illustrated in Fig. 1. GAL and PEI were dissolved in a sodium acetate/acetic acid buffer solution (0.2 M, pH = 4.5), and the mass ratio is 2
:
1. 50 mg of laccase was added to the solution mixture, which was stirred at 50 °C for 24 h. Then, the pH of the reaction system was adjusted to 8–9 using 2 M NaOH aqueous solution, and stirred at 70 °C for 24 h. Finally, the products were filtered, and dried at 50 °C for 8 h in a vacuum oven.
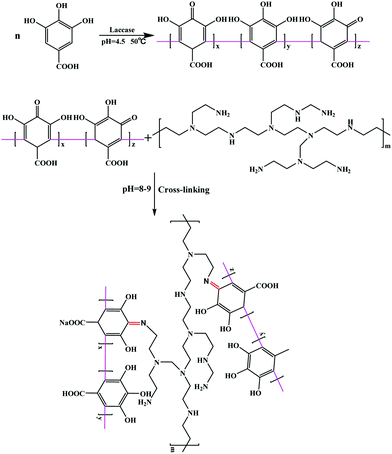 |
| Fig. 1 The possible reaction mechanism for the synthesis of the PGAL–PEI composite. | |
2.2 Characterization
The obtained products were characterized using scanning electron microscopy (FE-SEM, Hitachi S-4800, Japan) and X-ray photoelectron spectroscopy (XPS, PerkinElmer PHI 5000C ESCA System with Mg/Al Dual Anode Hel/Hell ultraviolet source, Kratos, XSAM800, UK)
2.3 Adsorption test
50 mg of PGAL–PEI was added to aqueous metal ion solutions of Zn(II), Fe(III), Cu(II), Pb(II), Cd(II) and Cr(III) at starting concentrations of 200 mg L−1. The solution system pH was adjusted to 5, and the mixture was agitated in a thermo shaker for 18 h at 25 °C. Afterwards, the solution mixture was filtered using a 0.2 μm water filtration membrane, and the amount of remaining metal ion concentration was measured using an atomic absorption spectrometer (Varian, SpectrAA-100, USA).
The adsorption capacity for metal ions on PGAL–PEI in the equilibrium, qe (mg g−1), was calculated as follows:
| 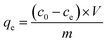 | (1) |
where
V is the volume of the solution and
m is the mass of adsorbent used in this study. The variables
c0 (mg L
−1) and
ce (mg L
−1) are the initial and equilibrium concentrations of metal ions, respectively.
2.4 Stability test
0.1 g PGAL–PEI powders were soaked in 100 ml of aqueous solutions with different pH values and ethanol at 25 °C for 24 h. Then, the solutions were filtered using a 0.2 μm water filtration membrane, the filter cake was dried at 50 °C in a vacuum oven, and the yield was calculated.
3. Results and discussion
The elemental composition of the PGAL–PEI was revealed using XPS spectroscopy. The XPS wide scan spectrum of PGAL–PEI is shown in Fig. 2a, and the peaks corresponding to C 1s (binding energy of 284.69 eV), N 1s (binding energy of 399.6 eV), O 1s (binding energy of 531.5 eV) and Na 1s (binding energy of 1071.08 eV) are presented in the spectrum. Six components at 284.32, 284.86, 285.38, 285.90, 286.46 and 287.86 eV in the C 1s region were observed for PGAL–PEI, which corresponded to C–C/C
C, C–N, C
N, C–OH, C–O and C
O, respectively. This is consistent with the chemical characteristics of PGAL–PEI as shown in Fig. 2b.
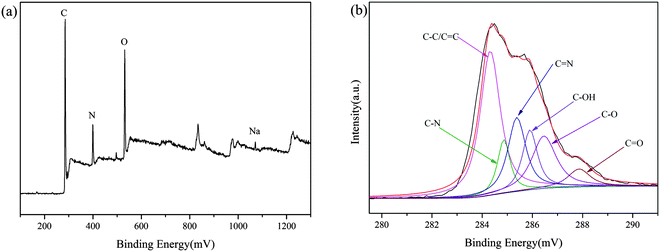 |
| Fig. 2 XPS wide scan survey spectra of PGAL–PEI (a) and C 1s (b). | |
FESEM was employed to characterize the surface morphology of the adsorbent (Fig. 3). It can be found that the PGAL–PEI powder particles prepared from the aqueous solution are micro- to nano-sized and the surface of the particles is uneven. This structural surface is very similar to silkworm cocoons, and greatly increases the specific surface area of the particles, thereby increasing the adsorption capacity for metal ions.
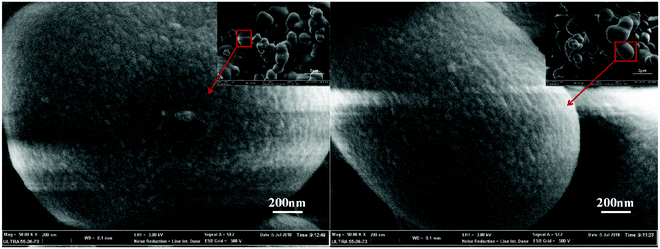 |
| Fig. 3 SEM images of PGAL–PEI powder. | |
In order to evaluate the adsorptivity of PGAL–PEI for metal ions from aqueous solutions, batch adsorption experiments were conducted. The results are summarized in Fig. 4(a). It can be clearly seen that PGAL–PEI has an excellent adsorption capacity for metal ions. For example, the adsorption capacities are 159 mg g−1, 88.23 mg g−1, 320.8 mg g−1, 511.36 mg g−1, 234.2 mg g−1 and 174.8 mg g−1 for Zn(II), Fe(III), Cu(II), Pb(II), Cd(II) and Cr(III), respectively. There are three main reasons for this phenomenon. Firstly, the amino, phenolic hydroxyl and carboxyl groups have strong complexation abilities to metal ions. These groups will form ligand bonds with heavy metal ions through the complexation reaction.21,22 Secondly, this structure of PGAL–PEI is three-dimensional and the surface is very similar to silkworm cocoons, leading to the specific surface area being larger and the adsorption site being increased, so resulting in a higher adsorption amount.23,24 Thirdly, different adsorption performances for metal ions are because of the varying ionic radius and binding energy. The acid, alkali and alcohol resistance of PGAL–PEI are shown in Fig. 4(b). It can be found that the PGAL–PEI is stable in strong acid, weak alkali and ethanol. However, when the aqueous solution pH is greater than 11, PGAL–PEI begins to decompose and dissolve with water. The main reason for this is that the C
N bond breaks under strong alkali conditions and some water-soluble molecules are formed, resulting in a reduced recovery rate. However, the metal ions in waste-water can only be stable under acidic conditions and precipitate under alkaline conditions, therefore, the prepared adsorbent would have potential application prospects in the fields of waste-water treatment and metal ion recovery.
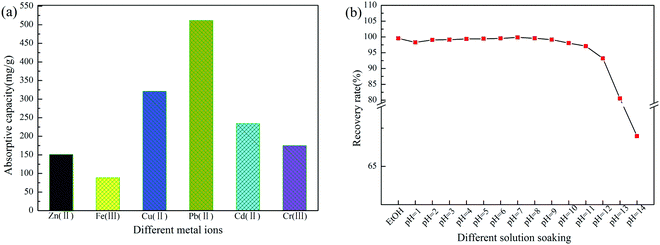 |
| Fig. 4 Adsorption capacity (a) and stability (b) of PGAL–PEI. | |
4. Conclusions
In summary, a novel and facile method was developed to prepare the adsorbent PGAL–PEI via the laccase-catalyzed self-polymerization of GAL cross-linked with PEI. This adsorbent has a high adsorption efficiency, excellent acid resistance stability and a good application structure. It has potential application prospects in the fields of waste-water treatment and metal ion recovery.
Conflicts of interest
There are no conflicts to declare.
Acknowledgements
We gratefully thank the National Natural Science Foundation of China (grant no. 21706238) and the Fund for the Excellent Postgraduate Thesis Program of Zhejiang Sci-Tech University (grant no. 2018-XWLWPY-B-03-07).
References
- S. S. Yu, X. X. Wang, H. W. Pang, R. Zhang, W. C. Song, D. Fu, T. Hayat and X. K. Wang, Chem. Eng. J., 2018, 333, 343–360 CrossRef CAS.
- G. X. Zhao, X. B. Huang, Z. W. Tang, Q. F. Huang, F. L. Niu and X. K. Wang, Polym. Chem., 2018, 9, 3562–3582 RSC.
- J. Lei, X. X. Wang, G. X. Zhao, C. L. Chen, Z. F. Chai, A. Alsaedi, T. Hayat and X. K. Wang, Chem. Soc. Rev., 2018, 47, 2322–2356 RSC.
- P. C. Gu, S. Zhang, X. Li, X. X. Wang, T. Wen, R. Jehan, A. Alsaedi, T. Hayat and X. K. Wang, Environ. Pollut., 2018, 240, 493–505 CrossRef CAS.
- M. Chanda, A. Sarkar and Z. W. Chen, J. Polym. Mater., 2004, 21, 21–29 CAS.
- K. Yamada, Y. Saitoh, Y. Haga, K. Matsuda and M. Hirata, J. Appl. Polym. Sci., 2006, 102, 5965–5976 CrossRef CAS.
- R. Molinari, P. Argurio and T. Poerio, Desalination, 2004, 162, 217–228 CrossRef CAS.
- R. Molinari, T. Poerio and P. Argurio, J. Membr. Sci., 2007, 295, 139–147 CrossRef CAS.
- S. S. Abolmaali, A. Tamaddon, H. Najafi and R. Dinarvand, J. Inorg. Organomet. Polym. Mater., 2014, 24, 977–987 CrossRef CAS.
- P. E. Duru, S. Bektas, S. Patir and A. Denizli, J. Appl. Polym. Sci., 2001, 81, 197–205 CrossRef CAS.
- R. Say, A. Tuncel and A. Denizli, J. Appl. Polym. Sci., 2002, 83, 2467–2473 CrossRef CAS.
- M. Owlad, M. Aroua and W. Daud, Bioresour. Technol., 2010, 101, 5098–5103 CrossRef CAS PubMed.
- C. Y. Yin, M. K. Aroua and M. A. W. D. Wan, Colloids Surf., A, 2007, 307, 128–136 CrossRef CAS.
- M. Chen, Y. Lin, C. Gu and J. Wang, Talanta, 2013, 104, 53–57 CrossRef CAS PubMed.
- B. Gao, F. An and K. Liu, Appl. Surf. Sci., 2006, 253, 1946–1952 CrossRef CAS.
- Z. Chen, M. Deng, Y. Chen and G. He, J. Membr. Sci., 2004, 235, 73–86 CrossRef CAS.
- A. Wu, J. Jia and S. Luan, Colloids Surf., A, 2011, 384, 180–185 CrossRef CAS.
- S. Inphonlek, N. Pimpha and P. Sunintaboon, Colloids Surf., B, 2010, 77, 219–226 CrossRef CAS PubMed.
- J. López, J. M. Hernández-Alcántara, P. Roquero, C. Montiel and K. Shirai, J. Mol. Catal. B: Enzym., 2013, 97, 100–105 CrossRef.
- H. Wang, J. Wu, C. Cai, J. Guo, H. Fan, C. Zhu, H. Dong, N. Zhao and J. Xu, ACS Appl. Mater. Interfaces, 2014, 6, 5602–5608 CrossRef CAS PubMed.
- S. J. Yu, Y. Liu, Y. J. Ai, X. X. Wang, R. Zhang, Z. S. Chen, Z. Chen, G. X. Zhao and X. K. Wang, Environ. Pollut., 2018, 242, 1–11 CrossRef CAS PubMed.
- P. C. Gu, J. L. Xing, T. Wen, R. Zhang, J. Wang, G. X. Zhao, T. Hayat, Y. J. Ai, Z. Lin and X. K. Wang, Environ. Sci.: Nano, 2018, 5, 946–955 RSC.
- A. Khan, J. L. Xing, A. M. Elseman, P. C. Gu, K. Gul, Y. J. Ai, R. Jehan, A. Alsaedi, T. Hayat and X. K. Wang, Dalton Trans., 2018, 47, 11327–11336 RSC.
- X. X. Wang, Y. Liu, H. W. Pang, S. J. Yu, Y. J. Ai, X. Y. Ma, G. Song, T. Hayat, A. Alsaedi and X. K. Wang, Chem. Eng. J., 2018, 344, 380–390 CrossRef CAS.
|
This journal is © The Royal Society of Chemistry and the Centre National de la Recherche Scientifique 2019 |