DOI:
10.1039/C8NA00192H
(Paper)
Nanoscale Adv., 2019,
1, 1181-1185
Direct aerobic oxidation of alcohols into esters catalyzed by carbon nanotube–gold nanohybrids†
Received
4th September 2018
, Accepted 24th December 2018
First published on 28th December 2018
Abstract
Gold nanoparticles supported on carbon nanotubes were shown to efficiently catalyze the oxidation of alcohols to methyl esters under mild and selective reaction conditions. The reaction works with low catalyst loadings and the nanohybrid could be readily recycled and reused.
Introduction
The selective oxidation of alcohols, under mild conditions, is of practical importance in synthetic chemistry. In addition to classical stoichiometric processes involving, for example, transition metals, hypervalent iodine reagents, or sulfoxides (Swern reaction), catalytic systems have been devised to perform such transformations.1 However, an ideal oxidation reaction should involve a highly active and selective recyclable catalyst able to work under benign conditions such as atmospheric pressure, room temperature, and using molecular oxygen from air as the sole oxidant. Our group has long been involved in the design of catalytic nanohybrids made by the assembly of metallic nanoparticles on carbon nanotubes (CNTs).2 In fact, nanostructured carbon allotropes such as CNTs have emerged as promising supports that provide unique advantages including stability, inertness, high specific surface area, and chemically tunable topography.3 In addition, CNTs act as synergistic platforms capable of enhancing the performances of the supported catalytic metal.4 For example, metallic gold nanoparticles on carbon nanotubes (AuCNT) were used by us in the heterogeneous catalysis of various organic transformations,5 including the aerobic oxidation of alcohols into either the corresponding aldehydes or carboxylic acids.6 The latter transformation was selective depending on the solvent mixture, as exclusive formation of aldehydes was observed in pure organic solvent whereas carboxylic acids were produced in a binary organic/aqueous mixture (e.g. toluene/water). The formation of carboxylic acid is likely going through the intermediacy of an aldehyde which, in the presence of water, becomes hydrated before being further oxidized by AuCNT (Scheme 1, path A). With these results in mind, we conceived that replacing water by an alcoholic solvent such as methanol could provide direct access to methyl esters, via the formation of a transient hemi-acetal intermediate derived from the initial aldehyde product (path B). The hemi-acetal would then further react with AuCNT, under aerobic conditions, and evolve into the corresponding ester, as shown by others.7
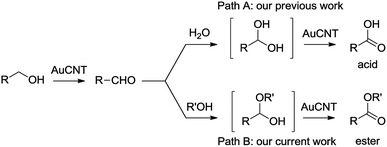 |
| Scheme 1 Pathways to the AuCNT-catalyzed oxidation of alcohols to acids or esters. | |
Results and discussion
Assembly of the nanohybrids
The AuCNT nanohybrids were assembled by a layer-by-layer approach using our previously reported procedure.8 Briefly, carbon nanotubes were dispersed in the presence of an amphiphilic nitrilotriacetic-diyne (DANTA) surfactant in water. This step led to the coating of the nanotubes with supramolecular structures having a ring shape. While the hydrophobic chains of DANTA were adsorbed at the surface of the CNTs by hydrophobic interactions, anionic hydrophilic heads were oriented towards the aqueous medium. The rings were subsequently polymerized by UV irradiation of the diacetylene units incorporated in the hydrophobic chains. This step permitted to reinforce the cohesion of the assembly around the CNT.9 In a second step, a polycationic polymer (PDADMAC) was deposited on the primary coating and ca. 3 nm gold nanoparticles (AuNP) were added to afford the final nanohybrid (AuCNT, Fig. 1) as an aqueous suspension. XPS analysis confirmed the metallic nature of gold and its concentration was measured by ICP-MS ([Au] = 5.4 mM).
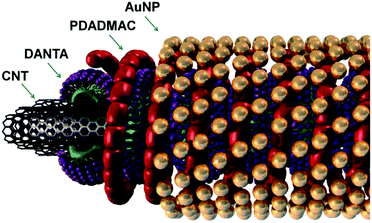 |
| Fig. 1 Illustration of the supramolecular assembly of gold nanoparticles on carbon nanotubes by a layer-by-layer approach. | |
Optimization of the oxidative esterification conditions
With the AuCNT nanohybrid in hands, we next looked at its performances in the aerobic conversion of alcohols into esters using methanol as a solvent. Anisyl alcohol (4-methoxy benzyl alcohol) 1a was selected as a model substrate and reacted with AuCNT under various conditions (Table 1). All reactions were conducted at room temperature. While the catalytic transformation was not efficient in the absence of a base (entry 1), the addition of potassium carbonate (entry 2) or cesium carbonate (entry 3) permitted to trigger the oxidation of 1a albeit with slow kinetics. In fact, ester 2a was efficiently produced in 70% yield in the presence of Cs2CO3 in MeOH but after 4 days of reaction. Reaction times could be improved by replacing carbonated bases with sodium hydroxide, as in the latter case methyl ester 2a was produced in 85% yield after 48 h at room temperature and under air (entry 4). The aerobic nature of the transformation was demonstrated by working under an inert atmosphere of nitrogen (entry 5) which fully inhibited the oxidative transformation. Replacing the methanolic solvent by a THF/MeOH mixture also prevented any conversion (entry 6), and no reaction occurred in the absence of the AuCNT catalyst (entry 7).
Table 1 Optimization of the AuCNT-catalyzed oxidative esterification of anisyl alcohol 1aa

|
Entry |
Conditions |
Time (d) |
Yieldb (%) |
Reaction conditions: 1a (0.1 mmol), AuCNT (0.54 mol%), solv. (1 mL), conds.
Yield of isolated product.
No catalyst added.
No reaction.
|
1 |
No base, MeOH, air |
2 |
nrd |
2 |
K2CO3, MeOH, air |
4 |
56 |
3 |
Cs2CO3, MeOH, air |
4 |
70 |
4 |
NaOH, MeOH, air |
2 |
85 |
5 |
NaOH, MeOH, N2 |
2 |
nrd |
6 |
NaOH, MeOH/THF, air |
2 |
nrd |
7c |
NaOH, MeOH, air |
2 |
nrd |
The direct esterification of 1a was attempted with other aliphatic alcohols as solvents and under the conditions of entry 4 (Table 1), but the use of ethanol, propanol or butanol instead of the conventional methanol led to an incomplete process which stopped at the anisaldehyde stage (primarily oxidized product), with no further conversion to the ester.
Exemplification of the AuCNT catalyzed reaction
The optimized reaction conditions (air, methanol, room temp., AuCNT, NaOH) were then applied to other substrates to try to find out the scope and limitations of the newly developed process. Several alcohols were thus treated under the above conditions (Table 2). The reaction was successfully extended to aromatic alcohols substituted with electron donating groups (entries 2 and 3) which were smoothly converted to the corresponding methyl esters in excellent yields (92 and 87%, respectively). Simple benzyl alcohol (entry 4), 4-methyl benzyl alcohol (entry 5), and 4-phenyl benzyl alcohol (entry 6) also reacted smoothly with however slower kinetics (3–4 days). The same comment applies to conjugated cinnamyl alcohol (entry 7) and 2-amino benzyl alcohol (entry 8) which were converted to esters 2g and 2h after 4 days of reaction in satisfactory 76 and 70% yields, respectively. In the latter case it is to be noted that the mildness of the oxidizing conditions left intact the oxidizable amino group of 1h, as only alcohol oxidation was observed. Noteworthy, we detected in some cases trace amounts (<5% yield) of carboxylic acids originating from residual water in the solvent. Although efficient on aromatic substrates substituted with electron donating or “neutral” groups, the nanohybrid-mediated oxidation was not successful on substrates substituted with electron withdrawing groups as no significant conversion of 4-fluoro benzyl alcohol (entry 9) or 3-nitro benzyl alcohol (entry 10) was observed after 4 days of reaction. The same holds true for an aliphatic alcohol (e.g. 3-phenyl-1-propanol, entry 11), propargylic 2-nonyn-1-ol (entry 12) and allylic phytol (entry 13) which failed to react under our reaction conditions.
Table 2 Scope of the AuCNT-promoted oxidative esterification of alcohols 1a
The lack of reactivity of substrates bearing electron withdrawing groups and of an aliphatic alcohol was disappointing and was attributed to the demanding oxidation towards the aldehyde which is likely the rate limiting step. To confirm this hypothesis, the oxidation/esterification sequence was attempted starting from the corresponding aldehydes. Thus 4-fluoro (Table 3, entry 1) and 3-nitro benzaldehyde (entry 2) were reacted with AuCNT under the optimized reaction conditions in methanol. In these cases, we were pleased to observe the clean formation, after 15 h, of methyl esters 2i and 2j from aldehydes 3i and 3j, respectively. As a reminder, no ester was produced starting the corresponding alcohols 1i and 1j.
Table 3 AuCNT-catalyzed oxidation of aldehydes 3a
Attempts to convert aliphatic aldehyde 3l into the corresponding ester was not successful as the reaction gave a complex mixture likely originating from the deprotonation of the acidic α-protons of the aldehyde, and further side reactions.
Proposed mechanism
Based on previous reports,10 we suggest that the AuCNT-catalyzed oxidative esterification of alcohols proceeds though three major steps: (i) deprotonation of the alcohol and formation of a gold-alcoholate intermediate with concomitant adsorption of oxygen onto the gold surface, (ii) dehydrogenation of the activated alcohol to the corresponding aldehyde‡ by NaOH with the release of hydrogen peroxide (or decomposed hydrogen peroxide), and (iii) hemi-acetalisation of the newly formed aldehyde by methanol followed by reiteration of step (ii) on the hemi-acetal intermediate to deliver the expected methyl ester (Scheme 2).
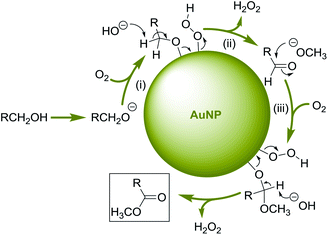 |
| Scheme 2 Proposed mechanism for the oxidative esterification of alcohols. | |
Recycling experiments
Recyclability of the AuCNT catalyst under our working conditions was evaluated over four consecutive oxidation/esterification reactions (Table 4). After each cycle, the catalyst was isolated from the mixture by mild centrifugation, washed with MeOH, and reused in the next run. It is to be noted that we observed a moderate deactivation of the catalyst over the consecutive runs which was evidenced by a slight increase in the reaction time needed to reach ca. 85% conversion. The origin of the deactivation is not yet understood as ICP-MS analysis of the mother liquors showed no leaching of gold, and transmission electron microscopy (TEM) indicated no alteration of supported catalyst.
Table 4 Recycling of AuCNTs for the conversion of 1a to 2aa

|
Cycle |
Time (h) |
Yieldb (%) |
Reaction conditions: 1a (0.1 mmol), AuCNTs (0.54 mol%), NaOH (0.15 mmol), MeOH (1 mL), air, room temp.
Yield of isolated product. The catalyst can be considered as being deactivated after the 8th run as the ester formation was no longer detected after 96 h.
71% yield at 48 h.
57% yield at 48 h.
|
Fresh |
48 |
85 |
1 |
48 |
85 |
2 |
60 |
84c |
3 |
72 |
81d |
Experimental
General procedure for synthesis of methyl esters 2 from alcohols 1 or aldehydes 3
100 μL of a 5.4 mM aqueous suspension of AuCNT (0.54 mol%) was first washed twice with dry methanol, centrifuged and the supernatant was discarded. The pellet was redispersed in 1 mL of dry methanol and added to a stirred solution of alcohol 1 or aldehyde 3 (0.1 mmol) and NaOH (0.15 mmol, 1.5 equiv.). The reaction mixture was stirred at room temperature under air until complete consumption of the starting material (as monitored by TLC). Solvent was then evaporated and the crude product was directly purified by silica gel column chromatography.
Conclusions
We showed that our AuCNT hybrid acted as an efficient catalyst for the aerobic oxidation of alcohol to methyl esters in good to excellent yields under very mild conditions. The catalytic system operates with low catalytic loadings (0.54 mol%), at room temperature, under atmospheric pressure of air (no added molecular oxygen), and the catalyst could be recycled and reused for subsequent oxidations. Alcohols and aldehydes were chemoselectively oxidized to the corresponding methyl esters. The CNT-based nanohybrid thus compares favorably to other catalytic systems in terms of recyclability, activity, selectivity, and mildness of the operating conditions.
Conflicts of interest
There are no conflicts to declare.
Acknowledgements
Elumalai Gopi thanks the “Enhanced Eurotalents” program for funding. The “Service de Chimie Bioorganique et de Marquage” belongs to the Laboratory of Excellence in Research on Medication and Innovative Therapeutics (ANR-10-LABX-0033-LERMIT).
Notes and references
-
(a) C. Parmeggiani and F. Cardona, Green Chem., 2012, 14, 547 RSC;
(b) Z. Guo, B. Liu, Q. Zhang, W. Deng, Y. Wang and Y. Yang, Chem. Soc. Rev., 2014, 43, 3480 RSC;
(c) A. S. Sharma, H. Kaur and D. Shah, RSC Adv., 2016, 6, 28688 RSC.
-
(a) N. Mackiewicz, G. Surendran, H. Remita, B. Keita, G. Zhang, L. Nadjo, A. Hagège, E. Doris and C. Mioskowski, J. Am. Chem. Soc., 2008, 130, 8110 CrossRef CAS PubMed;
(b) D. V. Jawale, E. Gravel, C. Boudet, N. Shah, V. Geertsen, H. Li, I. N. N. Namboothiri and E. Doris, Chem. Commun., 2015, 51, 1739 RSC;
(c) D. V. Jawale, E. Gravel, C. Boudet, N. Shah, V. Geertsen, H. Li, I. N. N. Namboothiri and E. Doris, Catal. Sci. Technol., 2015, 5, 2388 RSC;
(d) D. V. Jawale, E. Gravel, N. Shah, V. Dauvois, H. Li, I. N. N. Namboothiri and E. Doris, Chem.–Eur. J., 2015, 21, 7039 CrossRef CAS PubMed;
(e) S. Donck, E. Gravel, N. Shah, D. V. Jawale, E. Doris and I. N. N. Namboothiri, ChemCatChem, 2015, 7, 2318 CrossRef CAS;
(f) S. Donck, E. Gravel, A. Li, P. Prakash, N. Shah, J. Leroy, I. N. N. Namboothiri and E. Doris, Catal. Sci. Technol., 2015, 5, 4542 RSC;
(g) P. Prakash, V. Geertsen, F. Miserque, I. N. N. Namboothiri, E. Gravel and E. Doris, ChemistrySelect, 2017, 2, 5891 CrossRef CAS.
-
(a) D. Tasis, N. Tagmatarchis, A. Bianco and M. Prato, Chem. Rev., 2006, 106, 1105 CrossRef CAS PubMed;
(b) J. John, E. Gravel, I. N. N. Namboothiri and E. Doris, Nanotechnol. Rev., 2012, 1, 515 CAS.
- G. Rahman, D. Guldi, E. Zambon, L. Pasquato, N. Tagmatarchis and M. Prato, Small, 2005, 1, 527 CrossRef CAS PubMed.
-
(a) R. Kumar, E. Gravel, A. Hagège, H. Li, D. Verma, I. N. N. Namboothiri and E. Doris, ChemCatChem, 2013, 5, 3571 CrossRef CAS;
(b) D. V. Jawale, E. Gravel, V. Geertsen, H. Li, N. Shah, I. N. N. Namboothiri and E. Doris, ChemCatChem, 2014, 6, 719 CrossRef CAS;
(c) D. V. Jawale, E. Gravel, V. Geertsen, H. Li, N. Shah, I. N. N. Namboothiri and E. Doris, Tetrahedron, 2014, 70, 6140 CrossRef CAS;
(d) N. Shah, E. Gravel, D. V. Jawale, E. Doris and I. N. N. Namboothiri, ChemCatChem, 2014, 6, 2201 CrossRef CAS;
(e) N. Shah, E. Gravel, D. V. Jawale, E. Doris and I. N. N. Namboothiri, ChemCatChem, 2015, 7, 57 CrossRef CAS;
(f) S. Donck, E. Gravel, N. Shah, D. V. Jawale, E. Doris and I. N. N. Namboothiri, RSC Adv., 2015, 5, 50865 RSC;
(g) P. Basu, N. Shah, P. Prakash, S. Donck, E. Gravel, I. N. N. Namboothiri and E. Doris, Nanomaterials, 2016, 6, 37 CrossRef PubMed;
(h) E. Gravel, I. N. N. Namboothiri and E. Doris, Synlett, 2016, 27, 1179 CrossRef CAS;
(i) P. Prakash, E. Gravel, H. Li, H. Y. Li, F. Miserque, A. Habert, M. den Hertog, W. L. Ling, I. N. N. Namboothiri and E. Doris, Catal. Sci. Technol., 2016, 6, 6476 RSC. For some transformations involving non-supported gold, see:
(j) S. Yamazoe, K. Koyasu and T. Tsukuda, Acc. Chem. Res., 2014, 47, 816 CrossRef CAS PubMed;
(k) C. Zhang, Y. Chen, H. Wang, Z. Li, K. Zheng, S. Li and G. Li, Nano Res., 2018, 11, 2139 CrossRef CAS.
- R. Kumar, E. Gravel, A. Hagège, H. Li, D. V. Jawale, D. Verma, I. N. N. Namboothiri and E. Doris, Nanoscale, 2013, 5, 6491 RSC.
-
(a) A. Abad, P. Concepcion, A. Corma and H. Garcia, Angew. Chem., Int. Ed., 2005, 44, 4066 CrossRef CAS PubMed;
(b) H. Miyamura, T. Yasukawa and S. Kobayashi, Green Chem., 2010, 12, 776 RSC;
(c) L. L. Chng, J. Yang and J. Y. Ying, ChemSusChem, 2015, 8, 1916 CrossRef CAS PubMed.
- J. John, E. Gravel, A. Hagège, H. Li, T. Gacoin and E. Doris, Angew. Chem., Int. Ed., 2011, 50, 7533 CrossRef CAS PubMed.
- C. Thauvin, S. Rickling, P. Schultz, H. Célia, S. Meunier and C. Mioskowski, Nat. Nanotechnol., 2008, 3, 743 CrossRef CAS PubMed.
-
(a) T. Mallat and A. Baiker, Chem. Rev., 2004, 104, 3037 CrossRef CAS PubMed;
(b) B. N. Zope, D. D. Hibbitts, M. Neurock and R. J. Davis, Science, 2010, 330, 74 CrossRef CAS PubMed;
(c) T. Tsukuda, H. Tsunoyama and H. Sakurai, Chem.–Asian J., 2011, 6, 736 CrossRef CAS PubMed;
(d) M. L. Personick, R. J. Madix and C. M. Friend, ACS Catal., 2017, 7, 965 CrossRef CAS.
Footnotes |
† Electronic supplementary information (ESI) available: Experimental procedures and copies of NMR spectra. See DOI: 10.1039/c8na00192h |
‡ Intermediacy of the transient aldehyde was confirmed by stopping the esterification reaction of 4-methoxy benzyl alcohol at 18 h which permitted to isolate 4-methoxy benzaldehyde (for NMR data, see ESI†). |
|
This journal is © The Royal Society of Chemistry 2019 |